Abstract
Propose
Neoadjuvant chemotherapy has been widely used in locally advanced and inflammatory breast cancer. Generally, complete pathological response after neoadjuvant chemotherapy treatment predicts survival. Studies have shown that patient-derived organoids can be used in cancer research and drug development. Therefore, we aimed to generate a living organoid biobank from biopsy samples to predict the response of patients to neoadjuvant chemotherapy.
Method
We generated a living organoid biobank from locally advanced breast cancer patients receiving neoadjuvant chemotherapy. When the patient received neoadjuvant chemotherapy, the organoids were treated with similar drugs, thereby simulating the situation of the patient receiving treatment.
Result
We successfully constructed organoids from breast cancer biopsies, demonstrating that organoids can be generated from a small sample of tissue. The phenotype of breast cancer organoid often agreed with the original breast cancer according to the blinded histopathological analysis of H&E stain tissue and organoid sections. In addition, our data confirm that the patient’s response to chemotherapy closely matches the organoids’ response to drugs.
Conclusion
Our data indicate that patient-derived organoids can be used to predict the clinical response of breast cancer patients to neoadjuvant chemotherapy in vitro and to screen drugs that have different effects on different patients.
Complete pathological response (pCR) after adjuvant chemotherapy can predict, survival, therefore, predicting patient response to neoadjuvant chemotherapy is critical.
Patient-derived organoids (PDOs) matched the original tumour in terms of histopathology, hormone receptor levels and HER2 receptor status.
Patient-derived organoids can predict the responsiveness of patient to neoadjuvant chemotherapy.
Key message
1. Introduction
Breast cancer (BC) is the most diagnosed cancer globally and has the highest death rate among cancers affecting females [Citation1,Citation2]. BC can be divided into three subtypes based on the hormone receptor status and the expression of human epidermal growth factor receptor 2 (HER2) and proliferation marker Ki67 as follows: hormone receptor-positive luminal BC, primarily treated with endocrine therapy; HER2-positive BC, mainly treated with adjuvant chemotherapy combined with anti-HER2 antibodies such as trastuzumab and patolizumab; and oestrogen receptor (ER), progesterone receptor (PR), and human epidermal growth factor receptor 2 (HER2) expression-negative (triple-negative; TNBC) BC, mainly treated with adjuvant chemotherapy [Citation3–6]. TNBC does not respond well to targeted therapy and endocrine therapy [Citation7]. Surgery and chemotherapy are the main treatments for most TNBC patients.
Compared with traditional postoperative adjuvant therapy, systemic adjuvant therapy before surgery, namely, neoadjuvant therapy, is a new treatment model. The initial purpose of neoadjuvant chemotherapy (NAC) for operable BC is to reduce the tumour stage, achieve local control and increase the conservative operation rate. Another significant advantage of using NAC is the early identification of unresponsive tumours, predicting the sensitivity of new drugs in the body and promoting the development of new medicines and clinical trials [Citation8]. According to reports, after NAC, the overall survival rate of BC patients who achieve a complete pathological response (pCR) is much higher than that of patients with residual disease. Whether patients achieve pCR after neoadjuvant chemotherapy is closely related to the survival rate [Citation9]; however, the drug resistance mechanism of BC patients with residual disease after neoadjuvant chemotherapy is not fully understood. In recent decades, the survival rate of BC patients has increased significantly. In addition to early diagnosis, surgical treatment, radiotherapy, and chemotherapy, individualized and precise comprehensive treatment has led to high cure and survival rates [Citation10]. Despite the differences between different species and differences between in vivo and in vitro environments, research into the biological behaviour of tumours remains at the level of traditional tumour cell lines and animal models, which complicates the application of high-throughput genomics in clinical practice and research.
Over the past few decades, our understanding of the origin of cancer has dramatically increased. Although significant progress has been made in treating certain types, cancer remains a significant health problem worldwide [Citation11]. Screening examinations and early detection methods can reduce the number of deaths from cancer. In addition, new and more targeted individualized therapies provide patients with more effective treatment opportunities. However, one of the main obstacles to developing new treatment options is the challenge of translating scientific knowledge from experimental platforms into clinical practice. This complication mainly arises to the failure of many cancer models to recapitulate patient tumours [Citation12]; therefore, many drugs that perform well in cancer models eventually fail in clinical trials [Citation13]. Although animal-derived cancer models provide many opportunities for basic cancer research, developing these models is time-consuming. Additionally, studies have shown that these models usually do not faithfully reflect the course of the disease in humans [Citation14]. For example, the complexity and genetic heterogeneity of human cancer histology are generally not reflected in genetically modified mouse models [Citation15].
Recently developed 3 D cell culture technology has generated more novel and physiologically meaningful human healthy tissue and cancer models. Tissue-derived human stem cells can be used to efficiently grow tissue-derived organ-type structures, referred to as organoids, in a 3 D culture matrix. In 2009, Sato et al. [Citation13] demonstrated that 3 D epithelial organoids can be established from a single G protein-coupled receptor 5+ (LGR5) intestinal stem cell rich in leucine repeats [Citation16–18]. Using this culture method, a variety of organoids were cultivated, including colon [Citation19,Citation20], liver, pancreas [Citation21], prostate [Citation22,Citation23], stomach [Citation24], fallopian tube [Citation25], taste bud [Citation17], salivary gland [Citation26], oesophagus [Citation27], lung [Citation28], and endometrium [Citation29] and breast organoids [Citation30]. Studies have shown that organoids can be cultured for a long time, undergo freezing and genetic modification and remain genetically and phenotypically stable. This characteristic of organoids allows them to be widely applied in cancer research [Citation31].
To date, many organoids have been developed from patient-derived tumours and matched healthy tissues, and an organoid library has been constructed. These organoids can be used to predict whether individual patients will respond to a drug [Citation30,Citation32,Citation33].
2. Materials and methods
2.1. Experimental design
The biopsies obtained from patients hospitalized at the First Affiliated Hospital of Chongqing Medical University who met the criteria of neoadjuvant chemotherapy after ultrasound or mammography and pathological examinations were used to establish a biobank of patient-derived organoids (PDOs).
2.2. The patient’s neoadjuvant chemotherapy regimen is shown in
The histopathology and morphology of PDOs were characterized in detail and compared to their parental tumours, demonstrating high similarities between the two. It mainly reaches whether PDO, as a “carcinoid model”, is consistent with the patient’s clinical response in terms of chemotherapy response or how much consistency is it? The researchers were blinded to the patients’ responses to avoid biases.
Table 1. Neoadjuvant chemotherapy regimen.
2.3. Establishment and culture of PDOs from BC samples
BC biopsy samples were obtained with informed consent from patients suspected of having BC who required neoadjuvant treatment from Jun 2020 to December 2020. A total of 17 biopsy samples were collected (). Given that most patients with triple-negative breast cancer or HER2-positive breast cancer and patients with axillary lymph node metastasis usually receive neoadjuvant treatment before surgical resection, the PDO biobank is a valuable resource. It is worth noting that at the time of writing this manuscript, 15 patients had undergone surgery, and tumour pathology assessments were available. One patient was lost to follow-up. One patient was treated with an NP (gemcitabine combined with cisplatin) regimen due to bone and liver metastasis and did not receive surgery.
Figure 1. Study design and construction of BC organoids. (A) Flow diagram of the study, including clinical neoadjuvant chemotherapy before surgery and drug tests on organoids. (B) The puncture tissue of the patient obtained by the core puncture under the guidance of colour Doppler ultrasound is 1–2 cm strip shape. (C) Neoadjuvant chemotherapy regimen of patients with different kind of subtype. (D) Successful cultivation of biopsy samples of different subtypes.
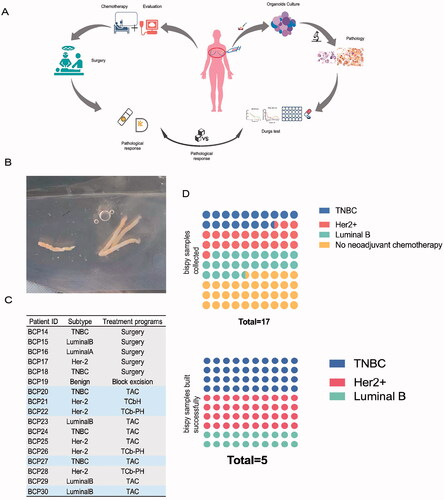
The collected biopsy specimens were placed in a 15 ml centrifuge tube containing 10 ml AdDF++ (Thermo Fisher Scientific), transported to the laboratory in an icebox for tumour cell isolation and culture, aspirated in a 15 ml centrifuge tube, transferred to an empty dish and weighed (x). The sample was then transferred into a small dish containing 10 ml AdDF++, and the weight of the empty dish (y) was used to determine the weight of the tumour (x-y=___).
The tumour was cut into small pieces with a diameter of 1–3 mm. The tumour block was divided into three parts. Two parts were placed into liquid nitrogen immediately after harvest for subsequent whole-exome sequencing and transcriptome sequencing. The other parts were used for tumour organoid construction. A Pasteur pipette was used to transfer the small piece of tissue into a 15 ml centrifuge tube containing 10 ml AdDF++; the tube was then centrifuged (300 g, 5 min) and the fragments were digested in digestion medium (advanced DMEM/F12 with 1 M Hepes (Invitrogen) and 1X GlutaMax (Invitrogen), Pen/Strep (Invitrogen), 1 mg/ml collagenase (Sigma) and 5 mM RHOK inhibitor ly27632 (Sigma-Aldrich, Y0503) at 37 °C with shaking for 60 or 90 min. Samples derived from resected tumours were filtered through a 70 μm cell strainer and centrifuged at 300 g for 5 min. Dissociated cells were collected in Advanced DMEM/F12 (Gibco), pelleted (1200 rpm, 5 min), resuspended in 40 μl Cultrex Reduced Growth Factor Basement Membrane Extract (BME), Type 2 (R&D Systems), and seeded into Matrigel in a well of a pre-warmed 24-well flat-bottom cell culture plate (Corning). Then, the cells were incubated for 20 min in a 37 °C and 5% CO2 cell incubator to solidify the Matrigel and were subsequently overlayed with 400 μl of complete human organ culture medium. The complete medium was refreshed every four days. PDOs were observed and photographed as required.
Organoids were typically passaged every one to two weeks. Passaging of PDOs was performed using TrypLe (Gibco). The PDO supernatant was aspirated, PBS was added to wash the PDO, and 1× TrypLe (including 1× Y27) was used to mechanically harvest the PDO from the Matrigel (pipetting) followed by incubation at 37 °C for 5–10 min. Then, the PDO was dissociated into smaller cell clusters by applying mechanical force (pipetting), precipitated (300 g, 5 min) with AdF+++10% FBS and resuspended in BME Matrigel according to the cell growth state to adjust the passaging ratio.
2.4. Human PDO culture medium
Human PDOs were cultured in Advanced DMEM/F12 medium containing HEPES, penicillin/streptomycin, Glutamax, B27, R-spondin 1, neuregulin 1, FGF7, FGF10, Noggin, n-acetylcysteine, nicotinamide, primocin, Y-27632, a selective inhibitor of TGF-βRI, ALK4, ALK7, p38 inhibitor and EGF. Detailed information regarding the cell culture medium is shown in .
Table 2. The main components of human PDO culture medium.
2.5. 3 D PDO drug assays
PDOs were harvested according to the above passage procedure and digested to an appropriate size as opposed to dissociating into a single-cell suspension. The cell pellet was resuspended in 500 μl of advanced DMED/F12, the cells were counted with a Countess automatic cell counter (Thermo Fisher Scientific), and appropriate cell dilutions were made in BME Matrigel. Twenty microliters of 50% BME Matrigel (diluted with organoid complete culture medium) containing 3500–5000 cells were seeded in standard 96-well cell culture plates (Corning), and the plates were incubated for 20 min in a 37 °C and 5% CO2 cell culture incubator to solidify the Matrigel. Then, the Matrigel was covered with 50 μl of complete human organoid medium. The complete media was replaced once after two days.
Two days after inoculation, the medium was removed and replaced with 50 μl of whole human organoid medium containing the drug. A total of three drugs were used in this experiment, docetaxel, carboplatin and epirubicin. The concentration gradient of the different drugs was set based on pre-experimental results from breast cancer cell lines. Both HER2-positive and HER2-negative BC patients receive preoperative adjuvant treatment with docetaxel. In the organoid drug sensitivity test, the concentration gradient of docetaxel as a single agent was 0 µM, 0.001 µM, 0.01 µM, 0.1 µM, 1 µM and 10 µM. The concentration of docetaxel used when the drug was combined with carboplatin or anthracycline was 0.1 µM. The concentration gradient of carboplatin used for HER2-positive organoids was as follows: 0 µM, 0.001 µM, 0.01 µM, 0.1 µM, 1 µM, 2 µM, 4 µM, 8 µM, 16 µM and 32 µM. The concentration gradient of epirubicin used for HER2-negative organoids was: 0 µM, 0.001 µM, 0.01 µM, 0.1 µM, 1 µM, 2 µM, 4 µM, 8 µM, 16 µM and 32 µM. Based on the growth rate of various organoid cultures, the drug-containing medium was changed every three days to provide the cytokines/additives required for organoid growth. At the end of the treatment (after six days), the medium was removed and replaced with 100 μl of complete human organoid medium containing 50% CellTiter-Glo3D Cell Viability Assay (Promega). The contents were mixed vigorously for 5 min to induce cell lysis and then incubated at room temperature for an additional 25 min to stabilize the luminescent signal. Luminescence was recorded using a microplate reader for chemiluminescence. The drugs used in the in vitro experiments and their concentrations are shown in .®
2.6. Pathological evaluation system after NAC
Currently, commonly used pathological assessment systems include the Miller–Payne (MP) system, the RCB system, the Sataloff system and AJCC ypTNM staging. The Pathology Department of the First Affiliated Hospital of Chongqing Medical University mainly uses MP grading to evaluate NAC treatment effects
(MP grade) Evaluation of surgical specimens. The definition of tumour grade according to the MP system is listed in [Citation34].
Table 3. Miller–Payne (MP) system.
2.7. Recist 1.1
We evaluated the efficacy of adjuvant chemotherapy based on the postoperative pathological examination of the patient and simultaneously assessed the change in tumour burden in conjunction with the RECIST 1.1 system shown in .
Table 4. RECIST 1.1 system.
2.8. Complete pathological response (pCR)
Complete pathological response (pCR) can be used as a surrogate marker to predict the prognosis of BC patients after NAC. The FDA (Food and Drug Administration) recommends yPT0/Tis yPN0 (i.e. absence of invasive tumour cells in the breast and the nodes) as the definition of pCR. The definition of pCR is based on the absence of invasive tumours at the primary site, even if ductal carcinoma in situ is present [Citation35].
2.9. PDO/tissue histology, immunohistochemistry
To maintain the 3 D structure of PDOs and dissolve the Matrigel, we incubated PDOs with 1 ml of Cell Recovery Solution (Corning) at 4 °C for 30 min. Then, the PDOs were collected in cold PBS, precipitated (300 g, 4 min, 4 °C) and fixed in 4% paraformaldehyde (Sigma-Aldrich) overnight. After fixation, the PDOs were washed with PBS, precipitated (300 g, 4 min, 4 °C), then resuspended in 40 µl histogel (Thermo scientific). The histogel containing the PDO precipitate was transferred to a cylindrical grinding tool, and after the tissue had solidified, 100 µl 1% agarose was added to coat the histogel. The agarose pellet was dehydrated with ethanol (EtOH) and embedded in paraffin using standard histological protocols. These deparaffinized organoid sections were then analysed using HE and immunohistochemistry staining.
Before HE and immunohistochemistry staining, 4-mm thick sections were dewaxed in xylene, rehydrated in decreasing ethanol concentrations, and washed with water. For histological analysis, the sections were stained with haematoxylin and eosin. Immunohistochemistry was performed to measure Ki-67, ER, PR and Erb-B2 expression (). Cells were then incubated with EDTA (sodium citrate) antigen retrieval solution (Solabio C1032). Endogenous peroxidase blockers were used for blocking, and the cells were incubated in a humid chamber at room temperature for 10–15 min (generally 15 min). Then, the sections were incubated with primary antibodies diluted with antibody diluent (ZSGB-BIO ZLI-9028) overnight at 4 °C. The primary antibodies and their concentrations used for immunohistochemistry are listed in .
Table 5. The primary antibodies and their concentrations used for immunohistochemistry.
After rinsing with PBS, the sections were incubated with secondary antibodies (ZSGB-BIO SP-9002, SP-9001) for 30 min at room temperature. Then, sections were stained with haematoxylin. Finally, the areas were dehydrated and made transparent in increasing concentrations of ethanol and xylene and covered with 60% neutral gum (diluted with xylene). HE and immunohistochemistry images were obtained with an upright microscope.
HE stains and immunohistochemistry of core biopsy samples and samples after neoadjuvant chemotherapy were obtained from the pathology department of the First Affiliated Hospital of Chongqing Medical University. Experimenters were blind to the pathology results during the histological analysis of the organoid. Pathologists also do not have prior knowledge of the experimenter’s histological analysis results.
2.10. Statistics
Quantitative variables are presented as the mean ± SD. Data analyses were conducted using GraphPad Prism 8.4.0.
3. Results
3.1. Establishing BC organoids from biopsy samples
With the patient’s informed consent, we obtained hollow needle biopsy samples from patients expected to receive neoadjuvant chemotherapy, removed the normal tissue, and separated the breast cancer cells through physical and mechanical disruption and chemical and enzymatic digestion. The isolated cells were seeded into BME droplets in pre-treated 24-well plates and covered with 400 µl complete breast cancer organoid medium ().
From June 2020 to December 2020, a total of 17 BC biopsy specimens were collected. The patients included those who did not require modified radical mastectomy and NAC due to benign pathological findings, those who needed modified radical mastectomy but not NAC, and those who were needed to receive NAC. Approximately 1–2 cm of tissue is taken for biopsy depending on the size of the tumour (); according to the clinical guidelines of the Chinese Society of Clinical Oncology, breast cancer patients who meet one of the following five conditions can choose preoperative neoadjuvant drug treatment: large mass (>5 cm); axillary lymph node metastasis; HER-2 positive tumour; triple-negative tumour and tumour too large to preserve breast in a patient with a desire to undergo breast-preserving surgery.
Among the 17 patients whose biopsy tissues were collected, 1 patient had a benign tumour that did not require modified radical mastectomy. Five patients did not require NAC before surgery. Eleven patients required NAC, and five organoids were successfully constructed from their tissue biopsies (). According to the BC subtype analysis, among the five successfully created BC organoids, two originated from TNBC, two from HER2+ BC and one from hormone receptor-positive BC (). Hence, these five organoid lines were used for the clinical validation study.
3.2. Long-term passage of organoids in vitro and maintenance of tumour heterogeneity
Some organoids lose their viability during culture and passage; therefore, we consider organoids that can be successively passaged for more than three generations but not more than 20 generations as successful construction. In the early passages, the organoids mainly existed as single cells or small cell clusters, and cell clusters were more commonly observed in thin-walled cysts; in the late passages, the humanoid cell clusters were larger and more compact (). To explore whether the constructed organoids could be passaged stably for a long time, we observed the growth morphology of the organoids and detected the survival rate every two days using the CellTiter-Glo 3 D cell viability assay. As the culture time increased, organoid growth persisted (). However, compared with cell lines, PDOs grow more slowly in the early stages.
Figure 2. Long-term passage of organoids in vitro and maintain tumour heterogeneity. (A) Bright field shows the morphology of organoids with different passage times and the proliferation ability of organoids. Scale bar, 250 mm. (B) The proliferation curve of organoids tested by CellTiter-Glo 3D cell viability assay proves that the cultured organoids can survive and inherit for a long time. (C) The morphology of individual BCOs with different subtype. Scale bar, 100 mm. (D) Morphological characteristics of tumour tissue and organoids of patients who achieved pCR. The HE stain of puncture samples and surgery tissues of patients who reached pCR after neoadjuvant chemotherapy, Scale bar, 100 mm.
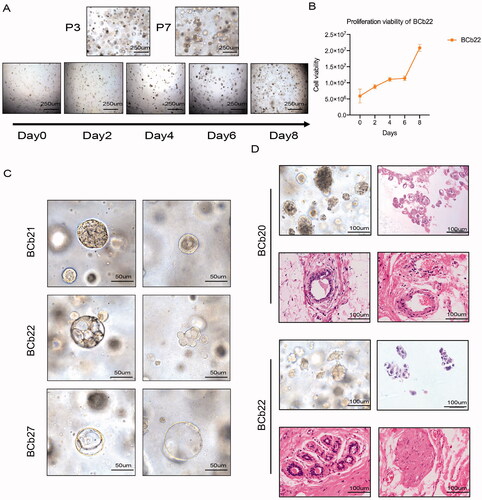
Consistent with the heterogeneity of patient tumours, we observed that the morphology of individual BCOs was different, although normal organoids always demonstrated a cystic phenotype. The organoids derived from BCP21 manifested as solid organoids, while those derived from BCP22 were cystic, and those from BCP27 were “grape-shaped” ().
Next, we evaluated the response of different patients to NAC. Patients who received NAC received modified radical mastectomy after chemotherapy and colour ultrasound evaluation. Surgical specimens were collected after chemotherapy, and the pathology department of the First Affiliated Hospital of Chongqing Medical University assessed whether the patient had achieved complete pathological response (pCR), which was defined as a primary tumour with no invasive cancer. The regional lymph nodes were negative (ypT0/Tis ypN0).
The surgical tissue evaluation revealed that, among the patients from whom the five successfully constructed organoids were derived, two patients achieved pCR after NAC. The bright-field images of the biopsy organoids and HE stain showed that the two specimens that responded well to chemotherapy showed “grape-like” cell clusters. HE stain of the postoperative tissues showed the tumours of patients who achieved pCR did not cross the myoepithelial cell layer. Cells and basement membranes entered the surrounding interstitial tissue. However, there was no infiltration of cancer cells into the adjacent tissues, or only a few cancer cells were seen ().
Overall, these results indicate that organoids from BC biopsy tissue can be passaged and survive for a long time in vitro; therefore, biopsy-derived organoids could be used to predict patient responses to NAC.
3.3. Histological characterization of BC organoids
Most BCOs were determined to be malignant due to cell and nuclear atypia, such as typical cancerous features, enlarged nuclei and polymorphisms, high mitotic activity, apoptosis and vacuole formation ().
Figure 3. Histological characterization of BC organoids. (A,B) Immunohistochemical comparison of tissues of luminal B and Her2+ breast cancer patients after neoadjuvant chemotherapy and organoids of biopsy specimens before neoadjuvant chemotherapy. Tissues generally present tumour epithelium surrounded by mesenchymal and inflammatory cells, while organoids are exclusively epithelial with tumour cell organization being remarkably well conserved (HE). ER, PR, HER2 status and proliferation index of original BCs are similarly well retained in the derived organoid lines. Scale bar, 100 mm.
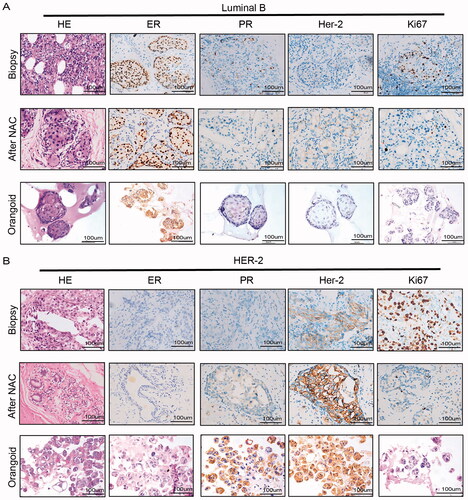
In addition to the histological features visible by HE stains, representative BCOs should retain the expression of the most critical and common breast cancer biomarkers, such as oestrogen receptor (ER), progesterone receptor (PR), antigen Ki-67 and human epidermal growth factor receptor (HER2). The ER and PR status could predict the outcome of hormone/endocrine therapy, while the level of HER2 could predict the outcome of targeted therapy [Citation36,Citation37]. Our immunohistochemistry results showed that hormone receptor (HR) and HER2 status remained unchanged in most breast cancer tissues and PDOs. In this study, hormone receptor (HR)-negative tumours produced HR-negative organoids, and HR-positive tumours produced HR-positive tumour organoids (). HER2 status was preserved in BC tissues and organoids (). Due to the small sample size, 100% of breast cancer biomarkers in the tissue were retained.
In summary, we found that most BCOs matched the original tumour in terms of histopathology, hormone receptor levels and HER2 receptor status.
3.4. Response of human epidermal growth factor receptor-2 (HER-2)-positive PDOs to docetaxel and carboplatin
Five patients from whom biopsy tissues were collected received NAC according to the BC clinical guidelines of the Chinese Society of Clinical Oncology. The clinical information and data regarding these five patients (BCP20-22, BCP27, BCP30) are summarized in . The biopsy results of BCP21 and BCP22 revealed HER-2 positivity, and they received TCb and TCbHP (T: paclitaxel, Cob: carboplatin, H: trastuzumab, P: epratuzumab) schemes, respectively. The specific treatment plans are shown in .
The organoids derived from the biopsy tissue of HER-2-positive patients were exposed to the same drugs administered to the patients. Since no HER-2 monoclonal antibody was used in this study, HER-2-positive organoids were only exposed to docetaxel and carboplatin. To assess the response of organoids to docetaxel and carboplatin, we observed changes in the size of organoids and the growth of the spheroids after the dynamic administration of docetaxel and carboplatin (). Then, the number of surviving cells was quantified by the CellTiter-Glo 3 D cell viability assay to verify the responsiveness of the organoids to the drugs (). As shown in ), after treatment with different concentrations of docetaxel for six days, the size and morphology of BCb21 and BCb22 organoids did not change. The survival rate of the two organoids was subsequently observed to be greater than 50%. The responsiveness of carboplatin treatment was also assessed, as shown in ), after six days of treatment with different concentrations of carboplatin, the size and morphology of BCb22 organoids was not significantly changed, while the cell clusters of BCb21 organoids decreased, the colour darkened, and the cell mass lysed into individual cells. The CellTiter-Glo 3 D cell viability assay showed that after treatment with different concentrations of carboplatin, the sensitivity of BCb22 organoids to carboplatin was significantly greater than that of BCb21 organoids. We compared the IC50 values of the two organoids to carboplatin and found that the logIC50 values of BCb21 and BCb22 were 16.90 µM and −1.299 µM, respectively (). The IC50 of the former was more than five times larger than that of the latter.
Figure 4. Sensitivity of PDOs derived from puncture tissues to docetaxel and carboplatin in patients with HER2-positive breast cancer. (A,C): The bright field shows the changes in the size and status of HER2-positive organoids after treatment with different drug concentrations of docetaxel (A) and carboplatin (C) (the upper row represents the organoids not treated with the drug, and the lower row represents the organoids treated with different drug concentrations for six days). Scale bar, 150 mm. (B,D): The line graph shows the survival of HER2-positive organoids treated with different drug concentrations of docetaxel (B) and carboplatin (D).
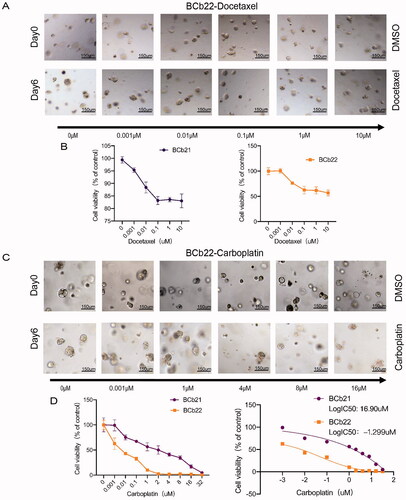
We observed that HER2-positive organoids were insensitive to the paclitaxel drug docetaxel through in vitro experiments. BCb22 was sensitive to carboplatin, while BCb21 was resistant to carboplatin.
Next, we matched the organoid drug sensitivity data with the patient’s clinical results. According to pathology, ypT0/Tis ypN0 is generally defined as pCR; ductal carcinoma in situ may remain in the breast after chemotherapy, but no residual cancer cells in the axillary lymph nodes can be present. According to the assessment of postoperative tissues by the Department of Pathology, the First Affiliated Hospital of Chongqing Medical University, BCP22 reached pCR after NAC, while BCP21 did not. Thus, among HER-2-positive patients, the response of the tissue-derived organoids to the drugs was consistent with the patients’ clinical response.
3.5. Response of organoids from patients receiving the TAC regimen to NAC
According to the classification of molecular subtypes, the biopsy, and pathological results of BCP20 and BCP27 showed that their tumours were negative for HR and HER-2, indicating TNBC. The pathological results of BCP30’s biopsy revealed ER positivity (60%), weak PR positivity (2%) and Ki67 positivity (40%). This patient’s cancer axillary lymph node biopsy was also positive. All these patients received NAC with a TAC regimen (T: paclitaxel, E: anthracycline, C: cyclophosphamide). The specific treatment plans are shown in .
While the patients received TAC for four to six months, the organoids derived from these patients could not be assessed for cyclophosphamide responsiveness as this drug can only be metabolized in vivo; thus, our drug sensitivity test was limited to docetaxel and anthracyclines (epirubicin).
To observe the response of the organoids to docetaxel and epirubicin, we assessed the changes in organoid size and the growth of spheroids after dynamic administration of docetaxel and epirubicin (). CellTiter-Glo 3 D cell viability measurements were directly compared to verify the organoid size measurement (). As shown in ), after treatment with different concentrations of docetaxel for six days, the size and morphology of BCb20, BCb27 and BCb30 organoids did not change. The CellTiter-Glo 3 D cell viability determination revealed that the survival rate of the two organoids was greater than 50%. As shown in ), after six days of treatment with different concentrations of epirubicin, the size and morphology of BCb27 organoids did not change significantly, while the cell clusters of BCb20 organoids decreased, the colour became darker, and the cell mass lysed into individual cells. The CellTiter-Glo 3 D cell viability assay showed that after treatment with different concentrations of epirubicin, the sensitivity of BCb27 and BCb30 organoids to epirubicin was significantly higher than that of BCb20 organoids. The logIC50 values of BCb20, BCb27, and BCb30 were −0.3728 µM, 26.7300 µM and 0.1719 µM, respectively (). The IC50 of BCb27 and BCb30 was more than five times higher than that of BCb20.
Figure 5. Sensitivity of PDOs derived from HER2-negative breast cancer patients to docetaxel and anthracyclines. (A,C): The bright field shows the changes in the size and status of HER2-negative organoids after treatment with different drug concentrations of docetaxel and epirubicin (the upper row represents the organoids treated without the drugs, and the lower row represents the organoids treated with different drug concentrations for six days). Scale bar, 150 mm. (B): The line graph shows the survival rate of HER2-negative organoids treated with different drug concentrations of docetaxel and epirubicin. (D): Comparison of the sensitivity of HER2-negative organoids from the biopsy sample to epirubicin, and the logIC50 of each type of organoid to epirubicin.
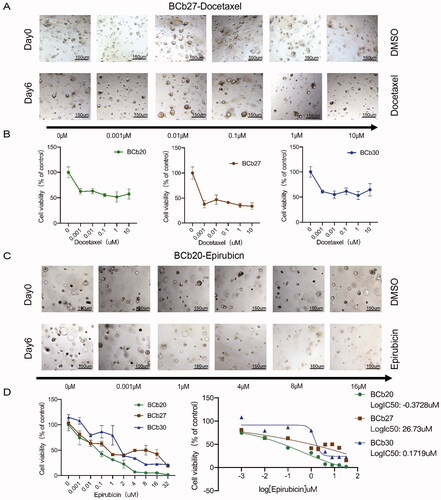
The in vitro experiments suggest that organoids derived from triple-negative and luminal B-type BCs responded in the same manner as the parental tumours and the HR-positive organoids were not sensitive to docetaxel. BCb20 was sensitive to epirubicin, and BCb27 and BCb30 were resistant to epirubicin.
Next, we matched the organoid drug sensitivity data with the patient’s clinical results. The pathological evaluation results of the Pathology Department of the First Affiliated Hospital of Chongqing Medical University showed that BCP20 achieved pCR after receiving TAC NAC, while BCP27 and BCP30 did not. In summary, the clinical response of the TNBC and HR + patients was consistent with the response of tissue-derived organoids to drugs.
3.6. Responsiveness of PDOs to combination medications
Breast cancer patients who meet the indications for NAC are typically administered multidrug combinations or NP sequential therapy when undergoing preoperative chemotherapy. Therefore, we also tried to match the clinical treatment plan for assessing BC organoid responses to drugs. TCbHP, TCBH, or THP regimens are often administered to HER2+ BC patients. Due to the lack of monoclonal antibodies, we used paclitaxel in combination with carboplatin to mimic the preoperative novelty of HER2-positive BC patients’ NAC. TAC is often used clinically for BC patients who are negative for HER-2 to reduce tumour staging, rapidly reduce tumour volume and increase breast-conserving opportunities. For HER2-negative breast cancer patients, preoperative adjuvant endocrine therapy and adjuvant immunotherapy are rarely performed clinically. Therefore, for organoids derived from HER2-negative breast cancer, we used anthracyclines combined with paclitaxel to treat simulated patients with neoadjuvant chemotherapy. For HER2-positive PDOs, as shown in ), the changes in organoid size and the growth of spheroids after docetaxel combined with carboplatin were dynamically observed. When using 0.1 µM docetaxel combined with different concentrations of carboplatin for six days, the size and morphology of BCb21 cells did not change. The CellTiter-Glo 3 D cell viability assay showed that the LogIC50 of BCb21 was 4.6860 µM.
Figure 6. Patient-derived organoids show different sensitivities to the combination of epirubicin and docetaxel and the combination of carboplatin and docetaxel. (A,C): The bright field shows the changes in the size and status of organoids treated with different drug concentrations of carboplatin and 0.1 μM docetaxel (the upper row represents the organoids without drug treatment, and the lower row represents the organoids treated with different drug concentrations for six days). Scale bar, 150 mm. (B): The line graph shows the survival rate and IC50 of carboplatin combined with docetaxel in the treatment of HER2-positive organoids. (C): The bright field shows the changes in the size and status of organoids treated with different drug concentrations of epirubicin and 0.1 μM docetaxel (the upper row represents the organoids without drug treatment, and the lower row represents the organoids treated with different drug concentrations for six days). Scale bar, 150 mm. (D): The line graph shows the survival rate and IC50 of the combined treatment of epirubicin and docetaxel in HER2-negative organoids. (E): The organoid response data matches the patient’s clinical results (the first row: the sensitivity of HER2-positive organoids to epirubicin; the second row: the sensitivity of HER2-negative organoids to epirubicin; the third row: The bottom of the sensitivity of different types of organoids to docetaxel; the fourth row: the drug reactivity of the two-drug combination of different types of organoids; bottom: the heat map of the organoid response and the patient’s clinical results, we can draw the conclusion that organoids can predict the prognosis of patients with neoadjuvant chemotherapy).
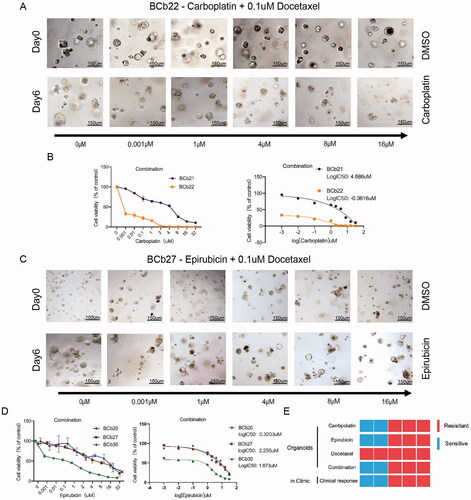
In contrast, cell spheres in BCb22 organoids treated with 0.1 µM docetaxel combined with different concentrations of carboplatin for six days did not increase and in some cases, the cell clusters broke down into single cells. The colour of the organoids darkened, and the CellTiter-Glo 3 D cell viability assay indicated that the LogIC50 of BCb21 was −0.3616 µM. Thus, in agreement with the monotherapy results, BCb21 was resistant to docetaxel combined with carboplatin, while BCb22 was sensitive to the combined treatment. As shown in ), we dynamically observed the changes in organoid size and the growth of spheres after treatment with anthracyclines combined with paclitaxel. When we treated the organoids with 0.1 µM docetaxel combined with different concentrations of epirubicin, after six days, the size and morphology of BCb27 and BCb30 cells did not change. The CellTiter-Glo 3 D cell viability assay showed that the logIC50 values of the two were 2.2550 µM and 1.8730 µM, respectively.
In contrast, in the observation of BCb20, after using 0.1 µM docetaxel combined with different concentrations of epirubicin to treat the organoids for six days, the organoid cell spheres did not increase, and in some cases, the cell clusters broke down into individual cells. The colour of the cells darkened, and the CellTiter-Glo 3 D cell viability assay indicated that the logIC50 of BCb20 was 0.3203 µM. Thus, consistent with the results observed with the monotherapy, BCb27 and BCb30 were resistant to anthracycline combined with docetaxel, while BCb20 was sensitive to the combined treatment.
Comparing the clinical responses with the results of the in vitro experiments, we concluded that our in vitro treatment data were consistent with the patient’s clinical responsiveness (). In addition, our data showed that when the patient’s tumour organoids were sensitive to at least one of the two therapeutic components, the patient achieved an excellent clinical response. Therefore, it may be possible to use organoids to test patient responses to monotherapy and predict the drug sensitivity of patients receiving NAC or adjuvant chemotherapy.
4. Discussion
In general, it is believed that patients who achieve pCR have a better outcome than those who do not [Citation36,Citation37]. Thus, pCR is an established prognostic factor. However, whether BC patients undergoing NAC can achieve pCR is unknown. In clinical practice, the value of a single treatment cannot be evaluated in individual patients undergoing combined therapy. Therefore, it is impossible to individually screen the sensitivity of BC patients with different molecular subtypes to drugs to achieve individualized and precise treatment.
Patient-derived tumour organoids are promising tools for personalized cancer medicine approaches [Citation38]. Importantly, PDOs may be superior models for drug screening [Citation39,Citation40] and the prediction of cancer treatment responses [Citation32,Citation39,Citation41].
We successfully constructed organoids from BC biopsies to simulate clinical treatment, demonstrating that viable tumoroid lines can be generated from a small sample of tissue. TCbH, TCbHP or THP are clinically administered as NAC for patients with HER2-positive breast cancer. For patients with HER2-positive breast cancer, dual-target therapy has been routinely recommended in clinical practice and achieved good results. Commonly used HER2 monoclonal antibodies include trastuzumab and pertuzumab. Trastuzumab is a humanized 4D5 monoclonal antibody, and its anti-tumour effects in HER2-positive breast cancer mainly include two types: (a) Competitively bind to the HER-2 receptor and block the HER-2 signalling pathway. turn on, thereby inhibiting tumour cell proliferation (b) indirect antitumour activity (ADCP) by binding to Fcγ receptors (FCGRs) on immune cells to induce antibody-dependent cellular cytotoxicity (ADCC) or antibody-dependent phagocytosis, or (c) activate the complement cascade leading to complement-dependent cytotoxicity (CDC) or complement-dependent phagocytosis [Citation42]. However, according to research reports, although trastuzumab has a high affinity for HER2 in vitro experiments, it loses the ability to resist cell proliferation. Even at high concentrations of trastuzumab, only partial or no effect on HER2 expression or signalling. Therefore, we abandoned HER2 monoclonal antibody therapy and adopted docetaxel combined with carboplatin as a treatment modality for HER2-positive breast cancer patient-derived organoids [Citation43]. TAC is administered as a NAC for patients with HER2-negative breast cancer. Therefore, we evaluated anthracycline combined with paclitaxel therapy or as a monotherapy using PDOs derived from HER2-negative patients. The histological verification of organoids and the results of the in vitro treatments showed that the organoids simulated the histological characteristics of the parental tumour and the clinical response of the individual patients to chemotherapy, demonstrating potential of PDOs in precision medicine.
However, our organoid construction success rate from puncture samples was insufficient. The main limitation of successfully establishing organoids from our biopsy tissues was the insufficient number of viable tumour cells in the biopsy material and the low mutation load of luminal BC. Furthermore, the duration required for organoid expansion in vitro varies from patient to patient and depends on the size of the biopsy tissue, the proportion of cancer cells in the tissue, and the cell proliferation rate. It took two weeks to successfully construct PDOs from BC biopsies, and approximately one month for the PDOs to achieve sufficient cell mass for in vitro treatment. It is unknown whether a patient’s tumour will progress during the one month in vitro drug screening of the organoids. Nevertheless, organoids were shown to be a viable in vitro model for predicting chemotherapy response and even radiotherapy response.
Since this study did not include monoclonal antibodies and cyclophosphamide, PDOs could not be used to assess the clinical responsiveness of patients without bias. A natural progression of this work is to construct a mouse model of patient-derived organoids and add monoclonal antibodies and cyclophosphamide to conduct in vivo experiments to further verify that organoids can be used as a tool for treatment response modelling.
We successfully constructed organoids from BC biopsy samples and proved the consistency of the organoids and tumour samples by histology. Our results indicate that organoid data can predict the clinical response of patients to NAC. The PDO model may identify BC patients who respond to NAC and thereby improve initial care. For patients with BC who are chemo-insensitive, PDOs could be used to select targeted or investigational agents, avoid overtreatment, and reduce treatment-induced side effects. In summary, our data imply that PDOs can predict BC patient responses in the clinic and could potentially be used as a companion tool for BC treatment selection.
5. Conclusions
The results of our analyses suggest that patient-derived organoids could predict the responses of BC patient in the clinic and may be possible to use organoids to test patient responses to monotherapy and predict the drug sensitivity of patients receiving NAC or adjuvant chemotherapy. In addition, PDOs could potentially be used as a companion tool for BC treatment selection.
Ethical approval
This study was performed in line with the principles of the Declaration of Helsinki. The studies involving human participants were reviewed and approved by the Human Research Ethics Committee of the First Affiliated Hospital of Chongqing Medical University. The approval number is 2020-279. This article does not contain any studies with animals performed by any of the authors.
Consent form
Informed consent was obtained from all individual participants included in the study.
Author contributions
S.C.L., A.S.J. and D.S. contributed to the study conception and design. Analysis and interpretation of the data were performed by D.S.; the drafting of the paper was written by D.S.; M.Y.S., K.L. and X.J.H. revised it critically for intellectual content; H.L., Z.F.T., Y.W., Y.P., Z.R.T. and C.Q. commented on previous versions of the manuscript, and that all authors agree to be accountable for all aspects of the work.
Abbreviations | ||
BC | = | Breast cancer |
NAC | = | Neoadjuvant chemotherapy |
PDOs | = | Patient-derived organoids |
BCO | = | Breast cancer-derived organoids |
BCb | = | Biopsy sample-derived breast cancer organoids |
BCP | = | Breast cancer patient |
pCR | = | Complete pathological response |
ER | = | Oestrogen receptor |
PR | = | Progesterone receptor |
HER-2 | = | Human epidermal growth factor receptor 2 |
TNBC | = | Triple-negative breast cancer |
LGR5 | = | G protein-coupled receptor 5+ |
BME | = | Cultrex Reduced Growth Factor Basement Membrane Extract |
Supplemental Material
Download JPEG Image (160.3 KB)Acknowledgments
All authors are acknowledged for their contribution to the study.
Disclosure statement
No potential conflict of interest was reported by the author(s).
Data availability statement
The data that support the findings of this study are available from the corresponding author, Shengchun Liu, upon reasonable request.
Additional information
Funding
References
- Sung H, Ferlay J, Siegel RL, et al. Global Cancer Statistics. 2020. GLOBOCAN estimates of incidence and mortality worldwide for 36 cancers in 185 countries. CA Cancer J Clin. 2021;71(3):209–249.
- DeSantis CE, Ma J, Goding Sauer A, et al. Breast cancer statistics, 2017, racial disparity in mortality by state. CA Cancer J Clin. 2017;67(6):439–448.
- McDonald ES, Clark AS, Tchou J, et al. Clinical diagnosis and management of breast cancer. J Nucl Med. 2016;57(Suppl 1):9s–16s.
- Perou CM, Sørlie T, Eisen MB, et al. Molecular portraits of human breast tumours. Nature. 2000;406(6797):747–752.
- Sorlie T, Tibshirani R, Parker J, et al. Repeated observation of breast tumor subtypes in independent gene expression data sets. Proc Natl Acad Sci U S A. 2003;100(14):8418–8423.
- Sørlie T, Perou CM, Tibshirani R, et al. Gene expression patterns of breast carcinomas distinguish tumor subclasses with clinical implications. Proc Natl Acad Sci U S A. 2001;98(19):10869–10874.
- Dent R, Trudeau M, Pritchard KI, et al. Triple-negative breast cancer: clinical features and patterns of recurrence. Clin Cancer Res. 2007;13(15):4429–4434.
- von Minckwitz G, Blohmer JU, Costa SD, et al. Response-guided neoadjuvant chemotherapy for breast cancer. J Clin Oncol. 2013;31(29):3623–3630.
- Early Breast Cancer Trialists' Collaborative Group (EBCTCG). Long-term outcomes for neoadjuvant versus adjuvant chemotherapy in early breast cancer: meta-analysis of individual patient data from ten randomised trials. Lancet Oncol. 2018;19(1):27–39.
- Aronson SJ, Rehm HL. Building the foundation for genomics in precision medicine. Nature. 2015;526(7573):336–342.
- Torre LA, Bray F, Siegel RL, et al. Global cancer statistics, 2012. CA Cancer J Clin. 2015;65(2):87–108.
- Kamb A. What’s wrong with our cancer models? Nat Rev Drug Discov. 2005;4(2):161–165.
- Caponigro G, Sellers WR. Advances in the preclinical testing of cancer therapeutic hypotheses. Nat Rev Drug Discov. 2011;10(3):179–187.
- Whittle JR, Lewis MT, Lindeman GJ, et al. Patient-derived xenograft models of breast cancer and their predictive power. Breast Cancer Res. 2015;17(1):17.
- Cheon DJ, Orsulic S. Mouse models of cancer. Annu Rev Pathol. 2011;6:95–119.
- Carmon KS, Gong X, Lin Q, et al. R-spondins function as ligands of the orphan receptors LGR4 and LGR5 to regulate Wnt/β-catenin signaling. Proc Natl Acad Sci U S A. 2011;108(28):11452–11457.
- Sato T, Vries RG, Snippert HJ, et al. Single Lgr5 stem cells build crypt-villus structures in vitro without a mesenchymal niche. Nature. 2009;459(7244):262–265.
- de Lau W, Barker N, Low TY, et al. Lgr5 homologues associate with Wnt receptors and mediate R-spondin signalling. Nature. 2011;476(7360):293–297.
- Jung P, Sato T, Merlos-Suárez A, et al. Isolation and in vitro expansion of human colonic stem cells. Nat Med. 2011;17(10):1225–1227.
- Sato T, Stange DE, Ferrante M, et al. Long-term expansion of epithelial organoids from human Colon, adenoma, adenocarcinoma, and Barrett’s epithelium. Gastroenterology. 2011;141(5):1762–1772.
- Boj SF, Hwang CI, Baker LA, et al. Organoid models of human and mouse ductal pancreatic cancer. Cell. 2015;160(1-2):324–338.
- Chua CW, Shibata M, Lei M, et al. Single luminal epithelial progenitors can generate prostate organoids in culture. Nat Cell Biol. 2014;16(10):951–961.
- Karthaus WR, Iaquinta PJ, Drost J, et al. Identification of multipotent luminal progenitor cells in human prostate organoid cultures. Cell. 2014;159(1):163–175.
- Bartfeld S, Bayram T, van de Wetering M, et al. In vitro expansion of human gastric epithelial stem cells and their responses to bacterial infection. Gastroenterology. 2015;148(1):126.e126–136.e126.
- Kessler M, Hoffmann K, Brinkmann V, et al. The Notch and Wnt pathways regulate stemness and differentiation in human fallopian tube organoids. Nat Commun. 2015;6:8989.
- Maimets M, Rocchi C, Bron R, et al. Long-term in vitro expansion of salivary gland stem cells driven by Wnt signals. Stem Cell Reports. 2016;6(1):150–162.
- DeWard AD, Cramer J, Lagasse E. Cellular heterogeneity in the mouse esophagus implicates the presence of a nonquiescent epithelial stem cell population. Cell Rep. 2014;9(2):701–711.
- Rock JR, Onaitis MW, Rawlins EL, et al. Basal cells as stem cells of the mouse trachea and human airway epithelium. Proc Natl Acad Sci U S A. 2009;106(31):12771–12775.
- Turco MY, Gardner L, Hughes J, et al. Long-term, hormone-responsive organoid cultures of human endometrium in a chemically defined medium. Nat Cell Biol. 2017;19(5):568–577.
- Sachs N, de Ligt J, Kopper O, et al. A living biobank of breast cancer organoids captures disease heterogeneity. Cell. 2018;172(1-2):373–386.e310.
- Drost J, Clevers H. Organoids in cancer research. Nat Rev Cancer. 2018;18(7):407–418.
- Vlachogiannis G, Hedayat S, Vatsiou A, et al. Patient-derived organoids model treatment response of metastatic gastrointestinal cancers. Science. 2018;359(6378):920–926.
- van de Wetering M, Francies HE, Francis JM, et al. Prospective derivation of a living organoid biobank of colorectal cancer patients. Cell. 2015;161(4):933–945.
- Ogston KN, Miller ID, Payne S, et al. A new histological grading system to assess response of breast cancers to primary chemotherapy: prognostic significance and survival. Breast. 2003;12(5):320–327.
- Wang-Lopez Q, Chalabi N, Abrial C, et al. Can pathologic complete response (pCR) be used as a surrogate marker of survival after neoadjuvant therapy for breast cancer? Crit Rev Oncol Hematol. 2015;95(1):88–104.
- Rastogi P, Anderson SJ, Bear HD, et al. Preoperative chemotherapy: updates of national surgical adjuvant breast and bowel project protocols B-18 and B-27. J Clin Oncol. 2008;26(5):778–785.
- Kong X, Moran MS, Zhang N, et al. Meta-analysis confirms achieving pathological complete response after neoadjuvant chemotherapy predicts favourable prognosis for breast cancer patients. Eur J Cancer. 2011;47(14):2084–2090.
- Pauli C, Hopkins BD, Prandi D, et al. Personalized in vitro and in vivo cancer models to guide precision medicine. Cancer Discov. 2017;7(5):462–477.
- Tiriac H, Belleau P, Engle DD, et al. Organoid profiling identifies common responders to chemotherapy in pancreatic cancer. Cancer Discov. 2018;8(9):1112–1129.
- Broutier L, Mastrogiovanni G, Verstegen MM, et al. Human primary liver cancer-derived organoid cultures for disease modeling and drug screening. Nat Med. 2017;23(12):1424–1435.
- Schütte M, Risch T, Abdavi-Azar N, et al. Molecular dissection of colorectal cancer in pre-clinical models identifies biomarkers predicting sensitivity to EGFR inhibitors. Nat Commun. 2017;8:14262.
- Tsao LC, Crosby EJ, Trotter TN, et al. Trastuzumab/pertuzumab combination therapy stimulates antitumor responses through complement-dependent cytotoxicity and phagocytosis. JCI Insight. 2022;7(6):e05000.
- Moasser MM. Two dimensions in targeting HER2. J Clin Oncol. 2014;32(19):2074–2077.