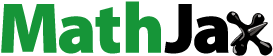
Abstract
Background
Occupational-related cancers are a substantial global health issue. The largest proportion of occupational-related cancers is tracheal, bronchus, and lung (TBL) cancer. This study aimed to explore the geographical and temporal trends in occupational carcinogens related to TBL cancer.
Methods
Data on TBL cancer attributable to occupational carcinogens were collected from the Global Burden of Disease Study 2019. Numbers and age-standardized rates (ASRs) of deaths, disability-adjusted life years (DALYs), and corresponding average annual percentage change (AAPC) were evaluated and stratified by geographic location, socio-demographic index (SDI) quintiles, age, and sex.
Results
Globally, ASRs of deaths and DALYs in TBL cancer attributable to occupational carcinogens showed a downward trend (AAPC = − 0.69%, − 1.01%) while increases were observed in the low, low-middle, and middle SDI quintiles. Although males accounted for 82.4% and 81.5% of deaths and DALYs in 2019, respectively, it showed an upward trend of ASRs in females (AAPC = 0.33%, 0.02%). Occupational exposure to asbestos, silica and diesel engine exhaust were the top three causes of age-standardized TBL cancer deaths and DALYs. Over the past three decades, the percentage of age-standardized TBL cancer deaths and DALYs attributable to occupational asbestos and silica exposure decreased by 18.24, 6.71 and 20.52%, 4.00% globally, but increased significantly in lower SDI regions, while the burden attributable to occupational diesel engine exhaust exposure increased by 32.76, 37.23% worldwide.
Conclusions
Occupational exposure remains an important risk factor for TBL cancer. The burden of TBL cancer attributable to occupational carcinogens showed obvious heterogeneity which decreased in higher SDI but increased in lower SDI regions. The burden of males was significantly higher than females, but the females showed an increasing trend. Occupational exposure to asbestos was the main causes of the burden. Therefore, effective prevention and control measures tailored to local conditions are necessary.
1. Introduction
Tracheal, bronchus, and lung (TBL) cancer is the leading cause of morbidity and mortality worldwide with 2.094 million new cases and 1.8 million deaths annually [Citation1]. TBL cancer is associated with genetic variation, smoking, environmental factors and occupational exposures. Previous studies have shown that up to 25% of all TBL cancer deaths were attributable to causes of occupational exposure [Citation2]. In addition, the International Labour Organization estimates that of the world’s 2.5 billion workers, 2 million die each year from occupational accidents or diseases, and a third of these diseases are respiratory cancers and interstitial lung diseases [Citation3]. At the same time, occupational exposure is the cause of cancer deaths and disability-adjusted life years worldwide, of which lung cancer is the primary outcome of most carcinogens and accounts for the largest proportion of the burden [Citation4]. Therefore, occupation-related TBL cancer is unquestionably a major global health problem.
With the changes of social and economic development, occupational exposure has undergone complex and profound changes, which is closely related to SDI value. Some studies showed that the total exposure increased in areas with SDI values ranging from 0.2 to 0.6, while the total exposure decreased in areas with SDI values ranging from 0.6 to 0.8 [Citation5]. However, there is still specificity in the variation trend of occupational risk exposure in different classifications. At the same time, the attributable cancer burden varies greatly between each occupational exposure, with significant regional heterogeneity. For example, silica was the leading occupational carcinogens only in East Asia, while asbestos was the leading occupational carcinogens in other 20 regions in 2017 [Citation6,Citation7]. These complex regional risk exposure differences and variations pose great challenges for the global public health prevention and control of TBL cancer attributable to occupational carcinogens, which is important events in need of improvement urgently.
At present, there were few analyses on the burden of TBL cancer attributable to occupational carcinogens and its epidemic trend, only a few regions or exposure factors had been studied, which is a lack of systematicity [Citation8,Citation9]. In this study, data was collected from the Global Burden of Disease (GBD) 2019 study to systematically estimate the degree of exposure to occupational carcinogens and quantify the deaths, DALYs burden, and trends in TBL cancer attributable to occupational carcinogens by geographic location, socio-demographic index (SDI) level, age, sex, and its change over time, the age-period-cohort model is also used to analyze the effects of age, period and cohort in different SDI regions, which will be a helpful guide for a strategy on TBL cancer attributable to occupational carcinogens prevention and control.
2. Methods
2.1. Data sources
Data for this study were derived from the Global Health Data Exchange Online Query Tool (GHDx) (http://ghdx.healthdata.org/gbd-results-tool), an ongoing global collaboration that used epidemiological data to compare health losses and 87 risk factors from 369 diseases and injuries between 1990 and 2019. Data were presented as values with a 95% uncertainty interval (UI), and 95% UIs were estimated by generating 1000 draws in each computational step and taking the 25th and 975th ordered values of the draws.
Data sources for GBD 2019 include several pooled cancer registries, including Cancer Incidence in five Continents (CI5), EUREG, US SEER Database, and NORDCAN [Citation10].
Our research meets the Accurate and transparent Health Assessment Reporting Guidelines (GATHER) statements. The research design and framework was shown in Supplementary Figure 1.
2.2. Definition
2.2.1. Tracheal, bronchus, and lung (TBL) cancer and occupational carcinogen factors
TBL cancer is defined based on the International Classification of Diseases, Tenth Revision (ICD-10) codes C33, C34–C34.92, Z12.2, Z80.1–Z80.2, and Z85.1–Z85.20 [Citation11].
Based on the GBD 2019 study, there were nine occupational carcinogen factors related to TBL cancer: occupational exposure to arsenic, occupational exposure to asbestos, occupational exposure to beryllium, occupational exposure to cadmium, occupational exposure to chromium, occupational exposure to diesel engine exhaust, occupational exposure to nickel, occupational exposure to polycyclic aromatic hydrocarbons, and occupational exposure to silica.
For a given risk–outcome pair, we estimated TBL cancer deaths and DALYs attributable to occupational carcinogens as total deaths and DALYs for the outcome multiplied by the population attributable fraction (PAF) for the risk–outcome pair for each age, sex, location, and year [Citation12, p.Citation5].
where RR(x) represents the relative risk corresponding to level x exposure and P(x) represents the proportion of persons at level x exposure in the relevant population.
2.2.2. Socio-demographic index (SDI) quintiles
SDI is a comprehensive measure of development, which is constructed from measures of per capita income, average years of education, and total fertility rates. SDI scores range from 0 (highest fertility, lowest income, and lowest educational level) to 1 (highest educational level and lowest fertility). Each GBD location has an annual SDI score, and based on SDI values in 2019, countries and territories were divided into 5 SDI quintiles (high, high-middle, middle, low-middle, and low).
2.2.3. Summary exposure values(SEVs)
The GBD 2019 summarized exposure distributions for dichotomous, polytomous, and continuous risk factors using the SEV [Citation13]. The SEV compares the distribution of excess risk times exposure level to a population where everyone is at maximum risk.
For a given risk r and outcome c pair where RRmax is the relative risk at the 99th percentile of the global distribution of exposure. We then averaged across outcomes to compute the SEV for a given risk as
where N (c) is the total number of outcomes for a risk.
A population’s risk-weighted exposure or risk-weighted prevalence of a single exposure was used to estimate the exposure value of the occupational carcinogens involved which ranges from 0 to 100%, which is 0 when the population is at no additional risk and 100 when the population is at the highest risk level and is defined as summary exposure values (SEV). A decrease in SEV indicates a decrease in exposure to a particular risk factor, while an increase in SEV indicates an increase in exposure.
2.2.4. Age-standardized rate and disability-adjusted life-years (DALYs)
Disability-adjusted life year (DALY) is a comprehensive indicator of health loss caused by lethal and non-lethal results. DALY is calculated as the sum of years lived with disability (YLDs) and years of life lost (YLLs). Losing a year’s health is equivalent to a DALY. The gap between the current health status and the overall health status is reflected in the sum of all DALY in all populations.
The age standardized rate (ASR) is a measure of changes in disease patterns in the distribution of a population. ASR is very useful for comparing different groups of people. All rates are reported per 100,000 population in a given year, and point estimates are presented with 95% uncertainty intervals (UIs). The specific calculation method of ASR is the same as that reported in previous studies [Citation14].
2.3. Statistical analysis
2.3.1. Analysis of temporal trends in SEV and ASRs
EXCEL software plotted the trends in SEVs and ASRs of occupational carcinogen factors related to TBL cancer over time.
The average annual percentage change (AAPC) and their 95% confidence interval (CI) was calculated to quantify the temporal trends of SEVs and ASRs from 1990 to 2019. The SEVs and ASRs were considered to be increasing when the estimated value and lower 95% CI of the AAPC >0. The SEVs and ASRs were considered to be decreasing when the estimated value and upper 95% CI of the AAPC <0. The Joinpoint regression analysis was performed by the Joinpoint Regression program (version 4.2.0.1). We select three ‘joinpoints’ to connect different line segments to describe the trend, which the linear slope of a trend changes in a statistically significant way over time. And Joinpoint selects the final model using Permutation Test, the overall significance level was 0.05 [Citation15].
2.3.2. Correlation analysis of ASRs and SDI values
We analysed the association between the SDI values and ASRs of death and DALYs was investigated in different SDI regions and GBD regions and analyses were performed using the R program, version 3.6.2. A 2-tailed p value <0.05 was considered statistically significant.
2.3.3. Age-period-cohort modelling analysis of ASRs
We used the age-period-cohort model to analyse the age, period, and cohort effects of TBL cancer attributable to occupational carcinogens. In the model, we converted the collected data into successive 5-year age groups and consecutive 5-year periods. The GBD datasets couldn’t provide the deaths and DALYs data of successive 5-year age groups for those over 85 years old, and the data under the age of 24 are equal to 0 in TBL cancer attributable to occupational carcinogens. Then we recorded into successive 5-year age groups for those aged from 25–29 years to 80–84 years and one group for those aged over 85 years and consecutive 5-year periods (1990–1994 to 2015–2019). A general linear model has been used to analyse the slope of the period/cohort RRs. The analysis was performed by the Age-Period-Cohort Web tool (https://analysistools.cancer.gov/apc/). The significance of annual percentage trends was tested using Wald’s chi–square test. Statistical tests were two-sided and p < 0.05 is considered significant [Citation16].
3. Results
3.1. SEV trends of occupational carcinogens
The age-standardized SEV rate of occupational carcinogen-associated TBL cancer increased globally (AAPC = 0.47%, 95% CI: 0.46–0.47%), from 0.97% (95% CI: 0.72–1.48%) in 1990 to 1.11% (95% CI: 0.85–1.67%) in 2019 per 100,000 persons. The age-standardized SEV rate of occupational carcinogens related to TBL cancer also showed an upward trend to varying degrees at the five SDI quintiles level (). The age-standardized SEV rate of occupational carcinogens risks related to TBL cancer increased more in females than in males at the GBD regional level, except for Southern Sub-Saharan Africa, which showed a downward trend (). The highest age-standardized SEV rate (1.38 in 1990 and 1.65 in 2019) has always been in Andean Latin America, followed by Southern Latin America, and then Central Latin America ().
Table 1. Age-standardized SEV rates of occupational carcinogens risks related to TBL cancer and average annual percentage change.
Trends of age-standardized SEV rates of nine occupational carcinogens are provided at a global level based on sex from 1990 to 2019 (). The age-standardized SEV rates from 1990 to 2019 in all populations all showed an upward trend, especially occupational exposure to diesel engine exhaust except occupational exposure to asbestos and nickel, which showed a downward trend. The age-standardized SEV rate of increase in polycyclic aromatic hydrocarbons exposure is significantly faster in women than in men.
3.2. Spatial and temporal TBL cancer burden attributable to occupational carcinogens
3.2.1. Global burden of occupational carcinogens-related TBL cancer
A total of 289,793 deaths (95% CI, 221524–359238) and 5,948,985 DALYs (95% CI, 4520238–7484430) associated with TBL cancer burden attributable to occupational carcinogens were identified globally in 2019 (). Deaths and DALYs of TBL cancer due to occupational carcinogens from 1990–2019 increased by 70.63 and 53.73%, respectively. The high SDI quintile always had the highest number of death cases, and DALYs of TBL cancer were attributable to occupational carcinogens, while the low SDI quintile always had the lowest ().
Table 2. The death, DALYs, ASRs and their change trends of TBL cancer attributable to occupational carcinogens from 1990 to 2019.
Globally, the age-standardized deaths rate (ASDR) (AAPC= −0.69%, 95% CI, −0.72 to −0.67%) and age-standardized DALYs rate (AAPC= −1.01%, 95% CI, −1.03 to −0.99%) showed a downward trend (). However, different SDI quintiles show different trend patterns of ASDR and age-standardized DALYs rate in the past 30 years (, ). Increases in ASDR and age-standardized DALYs rate of occupational carcinogen-associated TBL cancer were observed in the low (ASDR AAPC= 0.75%, 95% CI, 0.73–0.77%; age-standardized DALYs rate AAPC= 0.61%, 95% CI, 0.59–0.62%), low-middle (ASDR AAPC = 1.48%, 95% CI, 1.45–1.5%; age-standardized DALYs rate AAPC= 1.17%, 95% CI, 1.15–1.19%), and middle (ASDR AAPC= 1.04%, 95% CI, 0.99–1.1%; age-standardized DALYs rate AAPC = 0.55%, 95% CI, 0.5–0.59%) SDI quintiles. ASDR and age-standardized DALYs rate presented a continuous downward trend in the high SDI quintile (). However, ASDR and age-standardized DALYs rate presents a continuous upward trend in the low-middle and low SDI quintiles (,L)). ASDR has fluctuated significantly in the past 30 years and has shown a trend of ‘rising-falling-rising-falling’ pattern (1990–1994, APC = 2.29%; 1994–1998, APC = −2.18%; 1998–2011, APC = 0.25%; 2011–2019, APC = −1.85%) in the high-middle SDI quintile (). ASDR of the middle SDI quintile and age-standardized DALYs rate of high-middle and middle SDI quintiles show a trend of ‘rising-falling’ pattern ().
Figure 2. The ASDR and age-standardized DALYs rate trends of TBL cancer from 1990 to 2019 in global and 5 SDI categories regions. (A) APPC of ASDR in global; (B) APPC of ASDR in high SDI regions; (C) APPC of ASDR in high-middle SDI regions; (D) APPC of ASDR in middle SDI regions; (E) APPC of ASDR in low-middle SDI regions; (F) APPC of ASDR in low SDI regions; (G) APPC of age-standardized DALYs rate in global; (H) APPC of age-standardized DALYs rate in high SDI regions; (I) APPC of age-standardized DALYs rate in high-middle SDI regions; (J) APPC of age-standardized DALYs rate in middle SDI regions; (K) APPC of age-standardized DALYs rate in low-middle SDI regions; (L) APPC of age-standardized DALYs rate in low SDI regions.
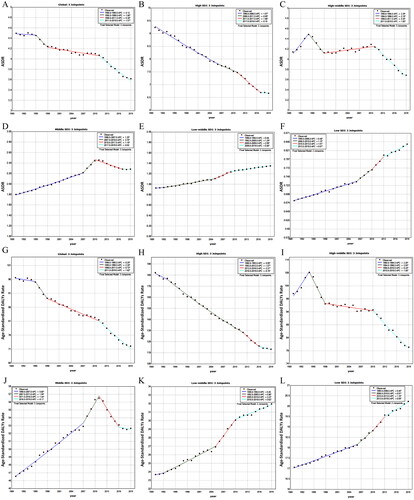
3.2.2. Regional burden of occupational carcinogens-related TBL cancer
Western Europe, high-income North America, and East Asia always had the top three greatest deaths burden of TBL cancer attributable to occupational carcinogens in both genders. The region with the highest DALYs changed from Western Europe in 1990 to East Asia in 2019. Oceania, Central Sub-Saharan Africa, and Andean Latin America always had the top three lowest deaths and DALYs burden of TBL cancer attributable to occupational carcinogens in both genders ().
Australasia had the highest ASDR and age-standardized DALYs rate in 1990 while Western Europe had the highest ASDR and age-standardized DALYs rate in 2019 (). ASDR increased the fastest in Central Europe (AAPC = 1.53%, 95% CI: 1.47–1.60%) and age-standardized DALYs rate increased the fastest in Oceania (AAPC = 1.23%, 95% CI: 1.2–1.27%) from 1990 to 2019. The ASDR decreased most in Andean Latin America (AAPC = −2.02%, 95% CI: −2.15 to −1.90%), but age-standardized DALYs rate decreased most in high-income North America (AAPC = −2.36%, 95% CI: −2.42 to −2.3%) ().
3.2.3. National burden of occupational carcinogens-related TBL cancer
Greenland and Monaco had the highest, while Nigeria and Mali had the lowest ASDR and age-standardized DALYs rate of occupational carcinogen-related TBL cancer in 2019 among 204 countries and territories ().
Figure 3. The spatial distribution of ASDR and age-standardized DALYs rate in TBL cancer attributable to occupational carcinogens in 2019, and their AAPC among 204 countries and territories. (A) ASDR in 2019; (B) Age-standardized DALYs rate in 2019; (C)AAPC of ASDR; (D) AAPC of age-standardized DALYs rate.
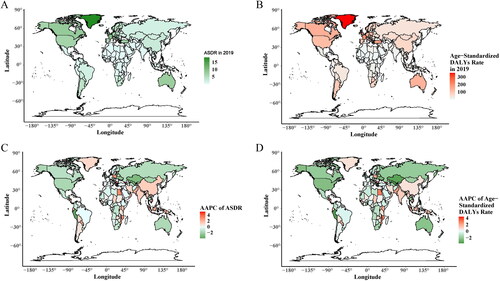
The ASDR and age-standardized DALYs rates show different trends among countries and territories from 1990–2019 (). The ASDR and age-standardized DALYs rates decreased in 97 and 113 countries and territories respectively. The highest increase of ASDR occurred in Georgia (AAPC = 5.2%), Croatia (AAPC = 4.05%), and Honduras (AAPC = 3.74%) while the highest decrease occurred in Peru (AAPC = 3.86%), Uzbekistan (AAPC = 3.22%), and Kazakhstan (AAPC = 3.05%). Georgia, Croatia, and Honduras have the highest increase (AAPC) in age-standardized DALYs rate, 4.38, 3.44, and 3.25%, respectively. The highest decrease occurred in Uzbekistan (AAPC = 3.7%), Peru (AAPC = 3.65%), and Singapore (AAPC = 3.44%) (Supplementary Table 1).
3.3. Global TBL cancer burden attributable to occupational carcinogens by age and gender
The cases of deaths and DALYs in the 20–39 age group first rose, then fell, and then rose again in both males and females in the past 30 years (1990–2019). However, in the 60–79 and 80 plus age groups, the number of deaths and DALYs showed continuous upward trend (Supplementary Table 2). The 60–79 age group is the main TBL cancer burden population whose deaths and DALYs proportions are significantly higher than other age groups in all regions (, Supplementary Table 2). The deaths proportions in the <60 years of age are significantly higher in low, low-middle, and middle SDI quintiles than in high and high-middle SDI quintiles ().
Figure 4. Contribution of different ages in deaths and DALYs of TBL cancer attributable to occupational carcinogens by sex among regions in 2019.
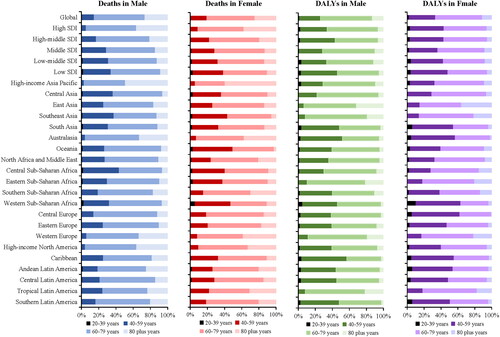
Males are the main TBL cancer burden population in 2019, accounting for 82.4 and 81.5% of TBL cancer deaths and DALYs due to occupational carcinogens, respectively (). However, there is an upward ASRs trend among females (ASDR AAPC = 0.33%, 95% CI, 0.29–0.36%; age- standardized DALYs rate AAPC = 0.02%, 95% CI, −0.01–0.04%) ().
In addition, a sex-related difference in correlation between ASRs and SDI was observed (, ). ASDR decreased in the male population in the high and high-middle SDI quintiles and increased in the female population in both five SDI quintiles from 1990 to 2019 (, ). Age-standardized DALYs rate increased in the female population of high-middle, middle, low-middle, and low SDI quintiles and decreased in the male population of high and high-middle SDI quintiles (, ). The ratio trends of male to female in ASDR and age-standardized DALYs rate of TBL cancer attributable to occupational carcinogens were downward in high, high-middle, and low SDI quintiles but were upward in the middle and low-middle SDI quintiles from 1990–2019 (). Positive correlations were found between ASDR and SDI in both, male and female genders ()). In the male gender, age-standardized DALYs rate and SDI were positively related when SDI was low; and when SDI was high, it reversed (). However, in females, age-standardized DALYs rate and SDI were positively related ().
Figure 5. The ASRs of occupational carcinogens attributable TBL cancer among 21 regions based on SDI from 1990 to 2019. The vertical axis is the age-standardized deaths and DALYs rate, and the horizontal axis is the SDI value. Each combination of colors and shapes represents a region. Each point represents the age-standardized death and DALYs rate that year in each region. Each combination of the same color and shape, from front to back, represents the data for each year from 1990 to 2019. (A) ASDR for both genders; (B) age-standardized DALYs rate for both genders; (C) ASDR for male; (D) age-standardized DALYs rate for male; (E) ASDR for female; and (F) age-standardized DALYs rate for female.
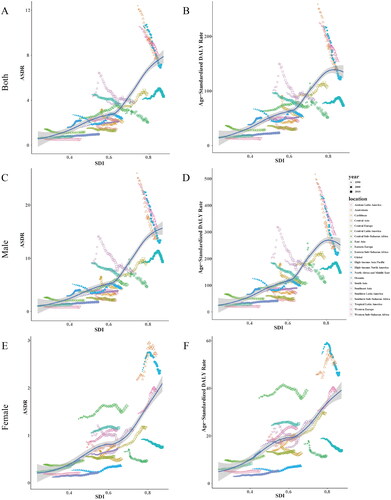
Figure 6. The ratio trends of male to female in ASDR and age-standardized DALYs rate of TBL cancer attributable to occupational carcinogens from 1990 to 2019. (A) The trends of male in ASDR of TBL cancer attributable to occupational carcinogens from 1990 to 2019; (B) The trends of female in ASDR of TBL cancer attributable to occupational carcinogens from 1990 to 2019; (C) The ratio trends of male to female in ASDR of TBL cancer attributable to occupational carcinogens from 1990 to 2019; (D) The trends of male in age-standardized DALYs rate of TBL cancer attributable to occupational carcinogens from 1990 to 2019; (E) The trends of female in age-standardized DALYs rate of TBL cancer attributable to occupational carcinogens from 1990 to 2019; (F) The ratio trends of male to female in age-standardized DALYs rate of TBL cancer attributable to occupational carcinogens from 1990 to 2019.
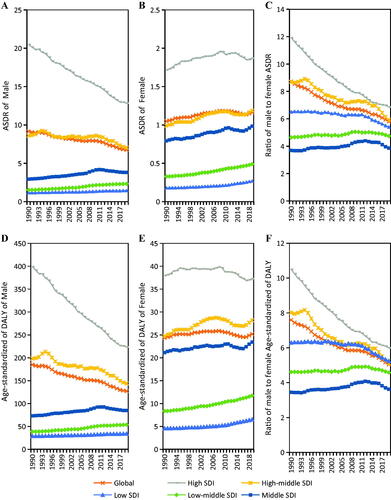
3.4. The age-period-cohort analysis of the mortality and DALYs rate of TBL cancer attributable to occupational carcinogens from 1990 to 2019
and show the detailed results of the age-period-cohort analysis of the mortality and DALYs rate of TBL cancer attributable to occupational carcinogens from 1990 to 2019. The age, period, and cohort effects were statistically significant.
Figure 7. The age-period-cohort analysis of the mortality rate of TBL cancer attributable to occupational carcinogens from 1990 to 2019. (A) The age effect; (B) The period effect; (C) The cohort effect.
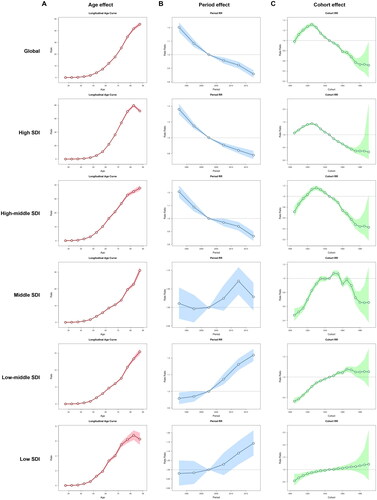
Figure 8. The age-period-cohort analysis of the DALYs rate of TBL cancer attributable to occupational carcinogens from 1990 to 2019. (A) The age effect; (B) The period effect; (C) The cohort effect.
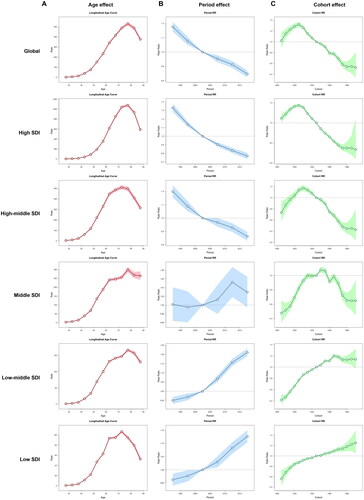
The overall net drift value of mortality rate attributable to occupational carcinogens per year was −1.253% (95% CI, −1.43% to −1.075%) in global, −2.151% (95% CI, −2.57% to −1.731%) in high SDI, −1.219% (95% CI, −1.448% to −0.989%) in high-middle SDI, 0.188% (95% CI, 0.008% to 0.367%) in middle SDI, 1.039% (95% CI, 0.894% to 1.184%) in low-middle SDI and 0.634% (95% CI, 0.355% to 0.914%) in low SDI regions. For the same birth cohort, the mortality rate attributable to occupational carcinogens increased with age in all SDI regions (). The period RRs attributable to occupational carcinogens trended downwards in high and high-middle SDI regions, while trending upwards in middle, low-middle, and low SDI regions (). The cohort RRs attributable to occupational carcinogens first increased and then decreased in high, high-middle, and middle SDI regions. While in low-middle and low SDI regions, the cohort RRs gradually increased among all the born cohorts (). Similar trends were observed in the DALYs rate ().
3.5. Occupational carcinogens
Occupational asbestos exposure (1990, DALYs = 2410020.29; 2019, DALYs = 3365428.55) was consistently responsible for the largest DALYs of TBL cancer attributable to occupational carcinogens, followed by occupational exposure to silica (1990, DALYs = 923859.91; 2019, DALYs = 1477739.93) and then occupational exposure to diesel engine exhaust (1990, DALYs = 247150.69; 2019, DALYs = 563382.73) from 1990–2019 (Supplementary Figure 2). TBL cancer-related ASRs of occupational carcinogens showed a stable trend except for occupational exposure to asbestos in males and occupational exposure to diesel engine exhaust in females in the past 30 years, as shown in . TBL cancer-related ASRs of occupational asbestos exposure showed a downward trend in males while a slight upward trend in females (Supplementary Figure 2, ).
Figure 9. The ASDR and age-standardized DALYs rate trends of TBL cancer attributable to different occupational carcinogens by genders from 1990 to 2019. (A) ASDR trends in both genders; (B) ASDR trends in male; (C) ASDR trends in female; (D) Age-standardized DALYs rate trends in both genders; (E) Age-standardized DALYs rate trends in male; (F) Age-standardized DALYs rate trends in female.
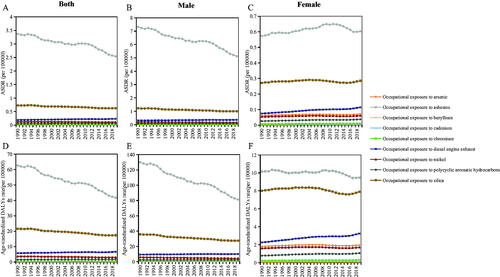
The leading risks for ASDR and age-standardized DALYs rate of TBL cancer attributable to occupational carcinogens varied in different age groups. Occupational exposure to silica was the leading risk in the 20–39 and 40–59 age groups, while occupational asbestos exposure was the leading risk in the 60–79 and 80-plus age groups (Supplementary Table 3).
Occupational asbestos exposure accounted for the highest age-standardized deaths and DALYs percentages of TBL cancer attributable to occupational carcinogens especially in Australasia, Western Europe, and high-income North America, as shown in . In addition, Australasia, North Africa and Middle East, Central Sub-Saharan Africa, Western Sub-Saharan Africa, Western Europe, high-income North America, Caribbean, and Andean Latin America showed a downward trend while other regions have shown an upward trend for age-standardized deaths and DALYs percentages of TBL cancer attributable to occupational exposure to asbestos from 1990 to 2019. The decline of age-standardized death and DALYs percentages was the largest among all occupational carcinogens except for occupational asbestos exposure in Southern Sub-Saharan Africa. In Eastern Sub-Saharan Africa, the TBL cancer age-standardized deaths and DALYs percentages proportion attributed to occupational exposure to diesel engine exhaust had a significant increase, ranked first globally.
Figure 10. Age-standardized deaths and DALYs percentages of TBL cancer attributable to occupational carcinogens among global, 5 SDI quintiles and 21 GBD regions in 1990 and 2019 and their changes from 1990 to 2019. (A) Age-standardized deaths percentages; (B) Age-standardized DALYs percentages.
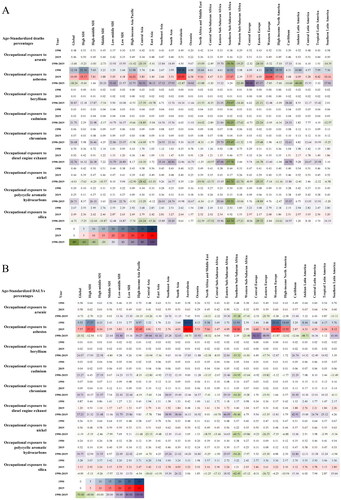
Attributable cancer deaths and DALYs were listed for each occupational carcinogen globally, five SDI quintiles, and 204 countries and territories in 2019 (Supplementary Table 4). The United States of America, China, and Japan were the top three countries with the highest attributable TBL cancer deaths and DALYs for occupational exposure to asbestos in 2019. China, India, and Indonesia were the top three countries with the highest attributable TBL cancer deaths and DALYs for occupational exposure to beryllium, cadmium, chromium, diesel engine exhaust, and polycyclic aromatic hydrocarbons in 2019. China, the United States of America, and India were the top three countries with the highest attributable TBL cancer deaths and DALYs for occupational exposure to arsenic, silica, and nickel in 2019 (Supplementary Table 4).
4. Discussion
4.1. Overview
Our research comprehensively investigated the latest data on occupational carcinogens related to the TBL cancer burden. The ASDR and age-standardized DALYs rate globally showed a downward trend in all populations while deaths and DALYs of TBL cancer due to occupational carcinogens increased by 70.63 and 53.73% from 1990 to 2019. Concerning trends were observed in certain populations and geographical regions in the past 30 years despite considerable progress on decreasing ASDR and age-standardized DALY rate of occupational carcinogens related to TBL cancer at the global level. Increases in ASDR and age-standardized DALYs rate of occupational carcinogen-associated TBL cancer were observed in the low, low-middle, and middle SDI quintiles. The 60–79 age group is the main occupational carcinogen-associated TBL cancer burden population in all regions while the deaths proportions of those <60 years old are significantly higher in the low, low-middle, and middle SDI quintiles than in the high and high-middle SDI quintiles. Males are the main occupational carcinogen-associated TBL cancer burden population while an upward trend of ASRs among female individuals cannot be ignored.
Occupational exposure to asbestos was the leading specific risk factor of the occupational carcinogen-associated TBL cancer. The burden was still quite heavy, although the age-standardized deaths and DALYs percentages of TBL cancer attributable to occupational exposure to asbestos showed a downward trend in the high SDI quintile. It should be noted that the age-standardized SEV rate of occupational exposure to diesel engine exhaust increased for both sexes combined, with an even larger increase among females. Furthermore, the ASRs of TBL cancer attributable to occupational exposure to diesel engine exhaust rose significantly in females over the past decade.
These trends of TBL cancer burden related to occupational carcinogens vary among certain populations and geographical regions and underline the need for optimal control programs and regulations to reduce the burden of TBL cancer attributable to occupational carcinogens.
4.2. Information from the differences in TBL cancer burden related to occupational carcinogens among different SDI regions
Trend analyses found that visible socioeconomic disparity persisted in the global burden of TBL cancer caused by occupational carcinogens. Socioeconomic inequality is one of the main reasons for this disparity [Citation17–21]. Our study showed that although the high SDI quintile has a downward ASDR trend and age-standardized DALYs rate, the ASDR and age-standardized DALYs rate of TBL cancer attributable to occupational carcinogens was nearly 6-8 times higher than in the low SDI quintile. This information reflects the industrialization and cumulative occupational exposures that occurred decades ago in the high SDI quintile. The results of the age-period-cohort analysis of the mortality and DALYs rate of TBL cancer attributable to occupational carcinogens from 1990 to 2019 showed significant differences among different SDI regions. Areas with low, low-middle and middle SDI regions have a higher relative risk of death after 2005, while high and high-middle SDI regions have a lower relative risk of death after 2005.
With the acceleration of international economic integration, the free-flowing production factors of commodities have shifted high-polluting industries to less developed regions, which has also been accompanied by a shift of TBL cancer-related occupational carcinogens to low-income countries [Citation22,Citation23]. Policymakers are so focused on the economic benefits of the industry as some developing countries are amid an upswing in economic development, and occupational hazards are overlooked [Citation24]. It is worth noting that increases in ASDR and age-standardized DALYs rate of occupational carcinogen-associated TBL cancer were observed in the lower SDI quintiles (low, low-middle, and middle SDI quintiles) in the past 30 years. Therefore, the ASRs trend of occupational carcinogen-associated TBL cancer in high SDI countries suggested that lower SDI quintiles should prevent and prohibit the use of products that contain occupational carcinogens in advance as these countries continue to industrialize, to minimize the burden of TBL cancer due to these carcinogens in the future.
4.3. Information from the differences of TBL cancer burden related to occupational carcinogens among genders and age groups
Our study confirms the presence of clear gender segregation in the global burden of TBL cancer caused by occupational carcinogens which is in line with previous studies [Citation25].
The TBL cancer burden caused by occupational carcinogens in female is of particular concern. Males are the main occupational carcinogen-associated TBL cancer burden population while the ASDR and age-standardized DALYs rate related to TBL cancer in females have shown an upward trend in the past 30 years. Specifically, TBL cancer-related ASRs due to occupational diesel engine exhaust exposure and polycyclic aromatic hydrocarbons showed a significantly upward trend in females compared to males. The age-standardized SEV rate increased in diesel engine exhaust and polycyclic aromatic hydrocarbons exposure is significantly faster in females than in males from the perspective of occupational exposure, in our study.
Research showed waitresses who have worked for more than 10 years have a significantly increased risk of lung cancer, which may be related to exposure to cooking fumes including polycyclic aromatic hydrocarbons [Citation26–29]. The dual exposure of work demands and responsibility for the household may also have an impact on the relationship between exposure and health, particularly for women. The burden of household responsibilities may result in less time for recovery after work [Citation30].
There is also a misconception that women are predominantly in occupations traditionally viewed as safe from hazards. However, in reality, they are exposed to health hazards [Citation31]. A research in New Zealand suggested that substantial differences in occupational exposure patterns between males and females and the influence of gender should not be overlooked in occupational health research, even within the same occupation, as reported in the literature [Citation30]. Additionally, many research tools and methodologies in occupational health were originally developed for male-dominated occupations and may not adequately reflect the experiences of women [Citation32]. Females may have different rates of chemical absorption, metabolism and bioavailability compared to males [Citation33]. Accordingly, at the same time, future occupational health studies need to recognize the possibility of measuring biases when determining occupational hazard exposures for males and females. They need to advance the field by developing measures that accurately reflect the type, intensity, and duration of the work that males and females do in their respective occupations. Gender analysis incorporation into work and health research can help to better understand the occupational safety needs of workers, particularly females [Citation34].
Occupational silica exposure was the leading risk in the 20–39 and 40–59 age groups while occupational exposure to asbestos was the leading risk in the 60–79 and 80 plus age groups. This may be related to the longer incubation period of asbestos-induced TBL cancer than in silica [Citation35]. Literature suggests that asbestos-attributable TBL cancer deaths are expected to persist for 40–50 years [Citation4]. This suggested that for TBL cancer attributable to different occupational carcinogens measures should be taken to reduce occupational exposure, and on the other hand, the optimal age of early screening for TBL cancer should be determined according to the incubation period.
Our studies suggested that the 60–79 age group is the main occupational carcinogens related TBL cancer burden population in all regions while lower SDI quintiles had higher death proportions in the <60-year-old population than the higher SDI quintiles. A possible reason for this phenomenon is that in developing countries more casual and young workers may be hired due to industrial expansion. These young temporary workers have less access to health care, and they may continue to receive hazard exposure elsewhere after leaving temporary jobs with occupational disease risk factors [Citation36,Citation37]. Therefore, in these developing countries, regulations should be formulated to limit the minimum working age for high-risk occupations, and to improve the protective measures for the protection of workers from occupational carcinogens.
4.4. Information from the differences in TBL cancer burden related to different occupational carcinogens
Overall, our research suggested that there were 13 occupational carcinogens included in the occupational risks in the GBD 2019 study, and nine occupational carcinogens were considered to be associated with TBL cancer. Occupational asbestos exposure was responsible for the largest number of deaths and DALYs of TBL cancer followed by occupational exposure to silica and diesel engine exhaust consistent with previous studies [Citation5].
Our data showed Australasia, Western Europe, and high-income North America with the highest ASDR and age-standardized DALYs rate of TBL cancer attributed to asbestos, both in 1990 and 2019. Over the past three decades, the number of new cases is still increasing although several countries in Western Europe and high-income North America, such as Italy and Canada, have taken steps to restrict the production and transportation of asbestos and minimize occupational asbestos carcinogen exposure [Citation38–41]. A plausible explanation is that due to the long incubation period, cancer deaths attributable to asbestos are expected to persist for 40–50 years even if asbestos exposure is completely stopped [Citation4]. Regions such as South Asia and East Asia have used increasing amounts of asbestos in recent decades and are still using it in a variety of occupational circumstances [Citation42–44]. Combined with their large workforces, this means the identified deaths from asbestos-related TBL cancer in these regions are a forerunner of what can be expected to be a far higher number of deaths in the coming decades.
The results presented here serve to emphasize the importance of eliminating occupational asbestos exposure, given its continuing legacy of past exposure in countries that have banned its use, and the likelihood that countries still using it will face a similar fate in future years. Therefore, it is necessary to legislate a worldwide ban on asbestos mining and the importation, manufacture, or use of asbestos-containing products [Citation45]. A possible method includes the use of less dangerous, but not necessarily risk-free, man-made mineral fibers such as rock wool are advocated in place of asbestos.
Occupational exposure to diesel engine exhaust is the third largest contributor to the occupational TBL cancer burden. Our study’s results revealed that the age-standardized SEV rate of occupational exposure to diesel engine exhaust increased for both sexes combined. Diesel engines are widely used in transportation and power supply (mining, construction work, professional driving, agriculture, and other activities that apply diesel-powered vehicles and tools), making occupational exposure to diesel exhaust common [Citation46]. Achieving an acceptable level of exposure to diesel engine exhaust is likely to require significant policy changes and prompt turnover of the existing fleet of old diesel-powered machinery and vehicles [Citation47]. Fortunately, the US and EU have introduced increasingly stringent diesel engine road emissions standards (US 2010 and Euro 6), followed by other countries (e.g. China, India, Brazil) [Citation48]. However, such exposure reductions will take decades to impact the TBL cancer burden.
Occupational carcinogen exposure is an important preventable cause of TBL cancer [Citation5, Citation49]. The results of our and previous studies highlight the need for all countries to work to eliminate or control occupational carcinogen exposure, which is insufficient in many lower SDI countries and is sometimes comparable to the high-exposure levels experienced in high-income countries over the past few decades. Suitable approaches include further development of global and regional frameworks for the control of occupational carcinogens; adopting and enforcing relevant legislation; strengthening exposure and outcome data collection and reporting at the country level and emphasizing the importance of primary prevention [Citation50]. At the same time, early TBL cancer detection must be implemented, with a special emphasis on strengthening treatment to reduce disease progression and severity [Citation51].
4.5. Comparison with other studies
Recently, there have been a few similar studies in part involving occupational carcinogen-associated TBL cancer. The GBD 2019 Respiratory Tract Cancers Collaborators analyzed all TBL cancer-related risk factors based on the GBD 2019 data [Citation10]. They conducted a comprehensive study of the disease burden and risk factors of TBL cancer. Studies suggested that occupational asbestos exposure was the third highest specific risk factor, for deaths from TBL cancer after smoking and ambient particulate matter pollution in 2019. However, occupational carcinogen-associated TBL cancer as a small part of the content has not been analyzed in detail. Our study added a SEV analysis of each occupational carcinogen and a spatial and temporal analysis of the TBL cancer burden attributable to occupational carcinogens among regions, countries and territories, genders, and age groups. In addition, Na Li et al. analyzed all cancers caused by 13 occupational carcinogens based on data from the GBD 2017 study [Citation5]. They focused on the analysis of the cancer burden caused by 13 occupational carcinogens. They similarly did not do a detailed analysis of occupational carcinogen-associated TBL cancer alone.
Using the latest GBD 2019 data, our study systematically and meticulously focused on occupational carcinogen-associated TBL cancer. This is the most detailed study of occupational carcinogen-associated TBL cancer based on the GBD 2019 database. Our study is the first to analyze the age-period-cohort effects in the temporal trends in TBL cancer mortality attributable to occupational carcinogens on a global scale. Our study complements the aforementioned similar studies and provides a more detailed basis for the precise formulation of measures to reduce the burden of occupational carcinogen-associated TBL cancer.
4.6. Limitations
Of course, our study has certain limitations. Firstly, the accuracy of GBD data depends on the quality and quantity of data collected. These figures undoubtedly underestimate the true burden since many occupational TBL cancer are not systematically documented and some economically underdeveloped regions lack high-quality cancer registries. Secondly, workforce data in some countries were extrapolated by modeling the local regional data; the exposure levels of the risk factors were measured by the distribution of industrial enterprises. These types of data assumptions would have introduced information bias into the current study. In addition, 47 occupational carcinogenic exposures have currently been identified according to the 2017 update from the IARC Monographs. Among them, 19 occupational carcinogens with sufficient evidence can cause lung cancer in humans [Citation52]. A total of 13 out of the total 47 occupational carcinogens were included in the GBD 2019 and nine were considered to be associated with TBL cancer in the GBD 2019 and were analyzed in our research. The remainder were excluded from the GBD because of a lack of suitable exposure data, and probable insufficient number of cases, insufficient exposure level, and/or proportion of persons exposed [Citation4]. Therefore, 10 occupational carcinogens associated with lung cancer that were not included in the GBD 2019 analysis [chloromethyl methyl ether (technical -grade); coal-tar pitch; sulfur mustard; particulate matter in outdoor air pollution; welding fumes; outdoor air pollution; tobacco smoke, secondhand; X-ray radiation and gamma-radiation; Plutonium; and Radon-222 and its decay products) may have been underestimated in our study.
5. Conclusion
The age-standardized SEV rate of occupational carcinogens related to TBL cancer increased globally. The ASDR and age-standardized DALYs rate of occupational carcinogens related to TBL cancer showed a downward trend in all populations globally while different SDI quintiles had different trend patterns in the past 30 years. The 60–79 age group is the main occupational carcinogen-associated TBL cancer burden population in all regions, while lower SDI quintiles had higher death proportions in the <60-year-old populations than in the higher SDI quintiles. Males are the main occupational carcinogen-associated TBL cancer burden population while an upward trend of ASRs among females cannot be ignored. Occupational exposure to asbestos, silica, and diesel engine exhaust were the top three factors responsible for the burden of occupational carcinogen-associated TBL cancer. The trends of TBL cancer burden caused by different occupational carcinogens vary significantly among SDI quintiles, gender, and age groups. Our findings provided some evidence to guide prevention policy planning and control programs to mitigate the TBL cancer burden attributable to occupational carcinogens.
Author contributions
Yan Zhang: Conceptualization, Methodology, Formal analysis, Investigation, Writing – Original Draft. Mi Mi: Methodology, Software, Formal analysis, Investigation, Data Curation, Writing – Original Draft. Ning Zhu: Software, Validation, Investigation, Data Curation, Writing – Original Draft. Zhijun Yuan: Validation, Investigation, Visualization. Yuwei Ding: Validation, Investigation, Visualization. Yingxin Zhao: Investigation, Visualization. Yier Lu: Investigation, Visualization. Shanshan Weng: Resources, Writing – Review & Editing, Supervision, Project administration. Ying Yuan: Conceptualization, Writing – Review & Editing, Supervision, Project administration, Funding acquisition.
Supplemental Material
Download PDF (1.2 MB)Acknowledgments
We highly appreciate the works of the Global Burden of Disease Study 2019 collaborators. Thanks to Xiao Ming ([email protected]) for his work in the GBD database. His excellent sharing of GBD database analysis procedure and other public database, makes it easier for us to explore the GBD database.
Disclosure statement
No potential conflict of interest was reported by the author(s).
Data availability statement
The datasets supporting the conclusions of this article are included within the article.
Additional information
Funding
References
- Bray F, Ferlay J, Soerjomataram I, et al. Global cancer statistics 2018: GLOBOCAN estimates of incidence and mortality worldwide for 36 cancers in 185 countries. CA Cancer J Clin. 2018;68(6):1–25.
- Lim SS, Vos T, Flaxman AD, et al. A comparative risk assessment of burden of disease and injury attributable to 67 risk factors and risk factor clusters in 21 regions, 1990–2010: a systematic analysis for the global burden of disease study 2010. Lancet. 2012;380(9859):2224–2260.
- Cullinan P, Munoz X, Suojalehto H, et al. Occupational lung diseases: from old and novel exposures to effective preventive strategies. Lancet Respir Med. 2017;5(5):445–455.
- G. B. D. Occupational Carcinogens Collaborators. Global and regional burden of cancer in 2016 arising from occupational exposure to selected carcinogens: a systematic analysis for the Global Burden of Disease Study 2016. Occup Environ Med. 2020a;77(3):151–159.
- Li N, Zhai Z, Zheng Y, et al. Association of 13 occupational carcinogens in patients with cancer, individually and collectively, 1990–2017. JAMA Netw Open. 2021;4(2):e2037530.
- Hoy RF, Chambers DC. Silica-related diseases in the modern world. Allergy. 2020;75(11):2805–2817.
- Thives LP, Ghisi E, Thives Junior JJ, et al. Is asbestos still a problem in the world? A current review. J Environ Manage. 2022;319:115716.
- Brims FJH, Kong K, Harris EJA, et al. Pleural plaques and the risk of lung cancer in asbestos-exposed subjects. Am J Respir Crit Care Med. 2020;201(1):57–62.
- Fan Y, Jiang Y, Li X, et al. Burden of lung cancer attributable to occupational carcinogens from 1990 to 2019 and projections until 2044 in China. Cancers. 2022;14(16):3883.
- G. B. D. Respiratory Tract Cancers Collaborators. Global, regional, and national burden of respiratory tract cancers and associated risk factors from 1990 to 2019: a systematic analysis for the global burden of disease study 2019. Lancet Respir Med. 2021;9(9):1030–1049.
- G. B. D., and Collaborators Injuries Diseases. Global burden of 369 diseases and injuries in 204 countries and territories, 1990–2019: a systematic analysis for the global burden of disease study 2019. Lancet. 2020;396(10258):1204–1222.
- G. B. D. Risk Factor Collaborators. Global, regional, and national comparative risk assessment of 84 behavioural, environmental and occupational, and metabolic risks or clusters of risks for 195 countries and territories, 1990–2017: a systematic analysis for the global burden of disease study 2017. Lancet. 2018;392(10159):1923–1994.
- G. B. D. Risk Factors Collaborators. Global burden of 87 risk factors in 204 countries and territories, 1990-2019: a systematic analysis for the global burden of disease study 2019. Lancet. 2020b;396(10258):1223–1249.
- Zhai M, Jiang Q, Liu S, et al. DALY trend and predictive analysis of COPD in China and its provinces: findings from the global burden of disease study. Front Public Health. 2022;10:1046773.
- Kim HJ, Fay MP, Feuer EJ, et al. Permutation tests for joinpoint regression with applications to cancer rates. Statist Med. 2000;19(3):335–351.
- Rosenberg PS, Check DP, Anderson WF. A web tool for age-period-cohort analysis of cancer incidence and mortality rates. Cancer Epidemiol Biomarkers Prev. 2014;23(11):2296–2302.
- Abtahi M, Koolivand A, Dobaradaran S, et al. National and Sub-national age-sex specific and cause-specific mortality and disability-adjusted life years (DALYs) attributable to household air pollution from solid cookfuel use (HAP) in Iran, 1990–2013. Environ Res. 2017;156:87–96.
- Aragon A, Partanen T, Felknor S, et al. Social determinants of workers’ health in Central America. Int J Occup Environ Health. 2011;17(3):230–237.
- Burrows S, Auger N, Gamache P, et al. Individual and area socioeconomic inequalities in cause-specific unintentional injury mortality: 11-year follow-up study of 2.7 million Canadians. Accid Anal Prev. 2012;45:99–106.
- Steenland K, Halperin W, Hu S, et al. Deaths due to injuries among employed adults: the effects of socioeconomic class. Epidemiology. 2003;14(1):74–79.
- Wang H, Chen G, Wang Z, et al. Socioeconomic inequalities and occupational injury disability in China: a population-based survey. Int J Environ Res Public Health. 2015;12(6):6006–6015.
- Mao Y, Yang D, He J, et al. Epidemiology of lung cancer. Surg Oncol Clin N Am. 2016;25(3):439–445.
- Wang Z, Hu L, Li J, et al. Magnitude, temporal trends and inequality in global burden of tracheal, bronchus and lung cancer: findings from the global burden of disease study 2017. BMJ Glob Health. 2020;5(10):e002788.
- Zhou H, Zhou Y, Zhang H, et al. Socio-economic disparity in the global burden of occupational noise-induced hearing loss: an analysis for 2017 and the trend since 1990. Occup Environ Med. 2021;78(2):125–128.
- Scarselli A, Corfiati M, Di Marzio D, et al. Gender differences in occupational exposure to carcinogens among Italian workers. BMC Public Health. 2018;18(1):413.
- Fullana A, Carbonell-Barrachina AA, Sidhu S. Comparison of volatile aldehydes present in the cooking fumes of extra virgin olive, olive, and canola oils. J Agric Food Chem. 2004;52(16):5207–5214.
- Guillen MD, Cabo N, Ibargoitia ML, et al. Study of both sunflower oil and its headspace throughout the oxidation process. Occurrence in the headspace of toxic oxygenated aldehydes. J Agric Food Chem. 2005;53(4):1093–1101.
- Lee CH, Ko YC, Goggins W, et al. Lifetime environmental exposure to tobacco smoke and primary lung cancer of non-smoking taiwanese women. Int J Epidemiol. 2000;29(2):224–231
- Xu M, Ho V, Siemiatycki J. Role of occupational exposures in lung cancer risk among women. Occup Environ Med. 2021;78(2):98–104.
- Eng A, ‘t Mannetje A, McLean D, et al. Gender differences in occupational exposure patterns. Occup Environ Med. 2011;68(12):888–894.
- McDiarmid MA, Gucer PW. The "GRAS" status of women’s work. J Occup Environ Med. 2001;43(8):665–669.
- Messing K, Östlin P. 2006. Gender equality, work and health: a review of the evidence. World Health Organization. Available from: https://apps.who.int/iris/handle/10665/43311.
- Gochfeld M. Sex differences in human and animal toxicology. Toxicol Pathol. 2017;45(1):172–189.
- Biswas A, Harbin S, Irvin E, et al. Sex and gender differences in occupational hazard exposures: a scoping review of the recent literature. Curr Environ Health Rep. 2021;8(4):267–280.
- Steenland K, Mannetje A, Boffetta P, et al. Pooled exposure-response analyses and risk assessment for lung cancer in 10 cohorts of silica-exposed workers: an IARC multicentre study. Cancer Causes Control. 2001;12(9):773–784.
- Kolstad HA, Olsen J. Why do short term workers have high mortality? Am J Epidemiol. 1999;149(4):347–352.
- Lamm SH, Levine MS, Starr JA, et al. Analysis of excess lung cancer risk in short-term employees. Am J Epidemiol. 1988;127(6):1202–1209.
- Baas P, van ‘t Hullenaar N, Wagenaar J, et al. Occupational asbestos exposure: how to deal with suspected mesothelioma cases–the Dutch approach. Ann Oncol. 2006;17(5):848–852.
- Bianchi C, Bianchi T. The possible role of asbestos exposure in the pathogenesis of a thoracic non-Hodgkin lymphoma. Arch Bronconeumol. 2016;52(9):490–491.
- De Vos Irvine H, Lamont DW, Hole DJ, et al. Asbestos and lung cancer in Glasgow and the west of Scotland. BMJ. 1993;306(6891):1503–1506.
- Tompa E, Kalcevich C, McLeod C, et al. The economic burden of lung cancer and mesothelioma due to occupational and para-occupational asbestos exposure. Occup Environ Med. 2017;74(11):816–822.
- Joshi TK, Gupta RK. Asbestos in developing countries: magnitude of risk and its practical implications. Int J Occup Med Environ Health. 2004;17(1):179–185.
- Le GV, Takahashi K, Park EK, et al. Asbestos use and asbestos-related diseases in Asia: past, present and future. Respirology. 2011;16(5):767–775.
- Leong SL, Zainudin R, Kazan-Allen L, et al. Asbestos in Asia. Respirology. 2015;20(4):548–555.
- Kirby T. Canada accused of hypocrisy over asbestos exports. Lancet. 2010;376(9757):1973–1974.
- Taxell P, Santonen T. Diesel engine exhaust: basis for occupational exposure limit value. Toxicol Sci. 2017;158(2):243–251.
- Kim J, Peters CE, Arrandale VH, et al. Burden of lung cancer attributable to occupational diesel engine exhaust exposure in Canada. Occup Environ Med. 2018;75(9):617–622.
- Scheepers PT, Vermeulen RC. Diesel engine exhaust classified as a human lung carcinogen. How will this affect occupational exposures? Occup Environ Med. 2012;69(10):691–693.
- Driscoll T, Nelson DI, Steenland K, et al. The global burden of disease due to occupational carcinogens. Am J Ind Med. 2005;48(6):419–431.
- Espina C, Porta M, Schuz J, et al. Environmental and occupational interventions for primary prevention of cancer: a cross-sectorial policy framework. Environ Health Perspect. 2013;121(4):420–426.
- Steenland K, Ward E. Silica: a lung carcinogen. CA Cancer J Clin. 2014;64(1):63–69.
- Loomis D, Guha N, Hall AL, et al. Identifying occupational carcinogens: an update from the IARC monographs. Occup Environ Med. 2018;75(8):593–603.