Abstract
Background: Glucose-6-phosphate dehydrogenase (G6PD) deficiency is an inherited disorder common in Sardinia. In this study, the frequency variation of G6PD-deficiency across age groups and birth cohorts was investigated using Age–Period–Cohort analysis.
Methods: Data were collected from the clinical records of 11,252 patients (6975 women, age range 17–94 years) who underwent endoscopy between 2000 and 2016 at a teaching hospital (University of Sassari), Italy. G6PD status was assessed by enzymatic assay based on G6PD/6GPD ratio. A Poisson log-linear regression model was used to identify age and time trend in G6PD deficiency.
Results: Enzyme deficiency was detected in 11.4% of the entire cohort (men: 7.9%; women: 13.6%). Age–Period–Cohort analysis showed no inflection points across age groups, especially after age 80. The effects of time period and birth cohorts on G6PD deficiency were negligible (frequencies before and after 1950 were 11.0% and 11.8%, respectively).
Conclusions: These findings indicate that the frequency of G6PD deficiency does not vary significantly in oldest subjects. The lack of evidence for selection across the malaria eradication time may be explained by other factors, including somatic cell selection or misclassification of heterozygotes women as G6PD normal in the older birth cohorts. Additional molecular studies may help clarify these issues.
The frequency of glucose-6-phosphate dehydrogenase deficiency is stable across age groups and does not vary in generations born before or after malaria eradication.
Key message
Introduction
d-glucose-6-phosphate dehydrogenase (G6PD, d-glucose-6-phosphate: NADP oxidoreductase; EC 1.1.1.49) is a “housekeeping” enzyme of the pentose phosphate pathway (PPP) which provides the extra-mitochondrial coenzyme nicotinamide-adenine dinucleotide phosphate (NADPH), as well as the ribose needed to synthesize DNA [Citation1]. NADPH enables cells to neutralize the action of several oxidative agents through the regeneration of reduced glutathione (GSH) from the disulfide form (GSSG). Loss-of-function mutations in the G6PD gene lead to enzyme deficiency which is the most common inherited enzymatic disorder. Affected patients may experience drug- or infection-induced haemolytic anaemia since red blood cell antioxidant defense depends almost exclusively on GSH levels [Citation2]. The G6PD gene is encoded on the X chromosome (Xq28 locus), therefore enzyme deficiency is inherited in an X-linked fashion [Citation3]. Total enzyme deficiency is therefore observed in hemizygous men and homozygous women, whereas in heterozygous women variable levels of residual enzyme activity may be detected, as the result of partial compensation by the normal allele and random inactivation of one X chromosome [Citation4]. Carriers of a G6PD-deficient allele, especially female heterozygotes, have a selective advantage against malaria caused by Plasmodium falciparum [Citation5,Citation6]. For this reason, in countries where malaria was endemic in the past, like the island of Sardinia, G6PD deficiency reaches a frequency ranging between 12 and 24% according to the area [Citation7], with the majority of cases caused by a C→T transition at nucleotide 563 of the G6PD coding region [Citation8].
A number of studies suggest that the frequency of G6PD deficiency varies across the adult lifespan, rising in advanced age owing to its protective effect against age-related diseases [Citation9–Citation12]. Interestingly, the frequency of G6PD deficiency was reported to be greater, nearly twofold, in Sardinian centenarians, especially in hemizygous males [Citation12,Citation13]. Conversely, the frequency of G6PD deficiency was reported to be lower in patients with coronary artery disease [Citation14], a finding that was related to reduced atherogenicity of serum lipoprotein patterns via different metabolic mechanisms [Citation15], to lower levels of pro-inflammatory cytokines released by G6PD-deficient leukocytes [Citation16], and to cholesterol synthesis inhibition within NADPH-depleted cells [Citation17]. Moreover, experimental evidence coming from in vivo and in vitro studies suggests that G6PD deficiency may offer protection against carcinogenesis [Citation18,Citation19]. Indeed, an inverse association between G6PD deficiency and the occurrence of some types of cancer such as colorectal [Citation20] and hepatocellular [Citation21] was recently reported. Nonetheless, the increased frequency of G6PD deficiency observed in previous studies among the oldest people living in Sardinia may just be an artefact created by the cohort effect, since the selective pressure of malaria ceased after it was eradicated in 1950, and in more recent generations G6PD deficiency has not conferred any selective advantage over normal enzyme activity.
In this study, the frequency distribution of G6PD deficiency was investigated in a large cohort of adult subjects from Northern Sardinia spanning several generations including those born before and after malaria eradication.
Patients and methods
Clinical records of patients scheduled for endoscopy at the Department of Internal Medicine, University of Sassari, Italy, from January 2000 to December 2016 were collected. Demographic data including gender, year of birth and age were entered in a digital database. In the case of multiple endoscopic procedures for the same patient within the given time period, only results from the first test were kept in the analysis. An additional group of subjects whose G6PD activity was measured at the local Hospital, were included in the analysis.
G6PD activity was determined in all patients by quantitative spectrophotometric method, based on the ratio between G6PD/6GPD in erythrocytes, as previously reported [Citation22]. A ratio below 0.1 was used to define the presence of G6PD enzyme total deficiency and between 0.1 and 0.85 to define partial deficiency (Nurex, Italy). No molecular analysis was performed in G6PD-deficient patients.
An Institutional Review Board approval was obtained from the local Ethics Committee (Prot N° 2099/CE).
Statistical analysis
All patients were stratified by age and birth cohort in 10-year intervals. The frequency distribution of patients according to gender, age and birth cohort (at the time of endoscopy) was expressed as a percentage. G6PD activity in males was recorded as normal/deficient and in females as normal, partially deficient or totally deficient.
A log-linear Poisson regression model [Citation23] was used to estimate the effects of age, time period and birth cohort on the frequency of G6PD deficiency. The analysis was conducted separately in males and females. Data for age and birth cohort were stratified in 10-year intervals, and for time periods in four intervals from 2000 to 2016. The number nijk of subjects cross-classified by i age groups (i = 1,…,8), j time periods (j = 1,…,4) and k birth cohorts (k = 1,…,7) were reported in specific tables. The frequency of G6PD deficiency was obtained by dividing the number of deficient subjects (dijk) by the number of patients (nijk) allocated to each cell and log-transformed according to the equation below:
where fijk is the frequency of G6PD deficiency in the i-th age group, in the j-th year and within the k-th birth cohort; dijk represents the expected number of G6PD-deficient subjects and is assumed to be distributed as a Poisson variable; nijk indicates the population in each cell; μ is the intercept; αi the effect of the i-th age group; βj the effect of the j-th time period and γk the effect of k-th birth cohort. To avoid the problem of multicollinearity in the regression model, potentially created by the linear relationship between age, birth cohort and period (birth cohort = period − age), only second-order changes were considered (inflection points detectable in the plots of regression estimates) as reported in a previous study [Citation24]. To evaluate the statistical significance of the coefficients, the log–likelihood ratio test was used. Significance level was set at 0.05 (two-sided tests). Analyses were performed using SPSS, version 16.0 (SPSS Inc., Chicago, IL).
Results
A total of 11,252 clinical records were available for the analysis. The proportion of women was 62% (6975). Enzyme deficiency was detected in 11.4% of the entire cohort, and more specifically in 7.9% of men and in 13.6% of women, in line with the frequency previously reported for Northern Sardinia [Citation25]. The distribution of G6PD deficiency across all age categories remained remarkably stable up to the age of 90 to decline slightly thereafter in men (Mantel–Haenszel test of trend: χ2=0.066, p = .797 among men; χ2=0.005, p = .946 among women with total deficiency; χ2=0.031; p = .860 among women with partial deficiency (). Overall, the proportion of patients with G6PD deficiency in subjects younger and older than 90 was 8.0% and of 6.8% among men, and 13.6% and 12.1% among women, respectively, with no statistically significant differences (p = .780 and .702, Fisher's exact test). The statistical power given by our sample size was 98%, large enough to allow rejection of the null hypothesis of a 4% difference around a G6PD deficiency frequency of roughly 10%.
Table 1. Frequency of glucose-6-phosphate dehydrogenase (G6PD) deficiency by age decade and gender in 11,252 Sardinian patients undergoing endoscopic procedures.
Assuming a deficient allele frequency equal to 7.9% (corresponding to the total proportion of hemizygous males reported in ) the expected frequency of heterozygous females is 2p(1–p) = 14.6%. This value is slightly higher than the observed figure of 11.5% for partially deficient females, a discrepancy that could be attributed to a minimal loss of specificity by the biochemical test.
The effect of birth cohort was also negligible (with an overall frequency of G6PD deficiency before and after 1950 of 11.0% and 11.8%, respectively) (). In particular, the proportion of females with partial G6PD deficiency, mostly heterozygotes, born before and after 1950 was very similar (11.53% vs 11.49%).
Table 2. Frequency of glucose-6-phosphate dehydrogenase (G6PD) deficiency according to birth cohort and gender in 11,252 Sardinian patients undergoing endoscopic procedures.
Age–period–cohort analysis is reported in . The regression coefficients for time period (calendar time) and birth cohort were estimated by the Poisson log-linear model. The age curve showed a remarkable steadiness up to advanced age in both males and females (, left). Similarly, birth cohort showed no influence on the frequency of G6PD deficiency, as no definite inflection points were detectable in the curves for both males and females (, middle). Also the effect of time period was not important, since the estimated prevalence remained basically unchanged throughout the interval 2000–2016 (, right).
Figure 1. Variation of G6PD deficiency estimated by Poisson log-linear regression according to age, birth cohort and time period (calendar time) in 11,252 Sardinian patients stratified by gender. The categories chosen for reference are as follows: by age (left) ≥ 90 years; by birth cohort (middle) the generations born after 1980; by time period (right) the interval 2000–2016.
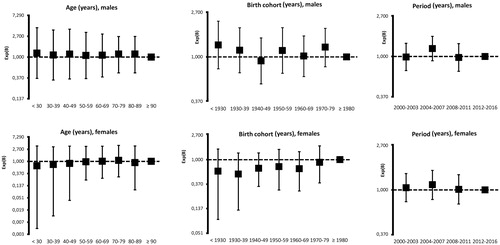
Discussion
Previous studies have suggested that G6PD activity might play a significant role in disease susceptibility, influencing survival fitness in a variety of animal species and also in humans [Citation11]. However, results are controversial, given the remarkable species-dependent differences. Moreover, results obtained in animal models may not always be transferable to humans. In Caenorhabditis elegans, G6PD knockout obtained by RNA interference determines defective oogenesis and embryogenesis [Citation26], whereas overexpression of the enzyme in Drosophila was associated with 30% lifespan extension [Citation27]. In rodents, even a modest increase in G6PD activity has been shown to be associated with increased levels of NADPH, a general strenghtening of antioxidant defence and prolonged healthspan [Citation28]. In the brain of aged mice, glucose-6-phosphate dehydrogenase is needed to protects against endogenous oxidative DNA damage and neurodegeneration [Citation29], a finding consistent with a previous report that enzyme deficiency increases the mutation rate in the brain of mice [Citation30]. In humans, the scenario is much more variegated, as evidence has been provided for both protective and harmful effects. A number of studies seem to mirror the results obtained in animal models, suggesting that G6PD deficiency is associated with increased risk of cardiovascular disease [Citation10] and perhaps neurodegenerative diseases [Citation31]. Enhanced oxidative stress and accelerated cellular senescence have been demonstrated in G6PD-deficient human fibroblasts [Citation32]. Others, including some studies carried out on the Sardinian population, highlighted a protective effect against cardiovascular risk [Citation9,Citation14], as well as cancer [Citation18–Citation21]. Previous investigations in Sardinia, where the frequency of G6PD deficiency was higher in the past owing to endemic malaria, suggested that the frequency of carriers increased in advanced age. Schwartz et al. [Citation13] reported a sharp increase in G6PD deficiency in centenarian men from Sardinia, suggesting that the enzymatic defect may protect elderly subjects from premature mortality, in contrast with our findings. The result reported by this author could have been determined by a cohort effect, since the oldest generations were more exposed to malarial risk, and further G6PD deficient carriers selection [Citation33]. In our study, this hypothesis was tested using an age–period–cohort (APC) model. Our results show that the frequency of G6PD deficiency is constant over the whole age range (17–94 years), in both men and women. In addition, the APC model also excluded any birth cohort effect on the frequency of G6PD deficiency, since it remained substantially stable across the 1920–1980 generations. This rules out a major role of malaria selection since the older generations up until 1950 were exposed to malaria for nearly one-third of their lifetime. As a consequence, our findings seem to indicate that deficiency carriers are neither advantaged nor disadvantaged in terms of survival fitness. This is particularly true for heterozygote women who are the only genotype demonstrably protective against malaria [Citation6]. In this respect, the discrepancy between the results of our investigation and those of the previously mentioned study could be explained, at least in part, by the great geographic variability of G6PD frequency within the island of Sardinia and, in particular, by the existence of an increasing gradient from North to South [Citation34,Citation35]. From this perspective, it cannot be ruled out that the centenarians surveyed in that study came from areas of higher frequency of G6PD deficiency compared with younger controls. The same could be said for epidemiological studies apparently showing a lower prevalence of cardiovascular disease in G6PD-deficient subjects [Citation9,Citation14]. Notably, our study was exempt from this bias, as the population was homogenous and living in the same area. Finally, although evidence obtained from large cohorts indicate a protective effect of G6PD deficiency for some type of cancers, but not for all, this may imply different pathways of carcinogenesis.
Genetic studies on human longevity have shown that the frequency of a list of allelic variants increases with age as they may confer a survival advantage (see for review [Citation36]). Clearly, as our study illustrates, mutations causing G6PD deficiency cannot be included in such a list. How can it be explained, however, that the opposite effect (a significant decrease in G6PD deficiency at extreme ages) was not observed in our study, too? This could be attributed to a number of causes. It has been postulated that in cells other than erythrocytes, the intra-mitochondrial NADPH generation may be sufficient to compensate for the lack of its production in the pentose phosphate pathway [Citation37], making G6PD deficiency in these cells clinically irrelevant. Alternatively, in the elderly a compensatory mechanism increasing the expression of residual enzyme activity is also possible. For example, in women who are heterozygous for G6PD deficiency it has been observed that X chromosome inactivation is not exactly random but tends to shift in favour of the normal cells, as if they were being gradually selected [Citation38]. In any case, even though G6PD deficiency impairs defence against oxidative stress, this would hardly reach a threshold to constitute a significant mortality risk factor in the elderly.
Our study has a number of strengths, including the large age interval of participants (17–94 years) as well as the genetic and socio-cultural homogeneity of the population investigated. Furthermore, the overall frequency of enzyme deficiency was similar to previous studies [Citation25]. On the other hand, two limitations should be taken into consideration: the lack of molecular genotyping and the possible problem of sample representativeness as the patients were referred to a gastrointestinal unit for dyspeptic symptoms. We think anyway that the homogeneity of the study population is great enough to minimize the effect of selection bias and residual confounders.
In conclusion, our results demonstrate that the frequency of G6PD deficiency does not change across age groups and specifically in generations preceding and following malaria eradication in Sardinia. The lack of evidence of selection across the malaria eradication time may be explained by other factors, including somatic cell selection or misclassification of heterozygotes women as G6PD normal in the older birth cohorts. Additional molecular studies may help clarify these issues. The positive association observed recently between G6PD deficiency and a lower risk of malignancies, although an interesting finding in itself, does not significantly affect the overall longevity of the population.
Acknowledgements
We wish to thank Mr. Giamaria Porcheddu and Dr. Alessandra Galistu for the help provided in retrieving G6PD data.
Disclosure statement
The authors report no conflicts of interest.
References
- Luzzatto L, Mehta AB, Vulliamy T. Glucose-6-phosphate dehydrogenase. In: Scriver CR, Beaudet AL, Sly WS, et al., editors. The metabolic and molecular bases of inherited disease. 8th ed. New York: McGraw-Hill; 2001. p. 4517–4553.
- Beutler E. G6PD: population genetics and clinical manifestations. Blood Rev. 1996;10:45–52.
- Szabo P, Purrello M, Rocchi M, et al. Cytological mapping of the human glucose-6-phosphate dehydrogenase gene distal to the fragile-X site suggests a high rate of meiotic recombination across this site. Proc Natl Acad Sci U S A. 1984;81:7855–7859.
- Rinaldi A, Filippi G, Siniscalco M. Variability of red cell phenotypes between and within individuals in an unbiased sample of 77 heterozygotes for G6PD deficiency in Sardinia. Am J Hum Genet. 1976;28:496–505.
- Luzzatto L, Notaro R. Malaria. Protecting against bad air. Science. 2001;293:442–443.
- Luzzatto L. G6PD deficiency: a polymorphism balanced by heterozygote advantage against malaria. Lancet Haematol. 2015;2:e400–e401.
- Siniscalco M, Bernini L, Latte B, et al. Favism and thalassemia in Sardinia and their relationship to malaria. Nature. 1961;190:1179–1180.
- Fiorelli G, Meloni T, Palomba V, et al. Gene frequency of glucose-6-phosphate dehydrogenase (G6PD) polymorphic variants in Sardinia. Gene Geogr. 1990;4:139–142.
- Cocco P, Todde P, Fornera S, et al. Mortality in a cohort of men expressing the glucose-6-phosphate dehydrogenase deficiency. Blood. 1998;91:706–709.
- Hecker PA, Leopold JA, Gupte SA, et al. Impact of glucose-6-phosphate dehydrogenase deficiency on the pathophysiology of cardiovascular disease. Am J Physiol Heart Circ Physiol. 2013;304:H491–H500.
- Yang HC, Wu YH, Liu HY, et al. What has passed is prolog: new cellular and physiological roles of G6PD. Free Radic Res. 2016;50:1047–1064.
- Manganelli G, Masullo U, Passarelli S, et al. Glucose-6-phosphate dehydrogenase deficiency: disadvantages and possible benefits. Cardiovasc Hematol Disord Drug Targets. 2013;13:73–82.
- Schwartz AG, Pashko LL. Dehydroepiandrosterone, glucose-6-phosphate dehydrogenase, and longevity. Ageing Res Rev. 2004;3:171–187.
- Meloni L, Manca MR, Loddo I, et al. Glucose-6-phosphate dehydrogenase deficiency protects against coronary heart disease. J Inherit Metab Dis. 2008;31:412–417.
- Muntoni S, Batetta B, Dessi S, et al. Serum lipoprotein profile in the Mediterranean variant of glucose-6-phosphate dehydrogenase deficiency. Eur J Epidemiol. 1992;8 Suppl 1:48–53.
- Sanna F, Bonatesta RR, Frongia B, et al. Production of inflammatory molecules in peripheral blood mononuclear cells from severely glucose-6-phosphate dehydrogenase-deficient subjects. J Vasc Res. 2007;44:253–263.
- Muntoni S, Muntoni S. Gene-nutrient interactions in G6PD-deficient subjects – implications for cardiovascular disease susceptibility. J Nutrigenet Nutrigenomics. 2008;1:49–54.
- Pascale R, Garcea R, Ruggiu ME, et al. Decreased stimulation by 12-O-tetradecanoylphorbol-13-acetate of superoxide radical production by polymorphonuclear leukocytes carrying the Mediterranean variant of glucose-6-phosphate dehydrogenase. Carcinogenesis. 1987;8:1567–1570.
- Feo F, Pirisi L, Pascale R, et al. Modulatory mechanisms of chemical carcinogenesis: the role of the NADPH pool in the benzo(a)pyrene activation. Toxicol Pathol. 1984;12:261–268.
- Dore MP, Davoli A, Longo N, et al. Glucose-6-phosphate dehydrogenase deficiency and risk of colorectal cancer in Northern Sardinia: a retrospective observational study. Medicine (Baltimore). 2016;95:e5254.
- Kowalik MA, Columbano A, Perra A. Emerging role of the pentose phosphate pathway in hepatocellular carcinoma. Front Oncol. 2017;7:87.
- Mosca A, Paleari R, Rosti E, et al. Simultaneous automated determination of glucose 6-phosphate dehydrogenase and 6-phosphogluconate dehydrogenase activities in whole blood. Eur J Clin Chem Clin Biochem. 1996;34:431–438.
- Holford TR. Understanding the effects of age, period, and cohort on incidence and mortality rates. Annu Rev Public Health. 1991;12:425–457.
- Pes GM, Cocco F, Bibbò S, et al. Cancer time trend in a population following a socio-economic transition: results of age-period-cohort analysis. Int J Public Health. 2017;62:407–414.
- Meloni T, Forteleoni G, Dore A, et al. Favism and hemolytic anemia in glucose-6-phosphate dehydrogenase-deficient subjects in North Sardinia. Acta Haematol. 1983;70:83–90.
- Yang HC, Chen TL, Wu YH, et al. Glucose 6-phosphate dehydrogenase deficiency enhances germ cell apoptosis and causes defective embryogenesis in Caenorhabditis elegans. Cell Death Dis. 2013;4:e616.
- Legan SK, Rebrin I, Mockett RJ, et al. Overexpression of glucose-6-phosphate dehydrogenase extends the life span of Drosophila melanogaster. J Biol Chem. 2008;283:32492–32499.
- Nóbrega-Pereira S, Fernandez-Marcos PJ, Thomas B, et al. G6PD protects from oxidative damage and improves healthspan in mice. Nat Commun. 2016;7:10894.
- Jeng W, Loniewska MM, Wells PG. Brain glucose-6-phosphate dehydrogenase protects against endogenous oxidative DNA damage and neurodegeneration in aged mice. ACS Chem Neurosci. 2013;4:1123–1132.
- Felix K, Rockwood LD, Pretsch W, et al. Moderate G6PD deficiency increases mutation rates in the brain of mice. Free Radic Biol Med. 2002;32:663–673.
- Ulusu NN. Glucose-6-phosphate dehydrogenase deficiency and Alzheimer's disease: Partners in crime? The hypothesis. Med Hypotheses. 2015;85:219–223.
- Ho HY, Cheng ML, Lu FJ, et al. Enhanced oxidative stress and accelerated cellular senescence in glucose-6-phosphate dehydrogenase (G6PD)–deficient human fibroblasts. Free Radic Biol Med. 2000;29:156–169.
- Tognotti E. The spread of malaria in Sardinia: an historical perspective. In: Greene LS, Danubio ME, editors. Adaptation to malaria: the interaction of biology and culture. New York (NY): Gordon & Breach; 1997. p. 237–247.
- Sanna E, Cosseddu GG, Floris G, et al. Micromapping the distribution of G6PD deficiency in Sardinia with data collected from the 1950s to the 1980s. In: Greene LS, Danubio ME, editors. Adaptation to malaria. The interaction of biology and culture. New York (NY): Gordon & Breach; 1997. p. 293–322.
- Pes GM, Bassotti G, Dore MP. Colorectal cancer mortality in relation to glucose-6-phosphate dehydrogenase deficiency and consanguinity in Sardinia: a spatial correlation analysis. Asian Pac J Cancer Prev. 2017;18:2403–2407.
- Santos-Lozano A, Santamarina A, Pareja-Galeano H, et al. The genetics of exceptional longevity: Insights from centenarians. Maturitas. 2016;90:49–57.
- Pandolfi PP, Sonati F, Rivi R, et al. Targeted disruption of the housekeeping gene encoding glucose 6-phosphate dehydrogenase (G6PD): G6PD is dispensable for pentose synthesis but essential for defense against oxidative stress. EMBO J. 1995;14:5209–5215.
- Christensen K, Kristiansen M, Hagen-Larsen H, et al. X-linked genetic factors regulate hematopoietic stem-cell kinetics in females. Blood. 2000;95:2449–2451.