Abstract
The use of tumor-specific monoclonal antibodies (MAbs) has revolutionize the field of cancer immunotherapy. Although treatment of malignant diseases with MAbs is promising, many patients fail to respond or relapse after an initial response. Both solid tumors and hematological malignancies develop mechanisms that enable them to evade the host immune system by usurping immune checkpoint pathways such as PD-1, PD-2, PDL-1, or PDL-2 (programmed cell death protein-1 or 2 and PD-Ligand 1 or 2), which are expressed on activated T cells and on T-regulatory, B cells, natural killers, monocytes, and dendritic cells. One of the most exciting anticancer development in recent years has been the immune checkpoint blockade therapy by using MAbs against immune checkpoint receptor and/or ligands. Anti-PD1 antibodies have been tested in clinical studies that included patients with hematological malignancies and showed remarkable efficacy in Hodgkin lymphoma (HL). In our review, we will focus on the effect of PD-1 activation on hematological malignancies and its role as a therapeutic target.
The programmed death 1 (PD1) immune checkpoint is an important homeostatic mechanism of the immune system that helps in preventing autoimmunity and uncontrolled inflammation in cases of chronic infections.
However, PD1 pathway is also operated by a wide variety of malignancies and represents one of the most important mechanisms by which tumor cells escape from the surveillance of the immune system.
Blocking of immune checkpoints by the use of monoclonal antibodies opened a new era in the field of cancer immunotherapy. Results from clinical trials are promising, and currently, this approach has been proven effective and safe in patients with solid tumors and hematological malignancies.
Key messages
Introduction
Activation of antigen-specific T cells is the final result of a complicated process mediated by the interaction with antigen-presenting cells (APCs) loaded with the respective antigen. APCs present antigenic peptides in the context of major histocompatibility antigens (MHC). The complex MHC–peptide is recognized by the T-cell receptor (TCR) of naive antigen-specific T cells, and this interaction represents signal-1 which is the most important mediator of activation. However, completion of activation occurs only in the presence of other costimulatory interactions between T cell and APC. CD28 is one of the most important costimulatory molecules implicated in the initial activation of the immune system. CD28 is expressed on the surface of naive CD4+ and CD8+ cells. Following binding of TCR to MHC–peptide complex, CD28 interacts with the cognate ligands present on the surface of APC and provides a strong costimulatory signal (signal 2) necessary for the activation of antigen-specific naive T cells. In general, successful T-cell activation requires the biochemical reactions mediated by signal 1 and signal 2 occurring concurrently. On the contrary, signal 1 in the absence of costimulation by signal 2 results in T-cell anergy, instead of activation (Citation1). Consistent with previous data, CD28-deficient mice are severely immune-suppressed due to impaired T-cell activation, resulting in lack of T-cell help for B cells and poor antibody and memory responses (Citation2). CD28 costimulation is mediated after receptor binding to CD80 (B7-1) and CD86 (B7-2) cognate ligands expressed on the surface of APCs (Citation3). Furthermore, upregulation of CD80 and CD86 expression occurs in the presence of inflammatory stimuli (Citation4).
Immune system homeostasis is orchestrated by the coordinated expression of stimulatory and inhibitory signals mediated mainly through interactions between the cell receptors and cognate ligands (Citation5). In addition to interaction with CD28, both CD80 and CD86 ligands bind also to inhibitory molecule CTLA-4. CTLA-4 is a CD28 homolog, expressed on activated T cells and regulatory T cells (Tregs) (Citation6,Citation7). CTLA-4 antagonizes CD28 for binding to cognate ligands providing the mechanism for controlling the immune response. Experimental data showed that CTLA-4 has a higher binding affinity for CD80 and CD86 as compared with CD28 (Citation8). Indeed, CTLA-4-deficient mice suffer from a lethal immune hyperactivation syndrome resulting in severe autoimmune reactions (Citation9).
Another important immune checkpoint is mediated by the programmed cell death protein 1 (PD1). PD1 is expressed on the surface of activated T cells during initial activation. However, PD1 regulates immune activity at a later stage, when effector T-cell tissue infiltration occurs. Besides activated T-cells, PD1 is expressed on the surface of various other immune cells such as (Citation1) activated B cells, (Citation2) natural killer cells (NK), and (Citation3) Tregs. Inflammatory cytokines secreted at the site of inflammation induce the expression of PD1 ligands. Two PD1 ligands have been identified so far, PD-L1 (CD274) and PD-L2 (PDCD1LG2 or CD273) (Citation10). PD-L1 protein is expressed on antigen-presenting cells, activated T cells as well as on a variety of other immune cells. On the other hand, PD-L2 expression is restricted mainly on macrophages, but it is also expressed on dendritic cells and B cells (Citation10). PD1 binding to its ligands helps in immune modulation by suppressing T-cell activity at the site of infection and therefore prevents excessive tissue damage and autoimmunity (Citation11,Citation12).
Rationale for the development of anti-PD1 blockade
The PD1 immune checkpoint has emerged as an important homeostatic mechanism of the immune system in cases of chronic infections such as HIV, hepatitis, and parasitic infections and in a variety of autoimmune diseases such as rheumatoid arthritis, inflammatory bowel disease, and diabetes mellitus type 1 (Citation13–16). However, PD1 pathway is also operated by a wide variety of malignancies and represents one of the most important mechanisms by which tumor cells escape from the surveillance of the immune system (Citation17). Expression of PD1 and PD1 ligands has been identified both on tumor cells and on cells infiltrating the tumor microenvironment. Interaction of PD1 expressed on tumor-infiltrating lymphocytes (TILs), with PD1 ligands expressed on tumor or other cells within the tumor microenvironment, results in local suppression of antitumor immunity (Citation18). The mechanism responsible for PD1 ligand expression by tumor cells remains largely unknown and is the focus of intensive research. In early 2000s, animal data showed that blocking PD1 immune checkpoint by using monoclonal antibodies (MAb) is effective in the treatment of many malignant disorders (Citation19,Citation20). The anti-tumor effect of immune checkpoint inhibition is shown in .
Figure 1. (A) CTLA4 expressed on tumor-specific T cell shows higher affinity for binding to CD80/CD86 ligands expressed on DC than CD28. The CTLA4/CD80/CD86 interaction inhibits T-cell activation. PD1 is also expressed on tumor-specific T cells and binds to PD-L1 ligand expressed on DC. The PD1/PD-L1 interaction inhibits T-cell activation. (B) Use of anti-CTLA4 and anti-PD1 Mabs to block immune checkpoints: CTLA4 is blocked by anti-CTLA4 and CD80/CD86 is released to bind with CD28. CD28/CD80/CD86 interaction activates T cell. PD1 is blocked by anti-PD1 and inhibition of PD1 checkpoint activates T cell. (C) PD1 is expressed on the surface of T cell after activation and binds to PD-L1 ligand expressed by tumor cell. The PD1/PD-L1 interaction inhibits the cytotoxic activity of T cell against the tumor cell. (D) Use of anti-PD1 MAb blocks the binding of PD1 to the ligand PD-L1. PD1/PD-L1 checkpoint blockade results in activation of T-cell cytotoxicity.
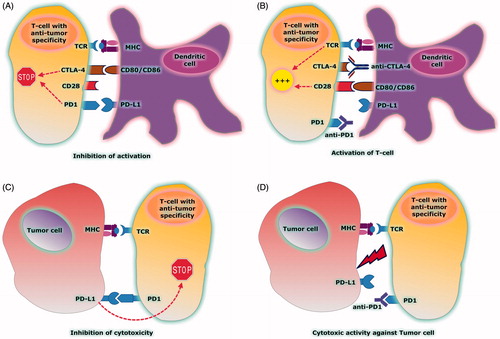
These data paved the way for clinical trials testing the feasibility and efficacy of PD1 blockade in the treatment of patients with solid tumors and hematological malignancies. The first MAb with a presumed anti-PD1 activity tested in human clinical trials was the humanized IgG1 monoclonal antibody CT-011 (pidilizumab) (Citation21). Pidilizumab showed promising antitumor activity when administered as consolidation therapy after autologous stem cell transplantation (auto-SCT) in patients with diffuse large B-cell (DLBCL) and primary mediastinal B-cell (PMBCL) lymphoma (Citation22). However, as of January 2016 the US Food and Drug Administration (FDA) put a hold on all pidilizumab trials, since it was discovered that pidilizumab is not an anti-PD1 antibody. Since March 2016, FDA has lifted the partial clinical hold on the Investigational New Drug (IND) application for pidilizumab in hematological malignancies and confirmed that the Phase-II clinical trial in patients with relapsed or refractory DLBCL, as well as other studies that cross reference the IND, may now proceed. Active research is currently ongoing to determine the compound's precise binding mechanism which probably modulates the body's innate immune response.
Topalian et al. assessed the safety and efficacy of the monoclonal anti-PD1 antibody BMS-936558 (nivolumab) in a large cohort of 296 patients with advanced cancer (melanoma, nonsmall cell lung, renal, colorectal, and castration-resistant prostate cancer). BMS-936558 was administered every two weeks in a dose escalation manner until disease progression or until achievement of complete response (CR). Objective responses were observed only in patients with nonsmall cell lung (18%), renal cell cancer (27%), and melanoma (28%). Surprisingly, many of these responses were durable, lasting for more than 1 year. Immunohistochemical analysis was performed for the assessment of PD-L1 expression by tumor cells. PD-L1 expression was predictive of response to treatment with no responder among the cohort of PD-L1-negative patients, while objective responses were observed in 36% of PD-L1-positive patients (Citation23).
Ipilimumab was the first immune checkpoint inhibitor (anti-CTLA4) approved by the FDA for the treatment of metastatic melanoma (Citation24). A few years later, nivolumab and pembrolizumab received approval for patients with B-raf (BRAF) gene unmutated or BRAF-mutated but ipilimumab-resistant melanoma (Citation25,Citation26).
Tumor microenvironment and prediction of response to anti-PD1 blockade
Malignant cells are surrounded by a reactive cellular background called tumor microenvironment (TME) and consisting of blood vessels, fibroblasts, tumor infiltrating lymphocytes (TILs), tumor associated macrophages, myeloid cells, cytokines, and extracellular matrix. There is a dynamic interplay between tumor cells and the surrounding cellular compartment. Malignant cells influence the composition and function of TME by releasing specific extracellular signals, promoting angiogenesis and recruiting immune suppressor cells. A large number of human studies, including patients with colorectal, breast, renal, lung cancer, and melanoma, have shown the significance of TILs as predictors of response to therapy and overall survival (Citation27). Inactivation of immune effectors with reactivity against the tumor occurs in the TME through interactions with immune checkpoint pathways. In this regard, binding of PD1 receptors to PD ligands expressed on the surface of malignant cells results in T-cell anergy and tumor escape from immune surveillance. Based on the theoretical background, response to anti-PD1 blockade is determined by the simultaneous occurrence of a significant number of immune effectors in the TME and the expression of PD-L1 on the tumor surface.
Depending on the presence or absence of TILs and the PD-L1 expression, tumors can be classified in four groups with different probabilities of response to immune checkpoint inhibition: (Citation1) Type I category includes tumors with PD-L1 expression and an abundant amount of TILs present in TME. (Citation2) Type-II category includes PD-L1-negative tumors with absence of TILs. (Citation3) Type-III category includes PD-L1-positive tumors without TILs, and (Citation4) Type-IV category includes PD-L1-negative tumors but with TILs present in TME. The proposed classification helps in identifying tumors with a high likelihood to respond to anti-PD1 blockade. Tumors of type-I category are the ideal candidates for anti-PD1 treatment, whereas other treatment approaches should be explored for patients with tumors of types II, III, and IV (Citation28).
However, the major limitation of this categorization is the fact that PD-L1 is potentially expressed not only on the surface of tumor cells but also on other nonmalignant immune cells present in TME. Therefore, PD-L1 expression on normal reactive cells surrounding the malignant cells may confer sensitivity to anti-PD1 treatment even in cases of PD-L1-negative tumors. Atezolizumab, a new monoclonal antibody with anti-PD-L1, specificity has shown promising activity against various types of cancers including nonsmall cell lung cancer (NSCLC). In a recent Phase-II randomized trial, patients with advanced relapsed/refractory NSCLC were randomized to receive atezolizumab or docetaxel chemotherapy. Patients were stratified according to the percentage of PD-L1-positive tumor-infiltrating immune cells. Patients in the atezolizumab arm had significantly improved survival compared with docetaxel. Response to treatment and prolongation of survival correlated with PD-L1 expression not only on tumor cells but also on tumor-infiltrating immune cells (Citation29).
Several genetic variants of the genes encoding for immune checkpoint pathway proteins exist in the general population. These variants are produced by single-nucleotide polymorphisms (SNPs). Certain polymorphisms have a major impact on T-cell activation and have been correlated with the development of autoimmune disorders (Citation30). CT60 is a SNP occurring in CTLA-4 gene and it is very important because previous studies have shown that affects the T-cell activation status. A recent study evaluated the effect of CT60 SNP on the outcome after donor lymphocyte infusion (DLI) in patients with acute leukemias after allogeneic stem cell transplantation. In multivariate analysis, the presence of a donor “AA” or “AG” CT60 genotype versus a “GG” genotype was an independent factor for improved DLI efficacy (Citation31). According to our knowledge, no study evaluating the correlation between certain PD-1/PD-L1 SNPs and response to anti-PD1 blockade has been published so far. However, such trials should be performed in the near future with the aim to identify biomarkers predictive of response to immune checkpoint inhibitors.
Methods of detection PD-L1 expression and limitations
PD-L1 is upregulated and expressed in various solid tumors and in hematological malignancies. PD1 and PD-L1 expression on cell surface can be detected by using immunohistochemistry (IHC). The prognostic significance of PD1 and PD-L1 as a marker predictive for response to treatment with PD1-axis blockade is a matter of controversy. Indeed, some of the previous trials in patients with solid tumors have shown remarkable efficacy independently of PD-L1 expression (Citation32). However, we should keep in mind that the existing data in the field of hematological malignancies are limited, and therefore, the predictive ability of PD-L1 expression is currently unknown. Reasons for the inability of PD-L1 expression to predict response after treatment with PD1/PD-L1 blockade are listed below: (i) PD-L1 is expressed not only on tumor cells but also on reactive lymphocytes infiltrating tumor microenvironment (TILs). TILs or other immune cells expressing PD-L1 may act as inhibitors of antitumor-specific lymphocytes (Citation33). (ii) PD1/PD-L1 expression is a dynamic process that occurs during treatment with various agents and therefore PD1/PD-L1 detection at a single time point should be interpreted with caution. (iii) PD1/PD-L1 expression is dependent of a sampling effect. Significant heterogeneity of PD1/PD-L1 expression has been observed in multiple biopsies from the same patient (Citation34). (iv) PD-L1 expression is also dependent on methodology and type of antibody used for IHC. (v) Finally, the reasonable cutoff level of expression for characterizing a case as positive or negative has not been defined yet. All these issues should be addressed in future clinical trials including patients with hematological malignancies.
Non-Hodgkin lymphoma
Lymphoma cells and non-neoplastic reactive lymphocytes infiltrating lymphoma microenvironment express PD1 and/or PD-L1, depending on the lymphoma histological subtype and the type of tumor microenvironment. As a general rule, PD1 is rarely expressed on lymphoma cells, while it is commonly expressed on lymphoma infiltrating lymphocytes (Citation35,Citation36). Similarly, PD-L1 expression is dependent on the histological non-Hodgkin lymphoma (NHL) subtype with PMBCL, and T-cell-rich DLBCL being high expressors, while mantle-cell lymphoma, marginal zone lymphoma, and Burkitt lymphoma were reported as PD-L1-negative (Citation37,Citation38). Moreover, PD-L1 is expressed on normal reactive lymphocytes, and it may alter their function by interacting with PD1 expressed on lymphoma cells or other immune cells present in the local tumor environment (Citation39).
Diffuse large cell B-lymphoma
The expression as well as the prognostic significance of PD-L1 expression in cases with DLBCL has been the subject of a recently published trial. PD-L1 expression was evaluated by using double immunostaining for PD-L1 and PAX5 in 1253 biopsy samples. Authors proposed a new definition for PD-L1(+) positivity: A case with DLBCL was classified as PD-L1(+) if 30% or more of lymphoma cells showed distinct PD-L1 immunostaining, independently of PD-L1 expression of nonmalignant cells present in TME. Microenvironmental PD-L1-positive [mPD-L1(+)] was defined a case if PD-L1-negative and PD-L1 expression was detected on nonmalignant stromal cells representing 20% or more of the total sample cellularity. By using this, definition 11% and 15% of the cases were classified as PD-L1(+) and mPD-L1(+), respectively. In multivariate analysis, PD-L1(+) was associated with statistically significantly decreased overall survival. On the contrary, there was no significant difference in OS between mPD-L1(+) and mPD-L1-negative cases (Citation40).
In another recent study, 571 cases of B-cell lymphomas were evaluated for the presence of 9q24 rearrangements by using FISH. Rearrangements of locus 9q24 were identified in 20% of primary mediastinal B-cell lymphoma (PMBCL) cases and were significantly correlated with PD-L1 expression (Citation41). Taken together, the above data suggest that anti-PD1 blockade might be effective in a subset of patients with aggressive B-cell lymphomas.
Follicular lymphoma
Follicular lymphoma (FL) does not generally express PD1 or its ligand PD-L1. FL is characterized by a specific lymphoma microenvironment that plays a fundamental role in the biology of disease. PD1 expressing lymphocytes are detectable within the tumor microenvironment (Citation35). In a previous study, lymphoma infiltrating lymphocytes showed reduced signaling after cytokine stimulation as compared with peripheral blood T cells, implying that these T cells were anergic. PD1 was expressed on 82% and 33% of tumor-infiltrating and peripheral blood T cells, respectively suggesting that T-cell anergy is related to PD1 expression (Citation42,Citation43). The prognostic significance of PD1 and PD-L1-positive lymphoma infiltrating lymphocytes is a subject of active research.
A Phase-I study evaluated the safety and efficacy of nivolumab in patients with relapsed or refractory lymphoid malignancies, including DLBCL, FL, PMBCL, T-NHL, multiple myeloma (MM), and classical Hodgkin lymphoma (cHL). Nivolumab was administered in an escalated manner every 2 weeks for a total of 2 years. In this trial, a total of 10 previously treated FL patients were included, and among them, one patient achieved CR, three patients achieved partial remission (PR), six patients had stable disease, and PFS at 6 months was 68% (Citation44).
T-cell NHL
PD1 expression is usually not observed on the surface of the malignant cells in cases of T-NHL. Exceptions to this rule are T-cell angioimmunoblastic lymphoma and adult T-cell leukaemia/lymphoma, where PD1 is strongly expressed on the vast majority of neoplastic cells (Citation35,Citation36). Similarly, PD1 is generally not expressed on non-neoplastic lymphocytes present in the lymphoma microenvironment.
PD-L1 shows variable expression on malignant cells of T-NHL, with anaplastic T-NHL, and extranodal NK/T-cell lymphoma displaying strong PD-L1 positivity, while tumor infiltrating lymphocytes are usually PD-L1 negative (Citation45,Citation46).
In the Phase-I trial performed by Lesokhin et al., five patients with relapsed/refractory peripheral T-cell NHL were included. Two out of these five patients achieved PR after treatment with nivolumab for an overall response rate (ORR) of 40% (all PR), and with PFS at 6 months of 30% (Citation44). At present, the number of patients with T-NHL included in published trials is too small for meaningful conclusions.
Hodgkin lymphoma
Classic HL is characterized by an excessive reactive inflammatory background surrounding the neoplastic Hodgkin or Reed Sternberg cells which comprise less than 1% of the total cell infiltrate (Citation38). PD-L1 and PD-L2 are overexpressed on the surface of malignant cells in the vast majority of patients with HL. The pathogenetic mechanisms responsible for PD-L1 and PD-L2 overexpression are briefly discussed below: (i) PD-L1 and PD-L2 genes located in 9p24 locus are overexpressed due to 9p24 amplification that is frequently observed in the nodular sclerosis histological subtype of HL (Citation47). (ii) JAK2 gene is located in 9p24 locus and chromosomal amplification induces JAK2 overexpression and JAK-STAT activation, finally resulting in PD-L1 overexpression (Citation47). (iii) Epstein–Barr viral (EBV) infection which is observed in approximately 30% of HL cases promotes PD-L1 upregulation through aberrant signaling induced by the EBV–DNA episome present in the infected cells (Citation48).
Although the malignant cells in HL usually do not express PD1, PD1-positive lymphocytes are frequently observed in tumor microenvironment (Citation49). Yamamoto et al. observed increased number of peripheral blood PD1-positive T cells in HL patients in comparison with healthy volunteers and patients with B-cell NHL (Citation50). Experimental data showed that signaling through PD1 induces an inhibitory effect on CD4-positive lymphocytes present in tumor microenvironment and setup the rationale for a possible therapeutic benefit by PD1 blockade (Citation51).
In a Phase-I dose escalation trial testing the safety and efficacy of anti-PD1 nivolumab, 23 patients with relapsed or refractory HL were included. Twenty out of 23 patients (87%) responded to anti-PD1 MAb with four patients (17%) achieving CR. PFS at 6 months was 86%. Interestingly, response to treatment was often observed after a significant delay in contrast to the rapid antitumor effect produced after most antilymphoma treatments (Citation52). In another Phase-I trial, 29 heavily pretreated patients with classical HL received treatment with pembrolizumab. All patients had previously received brentuximab vedotin (BV), while approximately 70% had failed a previous autograft. Twenty out of 29 patients showed an objective response for an ORR of 69%, while six patients achieved CR (Citation53). Toxicity was manageable in both trials.
Multiple myeloma
Preclinical data showed that PD1 and PD-L1 are expressed on the surface of both malignant plasma cells as well as on lymphocytes present in tumor microenvironment (Citation54,Citation55). Biopsy samples from 305 patients with monoclonal gammopathy of unknown significance (MGUS) and smoldering multiple myeloma (MM) were analyzed for the expression of proteins involved in immune checkpoints. Data analysis showed that expression of PD-L1 on clonal plasma cells as well as on tumor infiltrating lymphocytes was associated with increased incidence of progression to symptomatic MM (Citation56). Patients with MM were included in Phase-I trials testing the safety and efficacy of nivolumab and pembrolizumab. Unfortunately, no response was observed among 27 patients with MM treated with nivolumab (Citation44). However, 18 out of 27 patients (67%) had stable disease, which justifies further testing of PD1 blockade in future trials.
Myeloid malignancies
In a recent trial, authors examined the expression of PD1, PD-L1, PD-L2, and CTLA-4 in patients with myelodysplastic syndrome (MDS), chronic myelomonocytic leukemia (CMML), and acute myeloid leukemia (AML) (Citation57). Increased mRNA expression of PD1, PD-L1, PD-L2, and CTLA4 was observed in 15%, 34%, 14%, and 8% of the patients, respectively. Hypomethylating agents, such as azacytidine and decitabine, have shown significant activity in the treatment of patients with high-risk MDS. However, most of the responses are not durable, and the mechanism of resistance to demethylation is currently unknown. A recent trial showed that expression of PD1, PD-L1, and PD-L2 is regulated by DNA methylation. Treatment with demethylating agents induced increased expression of PD1, PD-L1, PD-L2, and CTLA-4 genes. Interestingly, patients who did not respond to demethylation displayed significant increase in gene expression as compared with responders. In vitro treatment of blasts from MDS patients and from MDS lines with decitabine resulted in overexpression of the genes encoding for the proteins involved in PD1-PD-L immune checkpoint. Taken together, these data support the concept that PD1 checkpoint is involved in MDS pathogenesis and in the resistance to demethylating agents (Citation57). PD1 blockade, especially in combination with demethylating agents might show significant activity in the treatment of MDS.
Furthermore, animal data showed that the PD1 immune checkpoint is involved in the inhibition of anti-leukemic immunity against AML and chronic myeloid leukemia (CML) cases. Blast cells from the murine leukemia line C1498 induced a lethal AML phenotype in all PD1+/+ animals after intravenous administration. On the contrary, a strong antileukemic immune response was observed in PD1-/- mice infused with C1498. PD1-/- mice showed significantly decreased AML tumor load and had significantly increased survival as compared with wild-type animals (Citation58,Citation59).
PD1 blockade as consolidation after transplantation
From a theoretical point of view, PD1 immune checkpoint blockade should be more effective when given as consolidation after autologous or allogeneic stem cell transplantation due to the following reasons: (i) the burden of disease is a significant factor predictive of response to treatment and the state of minimal disease usually achieved after conditioning offers this theoretical advantage, (ii) conditioning not only helps in eradication of disease, but also has a significant impact on tumor microenvironment by eliminating tumor infiltrating lymphocytes that suppress the antitumor immunity (Citation60,Citation61), (iii) after transplantation, a resetting of immune system occurs, and therefore, PD1 blockade may prevent exhaustion of newly generated antitumor lymphocytes, resulting in the eradication of minimal residual disease (Citation62,Citation63), and (iv) furthermore, after allo-SCT, PD1 blockade may augment the anti-tumor efficacy of alloreactive donor lymphocytes which is suppressed by the interaction with PD1 ligands expressed on the surface of malignant cells.
Preclinical data showed that marrow-infiltrating donor lymphocytes predict the response to donor lymphocyte infusion (DLI) after allo-SCT in patients with relapsed CML. In a recent trial, marrow infiltrating lymphocytes were analyzed in 29 patients who received CD4-positively selected DLI for treatment of CML, relapsed after a previous allo-SCT. The presence of CD8-marrow-infiltrating lymphocytes at levels above 4% was the stronger predictor of response to subsequent DLI administration. By using mRNA expression profiling, a specific gene expression pattern associated with T-cell exhaustion was observed in a proportion of marrow-infiltrating lymphocytes. Moreover, a reversal from exhausted to nonexhausted phenotype was observed after DLI treatment in responders. Data from this study showed that response to DLI is associated with the density of pre-existing marrow CD8+ infiltrating lymphocytes and the reversal from an exhausted to nonexhausted phenotype. T-cell exhaustion may be a reason for the failure of alloreactive lymphocytes to eradicate leukemia and support the potential use of PD1-blockade with or without the addition of DLI (Citation64). The impact of immune checkpoint pathways on alloreactivity is further supported by previous data showing significant correlation between certain CTLA-4 polymorphisms and the DLI efficacy (Citation31). Taken together, these data support the concept of increased graft-versus leukemia (GVL) effect by using PD1-blockade after allo-SCT.
Theoretical concerns regarding safety of PD1-blockade after allo-SCT
PD1 immune checkpoint is a key regulator of suppression of alloimmune response and tolerance induction in the setting of solid-organ transplantation. Upregulation of PD1 and PD-L1 expression on alloreactive T cells occurs in transplant recipients, while PD-L1 expression is induced on the parenchymal cell of grafted organs, such as heart and pancreatic islets, and PD1-PD-L1 interaction helps in the prevention of rejection (Citation65,Citation66). As a further proof of the concept, experimental data in solid organ transplant models showed that administration of PD-L1 blocking antibodies results in accelerated graft rejection (Citation67).
PD1 immune checkpoint also helps in the elimination of tissue damage induced by graft versus host disease (GVHD) in allogeneic chimeras. PD1 ligands are expressed on the surface of GVHD targets and interact with PD1 receptor expressed on activated alloreactive T cells. PD-L2 has a more restricted pattern of expression as compared with PD-L1. PD-L1 has a broad pattern of expression and is present on hematopoietic cells as well as on nonhematopoietic cells and its expression is induced in stress condition and under the influence of inflammatory stimuli (Citation5,Citation68,Citation69).
Data from animal models showed that PD1-ligands were strongly expressed in the spleen, liver, lung, and intestine of mice affected by GVHD, while the expression was weak or absent in GVHD-free mice. Immunofluorence, analysis revealed that PD-L1 was strongly expressed on both hematopoietic cells and on parenchymal cells of target organs, while PD-L2 expression was restricted on hematopoietic cells. A significant finding was that PD-L1 expression on parenchymal cells was critical for suppressing the severity of acute GVHD. By using blocking experiments, the authors observed that PD1/PD-L1 blockade exacerbates acute GVHD, while, PD1/PD-L2 blockade had minimal or no effect on GVHD (Citation70). In an animal model of chronic GVHD, analysis of data showed that PD1 receptor was continuously overexpressed on donor alloreactive T-cells, while PD-L1 ligand was only transiently overexpressed on host tissues during the initial phase of chronic GVHD. PD1 pathway blocking by using either anti-PD1 or anti-PD-L1 antibodies induced severe exacerbation of chronic GVHD (Citation71). Taken together, the results from the above studies and from many other animal experiments raise significant concerns about the safety of PD1 immune checkpoint blockade after allo-SCT, mainly due to the possible increased GVHD severity and mortality.
Clinical experience of PD1 blockade after allo-SCT
As already mentioned, the idea of blocking PD1-pathway after allo-SCT with the aim to augment the GVT effect is tempting. To our knowledge, there is no published experience regarding the safety and the therapeutic efficacy of PD1 pathway blockade after allo-SCT. However, the safety and efficacy of blocking the CTLA-4 molecule by the use of monoclonal antibody ipilimumab has been assessed in 29 patients with relapsed or progressive malignancies after allo-SCT. In contrast with the theoretical expectation, no dose-limiting toxicity was defined, and ipilimumab given in a single infusion did not induce GVHD exacerbation or graft rejection. However, organ-specific autoimmune disorders were observed in four patients (arthritis, hyperthyroidism, and pneumonitis). Objective responses were observed in a significant proportion of patients. Two out of 14 patients with HL achieved CR, while one patient with mantle cell lymphoma achieved PR (Citation72). Analysis of peripheral blood samples collected before and after administration of ipilimumab showed significant changes in T-cell subpopulations. A significant increase in both CD4+ and CD8+ T-cell counts was observed after ipilimumab infusion, as well as a decrease in the percentage of Tregs (Citation73).
PD1 blockade in combination with tumor vaccines
Immune system activation against tumor antigens by using cancer vaccines has been extensively studied in various neoplastic diseases, including solid tumors and hematological malignancies. Although the response rate to immunization is promising, eventually tumor cells escape immune surveillance and recurrence of the malignancy occurs in the vast majority of responders. Immune checkpoint activation represents at least one of the mechanisms by which tumor cells finally escape the cytotoxic action of immune effectors induced by vaccination. Indeed, data from animal models have shown the efficacy of combining tumor vaccines with immune checkpoint inhibitors. Soares et al. showed that administration of anti-PD-1/PD-L1 antibodies in combination with a tumor vaccine resulted in significant enhancement of antitumor immunity and prolongation of survival in a mouse model of pancreatic cancer (Citation74). Reappearance into the clinical scenario of vaccination against hematological malignancies is anticipated especially in combination with immune checkpoint inhibitors.
PD1 blockade in combination with other immune checkpoint inhibitors
The mechanism of resistance to immune checkpoint inhibitors is not known, but animal data clearly showed that down-regulation of the receptor and induced expression of another inhibitor are used by the tumor cells in order to escape immune surveillance. A recent study in a mouse model of melanoma showed that irradiation in combination with anti-CTLA4 resulted in induction of PD-L1 expression, leading to tumor resistance (Citation75). The combination treatment was also studied in a mouse model of multiple myeloma and showed significant efficacy (Citation76). These data led to clinical trials testing the blockade of more than one immune checkpoints acting in parallel, such as by using anti-CTLA4 in combination with an anti-PD1/PD-L1 antibody. Nivolumab in combination with ipilimumab have been tested in patients with metastatic melanoma, and the combination strategy proved to be safe and effective (Citation77). Currently, many trials are underway testing the combination approach in patients with hematological malignancies, mainly lymphomas.
Toxicity and response assessment
The toxicity profile of PD1 inhibitors is excellent with severe adverse reactions rarely observed and only a few treatment-related deaths have been reported among hundreds of patients treated so far (Citation21,Citation22,Citation44,Citation52,Citation53). Adverse reactions are directly related to immune de-regulation provoked by PD1 inhibition, and are autoimmune manifestations affecting almost any organ. Pneumonitis, thyroiditis, autoimmune colitis, skin rash occur in a substantial proportion of patients. Hematological side effects, mainly anemia, neutropenia and thrombocytopenia have been observed in a variable proportion of patients ranging from 10% to 30% depending on the drug used. In general, the side effects were manageable and resulted in drug discontinuation in a small percentage of patients. In most cases treatment consisted of short course of corticosteroids.
In contrast with the rapid responses expected in patients with lymphoma treated with conventional chemotherapy, responses after treatment with immune checkpoint inhibitors are usually delayed, sometimes occurring even after more than 4 months of treatment. Moreover, in many cases with solid tumors, an initial enlargement of tumor masses has been observed in the initial phase of treatment which is usually followed by a significant shrinkage (Citation46,Citation52,Citation53). This initial tumor flare has been named pseudoprogression and it has been attributed to lymphocyte infiltration and inflammation occurring in tumor microenvironment. This phenomenon often results in the misleading diagnosis of disease progression and should be taken into account when evaluating response. Therefore, a set of immune-related response criteria have been proposed as a more appropriate method of response evaluation in patients with solid tumors treated with immune checkpoint inhibitors (Citation78). In analogy, the current response assessment criteria used for hematological malignancies and in particular for lymphoma might not be appropriate for use with this class of drugs. Also the category of stable disease, which is not accepted as an objective response in patients treated with conventional chemotherapy, has a different meaning in the setting of immunotherapy. Patients with stable disease may enjoy prolonged survival as long as tumor cells express PD-ligands and remains under the control of the immune system.
Summary
Blocking of immune checkpoint pathways by monoclonal antibodies opens a new era in the field of immunotherapy. Results from clinical trials are promising, and currently, this approach has been proven effective and safe in patients with metastatic melanoma, head and neck cancer, lung carcinoma, and HL. Trials testing the feasibility and efficacy of checkpoint blockade after autologous or allogeneic stem cell transplantation are currently underway. One possible mechanism of resistance of neoplastic cells to blocking therapy is the up-regulation of other immune checkpoints. An active area of research is the identification of all the immune checkpoints that act in parallel and control the immune response. Combination of antibodies blocking different immune checkpoints is a tempting hypothesis that needs to be tested in future clinical trials.
Disclosure statement
The authors have no conflicts of interest to report.
References
- Linsley P, Ledbetter J. The role of the CD28 receptor during T cell responses to antigen. Annu Rev Immunol. 1993;11:191–12.
- Suh WK, Tafuri A, Berg-Brown NN, Shahinian A, Plyte S, Duncan GS, et al. The inducible costimulator plays the major costimulatory role in humoral immune responses in the absence of CD28. J Immunol. 2004;172:5917–23.
- Sansom DM, Manzotti CN, Zheng Y. What’s the difference between CD80 and CD86? Trends Immunol. 2003;24:313–18.
- Joffre O, Nolte MA, Sporri R, Reis e Sousa C. Inflammatory signals in dendritic cell activation and the induction of adaptive immunity. Immunol Rev. 2009;227:234–47.
- Keir ME, Butte MJ, Freeman GJ, Sharpe AH. PD-1 and its ligands in tolerance and immunity. Annu Rev Immunol. 2008;26:677–704.
- Walker LS. Treg and CTLA-4: Two intertwining pathways to immune tolerance. J Autoimmun. 2013;45:49–57.
- Gardner D, Jeffery LE, Sansom DM. Understanding the CD28/CTLA-4 (CD152) pathway and its implications for costimulatory blockade. Am J Transplant. 2014;14:1985–91.
- Linsley PS, Greene JL, Brady W, Bajorath J, Ledbetter JA, Peach R. Human B7-1 (CD80) and B7-2 (CD86) bind with similar avidities but distinct kinetics to CD28 and CTLA-4 receptors. Immunity. 1994;1:793–801.
- Tivol EA, Borriello F, Schweitzer AN, Lynch WP, Bluestone JA, Sharpe AH. Loss of CTLA-4 leads to massive lymphoproliferation and fatal multiorgan tissue destruction, revealing a critical negative regulatory role of CTLA-4. Immunity. 1995;3:541–7.
- Latchman Y, Wood CR, Chernova T, Chaudhary D, Borde M, Chernova I, et al. PD-L2 is a second ligand for PD-1 and inhibits T cell activation. Nat Immunol. 2001;2:261–8.
- Nishimura H, Nose M, Hiai H, Minato N, Honjo T. Development of lupus-like autoimmune diseases by disruption of the PD-1 gene encoding an ITIM motif-carrying immunoreceptor. Immunity. 1999;11:141–51.
- Francisco LM1, Salinas VH, Brown KE, Vanguri VK, Freeman GJ, Kuchroo VK, et al. PD-L1 regulates the development, maintenance, and function of induced regulatory T cells. J Exp Med. 2009;206:3015–29.
- Day CL, Kaufmann DE, Kiepiela P, Brown JA, Moodley ES, Reddy S, et al. PD-1 expression on HIV-specific T cells is associated with T-cell exhaustion and disease progression. Nature. 2006;443:350–4.
- Francisco LM, Sage PT, Sharpe AH. The PD-1 pathway in tolerance and autoimmunity. Immunol Rev. 2010;236:219–42.
- Lepenies B, Jacobs T. The role of negative costimulators during parasitic infections. Endocr Metab Immune Disord Drug Targets. 2008;8:279–88.
- Urbani S, Amadei B, Tola D, Pedrazzi G, Sacchelli L, Cavallo MC, et al. Restoration of HCV-specific T cell functions by PD-1/PD-L1 blockade in HCV infection: effect of viremia levels and antiviral treatment. J Hepatol. 2008;48:548–58.
- Dong H, Strome SE, Salomao DR, Tamura H, Hirano F, Flies DB, et al. Tumor-associated B7-H1 promotes T-cell apoptosis: a potential mechanism of immune evasion. Nat Med. 2002;8:793–800.
- Ahmadzadeh M, Johnson LA, Heemskerk B, Wunderlich JR, Dudley ME, White DE, et al. Tumor antigen-specific CD8 T cells infiltrating the tumor express high levels of PD-1 and are functionally impaired. Blood. 2009;114:1537–44.
- Iwai Y, Ishida M, Tanaka Y, Okazaki T, Honjo T, Minato N. Involvement of PD-L1 on tumor cells in the escape from host immune system and tumor immunotherapy by PD-L1 blockade. Proc Natl Acad Sci USA. 2002;99:12293–7.
- Strome SE1, Dong H, Tamura H, Voss SG, Flies DB, Tamada K, et al. B7-H1 blockade augments adoptive T-cell immunotherapy for squamous cell carcinoma. Cancer Res. 2003;63:6501–5.
- Berger R, Rotem-Yehudar R, Slama G, Landes S, Kneller A, Leiba M, et al. Phase I safety and pharmacokinetic study of CT-011, a humanized antibody interacting with PD-1, in patients with advanced hematologic malignancies. Clin Cancer Res. 2008;14:3044–51.
- Armand P, Nagler A, Weller EA, Devine SM, Avigan DE, Chen YB, et al. Disabling immune tolerance by programmed death-1 blockade with pidilizumab after autologous hematopoietic stem-cell transplantation for diffuse large B-cell lymphoma: results of an international phase II trial. J Clin Oncol. 2013;31:4199–206.
- Topalian SL, Hodi FS, Brahmer JR, Gettinger SN, Smith DC, McDermott DF, et al. Safety, activity, and immune correlates of anti-PD-1 antibody in cancer. N Engl J Med. 2012;28:2443–54.
- Hodi FS, O'Day SJ, McDermott DF, Weber RW, Sosman JA, Haanen JB, et al. Improved survival with ipilimumab in patients with metastatic melanoma. N Engl J Med. 2010;363:711–23.
- Hamid O, Robert C, Daud A, Hodi FS, Hwu WJ, Kefford R, et al. Safety and tumor responses with lambrolizumab (anti-PD-1) in melanoma. N Engl J Med. 2013;369:134–44.
- Robert C, Long GV, Brady B, Dutriaux C, Maio M, Mortier L, et al. Nivolumab in previously untreated melanoma without BRAF mutation. N Engl J Med. 2015;372:320–30.
- Balkwill F, Capasso M, Hagemann T. The tumor microenvironment at a glance. J Cell Sci. 2012;125:5591–6.
- Teng M, Ngiow S, Ribas A, Smyth M. Classifying cancers based on T-cell infiltration and PD-L1. Cancer Res. 2015;75:1–7.
- Fehrenbacher L, Spira A, Ballinger M, Kowanetz M, Vansteenkiste J, Mazieres J, et al. Atezolizumab versus docetaxel for patients with previously treated non-small-cell lung cancer (POPLAR): a multicentre, open-label, phase 2 randomised controlled trial. Lancet. 2016;387:1837–46.
- Ueda H, Howson JM, Esposito L, Esposito L, Heward J, Snook H, et al. Association of the T-cell regulatory gene CTLA-4 with susceptibility to autoimmune disease. Nature. 2003;423:506–11.
- Metaxas Y, Bertz H, Spyridonidis A, Spyroupoulou-Vlachou M, Porzelius C, Finke J. CT60 single-nucleotide polymorphism as a surrogate marker for donor lymphocyte infusion outcome after allogeneic cell transplantation for acute leukemia. Bone Marrow Transplant. 2012;47:411–15.
- Brahmer J, Reckamp KL, Baas P, Crinò L, Eberhardt WE, Poddubskaya E, et al. Nivolumab versus docetaxel in advanced squamous-cell non-small-cell lung cancer. N Engl J Med. 2015;373:123–35.
- Taube JM, Klein A, Brahmer JR, Xu H, Pan X, Kim JH, et al. Association of PD-1, PD-1 ligands, and other features of the tumor immune microenvironment with response to anti-PD-1 therapy. Clin Cancer Res. 2014;20:5064–74.
- Herbst RS, Soria JC, Kowanetz M, Fine GD, Hamid O, Gordon MS, et al. Predictive correlates of response to the anti-PD-L1 antibody MPDL3280A in cancer patients. Nature. 2014;515:563–7.
- Dorfman DM, Brown JA, Shahsafaei A, Freeman GJ. Programmed death-1 (PD-1) is a marker of germinal center-associated T cells and angioimmunoblastic T-cell lymphoma. Am J Surg Pathol. 2006;30:802–10.
- Xerri L, Chetaille B, Serriari N, Attias C, Guillaume Y, Arnoulet C, et al. Programmed death 1 is a marker of angioimmunoblastic T-cell lymphoma and B-cell small lymphocytic lymphoma/chronic lymphocytic leukemia. Hum Pathol. 2008;39:1050–8.
- Shimauchi T, Kabashima K, Nakashima D, Sugita K, Yamada Y, Hino R, et al. Augmented expression of programmed death-1 in both neoplastic and nonneoplastic CD4+ T-cells in adult T-cell leukemia/lymphoma. Int J Cancer. 2007;121:2585–90.
- Green MR, Rodig S, Juszczynski P, Ouyang J, Sinha P, O'Donnell E, et al. Constitutive AP-1 activity and EBV infection induce PD-L1 in Hodgkin lymphomas and posttransplant lymphoproliferative disorders: implications for targeted therapy. Clin Cancer Res. 2012;18:1611–88.
- Brown JA, Dorfman DM, Ma FR, Sullivan EL, Munoz O, Wood CR, et al. Blockade of programmed death-1 ligands on dendritic cells enhances T cell activation and cytokine production. J Immunol. 2003;170:1257–66.
- Kiyasu J, Miyoshi H, Hirata A, Arakawa F, Ichikawa A, Niino D, et al. Expression of programmed cell death ligand 1 is associated with poor overall survival in patients with diffuse large B-cell lymphoma. Blood. 2015;126:2193–201.
- Twa D, Chan F, Ben-Neriah S, Woolcock B, Mottok A, Tan K, et al. Genomic rearrangements involving programmed death ligands are recurrent in primary mediastinal large B-cell lymphoma. Blood. 2014;123:2062–5.
- Myklebust JH, Irish JM, Brody J, Czerwinski DK, Houot R, Kohrt HE, et al. High PD-1 expression and suppressed cytokine signaling distinguish T cells infiltrating follicular lymphoma tumors from peripheral T cells. Blood. 2013;121:1367–76.
- Carreras J, Lopez-Guillermo A, Roncador G, Villamor N, Colomo L, Martinez A, et al. High numbers of tumor-infi ltrating programmed cell death 1-positive regulatory lymphocytes are associated with improved overall survival in follicular lymphoma. J Clin Oncol. 2009;27:1470–6.
- Lesokhin AM, Ansell SM, Armand P, Scott EC, Halwani A, Gutierrez M, et al. Preliminary results of a phase I study of nivolumab (BMS-936558) in patients with relapsed or refractory lymphoid malignancies. Blood. 2014;124:291.
- Wilcox RA, Feldman AL, Wada DA, Yang ZZ, Comfere NI, Dong H, et al. B7-H1 (PD-L1, CD274) suppresses host immunity in T-cell lymphoproliferative disorders. Blood. 2009;114:2149–58.
- Han L, Liu F, Li R, Li Z, Chen X, Zhou Z, et al. Role of programmed death ligands in effective T-cell interactions in extranodal natural killer/T-cell lymphoma. Oncol Lett. 2014;8:1461–9.
- Green MR, Monti S, Rodig SJ, Juszczynski P, Currie T, O'Donnell E, et al. Integrative analysis reveals selective 9p24.1 amplification, increased PD-1 ligand expression, and further induction via JAK2 in nodular sclerosing Hodgkin lymphoma and primary mediastinal large B-cell lymphoma. Blood. 2010;116:3268–77.
- Chen BJ, Chapuy B, Ouyang J, Sun HH, Roemer MG, Xu ML, et al. PD-L1 expression is characteristic of a subset of aggressive B-cell lymphomas and virus-associated malignancies. Clin Cancer Res. 2013;19:3462–73.
- Chetaille B, Bertucci F, Finetti P, Esterni B, Stamatoullas A, Picquenot JM, et al. Molecular profiling of classical Hodgkin lymphoma tissues uncovers variations in the tumor microenvironment and correlations with EBV infection and outcome. Blood. 2009;113:2765–75.
- Yamamoto R, Nishikori M, Kitawaki T, Sakai T, Hishizawa M, Tashima M, et al. PD-1-PD-1 ligand interaction contributes to immunosuppressive microenvironment of Hodgkin lymphoma. Blood. 2008;111:3220–4.
- Chemnitz JM, Eggle D, Driesen J, Classen S, Riley JL, Debey-Pascher S, et al. RNA fingerprints provide direct evidence for the inhibitory role of TGFbeta and PD-1 on CD4+ T cells in Hodgkin lymphoma. Blood. 2007;110:3226–33.
- Ansell SM, Lesokhin AM, Borrello I, Halwani A, Scott EC, Gutierrez M, et al. PD-1 blockade with nivolumab in relapsed or refractory Hodgkin's lymphoma. N Engl J Med. 2015;372:311–19.
- Moskowitz CH, Ribrag V, Michot J-M, Martinelli G, Zinzani P-L, Gutierrez M, et al. PD-1 blockade with the monoclonal antibody pembrolizumab (MK-3475) in patients with classical Hodgkin lymphoma after brentuximab vedotin failure: preliminary results from a phase 1b study (KEYNOTE-013). Blood. 2014;124:290.
- Liu J, Hamrouni A, Wolowiec D, Coiteux V, Kuliczkowski K, Hetuin D, et al. Plasma cells from multiple myeloma patients express B7-H1 (PD-L1) and increase expression after stimulation with IFN-gamma and TLR ligands via a MyD88-, TRAF6-, and MEK-dependent pathway. Blood. 2007;110:296–304.
- Yousef S, Marvin J, Steinbach M, Langemo A, Kovacsovics T, Binder M, et al. Immunomodulatory molecule PD-L1 is expressed on malignant plasma cells and myeloma-propagating pre-plasma cells in the bone marrow of multiple myeloma patients. Blood Cancer J. 2015;5:e285.
- Dhodapkar M, Sexton R, Das R, Dhodapkar K, Zhang L, Sundaram R, et al. Prospective analysis of antigen-specific immunity, stem-cell antigens, and immune checkpoints in monoclonal gammopathy. Blood. 2015;126:2475–8.
- Yang H, Bueso-Ramos C, DiNardo C, Estecio MR, Davanlou M, Geng QR, et al. Expression of PD-L1, PD-L2, PD-1 and CTLA4 in myelodysplastic syndromes is enhanced by treatment with hypomethylating agents. Leukemia. 2014;28:1280–8.
- Zhang L, Gajewski TF, Kline J. PD-1/PD-L1 interactions inhibit antitumor immune responses in a murine acute myeloid leukemia model. Blood. 2009;114:1545–52.
- Mumprecht S, Schurch C, Schwaller J, Solenthaler M, Ochsenbein AF. Programmed death 1 signaling on chronic myeloid leukemia specific T cells results in T-cell exhaustion and disease progression. Blood. 2009;114:1528–36.
- Andorsky DJ1, Yamada RE, Said J, Pinkus GS, Betting DJ, Timmerman JM. Programmed death ligand 1 is expressed by non-Hodgkin lymphomas and inhibits the activity of tumor-associated T cells. Clin Cancer Res. 2011;17:4232–44.
- Ansell SM, Stenson M, Habermann TM, Jelinek DF, Witzig TE. Cd4+ T-cell immune response to large B-cell non-Hodgkin's lymphoma predicts patient outcome. J Clin Oncol. 2001;19:720–6.
- Guillaume T, Rubinstein DB, Symann M. Immune reconstitution and immunotherapy after autologous hematopoietic stem cell transplantation. Blood. 1998;92:1471–90.
- Porrata LF, Litzow MR, Markovic SN. Immune reconstitution after autologous hematopoietic stem cell transplantation. Mayo Clin Proc. 2001;76:407–12.
- Bachireddy P, Hainz U, Rooney M, Pozdnyakova O, Aldridge J, Zhang W. Reversal of in situ T-cell exhaustion during effective human antileukemia responses to donor lymphocyte infusion. Blood. 2014;123:1412–21.
- Sandner SE, Clarkson MR, Salama AD, Sanchez-Fueyo A, Domenig C. Habicht, et al. Role of the programmed death-1 pathway in regulation of alloimmune responses in vivo. J Immunol. 2005;174:3408–15.
- Ozkaynak E, Wang L, Goodearl A, McDonald K, Qin S, O'Keefe T, et al. Programmed death-1 targeting can promote allograft survival. J Immunol. 2002;169:6546–53.
- Ito T, Ueno T, Clarkson MR, Yuan X, Jurewicz MM, Yagita H, et al. Analysis of the role of negative T cell costimulatory pathways in CD4 and CD8 T cell-mediated alloimmune responses in vivo. J Immunol. 2005;174:6648–56.
- Yamazaki T, Akiba H, Iwai H, Matsuda H, Aoki M, Tanno Y, et al. Expression of programmed death 1 ligands by murine T cells and APC. J Immunol. 2002;169:5538–45.
- Nakae S, Suto H, Iikura M, Kakurai M, Sedgwick JD, Tsai M, et al. Mast cells enhance T cell activation: importance of mast cell costimulatory molecules and secreted TNF. J Immunol. 2006;176:2238–48.
- Saha A, Aoyama K, Taylor PA, Koehn BH, Veenstra RG, Panoskaltsis-Mortari A. Host programmed death ligand 1 is dominant over programmed death ligand 2 expression in regulating graft-versus-host disease lethality. Blood. 2013;122:3062–73.
- Fujiwara H, Maeda Y, Kobayashi K, Nishimori H, Matsuoka K, Fujii N, et al. Programmed death-1 pathway in host tissues ameliorates Th17/Th1-mediated experimental chronic graft-versus-host disease. J Immunol. 2014;193:2565–73.
- Bashey A, Medina B, Corringham S, Pasek M, Carrier E, Vrooman L, et al. CTLA4 blockade with ipilimumab to treat relapse of malignancy after allogeneic hematopoietic cell transplantation. Blood. 2009;113:1581–8.
- Zhou J, Bashey A, Zhong R, Corringham S, Messer K. CTLA-4 blockade following relapse of malignancy after allogeneic stem cell transplantation is associated with T cell activation but not with increased levels of T regulatory cells. Biol Blood Marrow Transplant. 2011;17:682–92.
- Soares KC, Rucki AA, Wu AA, Olino K, Xiao Q, Chai Y, et al. PD-1/PD-L1 blockade together with vaccine therapy facilitates effector T-cell infiltration into pancreatic tumors. J Immunother. 2015;38:1–11.
- Twyman-Saint Victor C, Rech AJ, Maity A, Rengan R, Pauken KE, Stelekati E, et al. Radiation and dual checkpoint blockade activate non-redundant immune mechanisms in cancer. Nature. 2015;520:373–7.
- Jing W, Gershan JA, Weber J, Tlomak D, McOlash Sabatos-Peyton C, et al. Combined immune checkpoint protein blockade and low dose whole body irradiation as immunotherapy for myeloma. J Immunother Cancer. 2015;3:2.
- Wolchok JD, Kluger H, Callahan MK, Postow MA, Rizvi NA, Lesokhin AM, et al. Nivolumab plus ipilimumab in advanced melanoma. N Engl J Med. 2013;362:122–33.
- Wolchok JD, Hoos A, O’Day S, Weber JS, Hamid O, Lebbé C, et al. Guidelines for the evaluation of immune therapy activity in solid tumors: immune-related response criteria. Clin Cancer Res. 2009;15:7412–20.