Abstract
Albumin is the most abundant plasma protein and albumin infusion is commonly used. Conventionally, the biologic and therapeutic effects of albumin have been thought to be due to its oncotic properties. However, albumin has a variety of biologic functions, including molecular transport, anti-oxidation, anti-inflammation, endothelial stabilisation, anti-thrombotic effects, and the adjustment of capillary permeability. Despite this, the functions of albumin have not been thoroughly investigated. Recent studies have shown non-alcoholic fatty liver disease (NAFLD), viral hepatitis, cirrhosis, and liver failure to be associated with impairments in albumin function, which are associated with impairments in liver function and disease prognosis. Post-translational modifications of albumin cause structural modifications that affect protein function. Recently, the concentration of albumin associated with normal function, the ‘efficient albumin concentration’, has been attracting more interest. In addition, although many biologic markers, including albumin concentration, are widely used for the assessment of early liver dysfunction in patients with liver diseases, the predictive values are unsatisfactory. However, clinical evidence has suggested that albumin function may represent a novel biomarker of early impairment in liver function. In this review, we summarise the factors affecting albumin function and discuss the clinical significance of impairments in albumin function in various liver diseases.
The importance of albumin depends not only on its concentration, but also on its various physiological functions.
Impaired albumin function has been reported in a variety of liver diseases, and is associated with disease severity and prognosis, thereby proposing the concept of ‘effective albumin concentration’.
Albumin dysfunction occurs earlier than other conventional indicators, and albumin dysfunction may be a new biomarker of early impairment in liver function.
Many exogenous and endogenous factors lead to post-translational modifications of albumin, which alters the three-dimensional structure of albumin, resulting in a decrease in its biological activity.
Key messages
Introduction
Human serum albumin (HSA) is the most abundant protein (Sleep Citation2015) in healthy individuals and it plays an important role in maintaining plasma colloid osmotic pressure. Therefore, it has been used as a volume expander since the 1940s (Garcia-Martinez et al. Citation2013; Vincent et al. Citation2014). However, over the past few decades, an increasing number of studies have demonstrated that HSA is a multifunctional protein (Fanali et al. Citation2012; Shalish et al. Citation2017; Setoyama et al. Citation2017; Kawakami et al. Citation2006) with roles in the binding and transport of endogenous and exogenous molecules (Sleep Citation2015), anti-oxidation and anti-inflammation, endothelial stabilisation, anti-thrombosis, and the adjustment of capillary permeability. It has been shown that albumin infusion can improve the prognosis of patients with spontaneous peritonitis (Sort et al. Citation1999; Jamtgaard, Manning, and Cohn Citation2016) or hepatorenal syndrome (Ortega et al. Citation2002; Martin-Llahi et al. Citation2008), not only via plasma volume expansion, but also because of its non-colloid osmotic function. However, the clinical significance of the non-oncotic functions of HSA has not been investigated in detail. It is likely that albumin function is altered during liver dysfunction, and recent studies (Jalan et al. Citation2009; Oettl et al. Citation2013) have shown that the three-dimensional structure of albumin is modified, along with its function, in liver diseases, and that these modifications are related to the specific clinical features and severity of the disease, and the prognosis.
A better understanding of albumin function and its clinical significance should permit better use of albumin measurements in liver disease. In this review, we focus on the biologic characteristics of albumin and the corresponding functions, summarise the main methods of assessing albumin function, and discuss the changes in albumin function associated with specific liver diseases and their potential mechanisms.
The functions of albumin
HSA has a variety of functions (Evans Citation2002), including the maintenance of plasma oncotic pressure; the solubilisation, binding, and transport of endogenous and exogenous molecules; anti-oxidative, anti-inflammatory, and haemostatic effects; endothelial stabilisation; and the adjustment of capillary permeability. These dynamic functions depend on the concentration, structure, and distribution of HSA. The principal biologic characteristics and their potential mechanisms are summarised in .
Table 1. Function of albumin.
Maintenance of oncotic pressure
HSA is responsible for ∼75% of the plasma oncotic pressure (Artigas et al. Citation2016); therefore, albumin is commonly used for plasma volume replacement. In clinical practice, it is often administered as a resuscitation fluid to critically ill patients (Artigas et al. Citation2016; Moss et al. Citation1981; Wilkes and Navickis Citation2001), such as those with severe sepsis, shock, burns, spontaneous bacterial peritonitis, or other conditions that require the reinstatement of blood volume. The high concentration and negative charge (Farrugia Citation2010) of HSA are responsible for this best-known property. Indeed, HSA accounts for >50% of the total plasma protein (Artigas et al. Citation2016), and it therefore has a direct osmotic effect, which is the main mechanism underlying its role in oncotic pressure. The negative charge of HSA is the result of a large percentage of its constituent amino acids being acidic. As a result of this, HSA draws sodium ions, and consequently water, into the intravascular compartment.
Solubilisation, binding, and transport of molecules
Many of the physiologic functions of HSA depend on its molecular binding ability, which can increase the solubility of a variety of endogenous and exogenous substances in plasma, and permits their transportation or detoxification. The ability of HSA to bind various molecules is dependent on its three-dimensional structure (Oettl and Stauber Citation2007). It is a macromolecule with a molecular weight of 66,438 that is composed of 585 amino acid residues (Otagiri and Chuang Citation2009). It has a heart-shaped tertiary structure that consists of a large number of α-helices and three homologous domains (I–III) (), each of which comprise two subdomains (A and B) (Spinella, Sawhney, and Jalan Citation2016). The specific structure is stable but flexible: the conformation is easily modified (Arroyo, García-Martinez, and Salvatella Citation2014), which enables the molecule to accommodate many compounds with protein-binding affinity. Furthermore, some molecules compete for HSA binding sites and alter the three-dimensional structure of the macromolecule, which in turn affects its function.
Figure 1. The three-dimensional structure of human serum albumin. HSA consists of three homologous domains (I-III), each of which contains two subdomains (A and B). Binding site I and binding site II, and Cys34 are indicated by arrows.
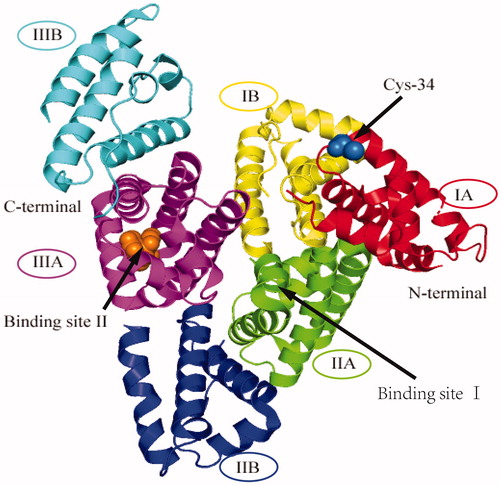
Such binding can occur at numerous different sites in HSA (Arroyo, García-Martinez, and Salvatella Citation2014), but sites I and II () predominate. Binding to albumin affects the distribution, biologic activity, and clearance of endogenous compounds, including bile acids, long-chain fatty acids, and bilirubin, and of exogenous compounds, including non-steroidal anti-inflammatory drugs, warfarin, antibiotics, and furosemide. In particular, the HSA-binding properties of drugs are important for both their pharmacodynamics and pharmacokinetics. In addition, the N-terminus of HSA can bind metals, such as copper, nickel, cobalt, and iron, and HSA can also bind lipopolysaccharide (LPS), reactive oxygen species, and nitric oxide (NO) (Artigas et al. Citation2016), which explains its anti-inflammatory and antioxidant roles.
Antioxidant function
Albumin can effectively scavenge reactive oxygen species using reduced sulfhydryl (-SH) moieties, and the antioxidant activity of albumin is mainly determined by the 34th cysteine residue (Cys-34, ) (Naldi et al. Citation2015). HSA represents the largest plasma reservoir of -SH (80%) (Garcia-Martinez et al. Citation2013; Jalan and Bernardi Citation2013; Pieniazek et al. Citation2018), because it contains 35 cysteine residues, 34 of which form 17 disulphide bonds. However, Cys-34 is not linked to another local residue by a disulphide bond, and can therefore be responsible for the dimerisation of HSA via an intermolecular disulphide bond (Naldi et al. Citation2017). In healthy individuals, 70%–80% of HSA circulates as human mercaptalbumin (HMA) (Garcia-Martinez et al. Citation2013), which is characterised by a reduced Cys-34 with antioxidant and scavenging activities, whereas the Cys-34 residue of 20%–30% of the HSA molecules is reversibly oxidised and bound to small thiol molecules, forming nonmercaptalbumin 1 (HNA1). The remaining 5% of HSA circulates as nonmercaptalbumin 2 (HNA2), in which the Cys-34 residue is irreversibly oxidised, resulting in a permanent loss of function. In addition, the binding of the N-terminal site of albumin to certain reduced metal ions, such as Cu(II) and Fe(II) (Taverna et al. Citation2013; Loban, Kime, and Powers Citation1997; Jing et al. Citation2016), also contributes to the antioxidant capacity of HSA.
Anti-inflammatory effects
HSA can bind a variety of inflammatory mediators, such as LPS (Artigas et al. Citation2016; Arroyo, García-Martinez, and Salvatella Citation2014; Kitano et al. Citation1996) and other bacterial antigens, and is responsible for regulation of the immune response through an interaction with toll-like receptor 4 in systemic inflammation and sepsis. In vitro experiments have shown that HSA can reduce endothelial inflammation by inhibiting the adhesion of inflammatory cells to the endothelium (Spinella, Sawhney, and Jalan Citation2016). Furthermore, an infusion of albumin can reduce the levels of LPS and other pro-inflammatory factors in patients with cirrhosis (Spinella, Sawhney, and Jalan Citation2016), and HSA can inhibit the respiratory burst of neutrophils in critically ill patients.
There is increasing evidence that HSA can regulate adaptive immunity rather than an immune-inert protein. Interestingly, HSA may behave differently under different pathological or physiological conditions. In vitro experiments (Kim et al. Citation2006) have shown that albumin can inhibit T cell adhesion. Graner et al. (Citation2004) found that albumin expressed in mouse tumours can inhibit T cell activation and response in vivo and in vitro, promoting tumour progression. They speculated that immunosuppression may be related to the transport capacity of albumin, and the albumin ship more immunosuppressive molecules to the tumour microenvironment, allowing tumour cells to achieve immune escape. The albumin extracted from the plasma of cancer patients shows similar immunosuppressive ability. However, it has also been reported (Zhang and Houtman Citation2016) that HSA can bind to glycerol monolaurate (GML) and reduce the immunosuppressive effect of GML.
Antithrombotic effects
Cys-34 residues can also bind NO, forming S-nitroso albumin, which may be responsible for the antithrombotic effect of HSA, because this prolongs the biologic activity of NO (Spinella, Sawhney, and Jalan Citation2016; Keaney et al. Citation1993) and permits it to reduce the aggregation of platelets.
Endothelial stabilisation
The antioxidant and anti-inflammatory properties of HSA protect the endothelium from pro-inflammatory factors and reduce endothelial oxidative stress (Spinella, Sawhney, and Jalan Citation2016; Lang et al. Citation2004). For example, the oxidation of HSA increases the risk of cardiovascular disease in the elderly by promoting endothelial aging and injury. This was illustrated by Anraku et al. (Citation2011), who used a vascular endothelial cell model to demonstrate that Cys-34 can mediate a reduction in vascular endothelial oxidative stress and protect the vascular endothelium from damage. Furthermore, clinical studies have confirmed that HSA can improve systemic hemodynamics in patients with spontaneous bacterial peritonitis by reducing endothelial cell activation (Fernández et al. Citation2005). Finally, animal studies have also shown that HSA concentration is associated with endothelial dysfunction (Garcia-Martinez et al. Citation2015). Thus, the binding of NO by HSA provides a possible mechanism for its involvement in hemodynamic regulation.
Adjustment of capillary permeability
Albumin can directly bind interstitial matrix and sub-endothelial molecules, which affects the permeability of capillaries to macromolecules. And albumin can also bind to arachidonic acid, which increases capillary permeability (Evans Citation2002). Animal studies have shown that albumin administration can reduce the permeability of the mesenteric capillary bed in endotoxemia (Evans Citation2002).
Methods of assessing albumin function
Measurement of albumin binding capacity
Fatty acid-binding capacity
The long-chain fatty acid-binding ability of albumin can be quantified using electron paramagnetic resonance (EPR) techniques (Jalan et al. Citation2009; Haeri et al. Citation2019). There are seven fatty acid-binding sites on an albumin molecule, of which three have high affinity and the other four have lower affinity. First, 16-Hexyl stearic acid is used as a spin label and is added to plasma samples at a specific concentration, then ethanol is added as a polar reagent and the mixture is incubated for 10 min. When the albumin structure is broken down, the free spin label increases and can be detected and recorded using EPR spectroscopy. ‘H’ and ‘L’ are used to represent the affinity of the high and low affinity fatty acid binding sites, respectively, and the ratio H/L reflects conformational changes in the albumin molecule (Ge, Liao, et al. Citation2016).
Dansylsarcosine-binding capacity
Albumin contains two major binding sites: site I, where the major heterocyclic complexes and dicarboxylic acids (such as arachidic acid, warfarin, and bilirubin) bind, and site II, which binds aromatic acids, such as diazepam, tryptophan, and guanidine. The binding properties of site II can be assessed using dansylsarcosine (DS) as a ligand, and Klammt et al. (Citation2008) have described a method for determining DS binding. Samples and a standard preparation of albumin as control are first reacted with the binding site II-specific fluorescent label (DS), then unbound DS molecules are removed by ultrafiltration. The same volume and concentration of albumin solution is added as a fluorescence amplifier, and the albumin-binding capacity (ABiC) (Klammt et al. Citation2008; Klammt et al. Citation2012) is determined using a spectrophotometer. ABiC (%) = fluorescence in the filtrate of the reference/fluorescence in the filtrate of the sample × 100%. Similar measurements can also be made using a high-performance liquid chromatography (HPLC) system (Oettl et al. Citation2013).
Metal ion-binding capacity
The metal ion-binding ability of the N-terminal metal ion binding site can be assessed using cobalt, and this is a method of determining the proportion of ischaemic modified albumin (IMA) in plasma (Jalan et al. Citation2009; Ge, Liao, et al. Citation2016). IMA is a marker of oxidative stress, which was initially studied in cardiac ischaemia, but which has recently been found to be associated with diabetes, tobacco-related oxidative stress, and perinatal asphyxia. IMA has been found to be associated with liver diseases, including acute liver injury, hepatitis, cirrhosis, and liver failure. Bar-Or, Lau, and Winkler (Citation2000) have described a method for determining IMA using the metal-binding ability of albumin. Normal albumin binds cobalt and dithiothreitol is added to allow it to react with free cobalt in the solution, then the free cobalt concentration is quantified by spectrophotometry. The free cobalt concentration is directly proportional to the IMA concentration, and therefore the IMA can be calculated on the basis of the difference between the samples to which dithiothreitol has or has not been added. Thus, the higher the absorbance, the larger the amount of cobalt that is not bound to albumin, and the lower the metal-binding ability of the albumin in the sample.
Antioxidant capacity
According to the oxidation state of Cys-34, HSA can be divided into three categories: HMA, HNA1, and HNA2. Increases in HNA1 and HNA2 have been documented in liver disease and are associated with the progression of liver disease, which imply a reduction in the antioxidant capacity of albumin. HMA, HNA1, and HNA2 can be separated and quantified using HPLC (Oettl et al. Citation2013) and fluorescence detection.
Isoforms of HSA
Post-translational modification (PTM) generates multiple isoforms of albumin that have diverse structures and play different roles in liver diseases. PTM is primarily studied using mass spectrometry methods (Baraka-Vidot et al. Citation2015), such as matrix-assisted laser desorption/ionization tandem mass spectrometry (MALDI-MS/MS) and liquid chromatography/mass spectrometry (LC/MS) (Naldi et al. Citation2017). A change in the chemical group of an amino acid side chain alters the molecular weight of the protein and this can be specifically identified using mass spectrometry.
Effects of cirrhosis on albumin function
Patients with advanced cirrhosis usually demonstrate severe deterioration in liver function, with cirrhosis deaths increasing in recent years (Saviano and Baumert Citation2019). The synthesis of albumin is impaired, which results in a reduction in albumin concentration, and a consequent poor prognosis. Recent studies have shown that the function of albumin is impaired in a number of ways and that this is associated with the clinical features of cirrhosis. In a study of 34 patients with cirrhosis (Jalan et al. Citation2009), the transport efficiency, detoxification efficiency, and metal-chelating ability of albumin were all lower in cirrhosis. The metal-chelating ability of patients with cirrhosis was half that of healthy individuals, and the transport efficiency of patients with cirrhosis was approximately one third of that of healthy individuals. Furthermore, the detoxification efficiency of patients with cirrhosis was 75% lower than in healthy individuals. After molecular adsorption recirculation system (MARS) treatment, albumin function did not change significantly, which suggests that functional impairments in albumin may be irreversible. In addition, an impairment in the binding function of site II was identified using dansylsarcosine studies in decompensated liver cirrhosis (Oettl et al. Citation2013). In particular, the binding capacity of albumin was independently associated with the bilirubin concentration and international normalised ratio (INR). There are multiple sites on the albumin molecule that can bind bilirubin, which may partially explain this phenomenon, but this association does not exist in patients with sepsis.
A reduction in the binding ability of albumin implies that higher concentrations of harmful substances that require metabolism remain in the blood, which may further aggravate the pathology. Indeed, such alterations in binding are associated with impairment in liver function, the severity of liver injury, and the prognosis. For instance, positive correlations have been identified between IMAR and serum bilirubin, INR, end-stage liver disease (MELD) score, and total antioxidant capacity (TAC) (Chen et al. Citation2011). Albumin treatment improve bilirubin and MELD scores in patients but does not correct IMA and IMAR. Furthermore, IMAR is significantly associated with oxidative stress markers (Jalan et al. Citation2009), meaning that there is an impairment in the plasma antioxidant status of patients with cirrhosis. Moreover, patients with higher IMAR have higher MELD scores, which implies a link between high IMAR and a poor prognosis. It has been reported that IMA is associated with bacterial infection in patients with cirrhosis (Naldi et al. Citation2017). IMA/IMAR is significantly higher in infected patients, implying that these values may be used as an alternative to C-reactive protein (CRP) to identify bacterial infections. In contrast, ascites, kidney damage, and hepatic encephalopathy did not affect IMA/IMAR values. However, IMAR may not be useful for diagnosis or prognosis because it lacks specificity: changes in IMAR can also result from myocardial ischaemia and cerebral ischaemia (Coverdale et al. Citation2018), for example. Thus, more research is needed to assess the usefulness of IMA as a diagnostic and prognostic marker for liver disease.
Oxidative stress plays an important role in cirrhosis: reactive oxygen species are usually present in higher concentrations and the antioxidant Defence mechanisms are weaker. HSA is important in the antioxidant capacity of the body mainly because of its free Cys-34 residue. The status of the Cys-34 residue is indicative of the antioxidant capacity of HSA, and according to the redox state of Cys-34, three components of albumin can be separated by HPLC: non-oxidised HMA, HNA1, and HNA2. Oxidative albumin damage is present in cirrhosis: the proportion of HNA2 is much higher and there is a positive connection between the proportion of HNA2 and the concentrations of bilirubin and C-reactive protein, and the INR (Oettl et al. Citation2013).
Patients with advanced cirrhosis are often hypoproteinemic, but in the early stages of cirrhosis, the albumin concentration is not usually lower than normal. Therefore, it is difficult to assess the severity of the disease using albumin concentration or other related indicators. However, albumin function can be altered at an earlier stage of the disease. We have shown (Ge, Liao, et al. Citation2016) that the fatty acid-binding capacity and metal chelating-ability of albumin are much lower in cirrhosis. In addition, IMAR is higher in livers with advanced fibrosis than in those with mild fibrosis; therefore, it may have potential as a marker of the severity of fibrosis (Cakir et al. Citation2012; Yavuz et al. Citation2017). However, there are no clear differences in these parameters between early cirrhosis and hepatitis. This may be accounted for by the substantial functional reserve of the liver with respect to the production of functional albumin.
These findings help explain why albumin infusion is therapeutically useful for specific liver diseases. Currently, albumin infusion is used to treat hepatorenal syndrome and spontaneous bacterial peritonitis, in which it principally supplements the plasma volume, but its benefits likely go far beyond this (Arroyo, García-Martinez, and Salvatella Citation2014; Taverna et al. Citation2013). Albumin supplementation may compensate for impairments in endogenous albumin function in such patients, ameliorating defects in transportation, detoxification, and antioxidant function, thereby improving the prognosis of the patients. As an example, hepatic encephalopathy (HE) is a common complication of cirrhosis that involves higher ammonia concentrations, inflammation, and circulatory disorders. A clinical study (Jalan and Kapoor Citation2004) that compared patients with HE treated with 4.5% HSA or colloid found that the improvement in HE was greater in patients treated with HSA, and was accompanied by reductions in oxidative stress markers. Spontaneous bacterial peritonitis represents another example. This is a common infectious condition in patients with cirrhosis and ascites that is usually accompanied by a systemic inflammatory response, and which can subsequently progress to multiple organ failure. Salerno, Navickis, and Wilkes (Citation2013) conducted a meta-analysis to appraise the efficacy of albumin infusion in patients with spontaneous bacterial peritonitis, and found that when used in addition to antibiotics, HSA reduces the incidence of renal failure and mortality, which may be partially attributable to the non-oncotic effects of HSA.
Effects of liver failure on albumin function
Acute-on-chronic liver failure (ACLF) syndrome is the cause of death in most patients with cirrhosis. Using the EASL‐Chronic Liver Failure (EASL-CLIF) Alliance definition, the reported global ACLF mortality is between 30 and 50% (Arroyo et al. Citation2016). In China (Li et al. Citation2016), the 28-day mortality rate for patients with ACLF varies from 23.6% to 60.2%, depending on its severity. However, there is a lack of suitable early predictors of ACLF, making the early diagnosis and assessment of disease severity difficult. Oettl et al. (Citation2013) have studied oxidative albumin damage in patients with chronic liver failure and found that the oxidation state of albumin and the binding capacity of site II in patients with liver failure is significantly impaired. The percentage of oxidised albumin is significantly higher in these patients than in healthy individuals, the proportion of HNA2 is directly related to their prognosis, and the 90-day mortality is significantly higher in patients with a proportion of HNA2 > 12%. These researchers also compared the accuracy with which HNA2 and MELD could be used to predict survival, and found that HNA2 is more effective at predicting short-term (30-day) survival than MELD. Fortunately, although the oxidation of HNA2 is irreversible, the albumin in these patients is still mainly in the form of reduced HMA and reversibly-oxidised HNA1. Jalan et al. (Citation2009) have also shown evidence of impaired albumin function in patients with liver failure. They evaluated 34 patients with cirrhosis, including 20 patients with ACLF, and found that the fatty acid-binding capacity of albumin is significantly lower in those patients, whereas IMA is higher.
In vitro liver support systems, such as MARS, remove toxins, including bile acids and bilirubin, from patients with liver failure (Hanish et al. Citation2017; Saliba et al. Citation2013), but do not provide a significant survival benefit in patients with ACLF (Jalan et al. Citation2009). MARS can ‘regenerate’ albumin, but does not remove damaged albumin (permanently oxidised HNA2), and subsequent studies should pay more attention to the severity of the oxidative damage to albumin.
Artificial liver is an effective method for treating liver failure. The ability to synthesise and secrete albumin is a vital indicator for testing the function of artificial liver (Su et al. Citation2014; Damania et al. Citation2018; Du et al. Citation2014; Che Abdullah et al. Citation2014), but researchers only detect the concentration of albumin. Whether albumin synthesised by the artificial liver exhibits the same biological properties as albumin in physiological state is unknown. Albumin function may become an effective indicator for the biological characteristics of the in vitro liver tissue model.
Effects of non-alcoholic fatty liver disease on albumin function
The prevalence of NAFLD is continuing to increase, such that it is now one of the most common chronic liver diseases worldwide. NAFLD is a progressive disease, progressing from simple steatosis to non-alcoholic steatohepatitis, fibrosis, cirrhosis, and hepatocellular carcinoma (Adinolfi, Marrone, and Rinaldi Citation2018; Lonardo et al. Citation2018). And recent literatures report that it is associated with various diseases such as cardiovascular disease (Lonardo et al. Citation2018; Adams et al. Citation2017). Therefore, early diagnosis of NAFLD is essential. However, routinely measured biochemical parameters, such as albumin concentration and liver enzyme activities, are normal in the early stage of NAFLD, making it impossible for clinicians to detect early liver damage using non-invasive methods. We previously studied 23 patients with NAFLD (Ge, Liao, et al. Citation2016) and found that the binding capacity of albumin, including for metals and fatty acids, decreases before the total plasma albumin or bilirubin concentration, or the prothrombin time show abnormalities. In addition, the H/L ratio was used to characterise conformational changes in HSA, and showed that indeed the albumin conformation in NAFLD patients does change. Interestingly, however, we found no correlation between the metal-binding capacity of albumin and its ability to bind fatty acids, suggesting that there are differences in the modifications to the binding sites of albumin during the progression of liver disease. Non-invasive clinical assessment of NAFLD is needed for its diagnosis (Yoneda, Imajo, and Nakajima Citation2018). Thus, albumin binding function may be used as an indicator of early liver injury, but whether this is superior to other indicators for the diagnosis of NAFLD requires further investigation, given the rising prevalence of NAFLD.
Effects of hepatitis on albumin function
Viral hepatitis is an important risk factor for cirrhosis and liver cancer, and it’s one of the leading causes of human death and is becoming an increasingly serious problem of world health. But just like NAFLD, conventional non-invasive measurements cannot detect injury caused by early hepatitis. Impairments in metal- and fatty acid-binding by albumin are found in patients with hepatitis B and C, and these occur before other routine parameters become abnormal (Ge, Liao, et al. Citation2016). Furthermore, these changes are more severe than in NAFLD patients. A study (Yavuz et al. Citation2017) of 74 patients with chronic hepatitis B and 25 patients with HBV-induced cirrhosis found that serum IMA concentration and IMAR was higher in these patients than healthy controls, and both groups demonstrated liver fibrosis. Therefore, the authors proposed the use of IMA and IMAR as non-invasive markers of liver fibrosis in HBV patients. Similar results have also been reported for children with chronic liver disease (Cakir et al. Citation2012).
Alterations of albumin function in perioperative patients
Liver resection may have a substantial effect on liver function and postoperative liver failure can endanger the lives of patients (Gyoeri et al. Citation2019). Therefore, the preoperative evaluation of liver functional reserve is very important for patients due to undergo hepatectomy. Preoperative albumin concentration can be used as an indicator to predict surgical outcomes (Wiklund Citation2004), but there are few studies on albumin function in perioperative patients. We have previously (Ge, Liao, et al. Citation2016) studied 42 patients with hepatocellular carcinoma and 17 patients with hepatic cavernous haemangioma who underwent surgical resection, and found that hepatectomy has a significant impact on albumin concentration and function, but that the changes in albumin function do not parallel perioperative changes in albumin concentrations. The metal ion- and fatty acid-binding capacity of patients with cirrhosis reached a nadir on postoperative day 3, and then increased gradually to near-preoperative levels by postoperative day 7. However, the albumin concentration was still lower than the preoperative level on postoperative day 7, implying that albumin function is more sensitive to surgery than concentration. However, further research is needed to confirm the usefulness of evaluating perioperative liver function.
The potential mechanisms of impairments in albumin function
Changes in albumin function result from structural changes, the most important cause of which is PTM, which leads to the formation of multiple HSA isoforms (Domenicali et al. Citation2014). Previous studies have focussed on the protein expression of HSA, but changes in protein expression do not accurately reflect the changes in complex biologic systems in vivo; instead, assessment of the PTM of albumin may be more informative. Covalent modification of the amino acid residues of HSA can modify its conformation, physicochemical properties, composition, activity, and stability, which facilitates the various functions of HSA (Maciążek-Jurczyk et al. Citation2018; Muller Citation2018). There are hundreds of known PTMs (Lee and Wu Citation2015), including phosphorylation, ubiquitination, acetylation, and glycosylation (Baraka-Vidot et al. Citation2015), which are involved in cell division and apoptosis (Zamaraev et al. Citation2017), autophagy (Wani et al. Citation2015), the regulation of cell cycle (Eifler and Vertegaal Citation2015), energy metabolism (Stram and Payne Citation2016), and many other cellular processes. The purposes of other less common PTMs, such as SUMOylation (Eifler and Vertegaal Citation2015), O-GlcNAc monoglycosylation (Toleman et al. Citation2018), and ADP-ribosylation (Luscher et al. Citation2018), are also now being reported. To date, most studies of the PTMs of HSA have been of oxidation, saccharification, nitrosylation, C- or N-terminal truncation, or the conversion of cysteine to dehydroalanine (DHA) (Naldi et al. Citation2017). Some of these PTMs have deleterious effects on HSA function, especially binding and antioxidant capacity. For example, the oxidation of Cys-34 is a very common PTM that modifies the ability of HSA to resist oxidation (Turell et al. Citation2014). Saccharification of amino acid residues at sites I and II alters the structure of HSA, which affects its binding ability (Monacelli et al. Citation2013). Furthermore, N-terminal truncation affects the ability of HSA to bind metal ions (Taverna et al. Citation2013). Recent studies have found that PTM of HSA is associated with a variety of liver diseases, and patients with cirrhosis demonstrate extensive PTMs at several sites of HSA. Domenicali et al. (Citation2014) studied the PTMs of HSA in 168 patients with cirrhosis, identified seven isoforms of HSA, and found that in these patients, the proportion of standard isoforms was significantly lower, whereas the proportion of isoforms with structural changes was significantly higher. Furthermore, these changes were associated with disease severity, the incidence of clinical complications, and survival rate.
Summary and prospects for the future
The importance of albumin depends not only on its concentration but also on its physiologic functions, leading to the growing interest in the concept of ‘effective albumin concentration’. Effective albumin concentration means the concentration of albumin with normal function, which reflects the importance of the biological function of albumin. Based on last research reports, it is speculated that effective albumin concentration is decreased in many kinds of liver disease, though there is still a lack of a comprehensive method for assessing effective albumin concentration. As shown in , many factors, including hepatitis virus, bacterial infection, metabolic disorders, surgical trauma and oxidative stress have deleterious effects on liver function, and some of these are associated with PTMs of albumin, which can cause three-dimensional structural changes and consequent dysfunction. Studies of impairments in albumin function have shed new light on the diagnosis, evaluation and treatment of patients with liver diseases, especially those in the early stage of liver diseases, such as viral hepatitis and NAFLD.
Figure 2. The alterations of albumin function in liver diseases. Many factors including hepatitis virus, alcohol intake, metabolic disorders, surgical trauma and oxidative stress result in the PTMs of albumin. The impaired three-dimensional structure will cause decreased albumin function in maintaining plasma oncotic pressure, dissolving, binding and transporting endogenous and exogenous molecules, antioxidant, anti-inflammatory, haemostatic effects, endothelial stabilisation and adjusting capillary permeability, which has an impact on a variety of liver diseases. PTM, Post-translational modifications

However, it is unclear whether impairments in albumin function are a component of the pathogenesis or are a consequence of liver disease, and the specific mechanisms involved remain to be established. In part, because the number of patients included in previous studies has been relatively small; therefore, larger studies are required. Moreover, albumin function has not been studied in many liver diseases, such as liver cancer, liver transplantation, autoimmune hepatitis, drug-induced liver damage, and hereditary metabolic liver disease. Thus, more high-quality research is urgently needed in this field.
Authors’ contributions
Yilei Mao proposed the study. Lejia Sun, Huanhuan Yin and Meixi Liu wrote the first manuscript. Meixi Liu and Gang Xu made charts and illustrations. Xiaoxiang Zhou, Penglei Ge and Huayu Yang collected the literature and revised the manuscript. All authors contributed to the design and interpretation of the study and to further drafts.
Disclosure statement
No potential conflict of interest was reported by the authors.
Additional information
Funding
References
- Adams LA, Anstee QM, Tilg H, Targher G. Non-Alcoholic Fatty Liver Disease and Its Relationship With Cardiovascular Disease and Other Extrahepatic Diseases. Gut 2017;66(6):1138–1153.
- Adinolfi LE, Marrone A, Rinaldi L. Non-Alcoholic Fatty Liver Disease: Beyond the Liver is an Emerging Multifaceted Systemic Disease. HepatoBiliary Surgery and Nutrition 2018;7(2):143–146.
- Anraku M, Takeuchi K, Watanabe H, Kadowaki D, Kitamura K, Tomita K, Kuniyasu A, Suenaga A, Maruyama T, Otagiri M. Quantitative Analysis Of Cysteine-34 on the Anitioxidative Properties of Human Serum Albumin in Hemodialysis Patients. Journal of Pharmaceutical Sciences 2011;100(9):3968–3976.
- Arroyo V, García-Martinez R, Salvatella X. Human Serum Albumin, Systemic Inflammation, and Cirrhosis. Journal of Hepatology 2014;61(2):396–407.
- Arroyo V, Moreau R, Kamath PS, Jalan R, Ginès P, Nevens F, Fernández J, To U, García-Tsao G, Schnabl B. Acute-on-Chronic Liver Failure in Cirrhosis. Nature Reviews Disease Primers 2016;2(1):16041.
- Artigas A, Wernerman J, Arroyo V, Vincent JL, Levy M. Role of Albumin in Diseases Associated with Severe Systemic Inflammation: Pathophysiologic and Clinical Evidence in Sepsis and in Decompensated Cirrhosis. Journal of Critical Care 2016;33:62–70.
- Baraka-Vidot J, Planesse C, Meilhac O, Militello V, van den Elsen J, Bourdon E, Rondeau P. Glycation Alters Ligand Binding, Enzymatic, and Pharmacological Properties of Human Albumin. Biochemistry 2015;54(19):3051–3062.
- Bar-Or D, Lau E, Winkler JV. A Novel Assay for Cobalt-Albumin Binding and its Potential as a Marker for Myocardial Ischemia-A Preliminary Report. The Journal of Emergency Medicine 2000;19(4):311–315.
- Cakir M, Karahan SC, Mentese A, Sag E, Cobanoglu U, Polat TB, Erduran E. Ischemia-Modified Albumin Levels in Children with Chronic Liver Disease. Gut and Liver 2012;6(1):92–97.
- Che Abdullah CA, Azad CL, Ovalle-Robles R, Fang S, Lima MD, Lepró X, Collins S, et al. Primary Liver Cells Cultured on Carbon Nanotube Substrates for Liver Tissue Engineering and Drug Discovery Applications. ACS Applied Materials and Interfaces 2014;6(13):10373–10380.
- Chen CY, Tsai WL, Lin PJ, Shiesh SC. The Value of Serum Ischemia-Modified Albumin for Assessing Liver Function in Patients with Chronic Liver Disease. Clinical Chemistry and Laboratory Medicine 2011;49(11):1817–1821.
- Coverdale JPC, Katundu KGH, Sobczak AIS, Arya S, Blindauer CA, Stewart AJ. Ischemia-Modified Albumin: Crosstalk Between Fatty Acid and Cobalt Binding. Prostaglandins Leukot Essent Fatty Acids 2018;135:147–157.
- Damania A, Kumar A, Teotia AK, Kimura H, Kamihira M, Ijima H, Sarin SK, Kumar A. Decellularized Liver Matrix-Modified Cryogel Scaffolds as Potential Hepatocyte Carriers in Bioartificial Liver Support Systems and Implantable Liver Constructs. ACS Applied Materials and Interfaces 2018;10(1):114–126.
- Domenicali M, Baldassarre M, Giannone FA, Naldi M, Mastroroberto M, Biselli M, Laggetta M, et al. Posttranscriptional Changes of Serum Albumin: Clinical and Prognostic Significance in Hospitalized Patients with Cirrhosis. Hepatology 2014;60(6):1851–1860.
- Du C, Narayanan K, Leong MF, Wan AC. Induced Pluripotent Stem Cell-Derived Hepatocytes and Endothelial Cells in Multi-Component Hydrogel Fibers for Liver Tissue Engineering. Biomaterials 2014;35(23):6006–6014.
- Eifler K, Vertegaal A. SUMOylation-Mediated Regulation of Cell Cycle Progression and Cancer. Trends in Biochemical Sciences 2015;40(12):779–793.
- Evans TW. Review Article: Albumin as a Drug–Biological Effects of Albumin Unrelated to Oncotic Pressure. Alimentary Pharmacology and Therapeutics 2002;16(Suppl 5):6–11.
- Fanali G, di Masi A, Trezza V, Marino M, Fasano M, Ascenzi P. Human serum albumin: From bench to bedside. Molecular Aspects of Medicine 2012;33(3):209–290.
- Farrugia A. Albumin Usage in Clinical Medicine: Tradition or Therapeutic?. Transfusion Medicine Reviews 2010;24(1):53–63.
- Fernández J, Monteagudo J, Bargallo X, Jiménez W, Bosch J, Arroyo V, Navasa M. A Randomized Unblinded Pilot Study Comparing Albumin Versus Hydroxyethyl Starch in Spontaneous Bacterial Peritonitis. Hepatology 2005;42(3):627–634.
- Garcia-Martinez R, Andreola F, Mehta G, Poulton K, Oria M, Jover M, Soeda J, et al. Immunomodulatory and Antioxidant Function Of Albumin Stabilises the Endothelium and Improves Survival in a Rodent Model of Chronic Liver Failure. Journal of Hepatology 2015;62(4):799–806.
- Garcia-Martinez R, Caraceni P, Bernardi M, Gines P, Arroyo V, Jalan R. Albumin: Pathophysiologic Basis of its Role in the Treatment of Cirrhosis and its Complications. Hepatology 2013;58(5):1836–1846.
- Ge P, Liao W, Yang H, Jingfen Lu, Wei Xu, Dandan Hu, Shunda Du. Reduction in Albumin Binding Function Following Liver Resection in Patients With and Without Cirrhosis. Translational Cancer Research 2016;5(6):756–764.
- Ge P, Yang H, Lu J, Liao W, Du S, Xu Y, Xu H, et al. Albumin Binding Function: The Potential Earliest Indicator for Liver Function Damage. Gastroenterology Research and Practice 2016;2016:1.
- Graner MW, Likhacheva A, Davis J, Raymond A, Brandenberger J, Romanoski A, Thompson S, Akporiaye E, Katsanis E. Cargo From Tumor-Expressed Albumin Inhibits T-cell Activation and Responses. Cancer Research 2004;64(21):8085–8092.
- Gyoeri GP, Pereyra D, Braunwarth E, Ammann M, Jonas P, Offensperger F, Klinglmueller F, Baumgartner R, et al. The 3-60 Criteria Challenge Established Predictors of Postoperative Mortality and Enable Timely Therapeutic Intervention After Liver Resection. HepatoBiliary Surgery and Nutrition 2019;8(2):111–124.
- Haeri HH, Schunk B, Tomaszewski J, Schimm H, Gelos MJ, Hinderberger D. Fatty Acid Binding to Human Serum Albumin in Blood Serum Characterized by EPR Spectroscopy. ChemistryOpen 2019;8(5):650–656.
- Hanish SI, Stein DM, Scalea JR, Essien EO, Thurman P, Hutson WR, Bartlett ST, Barth RN, Scalea TM. Molecular Adsorbent Recirculating System Effectively Replaces Hepatic Function in Severe Acute Liver Failure. Annals of Surgery 2017;266(4):677–684.
- Jalan R, Bernardi M. Effective Albumin Concentration And Cirrhosis Mortality: From Concept to Reality. Journal of Hepatology 2013;59(5):918–920.
- Jalan R, Kapoor D. Reversal of Diuretic-Induced Hepatic Encephalopathy with Infusion of Albumin but Not Colloid. Clinical Science (London) 2004;106(5):467–474.
- Jalan R, Schnurr K, Mookerjee RP, Sen S, Cheshire L, Hodges S, Muravsky V, Williams R, Matthes G, Davies NA. Alterations in the Functional Capacity of Albumin in Patients with Decompensated Cirrhosis is Associated With Increased Mortality. Hepatology 2009;50(2):555–564.
- Jamtgaard L, Manning SL, Cohn B. Does Albumin Infusion Reduce Renal Impairment and Mortality in Patients With Spontaneous Bacterial Peritonitis?. Annals of Emergency Medicine 2016;67(4):458–459.
- Jing M, Liu R, Yan W, Tan X. Investigations on the Effects of Cu2+ on the Structure and Function of Human Serum Albumin. Luminescence 2016;31(2):557–564.
- Kawakami A, Kubota K, Yamada N, Tagami U, Takehana K, Sonaka I, Suzuki E, Hirayama K. Identification and Characterization of Oxidized Human Serum Albumin. A Slight Structural Change Impairs Its Ligand-Binding And Antioxidant Functions. The FEBS Journal 2006;273(14):3346–3357.
- Keaney JF Jr., Simon DI, Stamler JS, Jaraki O, Scharfstein J, Vita JA, Loscalzo J. NO Forms an Adduct With Serum Albumin that has Endothelium-Derived Relaxing Factor-Like Properties. Journal of Clinical Investigation 1993;91(4):1582–1589.
- Kim YL, Im YJ, Lee YK, Ha NC, Bae YS, Lim SM, Okajima F, Im DS. Albumin Functions as an Inhibitor of T Cell Adhesion In Vitro. Biochemical and Biophysical Research Communications 2006;351(4):953–957.
- Kitano H, Fukui H, Okamoto Y, Kikuchi E, Matsumoto M, Kikukawa M, Morimura M, et al. Role of Albumin and High-Density Lipoprotein as Endotoxin-Binding Proteins in Rats with Acute and Chronic Alcohol Loading. Alcoholism: Clinical and Experimental Research 1996;20(Suppl 1):73a–76a.
- Klammt S, Mitzner SR, Stange J, Loock J, Heemann U, Emmrich J, Reisinger EC, Schmidt R. Improvement of Impaired Albumin Binding Capacity in Acute-On-Chronic Liver Failure by Albumin Dialysis. Liver transplantation 2008;14(9):1333–1339.
- Klammt S, Wojak H-J, Mitzner A, Koball S, Rychly J, Reisinger EC, Mitzner S. Albumin-Binding Capacity (ABiC) is Reduced in Patients with Chronic Kidney Disease Along with an Accumulation of Protein-Bound Uraemic Toxins. The Nephrology Dialysis Transplantation 2012;27(6):2377–2383.
- Lang JD Jr., Figueroa M, Chumley P, Aslan M, Hurt J, Tarpey MM, Alvarez B, Radi R, Freeman BA. Albumin and Hydroxyethyl Starch Modulate Oxidative Inflammatory Injury to Vascular Endothelium. Anesthesiology 2004;100(1):51–58.
- Lee P, Wu X. Review: Modifications of Human Serum Albumin and Their Binding Effect. Current Pharmaceutical Design 2015;21(14):1862–1865.
- Li H, Chen L-Y, Zhang N-n, Li S-T, Zeng B, Pavesi M, Amorós À, et al. Characteristics, Diagnosis and Prognosis of Acute-on-Chronic Liver Failure in Cirrhosis Associated to Hepatitis B. Scientific Reports 2016;6(1):25487.
- Loban A, Kime R, Powers H. Iron-binding Antioxidant Potential of Plasma Albumin. Clinical Science (London) 1997;93(5):445–451.
- Lonardo A, Nascimbeni F, Mantovani A, Targher G. Hypertension, Diabetes, Atherosclerosis and NASH: Cause or Consequence?. Journal of Hepatology 2018;68(2):335–352.
- Luscher B, Butepage M, Eckei L, Krieg S, Verheugd P, Shilton BH. ADP-Ribosylation, a Multifaceted Posttranslational Modification Involved in the Control of Cell Physiology in Health and Disease. Chemical Reviews 2018;118(3):1092–1136.
- Maciążek-Jurczyk M, Szkudlarek A, Chudzik M, Pożycka J, Sułkowska A. Alteration of Human Serum Albumin Binding Properties Induced by Modifications: A Review. Spectrochimica Acta Part A: Molecular and Biomolecular Spectroscopy 2018;188:675–683.
- Martin-Llahi M, Pepin MN, Guevara M, Díaz F, Torre A, Monescillo A, Soriano G, et al. Terlipressin and Albumin vs Albumin in Patients with Cirrhosis and Hepatorenal Syndrome: A Randomized Study. Gastroenterology 2008;134(5):1352–1359.
- Monacelli F, Storace D, D'Arrigo C, Sanguineti R, Borghi R, Pacini D, Furfaro AL, et al. Structural Alterations of Human Serum Albumin Caused by Glycative and Oxidative Stressors Revealed by Circular Dichroism Analysis. International Journal of Molecular Sciences 2013;14(6):10694–10709.
- Moss GS, Lowe RJ, Jilek J, Levine HD. Colloid or Crystalloid in the Resuscitation of Hemorrhagic Shock: A Controlled Clinical Trial. Surgery 1981;89(4):434–438.
- Muller MM. Post-Translational Modifications of Protein Backbones: Unique Functions, Mechanisms, and Challenges. Biochemistry 2018;57(2):177–185.
- Naldi M, Baldassarre M, Domenicali M, Bartolini M, Caraceni P. Structural and Functional Integrity of Human Serum Albumin: Analytical Approaches and Clinical Relevance in Patients with Liver Cirrhosis. Journal of Pharmaceutical and Biomedical Analysis 2017;144:138–153.
- Naldi M, Baldassarre M, Nati M, Laggetta M, Giannone FA, Domenicali M, Bernardi M, Caraceni P, Bertucci C. Mass Spectrometric Characterization of Human Serum Albumin Dimer: A New Potential Biomarker in Chronic Liver Diseases. Journal of Pharmaceutical and Biomedical Analysis 2015;112:169–175.
- Oettl K, Birner-Gruenberger R, Spindelboeck W, Stueger HP, Dorn L, Stadlbauer V, Putz-Bankuti C, et al. Oxidative Albumin Damage in Chronic Liver Failure: Relation to Albumin Binding Capacity, Liver Dysfunction and Survival. Journal of Hepatology 2013;59(5):978–983.
- Oettl K, Stauber RE. Physiological and Pathological Changes in the Redox State of Human Serum Albumin Critically Influence its Binding Properties. British Journal of Pharmacology 2007;151(5):580–590.
- Ortega R, Ginès P, Uriz J, Cárdenas A, Calahorra B, De Las Heras D, Guevara M, et al. Terlipressin Therapy With and Without Albumin for Patients with Hepatorenal Syndrome: Results of a Prospective, Nonrandomized Study. Hepatology 2002;36(4):941–948.
- Otagiri M, Chuang V. Pharmaceutically Important Pre- and Posttranslational Modifications on Human Serum Albumin. Biological and Pharmaceutical Bulletin 2009;32(4):527–534.
- Pieniazek A, Gwozdzinski L, Zbrog Z, Gwozdzinski K. Alterations in Conformational State of Albumin in Plasma in Chronic Hemodialyzed Patients. PLOS One 2018;13(3):e0192268.
- Salerno F, Navickis RJ, Wilkes MM. Albumin Infusion Improves Outcomes of Patients with Spontaneous Bacterial Peritonitis: A Meta-Analysis of Randomized Trials. Clinical Gastroenterology and Hepatology 2013;11(2):123–130.e1.
- Saliba F, Camus C, Durand F, Mathurin P, Letierce A, Delafosse B, Barange K, et al. Albumin Dialysis with a Noncell Artificial Liver Support Device in Patients with Acute Liver Failure: A Randomized, Controlled Trial. Annals of Internal Medicine 2013;159(8):522–531.
- Saviano A, Baumert T. Nutrition. Mortality From Liver Cirrhosis and HCC in the DAA Era: Success in Viral Control is Darkened By Raise Of Metabolic Disease. HepatoBiliary Surgery and Nutrition 2019;8(3):307–310.
- Setoyama H, Tanaka M, Nagumo K, Naoe H, Watanabe T, Yoshimaru Y, Tateyama M, et al. Oral Branched-Chain Amino Acid Granules Improve Structure and Function of Human Serum Albumin in Cirrhotic Patients. Journal of Gastroenterology 2017;52(6):754–765.
- Shalish W, Olivier F, Aly H, Sant'Anna G. Uses and Misuses of Albumin During Resuscitation and in the Neonatal Intensive Care Unit. Seminars in Fetal and Neonatal Medicine 2017;22(5):328–335.
- Sleep D. Albumin and its Application in Drug Delivery. Expert Opinion on Drug Delivery 2015;12(5):793–812.
- Sort P, Navasa M, Arroyo V, Aldeguer X., Planas R., Ruiz-del-Arbol L., et al. Effect of Intravenous Albumin on Renal Impairment and Mortality in Patients with Cirrhosis and Spontaneous Bacterial Peritonitis. The New England Journal of Medicine 1999;341(6):403–409.
- Spinella R, Sawhney R, Jalan R. Albumin in Chronic Liver Disease: Structure, Functions and Therapeutic Implications. Hepatology International 2016;10(1):124–132.
- Stram AR, Payne RM. Post-Translational Modifications in Mitochondria: Protein Signaling in the Powerhouse. Cellular and Molecular Life Sciences 2016;73(21):4063–4073.
- Su Z, Li P, Wu B, Wang Y, Liu G, Zeng H, Li Z, Wei X. PHBVHHx Scaffolds Loaded with Umbilical Cord-Derived Mesenchymal Stem Cells Or Hepatocyte-Like Cells Differentiated from These Cells for Liver Tissue Engineering. Materials Science and Engineering C-Materials for Biological Applications 2014;45:374–382.
- Taverna M, Marie A-L, Mira J-P, Guidet B. Specific Antioxidant Properties of Human Serum Albumin. Annals of Intensive Care 2013;3(1):4.
- Toleman CA, Schumacher MA, Yu SH, Zeng W, Cox NJ, Smith TJ, Soderblom EJ, Wands AM, Kohler JJ, Boyce M. Structural Basis of O-GlcNAc Recognition by Mammalian 14-3-3 Proteins. Proceedings of the National Academy of Sciences of the United States of America 2018;115(23):5956–5961.
- Turell L, Botti H, Bonilla L, Torres MJ, Schopfer F, Freeman BA, Armas L, Ricciardi A, Alvarez B, Radi R. HPLC Separation of Human Serum Albumin Isoforms Based on Their Isoelectric Points. Journal of Chromatography B: Analytical Technologies in the Biomedical and Life Sciences 2014;944:144–151.
- Vincent JL, Russell JA, Jacob M, Martin G, Guidet B, Wernerman J, Ferrer R, McCluskey SA, Gattinoni L. Albumin Administration in the Acutely Ill: What is New and Where Next?. Critical Care 2014;18(4):231.
- Wani WY, Boyer-Guittaut M, Dodson M, Chatham J, Darley-Usmar V, Zhang J. Regulation of Autophagy by Protein Post-Translational Modification. Laboratory Investigation 2015;95(1):14–25.
- Wiklund RA. Preoperative Preparation of Patients with Advanced Liver Disease. Critical Care Medicine 2004;32(Supplement):S106–S15.
- Wilkes MM, Navickis RJ. Patient Survival After Human Albumin Administration. A Meta-Analysis of Randomized, Controlled Trials. Annals of Internal Medicine 2001;135(3):149–164.
- Yavuz F, Biyik M, Asil M, Dertli R, Demir A, Polat H, Uysal S, Ataseven H. Serum Ischemic Modified Albumin (IMA) Concentration and IMA/albumin ratio in Patients with Hepatitis B-related Chronic Liver Diseases. Turkısh Journal of Medıcal Scıences 2017;47(3):947–953.
- Yoneda M, Imajo K, Nakajima A. Will the Magnetic Resonance Imaging Proton Density Fat Fraction Replace Liver Biopsy as the Gold Standard for Detecting Steatosis?. HepatoBiliary Surgery and Nutrition 2018;7(4):292–293.
- Zamaraev AV, Kopeina GS, Prokhorova EA, Zhivotovsky B, Lavrik IN. Post-translational Modification of Caspases: The Other Side of Apoptosis Regulation. Trends in Cell Biology 2017;27(5):322–339.
- Zhang MS, Houtman J. Human Serum Albumin (HSA) Suppresses the Effects of Glycerol Monolaurate (GML) on Human T Cell Activation and Function. PLoS One 2016;11(10):e0165083–e.