Abstract
Diabetic retinopathy (DR) is the most common microangiopathic complication of diabetes mellitus, representing a major cause of visual impairment in developed countries. Proliferative DR (PDR) represents the last stage of this extremely complex retinal disease, characterized by the development of neovascularization induced by the abnormal production and release of vascular endothelial growth factor (VEGF). The term VEGF includes different isoforms; VEGF-A represents one of the most important pathogenic factors of DR. Anti-VEGF intravitreal therapies radically changed the outcome of DR, due to combined anti-angiogenic and anti-edematous activities. Nowadays, several anti-VEGF molecules exist, characterized by different pharmacological features and duration. With respect to PDR, although anti-VEGF treatments represented a fundamental step forward in the management of this dramatic complication, a big debate is present in the literature regarding the role of anti-VEGF as substitute of panretinal photocoagulation or if these two approaches may be used in combination. In the present review, we provided an update on VEGF isoforms and their role in DR pathogenesis, on current anti-VEGF molecules and emerging new drugs, and on the current management strategies of PDR. There is an overall agreement regarding the relative advantage provided by anti-VEGF, especially looking at the management of PDR patients requiring vitrectomy, with respect to laser. Based on the current data, laser approaches might be avoided when a perfectly planned anti-VEGF therapeutic strategy can be adopted. Conversely, laser treatment may have a role for those patients unable to guarantee enough compliance to anti-VEGF injections.
VEGF increased production, stimulated by retinal hypoperfusion and ischaemia, is a major pathogenic factor of neovascular complication onset in diabetic retinopathy and of DR stages progression.
Nowadays, several anti-VEGF molecules are available in clinical practice and other molecules are currently under investigation. Each anti-VEGF molecule is characterized by different targets and may interact with multiple biochemical pathways within the eye.
All the data agreed in considering anti-VEGF molecules as a first line choice for the management of diabetic retinopathy. Laser treatments may have a role in selected advanced cases and for those patients unable to guarantee enough compliance to intravitreal treatments schemes.
Key messages
Introduction
Vascular endothelial growth factor (VEGF) is one of the most important mediators involved in the pathogenesis of diabetic retinopathy (DR). The abnormal production and release of VEGF induces vascular endothelial cell proliferation and migration and increased vascular permeability [Citation1]. VEGF is involved in the pathogenesis of DR-related complications, such as diabetic macular edoema (DME); furthermore, it represents a fundamental mediator for the development of retinal neovascularization leading to the possible onset of vitreous haemorrhage and tractional retinal detachment [Citation2]. The term VEGF includes different isoforms, namely VEGF-A, VEGF-B, VEGF-C, VEGF-D, VEGF-E, VEGF-F and PGF (placental growth factor), the first one representing one of the most important pathogenic factors of DR. The introduction of anti-VEGF intravitreal therapies radically changed the course of DR and patients’ outcome, having a remarkable impact on the incidence of legal blindness. Nowadays, several anti-VEGF molecules exist, acting on different metabolic pathways and VEGF isoforms. The usage of anti-VEGF as first line treatment provided undoubted benefits for the management of DME; on the other side, the treatment of the proliferative form is quite controversial. Indeed, a big debate is still present in the literature regarding the role of laser approaches, administered through different strategies (prompt, deferred, etc.) in the anti-VEGF era.
In the present review, we provided an overall description of VEGF-targeting drugs and the acting mechanisms against the development of retinal neovascularization in DR.
Methods
We used keywords to explore all English language human subject articles in the MEDLINE library. The keywords included the following: diabetic retinopathy, exudation, diabetic macular edoema, vascular endothelial growth factor, VEGF, anti-VEGF, intravitreal injections, neovascularization, ischaemia. All the references were carefully examined by two expert researchers (FB, AA), who collated and arranged all the relevant information, bearing in mind this review’s main theme as expressed in the manuscript title.
Vascular endothelial growth factor: biochemical properties and mechanisms of action
VEGF, also known as vascular permeability factor, was firstly described as an endothelial cell-specific mitogen [Citation3]. This group of molecules belong to the cystine-knot superfamily of hormones and extracellular signalling molecules, covering several functions in vertebrates [Citation4]. VEGF is a dimeric glycoprotein of ∼40 kDa and is fundamental in promoting growth metabolic cascades and angiogenesis during the development of the vertebrate retina [Citation5,Citation6]. VEGF family includes VEGF-A, VEGF-B, VEGF-C, VEGF-D, VEGF-E, VEGF-F, and PGF molecules. These mediators originate from the alternative splicing of a common source molecule and are further characterized by different isoforms [Citation7]. Alternative splicing of the human VEGF-A gene provides at least six different isoforms, namely 121, 145, 165, 183, 189, and 206 [Citation8]. VEGF-A121 and VEGF-A165 represent the most expressed forms in mammalians. With respect to VEGF-B, at least two isoforms are known, namely 167 and 186 [Citation9]. On the other side, although largely studied in mouse models, less is known about the VEGF-C and VEGF-D isoforms [Citation10–12]. VEGF-E is a molecule of ∼20 kDa, identified in the genome of Orf virus, a parapoxvirus that affects occasionally humans, generating lesions with angiogenesis [Citation13], whereas VEGF-F is a toxin identified in the snake venom, having several similarities with the other VEGF isoforms [Citation14].
All VEGF isoforms bind to different types of VEGF tyrosine kinase trans-membrane receptors; VEGFR-1/Flt-1 (fms-like tyrosine kinase) and VEGFR-2/KDR/Flk-1 (kinase insert domain containing receptor/fetal liver kinase) are mainly associated with angiogenesis [Citation15], whereas Flt-3/Flk-2 and VEGFR-3/Flt-4 are involved in haematopoiesis and lymphangiogenesis [Citation16].
VEGF-A isoforms exert the most powerful angiogenetic activity, also increasing vascular permeability, resulting in leakage of proteins and other molecules towards the blood vessels [Citation17]. Furthermore, previous findings support the involvement of VEGF-A in neurotrophic and neuroprotective activities [Citation18,Citation19]. The angiogenic role of VEGF-B is quite unclear; this isoform seems to act more as a regulator of cells homeostasis and survival than as a active pro-angiogenic factor [Citation20]. Conversely, VEGF-C has been found to play a major role in the lymphangiogenesis [Citation21], although this isoform can also stimulate the growth of blood vessels [Citation22]. Similarly, VEGF-D showed both angiogenic and lymphangiogenic activities [Citation23,Citation24].
VEGF is considered as the major factor involved in the angiogenesis of the human retina [Citation25], although many other molecules and mediators are involved in angiogenic physiologic processes, turning out to be dysregulated in retinal vascular diseases [Citation26,Citation27]. However, because of the central role of VEGF-A, with respect to the other isoforms, many times researchers and clinicians refer to this subtype as simply VEGF [Citation27].
Overall, different stimuli governed the production and release of VEGF. Its production is up-regulated by different isoforms of hypoxia inducible factor-1 (HIF-1), which are released to promote physiological angiogenesis, whereas other HIF isoforms act more as regulatory mediators of VEGF expression [Citation28]. Moreover, VEGF levels can also be increased by insulin-like growth factor 1 (IGF-1), playing an important role in retinal angiogenesis [Citation29]. In the human retina, VEGF is mainly produced by retinal pigmented epithelium (RPE) cells [Citation30], astrocytes [Citation31], Müller cells [Citation32], endothelium and ganglion cells [Citation33]. In retinal diseases, VEGF release is upregulated by increased hypoxia and oxidative stress [Citation34,Citation35]. Furthermore, the dysregulation of many other mediators is involved in the promotion of VEGF release, including erythropoietin (EPO) [Citation36], angiopoietins 1 and 2 (Ang-1 and Ang-2), Tie2 receptor [Citation15], platelet derived growth factor (PDGF) [Citation37] and advanced glycation end-products [Citation38]. In addition, VEGF isoforms may synergically act to further reinforce the cascades of events leading to VEGF metabolism alterations [Citation39]. On the other hand, VEGF abnormal release is also influenced by the downregulation of inhibitory mediators. For example, neovascularization and exudation processes are enhanced by the alterations of the VEGF/pigment epithelium derived factor (PEDF) ratio. PEDF is more released by the peripheral RPE cells than macular RPE, acting as a major inhibitor of angiogenesis [Citation40,Citation41]. From this point of view, the peripheral ischaemia characterizing several retinal vascular diseases, including DR, might be the basis for VEGF/PEDF ratio dysregulation, thus providing a possible basis to explain why neovascularization and macular edoema mainly occur in the macular region, compared with retinal periphery.
Vascular endothelial growth factor in diabetic retinopathy
DR is the most common microangiopathic complication of diabetes mellitus [Citation2], affecting more than 100 million people worldwide and representing a major cause of visual impairment and legal blindness in developed countries [Citation42]. DR is classically classified as non-proliferative (NPDR) and proliferative (PDR) (). NPDR, further categorized in different stages, is characterized by several alterations including intraretinal haemorrhages, hard and soft exudates, cotton-wool spots, microaneurysms, venous calibre abnormalities, intraretinal microvascular anomalies and both macular and peripheral capillary nonperfusion; the onset of neovascularization characterize the progression towards PDR [Citation2]. Most blindness cases are secondary to posterior PDR complications, such as vitreous haemorrhage and retinal detachment, whereas just 5% of blindness cases can be related to the onset of neovascular glaucoma [Citation43]. The pathogenesis of DR is mainly characterized by the combined presence of neurovascular unit impairment, breakdown of the blood retinal barrier (BRB), inflammation, capillary non-perfusion/ischaemia, and neoangiogenesis [Citation44]. All these anomalies lead to the increased production of angiogenic and inflammatory mediators. In this scenario, VEGF is undoubtedly a major pathogenic element characterizing DR and its complication. In DR, VEGF production is induced in response to ischaemic or hypoxic stimuli, causing several alterations at different levels. Indeed, VEGF increases the phosphorylation of tight junctions’ proteins, thus increasing retinal capillary permeability [Citation45]. VEGF-related hyperpermeability is also caused by the induced modifications of several intercellular molecules, such as occludin, catenins and cadherins [Citation46,Citation47], by the increased transcytosis [Citation48] and by the activated NOS-mediated mechanisms [Citation49]. All these VEGF-related phenomena, mainly governed by VEGF-A isoforms, in combination with an extremely complex cascade of other events and mediators, lead to the onset and progression of a frequent DR-related complication, namely DME [Citation50]. Furthermore, although few studies have been conducted, there are even growing evidences suggesting major roles of VEGF-B and PGF in DR pathogenesis. VEGF-B can promote neovascular phenomena and BRB breakdown towards non-inflammatory mechanisms, promoting potent survival stimuli on vascular and nonvascular cells [Citation51]. Similarly, PGF acts as a powerful pro-angiogenic mediator, showing its serum and ocular concentrations strictly correlated with DR severity and risk of progression [Citation51]. Hence, there is a consensus regarding the role of VEGF inhibition as a mandatory strategy for the management of DR [Citation52].
Figure 1. Angiographic findings in different stages of diabetic retinopathy. (A) Mild form of NPDR characterised by preserved posterior pole and minor capillary non-perfusion changes detected in the extreme periphery. (B) Advanced form of NPDR, showing extensive peripheral capillary non-perfusion and central involvement with several microaneurysms and macular edoema. (C) A case of PDR with extensive peripheral capillary non-perfusion and neovascularizations detected both at the level of the optic nerve head and the retinal periphery. (D) Optical coherence tomography angiography reconstruction of a PDR-related neovascularization.
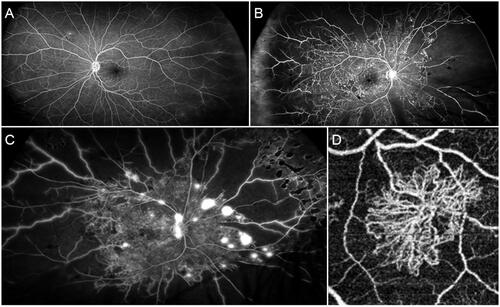
With respect to the neovascular complication, the increased production and release of VEGF activates endothelial cell proliferation and migration, thus promoting neoangiogenesis [Citation53]. Indeed, VEGF molecules activate two tyrosine kinase receptors, namely VEGFR-1 and VEGFR-2, stimulating endothelial proliferation, migration, and survival, thus promoting the progression to PDR [Citation54]. Also in this case, VEGF acts as a major cause of DR-related neovascular process, although being just a part of a more complex pathogenic pathway. Indeed, it was demonstrated that angiopoietin system, over than regulating vascular integrity, it can promote and enhance the effect of VEGF neovascular stimulus [Citation55]. Furthermore, neuropilin-1 (NRP1) was found to act as an isoform-specific receptor for VEGF165 and as a co-receptor of VEGF receptor 2 [Citation56]. In addition, another system implicated on DR pathogenesis and on the enhancement of the neovascular stimulus provided by VEGF is the renin/angiotensin system. Indeed, the severity of DR, expressed both as the rate of progression of NPDR or the progression to PDR, were found strictly related to the activity of the renin/angiotensin system [Citation57].
Anti-vascular endothelial growth factor molecules
Based on all the above-described data, the main therapeutic target of anti-VEGF molecules regards the VEGF-A isoforms. It is worth of notice that endogenous anti-VEGF mechanisms already exist, although resulting impaired in retinal diseases such as DR. We have already described the role of VEGF/PEDF ratio dysregulation causing a reduced modulation of VEGF activity [Citation40,Citation41]. The physiologic anti-VEGF mechanisms represent a topic not deeply explored yet. Scant information came from animal models, reporting, for example, the anti-VEGF activity of VEGF165b isoform [Citation58]. More in detail, VEGF165b seems to inhibit angiogenic stimuli induced by VEGF upregulation and hypoxia, also interfering with the migration and the proliferation of endothelial cells [Citation58]. However, this topic would benefit from further dedicated studies to better define the endogenous anti-VEGF mechanisms occurring in animals and in human retina.
With respect to anti-VEGF drugs, the available molecules currently include: Bevacizumab (Avastin®, Hoffmann-La Roche), Pegaptanib (Macugen, Eyetech/Pfizer), Ranibizumab (Lucentis®, Novartis Pharmaceuticals Canada Inc.), Aflibercept (Eylea®, BAYER Pharma AG, Germany), Conbercept (Chengdu Kanghong Biotech Company, China), Brolucizumab (Beovu®, Novartis Pharmaceuticals Canada Inc.), Abicipar-pegol (Allergan, Inc., Irvine, CA) and Faricimab (Hoffmann-La Roche) ().
Bevacizumab
Bevacizumab (Avastin®, Hoffmann-La Roche) is a fully humanized immunoglobulin G1 (IgG1) molecule of 148 kDa binding VEGF-A isoforms. This antibody was originally developed for cancer therapy [Citation59]; its intravitreal use for retinal diseases is still considered as “off-label” treatment [Citation60]. The mechanisms of action are quite simple; bevacizumab works as a pure anti-VEGF antibody, and its main effect is to block the neovascular stimulus and VEGF-induced increased vascular permeability [Citation61]. Furthermore, bevacizumab can interact with HIF-1, thus interfering with its stimulating effect on VEGF production [Citation62]. Although several studies described bevacizumab as an efficient and cost-effective treatment for retinal diseases [Citation63–65], its usage is partially limited by its “off-label” classification. In 2007, the Diabetic Retinopathy Clinical Research Network (DRCR.net) reported the positive results regarding the employment of bevacizumab in DME on the basis of a phase II clinical trial [Citation66]. Furthermore, the bevacizumab or laser therapy (BOLT) study reported the superiority of bevacizumab in the management of DME, with respect to laser approach [Citation67,Citation68]. Because of some doubts regarding the comparable profile of efficacy and safety of bevacizumab, the current recommendation of the European Society of Retina Specialists is to consider bevacizumab as a second choice, with respect to other anti-VEGF molecules [Citation69].
Pegaptanib
Pegaptanib (Macugen, Eyetech/Pfizer) was the first drug to obtain FDA approval for the treatment of retinal diseases through the intravitreal administration. This molecule is a pegylated‐aptamer binding preferentially the heparin-binding domain of VEGF165 isoform [Citation70]. Although pegaptanib was found efficient in inhibiting the neovascularization process [Citation71], its molecular features strongly limit its ability to block VEGF-related pathways, thus making it a poorly considered therapeutic choice.
Ranibizumab
Ranibizumab (Lucentis®, Novartis Pharmaceuticals Canada Inc.) is a recombinant humanized immunoglobulin G1κ isotype monoclonal antibody fragment (Fab) of 48 kDa binding different VEGF-A isoforms and interfering with the interaction with VEGF receptors 1 and 2. The lack of fragment crystallizable (Fc) domain and the small molecule size might allowed to expand its affinity for more isoforms of VEGF-A (VEGF165, VEGF121, and VEGF110), increasing the penetration of the molecule within the retina and choroid [Citation72,Citation73]. Ranibizumab is characterized by only one binding site for VEGF; for this reason, two molecules of ranibizumab bind to one VEGF dimer [Citation74]. This peculiar configuration allows the ranibizumab/VEGF-A complex having higher stability energy than bevacizumab [Citation75] and greater molecular affinity to VEGF than bevacizumab and aflibercept [Citation76]. Several clinical trials demonstrated high safety and efficacy of ranibizumab in the management of DME, exploring the usage of ranibizumab in different modalities and concentrations, as well as alone or in combination with laser, including READ-2 [Citation77], RESOLVE [Citation78], RESTORE [Citation79], RISE and RIDE [Citation80], LUCIDATE [Citation81], REVEAL [Citation82], RELIGHT [Citation83], RETAIN [Citation84] and READ-3 [Citation85]. DRCR network conducted several multicenter clinical trials analyse similarities and differences of ranibizumab, with respect to other approaches, including other anti-VEGF molecules, corticosteroids, or laser, in the management of DR: Protocol S (ranibizumab vs. laser in PDR) [Citation86,Citation87], Protocol T (ranibizumab vs. aflibercept vs. bevacizumab in DME) [Citation88] and Protocol I (fluocinolone acetonide vs. ranibizumab plus deferred laser in DME) [Citation89] studies. Other meaningful clinical trials involving ranibizumab were: TREX-DME (ranibizumab “treat and extend” regimen with or without laser in DME) [Citation90–92], ROTATE (ranibizumab in persistent DME after bevacizumab treatment) [Citation93], RELATION (ranibizumab plus laser vs. laser alone in DR) [Citation94] and REFINE (ranibizumab vs. laser in DME) [Citation95] studies.
Aflibercept
Aflibercept (Eylea®, BAYER Pharma AG, Germany) is a dimeric glycoprotein of 115 kDa, also known as VEGF Trap. This is obtained from the fusion of the first three Ig domains of VEGFR-1 and the Fc region of human IgG1 [Citation96]. These biochemical properties provide high affinity for VEGF-A isoforms and PGF, as well as relative affinity for VEGF-B. Another version of the molecule, differing from aflibercept only for its excipients and the higher osmolarity, and displaying an almost identical biochemical profile, is Ziv-aflibercept (Zaltrap; Sanofi-Aventis and Regeneron Pharmaceuticals, Inc., Tarrytown, NY, USA) [Citation97]. Although this molecule has been associated with promising effects in macular diseases, Ziv-aflibercept usage is still off-label [Citation98]. The main trials dedicated on the assessment of the efficacy and safety of aflibercept in DR were VIVID-VISTA (aflibercept vs. laser in DME) [Citation99,Citation100], ENDURANCE (extension of VIVID-VISTA studies) [Citation101], APOLLON (real-word data on aflibercept in DME) [Citation102] and PANORAMA (aflibercept in NPDR) [Citation103] studies. Furthermore, the DRCR network investigated the effect of aflibercept on DR progression, complications onset and outcome: Protocol V (aflibercept vs. laser vs. observation in DME) [Citation102] and Protocol W (aflibercept vs sham in preventing vision-threatening complications in NPDR without DME) [Citation103] studies.
Conbercept
Conbercept (Chengdu Kanghong Biotech Company, China) is a molecule belonging to VEGF Trap family. It represents a full human DNA sequence of 143 kDa, obtained from the fusion of extracellular domain 2 of VEGFR-1 and extracellular domains 3 and 4 of VEGFR-2 with the Fc portion of human IgG1 [Citation104,Citation105]. The molecular characteristics of Conbercept are quite similar to aflibercept’s; this molecule differs for the presence of a portion dedicated to VEGFR-2, which was developed to potentially increase the efficacy and stability of Conbercept and to produce relative affinity for VEGF-C [Citation104,Citation105]. It turned out to be superior to laser treatment in DME, as assessed by SAILING study [Citation106]. Further real-life reports described the efficacy and safety of Conbercept for DME management [Citation107–110].
Brolucizumab
Brolucizumab (Beovu®, Novartis Pharmaceuticals Canada Inc.) is a novel single-chain antibody fragment of 26 kDa, characterized by the absence of the Fc portion and developed to reduce molecule size and to improve the affinity for VEGF-A isoforms, compared with the other molecules [Citation111,Citation112]. Brolucizumab has been recently approved for the treatment of neovascular age-related macular degeneration, showing non-inferiority and higher penetrance within the retina and the choroid compared with the other anti-VEGF molecules [Citation113,Citation114]. With respect to DR, the ongoing KITE and KESTREL clinical trials reported preliminary positive results regarding the employment of brolucizumab in DME, compared with aflibercept [Citation115], thus suggesting it will be approved for DR management soon.
Abicipar-pegol
Abicipar-pegol (Allergan, Inc., Irvine, CA, USA) belongs to the family of designed ankyrin repeat proteins (DARPins) molecules, a class of proteins that can mimic antibodies showing high affinity for VEGF target [Citation116]. In more details, abicipar-pegol is a recombinant protein of 34 kDa coupled to a polyethylene glycol fraction binding all VEGF-A isoforms [Citation117]. Its affinity for VEGF-A turned out to be comparable to aflibercept’s but remarkably greater than bevacizumab’s and ranibizumab’s [Citation118]. A phase II clinical trial was completed in 2015 about the employment of abicipar-pegol in DME (ClinicalTrials.gov ID: NCT02186119). However, further studies are warranted to support its role in DME management.
Faricimab
Faricimab (Hoffmann-La Roche) is a novel molecule belonging to the DARPin family. This 150 kDa molecule can simultaneously and independently bind and neutralize both VEGF-A and Ang-2, the latter mechanism interfering with the Ang-1/Tie2 pathway [Citation119]. The Ang-1/Tie2 pathway is a major pathogenic factor in the development of neovascularization and exudation. From this point of view, the multitargets profile of faricimab offers interesting new perspectives for the management of exudative retinal diseases. The phase 2 BOULEVARD trial provided prosing data regarding the employment of faricimab in DME, showing its superiority in terms of visual gain, compared with ranibizumab [Citation120]. Furthermore, two Phase 3 clinical trials, RHINE and YOSEMITE (NCT03622593 and NCT03622580, respectively) are currently ongoing to compare the efficacy of faricimab to aflibercept [Citation121]. However, also in this case, further studies are needed to draw more definite conclusions about the role of faricimab in DME management.
Emerging anti-VEGF molecules
In this section, we would just mention two new anti-VEGF molecules currently under investigation. KSI-301 (KODIAK sciences, Palo Alto, CA), is a new generation antibody biopolymer conjugate, under study in a phase 1 b trial (NCT03790852) and in a DAZZLE phase 2 trial (NCT04049266), obtained from the combination of humanized anti-VEGF monoclonal antibody and a phosphorylcholine-based polymer, specifically designed to increase the duration of anti-VEGF activity. OPT-302 is a VEGF-C/D inhibitor, currently under investigation for exudative age-related macular degeneration in a Phase 2 b clinical trial (NCT03345082). In addition, a Phase 3 clinical trial (NCT03610646) is currently ongoing comparing aflibercept to intravitreal MYL-1701P, a recombinant fusion protein that is an aflibercept biosimilar [Citation121].
All the above-mentioned clinical trials are extensively reported in .
Table 1. Main clinical trials dedicated on the assessment of anti-VEGF molecules in diabetic retinopathy. The order of appearance follows the year of publication.
The role of anti-VEGF in DR-related retinal neovascularization
PDR is an extremely complex and vision-threating stage of DR. The management of this complicated form of DR has been mainly based on the employment of panretinal photocoagulation. The irreversible annihilation of peripheral ischaemic retina is associated with decreased production of VEGF and stabilization of the central retina, although having a remarkable impact on the visual field. Most of the above-described studies tried to assess the effect of anti-VEGF treatments on peripheral ischaemia and regression of the neovascularizations. The main question regards the possible replacement of panretinal photocoagulation with anti-VEGF injections alone. A Cochrane meta-analysis performed in 2014 reported low level of evidence regarding safety and efficacy of anti-VEGF in PDR, although the employment of intravitreal injections was associated with moderate reduction of the risk of intraocular bleeding [Citation128]. A comparable low level of evidence was reported by another meta-analysis, although highlighting the higher morpho-functional outcome and benefits on vitrectomy setting obtained from anti-VEGF injections administered as adjuncts to panretinal photocoagulation [Citation129]. The PROTEUS study assessed the role of adjunctive ranibizumab injections on panretinal photocoagulation vs laser treatment alone, reporting significant improvements of the visual acuity and fluorescein angiography features in PDR eyes undergoing combined approach [Citation124]. DRCR Protocol S was a clinical trial specifically designed to compare panretinal photocoagulation to ranibizumab injections in PDR [Citation86,Citation87]. Overall, this study showed ranibizumab being non inferior to panretinal photocoagulation for PDR management. Eyes treated with laser showed higher risk of PDR progression, DME onset, and higher need of vitrectomy, compared with eyes treated by ranibizumab injections. Furthermore, Protocol S highlighted the importance of the starting PDR severity, associated with the presence of further complications, such as epiretinal membrane, neovascularization of the disc with neovascularization elsewhere, and vitreous haemorrhage on determining the achievable outcome [Citation130]. The same attempt to compare panretinal photocoagulation with anti-VEGF injections was assessed by the CLARITY study, a phase 2 b, single-blind, noninferiority trial comparing aflibercept to panretinal photocoagulation in newly diagnosed or previously laser-treated active PDR [Citation123]. Also in this case, the employment of anti-VEGF injections was associated with better outcome, compared with only laser-treated eyes, especially looking at neovascularization regression, visual acuity values and the need of vitrectomy. DRCR Protocol N was a phase 3, double-masked, randomized, multicenter clinical trial comparing intravitreal ranibizumab with intravitreal saline injections on vitrectomy rates for vitreous haemorrhage from PDR [Citation122]. Because of the low overall rate of vitrectomies, the study could not detect differences in vitrectomy rates between groups. However, it showed how ranibizumab group showed better clinical outcome than eyes treated by saline injections. The non-inferiority of ranibizumab, compared with panretinal photocoagulation to manage PDR was furtherly confirmed by the five-year report of the DRCR network [Citation87], which also reported relatively higher probability to develop DME and to have worse visual field in laser-treated group. Moreover, the recent RECOVERY study showed a statistically significant impact of anti-VEGF injections (in this case of aflibercept) on the improvement in DR severity progression registered after one year of follow-up in PDR eyes without DME [Citation127]. Further recent meta-analysis studies provided even more support on the fact that, although not reaching enough level of statistical evidence, the use of anti-VEGF in PDR is associated with better visual outcome and less incidence of PDR-related complications, if compared with panretinal photocoagulation alone [Citation131,Citation132].
Overall considering all these findings, either anti-VEGF therapy or panretinal photocoagulation are feasible treatments for PDR patients. If panretinal photocoagulation was often criticized as inducing extensive loss of the visual field, the 5-year report of DRCR Protocol S highlighted a crucial point. Indeed, Maguire and colleagues [Citation133] showed that, if the deterioration of the visual field was higher in the PDR group treated by laser during the first year, if compared with the eyes treated by anti-VEGF injections, the long-term visual field outcome was statistically similar between both groups at the end of the 5-year follow-up. These findings, showing similar long-term effect of laser and peripheral ischaemia on the visual field, further reinforced the role either of anti-VEGF and panretinal photocoagulation as effective treatments for PDR. As concluded by DRCR network, the therapeutic strategy for PDR should consider treatment recommendations, the relative advantages of each treatment approach and patients’ compliance with follow-up planning [Citation134]. The other side of the medal is to always bear in mind the possible, although rare, risk of “anti-VEGF crunch syndrome”. This infrequent complication occurring in PDR is characterized by the progressive worsening of the fibrovascular tractional retinal detachment following anti-VEGF injections. This condition is still poorly defined, because of the very low incidence and the presence of many possible confounding factors, including panretinal photocoagulation. It might be determined by the fibrovascular regression induced by anti-VEGF injections, causing increased fibrosis leading to the worsening of the tractional component of the retinal detachment. As expected, the surgical approach and visual outcome are worse than PDR eyes not complicated by this occurrence [Citation135]. The main interventional clinical trials specifically focussed on PDR are reported in .
Table 2. Interventional clinical trials specifically focussed on proliferative diabetic retinopathy.
Final remarks and conclusions
Neovascular complication may have a remarkable negative impact on patients’ management and visual outcome in DR and is characterized by increased production of VEGF as a major causative factor. In the present review, we provided an overall description of the use of anti-VEGF molecules for the management of PDR, focussing on the current drugs, the molecules under investigation and the possible targets ruling the neovascular process and the development of further complications. It is unquestionable that the introduction of anti-VEGF therapy radically changed the management and prognosis of all the stages of DR, including PDR. Before the anti-VEGF era, the only therapeutic approach was represented by laser, performed on retinal periphery, through panretinal photocoagulation, or at the posterior pole, through focal/grid treatments. Laser approaches turned out to be useful in managing DME and neovascular progression, although the irreversible demolitive effect on retinal structures had a negative impact on the morpho-functional status of diabetic eyes. As highlighted by the present review, a big debate is nowadays present in the literature regarding the comparison between anti-VEGF and laser approaches, and the combined use of both treatments. Most of the current data do not provide enough level of evidence to draw definite conclusions. Through the many studies conducted, the DRCR network offered a strong contribution in the clinical and therapeutic management of DR, highlighting the importance of promptly and frequently repeated intravitreal injections. The overall feel is that laser approach might be avoided when a perfectly planned anti-VEGF therapeutic strategy can be adopted. However, we must bear in mind that the real-life situation is quite different from clinical trials settings. Probably, there is no absolute winning therapeutic choice for managing DR, but the choice of the treatments and of the follow-up timeline must be planned based on personalized strategies designed on patients’ characteristics. The clinical status, including glycemic control and presence of comorbidities, patient’s self-sufficiency, compliance with the frequency of follow-up visits and treatments represent key aspects ruling the overall clinical and ophthalmologic management of the DR patient. The future development of longer duration anti-VEGF treatments and of even more optimized molecules will have a remarkable impact on the feasibility and sustainability of DR patients care for the hospitals and the public health systems, and probably will modify the current indications to laser approaches. According to the EURETINA guidelines [Citation67], anti-VEGF molecules represent the treatment of choice for most of DR patients, because of the high efficacy and safety profiles, and the feasible management. Possible contra-indications regard those patients characterized by high cardiovascular risks, where other approaches, such as corticosteroids [Citation135–138], should be preferred. Laser approaches still maintain a role for diabetic eyes characterized by extremely severe form of DR and for those patients not guaranteeing adequate compliance to intravitreal treatments strategies.
Author contributions
FB and AA performed the revision of the current literature and wrote the manuscript.
Disclosure statement
No potential conflict of interest was reported by the author(s). Francesco Bandello is consultant for: Alcon (Fort Worth, Texas, USA), Alimera Sciences (Alpharetta, Georgia, USA), Allergan Inc (Irvine, California, USA), Farmila-Thea (Clermont-Ferrand, France), Bayer Shering-Pharma (Berlin, Germany), Bausch And Lomb (Rochester, New York, USA), Genentech (San Francisco, California, USA), Hoffmann-La-Roche (Basel, Switzerland), NovagaliPharma (Évry, France), Novartis (Basel, Switzerland), Sanofi-Aventis (Paris, France), Thrombogenics (Heverlee, Belgium), Zeiss (Dublin, USA). Alessandro Arrigo and Emanuela Aragona have no disclosures to declare.
Data availability statement
Data are available after a formal request to the corresponding author.
References
- Aiello LP, Wong JS. Role of vascular endothelial growth factor in diabetic vascular complications. Kidney Int Suppl. 2000;77:S113–S9.
- Antonetti DA, Klein R, Gardner TW. Diabetic retinopathy. N Engl J Med. 2012;366(13):1227–1239.
- Ferrara N, Houck K, Jakeman L, et al. Molecular and biological properties of the vascular endothelial growth factor family of proteins. Endocr Rev. 1992;13(1):18–32.
- Vitt UA, Hsu SY, Hsueh AJW. Evolution and classification of cystine knot-containing hormones and related extracellular signaling molecules. Mol Endocrinol. 2001;15(5):681–694.
- Ferrara N, Carver-Moore K, Chen H, et al. Heterozygous embryonic lethality induced by targeted inactivation of the VEGF gene. Nature. 1996;380(6573):439–442.
- Breier G. Functions of the VEGF/VEGF receptor system in the vascular system. Semin Thromb Hemost. 2000;26(5):553–559.
- Takahashi H, Shibuya M. The vascular endothelial growth factor (VEGF)/VEGF receptor system and its role under physiological and pathological conditions. Clin Sci. 2005;109(3):227–241.
- Robinson CJ, Stringer SE. The splice variants of vascular endothelial growth factor (VEGF) and their receptors. J Cell Sci. 2001;114(Pt 5):853–865.
- Olofsson B, Pajusola K, von Euler G, et al. Genomic organization of the mouse and human genes for vascular endothelial growth factor B (VEGF-B) and characterization of a second splice isoform. J Biol Chem. 1996;271(32):19310–19317.
- Wang ZG, Puri TS, Quigg RJ. Characterization of novel VEGF (vascular endothelial growth factor)-C splicing isoforms from mouse. Biochem J. 2010;428(3):347–354.
- Baldwin ME, Roufail S, Halford MM, et al. Multiple forms of mouse vascular endothelial growth factor-D are generated by RNA splicing and proteolysis. J Biol Chem. 2001;276(47):44307–44314.
- Ferrara N. Vascular endothelial growth factor: basic science and clinical progress. Endocr Rev. 2004;25(4):581–611.
- Ogawa S, Oku A, Sawano A, et al. A novel type of vascular endothelial growth factor, VEGF-E (NZ-7 VEGF), preferentially utilizes KDR/flk-1 receptor and carries a potent mitotic activity without heparin-binding domain. J Biol Chem. 1998;273(47):31273–31282.
- Yamazaki Y, Matsunaga Y, Tokunaga Y, et al. Snake venom vascular endothelial growth factors (VEGF-Fs) exclusively vary their structures and functions among species. J Biol Chem. 2009;284(15):9885–9891.
- Yancopoulos GD, Davis S, Gale NW, et al. Vascular-specific growth factors and blood vessel formation. Nature. 2000;407(6801):242–248.
- Jussila L, Alitalo K. Vascular growth factors and lymphangiogenesis. Physiol Rev. 2002;82(3):673–700.
- Dvorak HF, Brown LF, Detmar M, et al. Vascular permeability factor/vascular endothelial growth factor, microvascular hyperpermeability, and angiogenesis. Am J Pathol. 1995;146(5):1029–1039.
- Sondell M, Sundler F, Kanje M. Vascular endothelial growth factor is a neurotrophic factor which stimulates axonal outgrowth through the flk-1 receptor. Eur J Neurosci. 2000;12(12):4243–4254.
- Storkebaum E, Lambrechts D, Carmeliet P. VEGF: once regarded as a specific angiogenic factor, now implicated in neuroprotection. Bioessays. 2004;26(9):943–954.
- Li X, Lee C, Tang Z, et al. VEGF-B: a survival, or an angiogenic factor? Cell Adh Migr. 2009;3(4):322–327.
- Jeltsch M, Kaipainen A, Joukov V, et al. Hyperplasia of lymphatic vessels in VEGF-C transgenic mice. Science. 1997;276(5317):1423–1425.
- Cao Y, Linden P, Farnebo J, et al. Vascular endothelial growth factor C induces angiogenesis in vivo. Proc Natl Acad Sci USA. 1998;95(24):14389–14394.
- Marconcini L, Marchio S, Morbidelli L, et al. c-fos-induced growth factor/vascular endothelial growth factor D induces angiogenesis in vivo and in vitro. Proc Natl Acad Sci U S A. 1999;96(17):9671–9676.
- Stacker SA, Caesar C, Baldwin ME, et al. VEGF-D promotes the metastatic spread of tumor cells via the lymphatics. Nat Med. 2001;7(2):186–191.
- Campochiaro PA, Aiello LP, Rosenfeld PJ. Anti-vascular endothelial growth factor agents in the treatment of retinal disease: from bench to bedside. Ophthalmology. 2016;123(10S):S78–S88.
- Cabral T, Mello LGM, Lima LH, et al. Retinal and choroidal angiogenesis: a review of new targets. Int J Retina Vitreous. 2017;3:31.
- Penn JS, Madan A, Caldwell RB, et al. Vascular endothelial growth factor in eye disease. Prog Retin Eye Res. 2008;27(4):331–371.
- Wang GL, Semenza GL. Purification and characterization of hypoxia-inducible factor 1. J Biol Chem. 1995;270(3):1230–1237.
- Grant MB, Mames RN, Fitzgerald C, et al. Insulin-like growth factor I as an angiogenic agent. In vivo and in vitro studies. Ann NY Acad Sci. 1993;692:230–242.
- Miller JW, Adamis AP, Aiello LP. Vascular endothelial growth factor in ocular neovascularization and proliferative diabetic retinopathy. Diabetes Metab Rev. 1997;13(1):37–50.
- Stone J, Itin A, Alon T, et al. Development of retinal vasculature is mediated by hypoxia-induced vascular endothelial growth factor (VEGF) expression by neuroglia. J Neurosci. 1995;15(7 Pt 1):4738–4747.
- Reichenbach A, Bringmann A. Glia of the human retina. Glia. 2020;68(4):768–796.
- Ida H, Tobe T, Nambu H, et al. RPE cells modulate subretinal neovascularization, but do not cause regression in mice with sustained expression of VEGF. Invest Ophthalmol Vis Sci. 2003;44(12):5430–5437.
- Caldwell RB, Bartoli M, Behzadian MA, et al. Vascular endothelial growth factor and diabetic retinopathy: role of oxidative stress. Curr Drug Targets. 2005;6(4):511–524.
- Shankar A, Mitchell P, Rochtchina E, et al. Association between circulating white blood cell count and long-term incidence of age-related macular degeneration: the blue mountains eye study. Am J Epidemiol. 2006;165(4):375–382.
- Watanabe D, Suzuma K, Matsui S, et al. Erythropoietin as a retinal angiogenic factor in proliferative diabetic retinopathy. N Engl J Med. 2005;353(8):782–792.
- Betsholtz C. Insight into the physiological functions of PDGF through genetic studies in mice. Cytokine Growth Factor Rev. 2004;15(4):215–228.
- Puddu A, Sanguineti R, Durante A, et al. Vascular endothelial growth factor-C secretion is increased by advanced glycation end-products: possible implication in ocular neovascularization. Mol Vis. 2012;18:2509–2517.
- Zhao B, Ma A, Cai J, et al. VEGF-A regulates the expression of VEGF-C in human retinal pigment epithelial cells. Br J Ophthalmol. 2006;90(8):1052–1059.
- Zhang SX, Wang JJ, Gao G, et al. Pigment epithelium-derived factor downregulates vascular endothelial growth factor (VEGF) expression and inhibits VEGF-VEGF receptor 2 binding in diabetic retinopathy. J Mol Endocrinol. 2006;37(1):1–12.
- Kociok N, Joussen AM. Varied expression of functionally important genes of RPE and choroid in the macula and in the periphery of normal human eyes. Graefes Arch Clin Exp Ophthalmol. 2007;245(1):101–113.
- Leasher JL, Bourne RR, Flaxman SR, on behalf of the Vision Loss Expert Group of the Global Burden of Disease Study, et al. Vision loss expert group of the global burden of disease study. Global estimates on the number of people blind or visually impaired by diabetic retinopathy: a meta-analysis from 1990 to 2010. Diabetes Care. 2016;39(9):1643–1649.
- Havens SJ, Gulati V. Neovascular glaucoma. Dev Ophthalmol. 2016;55:196–204.
- Stitt AW, Curtis TM, Chen M, et al. The progress in understanding and treatment of diabetic retinopathy. Prog Retin Eye Res. 2016;51:156–186.
- Antonetti DA, Barber AJ, Hollinger LA, et al. Vascular endothelial growth factor induces rapid phosphorylation of tight junction proteins occludin and zonula occluden 1. A potential mechanism for vascular permeability in diabetic retinopathy and tumors. J Biol Chem. 1999;274(33):23463–23467.
- Behzadian MA, Windsor LJ, Ghaly N, et al. VEGF-induced paracellular permeability in cultured endothelial cells involves urokinase and its receptor. Faseb J. 2003;17(6):752–754.
- Gavard J, Gutkind JS. VEGF controls endothelial-cell permeability by promoting the beta-arrestin-dependent endocytosis of VE-cadherin. Nat Cell Biol. 2006;8(11):1223–1234.
- Feng Y, Venema VJ, Venema RC, et al. VEGF-induced permeability increase is mediated by caveolae. Invest Ophthalmol Vis Sci. 1999;40(1):157–167.
- Hofman P, Blaauwgeers HG, Tolentino MJ, et al. VEGF-A induced hyperpermeability of blood-retinal barrier endothelium in vivo is predominantly associated with pinocytotic vesicular transport and not with formation of fenestrations. Vascular endothelial growth factor-A. Curr Eye Res. 2000;21(2):637–645.
- Daruich A, Matet A, Moulin A, et al. Mechanisms of macular edema: beyond the surface. Prog Retin Eye Res. 2018;63:20–68.
- Mesquita J, Castro-de-Sousa JP, Vaz-Pereira S, et al. Vascular endothelial growth factors and placenta growth factor in retinal vasculopathies: current research and future perspectives. Cytokine Growth Factor Rev. 2018;39:102–115.
- Singh RP, Elman MJ, Singh SK, et al. Advances in the treatment of diabetic retinopathy. J Diabetes Complications. 2019;33(12):107417.
- Witmer AN, Vrensen GF, Van Noorden CJ, et al. Vascular endothelial growth factors and angiogenesis in eye disease. Prog Retin Eye Res. 2003;22(1):1–29.
- Shibuya M. Differential roles of vascular endothelial growth factor receptor-1 and receptor-2 in angiogenesis. J Biochem Mol Biol. 2006;39(5):469–478.
- Takagi H, Koyama S, Seike H, et al. Potential role of the angiopoietin/tie2 system in ischemia-induced retinal neovascularization. Invest Ophthalmol Vis Sci. 2003;44(1):393–402.
- Oh H, Takagi H, Otani A, et al. Selective induction of neuropilin-1 by vascular endothelial growth factor (VEGF): a mechanism contributing to VEGF-induced angiogenesis. Proc Natl Acad Sci USA. 2002;99(1):383–388.
- Chaturvedi N, Sjolie AK, Stephenson JM, et al. Effect of lisinopril on progression of retinopathy in normotensive people with type 1 diabetes. The EUCLID study group. EURODIAB controlled trial of lisinopril in Insulin-Dependent diabetes mellitus. Lancet. 1998;351(9095):28–31.
- Rennel E, Guan WE, Schüler H, et al. S. The endogenous anti-angiogenic VEGF isoform, VEGF165b inhibits human tumour growth in mice. Br J Cancer. 2008;98(7):1250–1257.
- Yang JC, Haworth L, Sherry RM, et al. A randomized trial of bevacizumab, an anti-vascular endothelial growth factor antibody, for metastatic renal cancer. N Engl J Med. 2003;349(5):427–434.
- Rosenfeld PJ, Moshfeghi AA, Puliafito CA. Optical coherence tomography findings after an intravitreal injection of bevacizumab (avastin) for neovascular age-related macular degeneration. Ophthalmic Surg Lasers Imaging. 2005;36(4):331–335.
- Kreisl TN, Kim L, Moore K, et al. Phase II trial of single-agent bevacizumab followed by bevacizumab plus irinotecan at tumor progression in recurrent glioblastoma. J Clin Oncol. 2009;27(5):740–745.
- Spasic M, Chow F, Tu C, et al. Molecular characteristics and pathways of avastin for the treatment of glioblastoma multiforme. Neurosurg Clin N Am. 2012;23(3):417–427.
- Haritoglou C, Kook D, Neubauer A, et al. Intravitreal bevacizumab (avastin) therapy for persistent diffuse diabetic macular edema. Retina. 2006;26(9):999–1005.
- Stefanini FR, Arevalo JF, Maia M. Bevacizumab for the management of diabetic macular edema. World J Diabetes. 2013;4(2):19–26.
- Choovuthayakorn J, Tantraworasin A, Phinyo P, et al. Factors associated with 1-year visual response following intravitreal bevacizumab treatment for diabetic macular edema: a retrospective single center study. Int J Retina Vitreous. 2021;7(1):17.
- Scott IU, Edwards AR, Beck RW, Diabetic Retinopathy Clinical Research Network, et al. A phase II randomized clinical trial of intravitreal bevacizumab for diabetic macular edema. Ophthalmology. 2007;114(10):1860–1867.
- Michaelides M, Kaines A, Hamilton RD, et al. A prospective randomized trial of intravitreal bevacizumab or laser therapy in the management of diabetic macular edema (BOLT study) 12-month data: report 2. Ophthalmology. 2010;117(6):1078–1086.e2.
- Rajendram R, Fraser-Bell S, Kaines A, et al. A 2-year prospective randomized controlled trial of intravitreal bevacizumab or laser therapy (BOLT) in the management of diabetic macular edema: 24-month data: report 3. Arch Ophthalmol. 2012;130(8):972–979.
- Schmidt-Erfurth U, Garcia-Arumi J, Bandello F, et al. A. Guidelines for the management of diabetic macular edema by the european society of retina specialists (EURETINA). Ophthalmologica. 2017;237(4):185–222.
- Lee JH, Canny MD, De Erkenez A, et al. A therapeutic aptamer inhibits angiogenesis by specifically targeting the heparin binding domain of VEGF165. Proc Natl Acad Sci USA. 2005;102(52):18902–18907.
- Gragoudas ES, Adamis AP, Cunningham ET, Jr, et al. VEGF inhibition study in ocular neovascularization clinical trial group. Pegaptanib for neovascular age-related macular degeneration. N Engl J Med. 2004;351(27):2805–2816.
- Ferrara N, Damico L, Shams N, et al. Development of ranibizumab, an anti-vascular endothelial growth factor antigen binding fragment, as therapy for neovascular age-related macular degeneration. Retina. 2006;26(8):859–870.
- Lowe J, Araujo J, Yang J, et al. Ranibizumab inhibits multiple forms of biologically active vascular endothelial growth factor in vitro and in vivo. Exp Eye Res. 2007;85(4):425–430.
- Vaidyanathan U, Moshirfar M. Ranibizumab. In: StatPearls [Internet]. Treasure Island (FL): StatPearls Publishing; 2021.
- Platania CB, Di Paola L, Leggio GM, et al. Molecular features of interaction between VEGFA and anti-angiogenic drugs used in retinal diseases: a computational approach. Front Pharmacol. 2015;6:248.
- Yang J, Wang X, Fuh G, et al. Comparison of binding characteristics and in vitro activities of three inhibitors of vascular endothelial growth factor A. Mol Pharm. 2014;11(10):3421–3430.
- Nguyen QD, Shah SM, Khwaja AA, et al. READ-2 study group. Two-year outcomes of the ranibizumab for edema of the mAcula in diabetes (READ-2) study. Ophthalmology. 2010;117(11):2146–2151.
- Massin P, Bandello F, Garweg JG, et al. Safety and efficacy of ranibizumab in diabetic macular edema (RESOLVE study): a 12-month, randomized, controlled, double-masked, multicenter phase II study. Diabetes Care. 2010;33(11):2399–2405.
- Mitchell P, Bandello F, Schmidt-Erfurth U, et al. Weichselberger A; RESTORE study group. The RESTORE study: ranibizumab monotherapy or combined with laser versus laser monotherapy for diabetic macular edema. Ophthalmology. 2011;118(4):615–625.
- Nguyen QD, Brown DM, Marcus DM, RISE and RIDE Research Group, et al. Ranibizumab for diabetic macular edema: results from 2 phase III randomized trials: RISE and RIDE. Ophthalmology. 2012;119(4):789–801.
- Comyn O, Sivaprasad S, Peto T, et al. A randomized trial to assess functional and structural effects of ranibizumab versus laser in diabetic macular edema (the LUCIDATE study). Am J Ophthalmol. 2014;157(5):960–970.
- Ishibashi T, Li X, Koh A, REVEAL Study Group, et al. The REVEAL study: ranibizumab monotherapy or combined with laser versus laser monotherapy in Asian patients with diabetic macular edema. Ophthalmology. 2015;122(7):1402–1415.
- Pearce I, Banerjee S, Burton BJ, RELIGHT Study Group, et al. Ranibizumab 0.5 mg for diabetic macular edema with bimonthly monitoring after a phase of initial treatment: 18-Month, multicenter, phase IIIB RELIGHT study. Ophthalmology. 2015;122(9):1811–1819.
- Prünte C, Fajnkuchen F, Mahmood S, RETAIN Study Group, et al. Ranibizumab 0.5 mg treat-and-extend regimen for diabetic macular oedema: the RETAIN study. Br J Ophthalmol. 2016;100(6):787–795.
- Sepah YJ, Sadiq MA, Boyer D, READ-3 Study Group, et al. Twenty-four-Month outcomes of the ranibizumab for edema of the macula in Diabetes – Protocol 3 with high dose (READ-3) study. Ophthalmology. 2016;123(12):2581–2587.
- Gross JG, Glassman AR, Jampol LM, Writing Committee for the Diabetic Retinopathy Clinical Research Network, et al. Panretinal photocoagulation vs intravitreous ranibizumab for proliferative diabetic retinopathy: a randomized clinical trial. JAMA. 2015;314(20):2137–2146.
- Gross JG, Glassman AR, Liu D, Diabetic Retinopathy Clinical Research Network, et al. Diabetic retinopathy clinical research network. Five-year outcomes of panretinal photocoagulation vs. intravitreous ranibizumab for proliferative diabetic retinopathy: a randomized clinical trial. JAMA Ophthalmol. 2018;136(10):1138–1148.
- Wells JA, Glassman AR, Ayala AR, Diabetic Retinopathy Clinical Research Network, et al. Aflibercept, bevacizumab, or ranibizumab for diabetic macular edema. N Engl J Med. 2015;372(13):1193–1203.
- Singer MA, Miller DM, Gross JG, et al. Visual acuity outcomes in diabetic macular edema with fluocinolone acetonide 0.2 μg/day versus ranibizumab plus deferred laser (DRCR protocol I). Ophthalmic Surg Lasers Imaging Retina. 2018;49(9):698–706.
- Payne JF, Wykoff CC, Clark WL, TREX-DME Study Group, et al. Randomized trial of treat and extend ranibizumab with and without navigated laser for diabetic macular edema: TREX-DME 1 year outcomes. Ophthalmology. 2017;124(1):74–81.
- Payne JF, Wykoff CC, Clark WL, TREX-DME Study Group, et al. Randomized trial of treat and extend ranibizumab with and without navigated laser versus monthly dosing for diabetic macular edema: TREX-DME 2-Year outcomes. Am J Ophthalmol. 2019;202:91–99.
- Payne JF, Wykoff CC, Clark WL, TREX-DME Study Group, et al. Long-term outcomes of treat-and-extend ranibizumab with and without navigated laser for diabetic macular oedema: TREX-DME 3-year results. Br J Ophthalmol. 2021;105(2):253–257.
- Fechter C, Frazier H, Marcus WB, et al. Ranibizumab 0.3 mg for persistent diabetic macular edema after recent, frequent, and chronic bevacizumab: the ROTATE trial. Ophthalmic Surg Lasers Imag Retina. 2016;47(11):1–18.
- Lang GE, Liakopoulos S, Vögeler J, et al. The RELATION study: efficacy and safety of ranibizumab combined with laser photocoagulation treatment versus laser monotherapy in NPDR and PDR patients with diabetic macular oedema. Acta Ophthalmol. 2018;96(3):e377–e385.
- Li X, Dai H, Li X, REFINE study group, et al. Efficacy and safety of ranibizumab 0.5 mg in chinese patients with visual impairment due to diabetic macular edema: results from the 12-month REFINE study. Graefes Arch Clin Exp Ophthalmol. 2019;257(3):529–541.
- Holash J, Davis S, Papadopoulos N, et al. VEGF-Trap: a VEGF blocker with potent antitumor effects. Proc Natl Acad Sci USA. 2002;99(17):11393–11398.
- Cheng YD, Yang H, Chen GQ, et al. Molecularly targeted drugs for metastatic colorectal cancer. Drug Des Devel Ther. 2013;7:1315–1322.
- Mansour AM, Al-Ghadban SI, Yunis MH, et al. Ziv-aflibercept in macular disease. Br J Ophthalmol. 2015;99(8):1055–1059.
- Korobelnik JF, Do DV, Schmidt-Erfurth U, et al. Intravitreal aflibercept for diabetic macular edema. Ophthalmology. 2014;121(11):2247–2254.
- Brown DM, Schmidt-Erfurth U, Do DV, et al. Intravitreal aflibercept for diabetic macular edema: 100-Week results from the VISTA and VIVID studies. Ophthalmology. 2015;122(10):2044–2052.
- Wykoff CC, Le RT, Khurana RN, ENDURANCE Study Group, et al. Outcomes with as-needed aflibercept and macular laser following the phase III VISTA DME trial: ENDURANCE 12-month extension study. Am J Ophthalmol. 2017;173:56–63.
- Baker CW, Glassman AR, Beaulieu WT, DRCR Retina Network, et al. Effect of initial management with aflibercept vs laser photocoagulation vs observation on vision loss among patients with diabetic macular edema involving the center of the macula and good visual acuity: a randomized clinical trial. JAMA. 2019;321(19):1880–1894.
- Maturi RK, Glassman AR, Josic K, DRCR Retina Network, et al. Effect of intravitreous anti-Vascular endothelial growth factor vs sham treatment for prevention of Vision-Threatening complications of diabetic retinopathy: the protocol W randomized clinical trial. JAMA Ophthalmol. 2021;139(7):701–712.
- Zhang M, Zhang J, Yan M, et al. Recombinant anti-vascular endothelial growth factor fusion protein efficiently suppresses choridal neovasularization in monkeys. Mol Vis. 2008;14:37–49.
- Suto K, Yamazaki Y, Morita T, et al. Crystal structures of novel vascular endothelial growth factors (VEGF) from snake venoms: insight into selective VEGF binding to kinase insert domain-containing receptor but not to fms-like tyrosine kinase-1. J Biol Chem. 2005;280(3):2126–2131.
- Liu K, Wang H, He W, et al. Intravitreal conbercept for diabetic macular oedema: 2-year results from a randomised controlled trial and open-label extension study. Br J Ophthalmol. 2021;:2020–318690.
- Li F, Zhang L, Wang Y, et al. One-year outcome of conbercept therapy for diabetic macular edema. Curr Eye Res. 2018;43(2):218–223.
- Cai S, Yang Q, Li X, et al. The efficacy and safety of aflibercept and conbercept in diabetic macular edema. Drug Des Devel Ther. 2018;12:3471–3483.
- Xu Y, Rong A, Bi Y, et al. Intravitreal conbercept injection with and without grid laser photocoagulation in the treatment of diffuse diabetic macular edema in real-life clinical practice. J Ophthalmol. 2016;2016:1–7.
- Wang H, Guo J, Tao S, et al. One-Year effectiveness study of intravitreously administered conbercept® monotherapy in diabetic macular degeneration: a systematic review and meta-analysis. Diabetes Ther. 2020;11(5):1103–1117.
- Yannuzzi NA, Freund KB. Brolucizumab: evidence to date in the treatment of neovascular age-related macular degeneration. Clin Ophthalmol. 2019;13:1323–1329.
- Sharma A, Kumar N, Kuppermann BD, et al. Brolucizimab-leading an era of structural revolution for long-term VEGF suppression. Eye. 2020;34(4):611–613.
- Nguyen QD, Das A, Do DV, et al. Brolucizumab: evolution through preclinical and clinical studies and the implications for the management of neovascular age-related macular degeneration. Ophthalmology. 2020;127(7):963–976.
- Tadayoni R, Sararols L, Weissgerber G, et al. Brolucizumab: a newly developed anti-VEGF molecule for the treatment of neovascular age-related macular degeneration. Ophthalmologica. 2021;244(2):93–101.
- Garweg JG. A randomized, Double-Masked, multicenter, phase III study assessing the efficacy and safety of brolucizumab versus aflibercept in patients with visual impairment due to diabetic macular edema (KITE). Klin Monbl Augenheilkd. 2020;237(4):450–453.
- Stumpp MT, Binz HK, Amstutz P. DARPins: a new generation of protein therapeutics. Drug Discov Today. 2008;13(15–16):695–701.
- Souied EH, Devin F, Mauget-Faÿsse M, MP0112 Study Group, et al. Treatment of exudative age-related macular degeneration with a designed ankyrin repeat protein that binds vascular endothelial growth factor: a phase I/II study. Am J Ophthalmol. 2014;158(4):724–732.e2.
- Rodrigues GA, Mason M, Christie LA, et al. Functional characterization of Abicipar-Pegol, an anti-VEGF DARPin therapeutic that potently inhibits angiogenesis and vascular permeability. Invest Ophthalmol Vis Sci. 2018;59(15):5836–5846.
- Nicolò M, Ferro Desideri L, Vagge A, et al. Faricimab: an investigational agent targeting the tie-2/angiopoietin pathway and VEGF-A for the treatment of retinal diseases. Expert Opin Investig Drugs. 2021;30(3):193–200.
- Sahni J, Patel SS, Dugel PU, et al. Simultaneous inhibition of angiopoietin-2 and vascular endothelial growth Factor-A with faricimab in diabetic macular edema: BOULEVARD phase 2 randomized trial. Ophthalmology. 2019;126(8):1155–1170.
- Striglia E, Caccioppo A, Castellino N, et al. Emerging drugs for the treatment of diabetic retinopathy. Expert Opin Emerg Drugs. 2020;25(3):261–271.
- Diabetic Retinopathy Clinical Research Network* Randomized clinical trial evaluating intravitreal ranibizumab or saline for vitreous hemorrhage from proliferative diabetic retinopathy. JAMA Ophthalmol. 2013;131(3):283–293.
- Sivaprasad S, Prevost AT, Vasconcelos JC, CLARITY Study Group, et al. Clinical efficacy of intravitreal aflibercept versus panretinal photocoagulation for best corrected visual acuity in patients with proliferative diabetic retinopathy at 52 weeks (CLARITY): a multicentre, single-blinded, randomised, controlled, phase 2b, non-inferiority trial. Lancet. 2017;389(10085):2193–2203.
- Figueira J, Fletcher E, Massin P, EVICR.net Study Group., et al. Ranibizumab plus panretinal photocoagulation versus panretinal photocoagulation alone for high-risk proliferative diabetic retinopathy (PROTEUS study). Ophthalmology. 2018;25(5):691–700.
- Korobelnik JF, Daien V, Faure C, et al. Real-world outcomes following 12 months of intravitreal aflibercept monotherapy in patients with diabetic macular edema in France: results from the APOLLON study. Graefes Arch Clin Exp Ophthalmol. 2020;258(3):521–528.
- Brown DM, Wykoff CC, Boyer D, et al. Evaluation of intravitreal aflibercept for the treatment of severe nonproliferative diabetic retinopathy: Results from the PANORAMA randomized clinical trial. JAMA Ophthalmol. 2021;:e212809.
- Alagorie AR, Velaga S, Nittala MG, et al. Effect of aflibercept on diabetic retinopathy severity and visual function in the RECOVERY study for proliferative diabetic retinopathy. Ophthalmol Retina. 2021;5(5):409–419.
- Martinez-Zapata MJ, Martí-Carvajal AJ, Solà I, et al. Anti-vascular endothelial growth factor for proliferative diabetic retinopathy. Cochrane Database Syst Rev. 2014;2014(11):CD008721.
- Simunovic MP, Maberley DA. Anti-vascular endothelial growth factor therapy for proliferative diabetic retinopathy: a systematic review and meta-analysis. Retina. 2015;35(10):1931–1942.
- Bressler SB, Beaulieu WT, Glassman AR, et al. Diabetic retinopathy clinical research network. Factors associated with worsening proliferative diabetic retinopathy in eyes treated with panretinal photocoagulation or ranibizumab. Ophthalmology. 2017;124(4):431–439.
- Gao S, Lin Z, Shen X. Anti-Vascular endothelial growth factor therapy as an alternative or adjunct to pan-retinal photocoagulation in treating proliferative diabetic retinopathy: meta-analysis of randomized trials. Front Pharmacol. 2020;11:849.
- Fallico M, Maugeri A, Lotery A, et al. Intravitreal anti-vascular endothelial growth factors, panretinal photocoagulation and combined treatment for proliferative diabetic retinopathy: a systematic review and network meta-analysis. Acta Ophthalmol. 2021;99(6):e795–e805.
- Maguire MG, Liu D, Glassman AR, DRCR Retina Network, et al. Visual field changes over 5 years in patients treated with panretinal photocoagulation or ranibizumab for proliferative diabetic retinopathy. JAMA Ophthalmol. 2020;138(3):285–293.
- Sun JK, Glassman AR, Beaulieu WT, et al. Diabetic retinopathy clinical research network. Rationale and application of the protocol S anti-vascular endothelial growth factor algorithm for proliferative diabetic retinopathy. Ophthalmology. 2019;126(1):87–95.
- Tan Y, Fukutomi A, Sun MT, et al. Anti-VEGF crunch syndrome in proliferative diabetic retinopathy: a review. Surv Ophthalmol. 2021;66(6):926–932.
- Boyer DS, Yoon YH, Belfort R, Jr, Ozurdex MEAD Study Group., et al. Three-year, randomized, sham-controlled trial of dexamethasone intravitreal implant in patients with diabetic macular edema. Ophthalmology. 2014;121(10):1904–1914.
- Campochiaro PA, Brown DM, Pearson A, et al. Kane FE; FAME study group. Long-term benefit of sustained-delivery fluocinolone acetonide vitreous inserts for diabetic macular edema. Ophthalmology. 2011;118(4):626–635.e2.
- Campochiaro PA, Brown DM, Pearson A, FAME Study Group, et al. Sustained delivery fluocinolone acetonide vitreous inserts provide benefit for at least 3 years in patients with diabetic macular edema. Ophthalmology. 2012;119(10):2125–2132.