Abstract
Cardiorenal syndrome type I (CRS I) is defined as the development of acute kidney injury (AKI) following acute decompensated heart failure (ADHF). The clinical significance of endothelial markers in ADHF-associated AKI has yet to be clarified. This study therefore investigated the biological processes linking ADHF and AKI with the aim of determining whether the plasma markers of endothelial injury and activation are associated with AKI in patients with ADHF. The study prospectively recruited 125 consecutive patients admitted to a coronary critical unit due to ADHF. Patients with and without AKI were compared in terms of soluble thrombomodulin (sTM), angiopoietin (Ang)-1 and −2 plasma levels as well as baseline characteristics. Among the study population, 14 (11.2%) patients developed CRS within 7 days after admission. The hemoglobin levels (median [IQR]11.3[10.8–12.6] vs. 13.5 [12.2–15.0] g/dL, p = 0.003) and baseline eGFR (66.5[35.7–87.9] vs. 78.5 [64.9–107.5] mL/minute/1.73m2, p = 0.044) of patients with CRS were lower than those of patients without CRS. Patients with CRS also presented elevated plasma levels of BNP (1317.5 [222.6–3375.5] vs. 258.2 [63.2–925.8] pg/mL, p = 0.008), Ang-2 (3993.0 [1561.3–15722.7] vs. 1805.9 [1196.9–3302.3] pg/mL, p = 0.006), and sTM (6665.7 [4707.1–11947.3] vs. 4132.2 [3338.0–5531.8] ng/mL, p < 0.001), compared to patients without CRS. Multivariate logistic regression analysis based on forward stepwise method identified that log sTM was the only independent risk factor for AKI (OR, 13.83; 3.02–63.28, p = 0.001). Furthermore, higher sTM levels were associated with AKI in patients with ADHF. These findings suggest a novel approach to dealing with kidney injury in the context of ADHF, involving the use of baseline biomarker profiles to identify individuals at risk of developing AKI.
The clinical significance of endothelial markers in acute decompensated heart failure (ADHF)-associated acute kidney injury (AKI) has not previously been clarified. This study revealed that markers of endothelial injury (i.e. plasma soluble thrombomodulin (sTM) levels) were higher in ADHF patients with AKI than in those without AKI.
Multivariate analysis identified sTM level > cutoff value of 4,855.2 pg/mL as an independent factor associated with the development of AKI. sTM could potentially be used as a biomarker to predict the development of AKI in patients with heart failure.
These findings suggest a novel approach to dealing with kidney injury in the context of ADHF, involving the use of baseline biomarker profiles to identify individuals at risk of developing AKI.
KEY MESSAGES
Introduction
There is emerging evidence indicating a link between heart failure and renal insufficiency. The occurrence of cardiac and renal dysfunction can have a profound impact on clinical outcomes [Citation1,Citation2]. Cardiorenal syndromes are disorders of the heart and kidneys, whereby dysfunction in one organ leads to dysfunction in the other organ. Cardiorenal syndrome type I (CRS I) is defined as the development of acute kidney injury (AKI) following acute decompensated heart failure (ADHF) [Citation3]. According to previous reports, AKI events developed in 12–37% of patients hospitalized for ADHF [Citation4]. Patients with CRS I may have worse clinical outcomes including increased cardiovascular mortality rate, longer length of hospital stays, and higher chances of re-hospitalization [Citation5].
The complex pathophysiology of CRS type 1 has yet to be fully elucidated. Recent evidence suggests that endothelial activation/injury are involved in pathogenesis of CRS I [Citation6]. Endothelial injury may cause decreased glomerular filtration rates and impaired diuretic efficacy. These effects subsequently result in fluid retention and venous congestion, therefore, the cardiac output and renal perfusion are depressed [Citation7]. Thrombomodulin (TM) and angiopoietins (Ang) play important roles in the process of endothelial activation and injury [Citation8,Citation9]. TM, a membrane-bound glycoprotein predominantly expressed in endothelial cells, has been shown to neutralize the clotting activity of thrombin and activate the anti-coagulant and anti-inflammatory properties of protein C [Citation10]. A circulating soluble form of TM (sTM) is also released into the plasma through proteolytic degradation. The fact that sTM is released from the surfaces of endothelial cells only after injury makes it a recognizable marker of endothelial injury [Citation11]. One previous study reported elevated sTM levels in patients with acute heart failure but not in those with chronic heart failure [Citation12]. Researchers have also reported that elevated sTM plasma levels are associated with kidney injury following sepsis [Citation8] and acute myocardial infarction [Citation13].
The site to which Ang-1 and Ang-2 bind is the same as that of the endothelial cell-specific Tie-2 receptor [Citation14]. Ang-1 and Ang-2 are crucial to vascular development, maturation, and inflammation. After binding to the Tie-2 receptor, Ang-1 triggers the activation of Tie-2, whereas Ang-2 exerts an antagonistic response to Tie-2. Ang-1 is protective in its anti-inflammatory effects and stabilization of the endothelium, whereas Ang-2 promotes an inflammatory response by activating endothelial cells and enhancing vascular leakage [Citation15]. The importance of angiopoietins in endothelial activation and injury to the vascular barrier has prompted extensive research into their role as biomarkers in critical illness [Citation16]. Plasma Ang-2 levels are significantly higher in cases of acute heart failure than in healthy controls, making them a predictor of poor outcomes [Citation17]. They are also an independent predictor of mortality in patients undergoing dialysis in intensive care units [Citation18].
The markers of endothelial injury and dysfunction have been associated with poor outcomes in patients with cardiovascular disease; however, the clinical role of endothelial injury markers in predicting AKI events of heart failure patients has not been clarified. This study investigated the underlying biological processes linking ADHF and AKI, with the aim of determining whether the plasma markers of endothelial injury and activation are associated with AKI in patients with ADHF.
Materials and methods
Study population
This study was conducted between November 2012 and September 2013 in the 14-bed coronary care unit (CCU) of a tertiary medical center (Chang Gung Memorial Hospital). The study prospectively recruited 125 consecutive patients who were admitted to the CCU due to ADHF. The reasons for CCU admission included: acute coronary syndrome, ADHF, or impending HF with the need for intensive monitoring. ADHF was diagnosed in accordance with the European Society of Cardiology criteria [Citation19]. Exclusion criteria included age under 18 years old, having undergone organ transplant prior to admission, or end-stage renal failure. Plasma specimens were obtained from all patients within 24 h of CCU admission, and all patients were followed until hospital discharge or death. The Institutional Review Board of Chang Gung Memorial Hospital approved the protocols for recruitment and sample collection. Informed written consent was obtained from all participants.
Data collection
The study collected the demographic data of patients on the first day of admission to the CCU. The underlying comorbidities were recorded. The left ventricular ejection fraction (LVEF) was derived using the modified Simpson’s method via echocardiography at the time of admission. The major adverse cardiac events (MACE) were defined as patients with previous history of recurrent myocardial infarction (MI), hospital admission for heart failure, unplanned repeat revascularization, malignant dysrhythmia, stroke, and pulmonary embolism [Citation16].
The estimated glomerular filtration rate (eGFR) was calculated using the Chronic Kidney Disease Epidemiology Collaboration (CKD-EPI) equation [Citation20]. Cases of baseline eGFR <60 were defined as chronic kidney disease (CKD). High-sensitivity C-reactive protein (hsCRP), hemoglobin (Hb), platelet, and white blood cell (WBC) counts were measured upon admission. The blood samples were collected into EDTA vacutainers and placed on ice immediately. Within 30 min of collection, samples were centrifuged at 3000 rpm (1000 g) and 4 °C for 15 min. Plasma was kept frozen in aliquots. Complete blood cell count and all biochemical analyses were performed in the Department of Laboratory Medicine, Chang Gung Memorial Hospital. Our hospital authority has permitted the checking of serum BNP levels in all patients with heart failure since January 2006. Serum BNP levels were measured using the microparticle enzyme immunoassay test (Abbott, Chicago, IL, USA). In this study, all BNP levels were obtained within one hour after presentation at the emergency department or ICU. The low osmolar iodine contrast was used when imaging examination was needed.
Outcome assessment
The dependent variable in this study was the occurrence of acute CRS 1. AKI events were defined as any of the following criteria occurring within 7 days after admission: an increase in serum creatinine levels of ≥0.3 mg/dL within a period of 48 h or an increase in serum creatinine level of ≥1.5 times from baseline within a period of 7 days. Note that both of these criteria are recommended in the Kidney Disease Improving Global Outcomes practical clinical guidelines for AKI [Citation21].
Enzyme-linked immunosorbent assay (ELISA)
Plasma was collected from blood taken at the time of admission. The concentrations of sTM, von Willebrand factor (vWF), Ang-1, Ang-2, and Tie-2 were determined by ELISA in accordance with the manufacturer’s instructions (Sekisui Diagnostics, MA). The measured values were compared between patients with and without AKI.
Statistical analysis
All data are expressed as medians with interquartile ranges (IQR) or mean ± standard deviation (S.D.) unless otherwise indicated. Equality of variances between groups was be assessed by Levene’s test. An unpaired Student’s t-test was used for normally distributed quantitative variables, while the Mann–Whitney test was used for non-normally distributed variables. Categorical variables were tested using the Chi-square test or Fisher’s exact test. Receiver operating characteristic (ROC) curves for day 1 plasma levels of Ang-2 and sTM were plotted to predict the development of AKI, and the respective areas under the ROC curves (AUROC) were calculated. Cut-off values were derived by the ROC curves using threshold values maximizing the sum of sensitivity and specificity. Univariate analysis was primarily performed to search for predictors for AKI. After identifying the variables with a p-value <0.1 in univariate regression analysis, a multivariate logistic regression analysis (stepwise forward) was used to determine independent predictive factors for the development of AKI. A p-value <0.05 using a two-sided test was considered statistically significant. All analysis was performed using SPSS software package, version 20.0 (SPSS, Inc, Chicago, IL, USA).
Results
This study recruited a total of 125 consecutive patients. Among them, 14 (11.2%) patients developed CRS within 7 days after admission to the CCU. The demographic characteristics, biomarkers, and clinical presentations of this group are listed in . Compared with patients without CRS, those with CRS were older (median [IQR], 73[66.8–75.8]) vs.63 [54–74] years old; p = 0.024), had a lower incidence of coronary heart disease (35.7% vs. 71.2%, p = 0.013), were less likely to use ACEI/ARB (57.1 vs. 84.7%, p = 0.022), and were more likely to have LVEF <40% (50.0 vs. 22.5%, p = 0.026). Compared to patients without CRS, those with CRS presented lower median hemoglobin levels (11.3[10.8–12.6] vs. 13.5 [12.2–15.0] g/dL, p = 0.003) and baseline eGFR levels (66.5[35.7–87.9] vs. 78.5 [64.9–107.5] mL/minute/1.73m2, p = 0.048). Patients with CRS also presented higher BNP median plasma levels (1317.5 [222.6–3375.5] vs. 258.2 [63.2–925.8] pg/mL, p = 0.008), Ang-2 (3993.0 [1561.3–15722.7] vs. 1805.9 [1196.9–3302.3] pg/mL, p = 0.006), and sTM (6665.7 [4707.1–11947.3] vs. 4132.2 [3338.0–5531.8] ng/mL, p < 0.001). No significant differences were observed between the two groups in terms of gender, comorbidities, CKD, smoking status, body mass index (BMI), blood pressure, heart rate, leukocyte count, hsCRP, VEGF, vWF, Tie-2, or Ang-1 levels. The 90 days mortality rate was 7.1% (1/14) and 5.4% (6/111) in patients with and without AKI, respectively. Among the cohort, there was 1 patient developed end-stage kidney disease in 1 year period.
Table 1. Baseline characteristics of patients with acute decompensated heart failure.
In the study population, 99 of the patients presented baseline eGFR > 60 mL/min/1.73m2, whereas 26 patients (with CKD) presented eGFR <60 mL/min/1.73m2. The incidence of CKD among patients with AKI (5/14, 35.7%) was similar to that among patients without CKD (21/111, 18.9%; OR, 2.38; 95% CI 0.72–7.84; p = 0.166). Among patients with eGFR < 60 mL/min/1.73m2, we observed higher Ang-2 plasma levels (11,573 ± 9,441 pg/mL, n = 5 vs. 4,652 ± 7,415 pg/mL, n = 21; p = 0.021) and sTM plasma levels (11,641 ± 3,795 pg/mL, n = 5 vs. 7,032 ± 2,147 ng/mL, n = 2; p = 0.008) in patients with AKI than in patients without AKI (). Among patients with eGFR >60 mL/min/1.73m2, we also observed elevated Ang-2 plasma levels (5,883 ± 7,425 pg/mL, n = 9 vs. 2,951 ± 3,825 pg/mL, n = 90; p = 0.05) and sTM plasma levels (6,165 ± 2,874 pg/mL, n = 9 vs. 4,102 ± 1,323 pg/mL, n = 90; p = 0.0002) in AKI patients than in patients without AKI. This indicates that baseline eGFR levels were not associated with the difference between Ang-2 and sTM levels in patients with and without CRS.
Figure 1. Concentrations of angiopoietin-2 (A) and thrombomodulin (B) in patients with and without acute kidney injury (AKI). Open bars, patients without AKI; solid bars, patients with AKI. *p < 0.05 compared to patients without AKI in the subgroup in which the estimated glomerular filtration rate (eGFR) was <60 ml/minute/1.73 m2. Data are expressed as mean ± standard deviation.
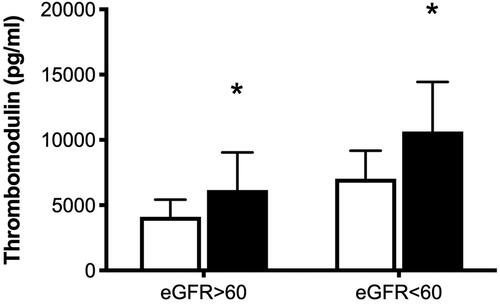
lists the ROC curves of Ang-2 and sTM used to predict development of CRS. AUROC revealed that Ang-2 and TM plasma levels had modest discriminative powers pertaining to the development of CRS (0.704, 95% CI 0.55–0.859, p = 0.013; and 0.789, 95% CI 0.675–0.903, p < 0.001, respectively). The cutoff values for Ang-2 and sTM in predicting AKI were 2,479 and 4,855.2 g/mL, respectively.
Table 2. Area under the receiver operating characteristic curves (AUROC) for day-1 plasma levels of angiopoietin-2 and thrombomodulin in predicting development of acute kidney injury.
lists the results of univariate and multivariate analysis of possible risk factors for AKI development in ADHF patients. Univariate regression analysis indicated that age, coronary heart disease, hypertension, MACE, LVEF, use of ACEI/ARB, levels of hemoglobin, BNP, sTM and Ang-2 were related to the development of AKI. After adjusting for the factors mentioned above using multivariate logistic regression analysis based on forward stepwise method, coronary heart disease and log sTM were selected for analysis. The log sTM was the only independent risk factor for AKI (OR, 13.83; 3.02–63.28, p = 0.001).
Table 3. Univariate and multivariate analysis of variables associated with acute kidney injury in heart failure patients.
shows the incidence of AKI stratified by quartiles of sTM plasma levels. The incidence of AKI was markedly higher in the 4th quartile (8/30, 26.7%; p = 0.001) than in the other three quartiles. By contrast, the incidence of AKI was lower in the 1st quartile (0/32, 0%) than in the other three quartiles.
Figure 2. Percentage of acute kidney injury (y-axis) among subjects with acute decompensated heart failure stratified by quartiles of plasma thrombomodulin.
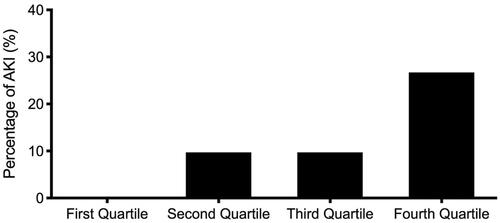
We also examined the correlations among sTM, Hb concentration, WBC count, hsCRP, and BNP levels. lists the correlations among serum mediators and these variables. We observed a correlation between sTM levels and the concentrations of Hb (r = −0.516; p < 0.001) and BNP (r = 0.434; p = 0.001). sTM plasma levels were higher in patients with MACE than in those without MACE (5,892.1 ± 3,495.9 vs. 4,754.7 ± 1,896.8 pg/mL, p = 0.026) (). In addition, there was a correlation between Ang-2 levels and eGFR (r = −0.270; p = 0.002).
Figure 3. Plasma levels of thrombomodulin were significantly lower in patients without major adverse cardiac events (4,754.7 ± 1,896.8 pg/ml) than in patients with multivessel disease (5,892.1 ± 3,495.9 pg/ml, p = 0.026).
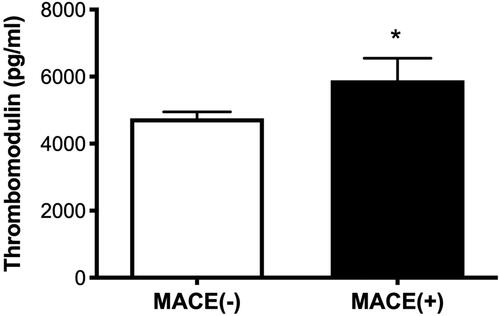
Table 4. Correlation between levels of thrombomodulin and other markers.
Discussion
The study showed that BNP, Ang-2, and sTM plasma levels were higher and Hb levels were lower in ADHF patients with AKI than in those without AKI. ROC curves revealed that day-1 Ang-2 and sTM levels had modest discriminative power in predicting the development of AKI. The difference between patients with and without CRS in terms of Ang-2 and sTM levels was unaffected by baseline renal function, as indicated by eGFR. Multivariate analysis identified sTM level was an independent factor associated with the development of AKI.
Our results revealed higher sTM plasma levels in ADHF patients with AKI [Citation22]. In previous studies, sTM levels were identified as a specific marker of endothelial injury [Citation11]. Our data suggest that endothelial injury may play a crucial role in mediating ADHF associated kidney injury. Heart failure associated altered hemodynamics, hypoxia, and inflammatory may be responsible for endothelial injury [Citation6]. The procoagulatory processes caused by injured endothelial cells may promote microthrombotic foci formation leading to organ microcirculation failures or complete organ failure [Citation23]. Elevated sTM levels have been reported in patients sepsis [Citation8] and acute myocardial infarction [Citation13] associated AKI. However, elevated sTM levels have never been reported in patients with CRS type I. Elevated sTM levels are related to several vascular-related diseases, including coronary disease, stroke, and peripheral occlusive arterial disease [Citation24]. MACE has also been associated with poor prognosis in patients with AMI [Citation16] or diabetes [Citation25], and endothelial injury is reportedly involved in the development of MACE [Citation26]. In the current study, we observed higher sTM levels in patients with MACE than in those without MACE, due perhaps to vascular atherosclerosis or endothelial injury of greater severity. As in one previous study [Citation27], we found that LVEF <40% was associated with AKI. A decrease in ejection fraction reflects reduced cardiac output and renal hypoperfusion leading to functional AKI and parenchymal damage [Citation28]. Endothelial injury is commonly observed in patients with CKD [Citation29]. However, we found that the incidence of CKD in patients with AKI was similar to that in patients without AKI. The difference between patients with and without CRS in terms of Ang-2 and sTM levels was unaffected by baseline renal function, as indicated by eGFR. These results reflect that endothelial activation/injury is implicated in the acute episode of kidney injury in patients with ADHF. In addition, the elevated Ang2 and sTM levels in patients with ADHF were not caused by cardiorenal syndrome type 3 or 4.
As in previous studies, we determined that decreased hemoglobin levels and increased BNP levels are associated with CRS. Under cardiac stretch and ischemia, BNP is secreted by cardiomyocytes. Evidence suggests that BNP plays an important role in cardiorenal protection [Citation30]. One recent study showed that ADHF patients with renal dysfunction had higher BNP levels than those with normal renal function [Citation31]. In patients with heart failure, endothelial dysfunction may be caused by the decreased shear stress due to pump failure [Citation32]. In addition to blood flow, shear stress was also affected by blood viscosity. Decreased blood viscosity due to a reduction in Hb concentration can result in depressed shear stress and subsequent ischemia-induced vasodilation [Citation33]. We therefore postulate that anemia (common in cases of CRS) is a contributor to decreased shear stress, leading to degraded endothelial function and contributing to degraded cardiac and renal function [Citation34]. This study also identified correlations among BNP, hemoglobin, and the markers of endothelial injury, as indicated by sTM plasma levels. These data provide evidence of a link between endothelial injuries induced by heart failure and subsequent kidney injury.
The results in this study demonstrate that sTM plasma levels early in the course of heart failure may be predictive of AKI development. The plasma levels of sTM could be used as a biomarker for the prediction of clinical outcomes or treatment decisions. The increased sTM plasma levels are associated with AKI development, which also suggests that endothelial injury may play a role in pathogenesis of type I CRS. Researchers are increasingly recognizing the clinical and biological heterogeneity of CRS. The pathophysiology of CRS represents the confluence of several hemodynamic, neuro-hormonal, inflammatory, and endothelial perturbations [Citation35]. Our previous study had disclosed that elevated Ang-2 and sTM plasma levels were associated with development of AKI in patients with AMI [Citation13]. In the present study, sTM was identified as an independent predictor for AKI in patients with heart failure. By identifying the markers of endothelial injury associated with AKI in different recruited subjects with various cardiovascular problems, these findings further emphasize the central role of endothelial injury in dysfunction of heart leading to kidney injury. Among mechanisms that link dysfunction of heart and kidney, endothelial injury seems to be one final common pathway. Incorporating novel biomarkers in combination with clinical predictors could greatly enhance the efficacy of diagnostic algorithms in predicting CRS [Citation35]. These data may enhance our understanding of dysregulated coagulation and endothelial dysfunction as a pathogenic mechanism in CRS. The impact of endothelial dysfunction in AKI development may also be an important target for future research in heart failure patients.
This is the first study to report on the potential role of endothelial biomarkers for the prediction of AKI in ADHF patients. Note however that this study has a number of limitations that should be considered in interpreting the results. This observational study included only a small number of patients recruited from a single medical facility, which prevented the use of multivariate analysis. It is highly likely that baseline characteristics, such as illness severity, are linked to endothelial dysregulation as well as the risk of developing AKI. Furthermore, we were unable to establish a causal association between the endothelial dysfunction and AKI development based solely on these results. It will be necessary in the future to conduct larger-scale studies with adjustments for potential confounding factors. Further studies should also be conducted to evaluate the efficacy of monitoring plasma sTM levels for the prevention of AKI in patients with ADHF.
Conclusions
In conclusion, this study revealed that higher sTM levels are associated with AKI in patients with ADHF. Subject to confirmation in large-scale prospective studies, our results suggest that ADHF patients with elevated sTM levels may require close monitoring of renal function or avoidance of nephrotoxic agents. Our current findings suggest a novel avenue by which to address kidney injury in the context of ADHF, wherein baseline biomarker profiles could potentially be used to identify individuals at risk of developing AKI.
Author contributions
All authors participated in the design, analysis, and interpretation of the data as well as in the review of the manuscript. CHC, SML, CHL, and ACCH conducted data collection; PHC and YCC provided conceptualization; and TYL and SML wrote the manuscript.
Acknowledgment
We would like to thank the Center for Big Data Analytics and Statistics, Chang Gung Memorial Hospital, Linkou, especially Shang-Hung Chang, M.D. and Yu-Tung Anton Huang, Ph.D. for their valuable help on the statistical consultation.
Disclosure statement
No potential conflict of interest was reported by the author(s).
Data availability statement
The datasets compiled in the current study are available from the corresponding author upon reasonable request.
Additional information
Funding
References
- Hillege HL, Nitsch D, Pfeffer MA, Candesartan in Heart Failure: assessment of Reduction in Mortality and Morbidity (CHARM) Investigators, et al. Candesartan in heart failure: assessment of reduction in M, morbidity I. Renal function as a predictor of outcome in a broad spectrum of patients with heart failure. Circulation. 2006;113(5):671–678.
- Smith GL, Lichtman JH, Bracken MB, et al. Renal impairment and outcomes in heart failure: systematic review and meta-analysis. J Am Coll Cardiol. 2006;47(10):1987–1996.
- Ronco C, Haapio M, House AA, et al. Cardiorenal syndrome. J Am Coll Cardiol. 2008;52(19):1527–1539.
- Fan PC, Chang CH, Chen YC. Biomarkers for acute cardiorenal syndrome. Nephrology. 2018;23(Suppl 4):68–71.
- Ronco C, McCullough P, Anker SD, Acute Dialysis Quality Initiative (ADQI) Consensus Group, et al. Acute dialysis quality initiative consensus g. Cardio-renal syndromes: report from the consensus conference of the acute dialysis quality initiative. Eur Heart J. 2010;31(6):703–711.
- Zhang J, Bottiglieri T, McCullough PA. The Central role of endothelial dysfunction in cardiorenal syndrome. Cardiorenal Med. 2017;7(2):104–117.
- Cohen L. The cardiorenal syndrome: pathophysiologic crosstalk, outcomes, and treatment targets. Cardiovasc Hematol Disord Drug Targets. 2014;14(3):170–176.
- Lin SM, Wang YM, Lin HC, et al. Serum thrombomodulin level relates to the clinical course of disseminated intravascular coagulation, multiorgan dysfunction syndrome, and mortality in patients with sepsis. Crit Care Med. 2008;36:683–689.
- Lemieux C, Maliba R, Favier J, et al. Angiopoietins can directly activate endothelial cells and neutrophils to promote proinflammatory responses. Blood. 2005;105(4):1523–1530.
- Takano S, Kimura S, Ohdama S, et al. Plasma thrombomodulin in health and diseases. Blood. 1990;76(10):2024–2029.
- Boehme MW, Galle P, Stremmel W. Kinetics of thrombomodulin release and endothelial cell injury by neutrophil-derived proteases and oxygen radicals. Immunology. 2002;107(3):340–349.
- Chong AY, Freestone B, Patel J, et al. Endothelial activation, dysfunction, and damage in congestive heart failure and the relation to brain natriuretic peptide and outcomes. Am J Cardiol. 2006;97(5):671–675.
- Liu KL, Lee KT, Chang CH, et al. Elevated plasma thrombomodulin and angiopoietin-2 predict the development of acute kidney injury in patients with acute myocardial infarction. Crit Care. 2014;18(3):R100.
- van Meurs M, Kumpers P, Ligtenberg JJ, et al. Bench-to-bedside review: angiopoietin signalling in critical illness – a future target? Crit Care. 2009;13(2):207.
- Fiedler U, Reiss Y, Scharpfenecker M, et al. Angiopoietin-2 sensitizes endothelial cells to TNF-alpha and has a crucial role in the induction of inflammation. Nat Med. 2006;12(2):235–239.
- Lin SM, Chung FT, Kuo CH, et al. Circulating angiopopietin-1 correlates with the clinical course of multiple organ dysfunction syndrome and mortality in patients with severe sepsis. Medicine. 2015;94(20):e878.
- Poss J, Ukena C, Kindermann I, et al. Angiopoietin-2 and outcome in patients with acute decompensated heart failure. Clin Res Cardiol. 2015;104(5):380–387.
- Kumpers P, Hafer C, David S, et al. Angiopoietin-2 in patients requiring renal replacement therapy in the ICU: relation to acute kidney injury, multiple organ dysfunction syndrome and outcome. Intensive Care Med. 2010;36(3):462–470.
- Nieminen MS, Bohm M, Cowie MR, Guideline ESCCfP, et al. Executive summary of the guidelines on the diagnosis and treatment of acute heart failure: the task force on acute heart failure of the European Society of Cardiology. Eur Heart J. 2005;26:384–416.
- Levey AS, Stevens LA, Schmid CH, CKD-EPI (Chronic Kidney Disease Epidemiology Collaboration), et al. A new equation to estimate glomerular filtration rate. Ann Intern Med. 2009;150(9):604–612.
- Palevsky PM, Liu KD, Brophy PD, et al. KDOQI US commentary on the 2012 KDIGO clinical practice guideline for acute kidney injury. Am J Kidney Dis. 2013;61(5):649–672.
- Lin SM, Cch Lin TY, Huang ACC, et al. The role of endothelial dysfunction in development of acute kidney injury in patients with acute decompensated heart failure. Research Square (Preprint); 2020 October 19 [cited 2022 Aug 20]. Available from:
- Levi M, Ten Cate H. Disseminated intravascular coagulation. N Engl J Med. 1999;341(8):586–592.
- Constans J, Conri C. Circulating markers of endothelial function in cardiovascular disease. Clin Chim Acta. 2006;368(1-2):33–47.
- Koo BK, Chung WY, Moon MK. Peripheral arterial endothelial dysfunction predicts future cardiovascular events in diabetic patients with albuminuria: a prospective cohort study. Cardiovasc Diabetol. 2020;19(1):82.
- Jaski BE, Cohen JD, Trausch J, et al. Outcome of urgent percutaneous transluminal coronary angioplasty in acute myocardial infarction: comparison of single-vessel versus multivessel coronary artery disease. Am Heart J. 1992;124(6):1427–1433.
- Matějka J, Varvařovský I, Rozsíval V, et al. Heart failure is the strongest predictor of acute kidney injury in patients undergoing primary percutaneous coronary intervention for ST-elevation myocardial infarction. Kardiol Pol. 2016;74(1):18–24.
- Chen YT, Jenq CC, Hsu CK, et al. Acute kidney disease and acute kidney injury biomarkers in coronary care unit patients. BMC Nephrol. 2020;21(1):207.
- Yilmaz MI, Saglam M, Caglar K, et al. The determinants of endothelial dysfunction in CKD: oxidative stress and asymmetric dimethylarginine. Am J Kidney Dis. 2006;47(1):42–50.
- McCullough PA, Nowak RM, McCord J, et al. B-type natriuretic peptide and clinical judgment in emergency diagnosis of heart failure: analysis from breathing not properly (BNP) multinational study. Circulation. 2002;106(4):416–422.
- Dos Reis D, Fraticelli L, Bassand A, et al. Impact of renal dysfunction on the management and outcome of acute heart failure: results from the French prospective, multicentre, DeFSSICA survey. BMJ Open. 2019;9(1):e022776.
- Ferrari R, Bachetti T, Agnoletti L, et al. Endothelial function and dysfunction in heart failure. Eur Heart J. 1998;19(Suppl G):G41–7.
- Giannattasio C, Piperno A, Failla M, et al. Effects of hematocrit changes on flow-mediated and metabolic vasodilation in humans. Hypertension. 2002;40(1):74–77.
- Hatamizadeh P, Fonarow GC, Budoff MJ, et al. Cardiorenal syndrome: pathophysiology and potential targets for clinical management. Nat Rev Nephrol. 2013;9(2):99–111.
- Rangaswami J, Mathew RO. Pathophysiological mechanisms in cardiorenal syndrome. Adv Chronic Kidney Dis. 2018;25(5):400–407.