Abstract
Background
Trimethylamine N-oxide (TMAO) derived from gut microbiota causes kidney-heart damage in chronic kidney disease (CKD) patients. However, it is controversial whether CKD patients with higher TMAO are associated with a higher risk of death. We aimed to assess the correlation between circulating TMAO concentration and the risk of all-cause and cardiovascular death in CKD patients of different dialysis statuses and different races by dose-response analyses, and the underlying mechanisms were also explored by analyzing the correlations of TMAO with glomerular filtration rate (GFR) and inflammation.
Method
PubMed, Web of Science, and EMBASE were systematically searched up to 1 July 2022. A total of 21 studies involving 15,637 individuals were included. Stata 15.0 was used to perform the meta-analyses and dose-response analyses with extracted data. Subgroup analyses were conducted to recognize possible sources of heterogeneity.
Results
The risk of all-cause mortality was increased in non-dialysis CKD patients (RR = 1.26, 95%CI = 1.03–1.54, p = 0.028) and non-black dialysis patients (RR = 1.62, 95%CI = 1.19–2.22, p = 0.002) with the highest circulating TMAO concentration, and the association was confirmed to be linear. In addition, an increased risk of cardiovascular mortality was also found in non-black dialysis patients with the highest circulating TMAO concentration (RR = 1.72, 95%CI = 1.19–2.47, p = 0.004), likewise, a linear association was identified. However, for dialysis patients including blacks with high TMAO concentrations, there was no significant increase in either all-cause mortality (RR = 0.98, 95%CI = 0.94-1.03, p = 0.542) or cardiovascular mortality (RR = 0.87, 95% CI = 0.65–1.17, p = 0.362). Meanwhile, we verified strong correlations between TMAO and both GFR (r= −0.49; 95% CI= −0.75, −0.24; p < 0.001) and inflammatory markers (r = 0.43; 95% CI= 0.03, 0.84; p = 0.036) in non-dialysis patients.
Conclusions
Increased circulating TMAO concentrations increase the risk of all-cause mortality in non-dialysis and non-black dialysis CKD patients. Moreover, elevated TMAO levels raise the cardiovascular mortality risk in non-black dialysis patients.
Non-dialysis and non-black dialysis CKD patients with higher circulating TMAO concentrations are associated with an increased risk of all-cause mortality.
Non-black dialysis patients with higher concentrations of TMAO are associated with an increased risk of cardiovascular mortality.
Circulating TMAO concentrations have a strong negative correlation with GFR and a positive correlation with inflammation biomarkers in non-dialysis CKD patients.
Key messages
Introduction
Chronic kidney disease (CKD) is a major threat to human health, which can cause multiple organs and system complications, and eventually develop into end-stage renal disease, which requires continuous dialysis or kidney transplantation, resulting in a decline in the quality of life and a heavy socioeconomic burden [Citation1]. In 2017, there were about 697.5 million CKD patients nationwide, with a prevalence rate of 9.1%, of which 1.2 million people died from CKD. Compared with 1990, the all-age mortality rate from CKD increased by 41.5% [Citation2]. Furthermore, cardiovascular mortality is significantly higher in individuals with CKD. [Citation3] Therefore, the prevention and treatment of CKD is becoming more and more urgent.
The gut-kidney-heart axis in chronic kidney disease has gradually attracted extensive attention [Citation4,Citation5], that is, the accumulation of gut microbial-derived uremic toxins leads to the occurrence and development of CKD, and increases the risk of cardiovascular events in CKD. Trimethylamine-N-oxide (TMAO), derived from dietary intake of animal-derived choline, L-carnitine, and plant-derived betaine, is one of the uremic toxins that cause kidney-heart damage. When food is transported to the intestinal lumen, it is metabolized by the intestinal flora to produce trimethylamine (TMA), which is absorbed through the hepatic portal venous system and converted to TMAO by flavin-containing monooxygenase (FMO) [Citation6,Citation7]. As we all know, the number of microbes that colonize the human gastrointestinal tract is 10 times greater than the number of the host’s own somatic and germ cells [Citation8], which defends against harmful substances and also produces absorbable nutrients. However, the gut flora in CKD patients becomes unbalanced and disordered [Citation9–12]. It is confirmed that the abundance of TMA-producing gut microbiota is increased in patients with T2DM-CKD [Citation13]. Under physiological conditions, circulating TMAO is excreted almost exclusively by the kidneys in the form of glomerular filtration and tubular secretion [Citation7,Citation14]. Thus, disruption of kidney function, together with dysbiosis of gut microbiota, leads to increased circulating TMAO concentrations in patients with CKD including end-stage kidney disease (ESKD) [Citation15–17], which in turn exacerbates kidney damage by promoting inflammation [Citation18–21].
Based on the pro-inflammatory and pro-fibrotic effects of TMAO, the association between TMAO and mortality in CKD patients has been a well-concerning focus over recent years, while the findings are not completely uniform. Studies have shown that TMAO level is an independent risk factor for death (5-year survival rate) in patients with CKD 3-5 [Citation22] and for hospitalization events in patients receiving maintenance hemodialysis [Citation23]. On the contrary, no significant correlation was found between plasma TMAO concentrations and the progression of disease or mortality in patients with CKD after adjusting for confounders [Citation24]. Therefore, we performed the current systematic review and meta-analyses to reveal the dose-response association between circulating TMAO concentrations and mortality. Meanwhile, from the perspective of etiology, the correlations of TMAO with renal function and inflammatory biomarkers in CKD patients were studied. To our knowledge, this is the first report to study dialysis and non-dialysis patients separately, which greatly increases the specificity of results.
Methods
The protocol of the recent study has been registered in the International prospective register of systematic reviews (PROSPERO) with the identification number CRD42022321968.
Data source and search strategy
A systematic search was performed in Web of Science, PubMed, and EMBASE through 1 July 2022. The literature search was carried out by the combination of Medical Subject Headings (MeSH) and free-text terms, with no date and language restrictions. Search strategies are presented in Supplementary Table 1. The reference list of all relevant articles and reviews is also manually searched to ensure that all relevant literature was included. Two authors searched the literature (YC. Li and HM. Lu), and two other authors (J. Guo and ML. Zhang) reviewed uncertain cases. The final decisions were made by consensus.
Study selection
The relevant observational studies with cohort, case-control, nested case-control, or analytic cross-sectional studies were included in the current review if they: (1) included CKD patients; (2) involved participants aged >18 y; (3) reported circulating TMAO concentrations for at least 2 and 3 categories and conducted 2-class and dose-response meta-analysis, respectively; (4) reported the correlation between circulating TMAO concentrations and GFR; (5) reported the correlation between circulating TMAO concentrations and inflammation-related indicators. Interventional studies, animal studies, and studies involving pregnant women and other serious diseases such as cancer or immune-related diseases were excluded.
Data extraction and quality assessment
The quality of all studies was assessed independently by two authors (YC. Li and HM. Lu). For studies with controversial evaluation results, we consulted with the third investigator (HJ. Zheng) to make the decision. The Newcastle–Ottawa Scale (NOS) [Citation25] and the Agency for Healthcare Research and Quality (AHRQ) [Citation26] checklist was used to assess the quality of cohort studies and cross-sectional studies, respectively. The items were scored ‘1’ if the answer was ‘YES’, and ‘0’ if the answer was ‘NO’ or ‘UNCLEAR’. The final quality assessment scores were as follows: low quality=0–3; moderate quality=4–7; high quality=8–11. In addition, the Quality in Prognosis Studies (QUIPS) tool, which is considered to be the newest and most robust method of assessing the risk of bias in systematic reviews/meta-analyses, was also used to evaluate the inexpensive risks of prognostic studies.
The standard extraction form was used to extract the data by two investigators (J. Guo and ML. Zhang) to independently obtain the below information: study characteristics (first author, publication year, location of the study, sample size, follow-up time, and study design), baseline characteristics of participants (age, gender, and disease status), TMAO characteristics (sample source from serum or plasma, and median, mean or range in each category of TMAO concentrations), and adjusted confounders. In addition, the number of deaths, sample size as well as HR, and 95% CI in each category of TMAO were extracted to conduct dose-response analyses. If relevant data were not directly reported in the original literature, the number of deaths and HR were estimated from survival curves. Besides, the r or rho values between TMAO concentration and GRF or inflammatory biomarkers were also recorded for meta-analyses of the correlations.
2.4. Data synthesis and analysis
First, we performed meta-analyses of the HRs of all-cause mortality and cardiovascular mortality in the category of highest TMAO concentration category. Second, dose-response analyses require the median or mean of TMAO concentrations for each category, which was preferentially extracted. If not given directly, data were calculated as the median of the TMAO concentration range for each category. If the TMAO concentration is an open interval, it is derived using the interval of adjacent classes. Third, for correlation analyses, confidence intervals are calculated after converting the correlation coefficient (r) to fisher’Z, and then converting back to the correlation coefficient (r) [Citation27].
Furthermore, we performed a heterogeneity test for each effect size, and significant statistical heterogeneity was affirmed if I2>50%. Riley et al. [Citation28] proposed that the random-effects model was considered the best method to assess the effect size, because of the inconsistencies in study design, sample source, or the number of patients in all included studies. In this case, a random effect model was used for meta-analyses. A variety of different subgroup analyses were conducted to explore sources of literature heterogeneity. Funnel plots were used to assess the publication bias. If the number of articles included to assess each effect size is less than 10, the detection efficiency of publication bias is too low to be tested [Citation29].
Results
Literature search and study characteristics
A total of 691 articles were retrieved, and 462 articles remained after removing duplicate articles, which were screened by reading the title and abstract. 50 literature were left for full-text assessment. Finally, we included 21 studies for meta-analyses. According to the consistency test of the results of the two authors on whether the literature was included or not, the kappa value was 0.836, indicating a high consistency of the included literature. The flow chart of literature search and screening has been presented in .
The characteristics of the included studies and the demographic and mean baseline parameters of the patients are summarized in . We included studies from almost all over the world, with 5 from China and Japan in Asia [Citation33,Citation47,Citation41,Citation42,Citation44], 9 from the US and Canada in North America [Citation24,Citation30,Citation35,Citation31,Citation32,Citation34,Citation13,Citation36,Citation37,Citation46], 5 from Sweden, the Netherlands and Denmark in Europe [Citation22,Citation34,Citation39,Citation43,Citation45], and 1 from Egypt in Africa [Citation40]. The research type was mainly cohort with 17 studies, and cross-sectional with 3 studies [Citation33,Citation13,Citation40]. The average age of the participants ranged from 46 to 70 years old. Median follow-up time fluctuated in the range of 2.3–15 years. All studies included both males and females.
Table 1. Characteristics of the selected studies.
A total of 21 studies involving 15,637 participants from 2015 to 2022 were included in our meta-analysis. After excluding 1 literature by Stubbs [Citation32] containing both dialysis and non-dialysis patients, we included a total of 7 articles [Citation24,Citation30,Citation22,Citation35,Citation46,Citation39,Citation43] to analyze the association between circulating TMAO concentrations and all-cause mortality, and 3 articles [Citation46,Citation39,Citation43] to analyze the association between circulating TMAO and cardiovascular mortality, in non-dialysis CKD patients. Three out of 7 studies were conducted on patients with diabetes complicated by nephropathy, of which 1 for type 1 diabetes mellitus with proteinuria [Citation43], 1 for type 2 diabetes mellitus with macroalbuminuria [Citation39], and 1 for non-dialysis DKD patients [Citation46]. In addition, 8 [Citation31,Citation41,Citation42,Citation44,Citation36,Citation37,Citation45)] and 6 [Citation41,Citation42,Citation44,Citation36,Citation37] studies only observing dialysis patients were included to analyze the association of TMAO and all-cause and cardiovascular mortality respectively. Of note, the study by Shafi et al. [Citation36] was included as 2 independent studies due to racial differences. Of all studies on dialysis patients, 4 studies [Citation41,Citation42,Citation44,Citation36] only included non-blacks, and the remaining included black patients. Moreover, 8 [Citation30,Citation22,Citation33,Citation34,Citation35,Citation46,Citation40] and 7 [Citation30,Citation31,Citation22,Citation13,Citation47,Citation45,Citation40] correlation coefficients were reported between TMAO concentrations and GFR as well as inflammatory markers in non-dialysis CKD patients, respectively. Based on the results of subgrouping, we further performed dose-response dialysis of two-class variables for studies, which contains at least 3 categories of TMAO concentrations.
Meta-analysis of the association between circulating TMAO concentrations and all-cause mortality risk
15 studies with 5628 participants were included for the analysis of the association between TMAO and all-cause mortality, 7 of which reported non-dialysis patients only [Citation24,Citation30,Citation22,Citation35,Citation46,Citation43]. The results showed that the risk of all-cause mortality was significantly increased in non-dialysis CKD patients with high levels of TMAO (RR = 1.26, 95%CI = 1.03–1.54, p = 0.028, ). Subgrouping () according to age, GFR, and underlying diseases indeed reduced the heterogeneity, and also showed that the statistical association of circulating TMAO with the risk of all-cause mortality was not found in patients with aged <60, GFR > 60, and underlying diabetes mellitus.
Figure 2. Meta-analysis of the association between circulating TMAO concentrations and all-cause mortality in non-dialysis patients and dialysis patients respectively. RR: relative risk; CI: confidence interval.
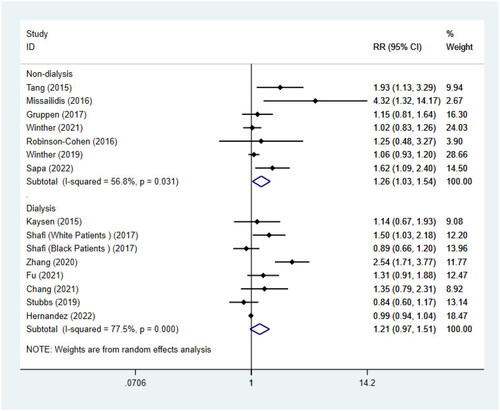
Table 2. Subgroup analysis of the association between circulating TMAO concentrations and all-cause mortality in non-dialysis CKD patients.
For the remaining 8 studies [Citation31,Citation41,Citation42,Citation44,Citation36,Citation37] on dialysis patients, we didn’t find a significant statistical association between TMAO and all-cause mortality (RR = 1.21, 95%CI = 0.97–1.51, p = 0.094, ). When we further performed a subgroup analysis () by race, age, and dialysis modality, heterogeneity was significantly reduced. Interestingly, it showed that there was a notable association between the highest quartile of TMAO and the risk of all-cause mortality in 4 studies [Citation41,Citation42,Citation44,Citation36] only including non-blacks (RR = 1.62, 95%CI = 1.19–2.22, p = 0.002). The statistical association between TMAO and all-cause mortality was still not found in studies involving blacks (RR = 0.98, 95%CI = 0.94-1.03, p = 0.542). The article by Kaysen [Citation31] mentioned that non-blacks were associated with higher mortality. This is consistent with the conclusions of our meta-analyses. In addition, subgrouping () also revealed the association between TMAO concentrations and all-cause mortality was influenced by age of individuals, but not influenced by dialysis modality.
Table 3. Subgroup analysis of the association between circulating TMAO concentrations and all-cause mortality in dialysis patients.
Meta-analysis of the association between circulating TMAO concentrations and cardiovascular mortality
9 studies were included to conduct the association of TMAO concentrations and cardiovascular mortality, coincidentally, of which 3 for non-dialysis CKD patients with diabetes complicated by nephropathy [Citation46,Citation39,Citation43] and 6 for dialysis patients [Citation41,Citation42,Citation44,Citation36,Citation37]. A report by Shafi [Citation36] was considered to be 2 independent studies. Consistent with the above results, there was no statistical association between TMAO concentrations and cardiovascular death in patients with diabetes and nephropathy (RR = 1.00, 95%CI = 0.87–1.15, p = 0.959, ). For the remaining 6 studies of dialysis patients, no significant association was also found between TMAO and the risk of cardiovascular mortality (RR = 1.35, 95%CI = 0.94–1.93, p = 0.110, ), and the same results were seen in the subgroup analysis by dialysis modality (). However, similar to the results of all-cause mortality risk, the result of subgrouping based on race showed that there was a remarkable association between TMAO and cardiovascular mortality in non-black patients (RR = 1.72, 95%CI = 1.19–2.47, p = 0.004, ). Of course, heterogeneity was also significantly decreased. Because all studies included patients aged <60, we did not perform subgroup analysis by age ().
Figure 3. Meta-analysis of the association between circulating TMAO concentrations and cardiovascular mortality in non-dialysis patients and dialysis patients respectively. HR: hazard risk; CI: confidence interval.
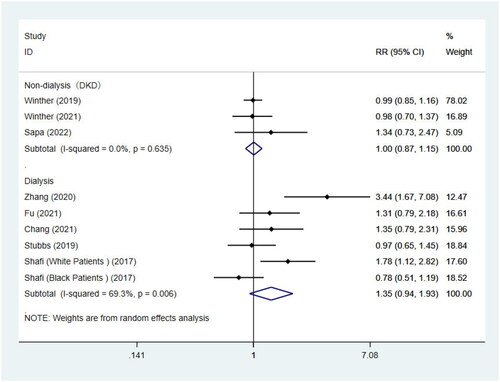
Table 4. Subgroup analysis of the association between circulating TMAO concentrations and cardiovascular mortality in dialysis patients.
Dose-response meta-analysis of the association between circulating TMAO concentrations and all-cause mortality
Based on the results of subgrouping, we performed dose-response analyses of studies with ≥3 categories of TMAO concentrations, including 3 articles [Citation24,Citation30,Citation35] on non-dialysis CKD patients and 3 studies [Citation42,Citation44,Citation36] on non-black dialysis patients. Dose-response analyses showed that the TMAO concentrations were linearly related to the risk of all-cause mortality in both non-dialysis CKD () and non-black dialysis patients (), which revealed that the risk of all-cause mortality increased gradually with the increase of TMAO concentrations.
Dose-response meta-analysis of the association between circulating TMAO concentrations and cardiovascular mortality
The results of the above subgrouping unraveled that TMAO concentrations were strongly correlated with cardiovascular mortality only in the non-black dialysis population. Therefore, we included 3 studies [Citation42,Citation44,Citation36] containing at least 3 categories of TMAO concentrations to perform the dose-response analysis. It also showed a linear relationship between TMAO concentrations and the risk of cardiovascular mortality (). That is, higher circulating TMAO concentrations were associated with a greater risk of cardiovascular death in non-black dialysis patients.
Meta-analysis of the correlations between circulating TMAO concentrations and GFR
We included 8 studies [Citation30,Citation32,Citation22,Citation33,Citation34,Citation35,Citation46,Citation40] involving 9524 individuals to perform the meta-analyses of correlations between TMAO and GFR. Results suggested that circulating TMAO concentrations are strongly correlated with GFR in non-dialysis CKD patients (r= −0.49; 95% CI= −0.75, –0.24; p <0.001, ). To examine sources of heterogeneity, and to verify effect sizes across groups, subgrouping was performed according to age (>60 or <60) and GFR levels (>60 or <60). The negative correlation between TMAO concentrations and GFR was stronger among patients with GFR <60 than among those with GFR >60 [GFR <60 (r= −0.58; 95% CI= −0.83, –0.33; p < 0.001), GFR >60 (r= −0.24; 95% CI= −0.40, –0.07; p = 0.006), ].
Figure 7. Meta-analysis of the correlations between circulating TMAO concentrations and GFR in non-dialysis CKD patients. r, coefficient of association; CI, confidence interval.
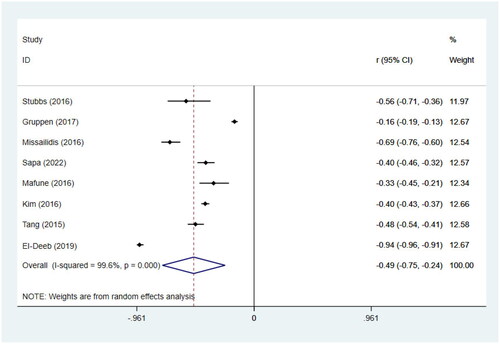
Table 5. Subgroup analysis of the correlations between circulating TMAO concentrations and GFR in non-dialysis CKD patients.
Meta-analysis of the correlations between circulating TMAO concentrations and inflammation biomarkers
7 correlation coefficients in 4 studies [Citation30,Citation22,Citation13,Citation40] for non-dialysis patients and 3 correlation coefficients in 3 studies [Citation31,Citation47,Citation45] for dialysis patients were included to perform meta-analyses of the correlations between circulating TMAO concentrations and inflammation biomarkers (). A remarkable correlation between TMAO concentrations and inflammation biomarkers was found in non-dialysis patients (r = 0.43; 95% CI= 0.03, 0.84; p = 0.036, ). However, in dialysis patients, TMAO concentrations did not appear to be statistically correlated with inflammatory markers (r = 0.02; 95% CI= −0.43, 0.46; p = 0.937, ). It’s worth noting that, due to the relatively few studies included for dialysis patients, the results may be greatly biased. Interestingly, we observed that 2 studies [Citation31,Citation45] reported negative correlations between TMAO and inflammatory markers, conversely, 1 reported a positive correlation [Citation47]. These results remind us that more extensive researches are needed to verify the relationship between TMAO and inflammatory markers in dialysis patients and contribute to the clinical treatment scheme.
Study quality assessment
NOS was used to assess the quality of the included cohort studies with NOS scores of 5-9 (Supplementary Table 2). The AHRQ checklist was used for cross-sectional studies, and the scores were 6–8 (Supplementary Table 3). Almost all studies were judged as moderate or high quality. The QUIPS checklist was used for prognosis studies, and 6 studies were rated as high quality, 10 as moderate quality, and 1 as low quality (Supplementary Table 4).
Publication bias
The meta-analysis included less than 10 studies for each outcome indicator. Therefore, only a funnel plot was used to detect publication bias (Supplementary Figure 1).
Discussion
The association between TMAO and mortality has been reported in several previous studies, most recently four months ago [Citation48,Citation49,Citation50]. However, based on including more literature, we have reached further and more precise conclusions in CKD patients. Because of the remarkable difference between dialysis and non-dialysis patients in TMAO concentrations and health status, this is the first report to study the dialysis and non-dialysis CKD patients separately and the first dose-response analyses of the association between circulating TMAO and mortality in CKD patients. Meanwhile, this is also the first systematic review and meta-analysis to focus on ethnic differences in the association of TMAO concentrations and mortality in CKD patients. In addition, we deeply studied the correlation between TMAO and GFR or inflammatory biomarkers to explore the reasons why TMAO caused kidney damage and increased the risk of death. Ultimately, we hope that more precise treatment strategies of dietary modification or drug therapy can be implemented in patients with CKD who are at increased risk of adverse outcomes.
In this study, it was confirmed that elevated TMAO concentrations were associated with increased risk of death in non-dialysis and non-black dialysis CKD patients through both two-class and dose-response analyses, which could be explained by the following mechanisms. First of all, renal fibrosis is the common final outcome of almost all CKD. Administration of choline or TMAO can induce and aggravate renal impairment and renal fibrosis in experimental animals [Citation30,Citation51], while inhibiting the production of TMAO can improve renal pathological injury, protect renal function, and delay the progression of disease in CKD mice [Citation52], suggesting that TMAO is not only a biomarker of impaired renal function but also a potential pathogenic factor that destroys renal function in CKD. Our results of meta-analyses validated this, and found that circulating TMAO concentrations indeed had a clear negative correlation with GFR. The specific mechanism may involve the activation of the TGF-β RI/Smad2 pathway by TMAO, which upregulates the expression of α-SMA and collagen I [Citation53]. TMAO stimulation can induce the proliferation of renal fibroblasts and subsequently increase total collagen expression in a dose-dependent manner with TMAO concentration [Citation54]. Taken together, TMAO can damage renal function by promoting renal fibrosis, therefore, there is a strong correlation between TMAO and GFR, which is consistent with our conclusion of the correlation meta-analysis. However, we found that the association between TMAO and all-cause mortality in almost all studies of non-dialysis patients was adjusted for GFR. The results suggest that the predictive value of circulating TMAO concentrations for the risk of all-cause death is not entirely or directly dependent on the decline in renal function, and may be related to the deterioration of other health conditions caused by the decline in renal function.
As we all know, inflammation is a crucial factor in the progression of CKD, and TMAO has been shown to play a pro-inflammatory role in cardiovascular disease, atherosclerosis, and neurological disorders [Citation55,Citation56,Citation57]. In addition, peritoneal dialysis patients with higher TMAO concentrations have been validated to have a higher risk of developing new-onset peritonitis [Citation47]. Therefore, we performed a meta-analysis of the correlation between TMAO and inflammatory markers in CKD patients. In non-dialysis patients, TMAO concentrations did show strong correlations with inflammatory markers. It may involve the following mechanisms. The elevation of circulating TMAO triggers M1 polarization of macrophages and stimulates the expression of NLRP3 and Caspase-1 to activate the inflammasome [Citation54,Citation58,Citation59]. Meanwhile, TMAO can also activate NFκB and promote the adhesion of leukocytes to the endothelial cells, thereby leading to vascular inflammation [Citation20]. The activation of p38MAPK and the up-regulation of human antigen R are also one of the mechanisms by which TMAO upregulates inflammatory factors, increases the infiltration of inflammatory cells, and causes renal failure [Citation60]. Therefore, inflammation, which is strongly correlated with TMAO in non-dialysis patients, is one of the factors by which high TMAO concentrations increase the risk of death. Apart from these, TMAO can directly contribute to heightened platelet reactivity and increase the thrombosis (heart attack and stroke) risk [Citation38]. TMAO has been found to impair pyruvate and fatty acid oxidation in cardiac mitochondria, leading to disturbances in energy metabolism [Citation61], which may elucidate higher cardiovascular mortality associated with TMAO. Surprisingly, of the only 3 reports on the correlation of TMAO with inflammatory markers, 2 reported a negative correlation. More studies on dialysis patients should be done to minimize bias in trials and reflect the facts.
Our meta-analysis manifested an interesting finding that TMAO concentrations were linearly associated with both all-cause and cardiovascular mortality in non-black dialysis patients, but not in studies involving black patients, which has not been reported in previous meta-analyses. We consulted the relevant literature and wondered whether this result might be caused by differences in TMAO concentrations between different races, and found contradictory results. No statistically significant difference was reported in serum TMAO concentrations between black and white dialysis patients by Shafi [Citation36], whereas the opposite result has been presented in a study on patients with the risk for atherosclerotic cardiovascular disease. It shows that compared with whites, non-white participants were 2.92 times more likely to be in the top quartile of TMAO. However, there are few studies on the difference of blood TMAO concentrations in Asians. In a word, the current evidence on the variability of TMAO concentrations among ethnic can’t explain the results of our meta-analyses, and further research is needed.
In addition to the difference in TMAO concentrations among races, the study by Elaine Ku [Citation62] has shown that black participants are younger and in better health status when dialysis started compared with whites. Thus, the baseline differences lead to a survival advantage for black adults who received dialysis over whites. Beyond those, research on Asians is still lacking. This suggests that in future studies on dialysis mortality in different ethnic groups, we should adjust as much as possible for the effects of underlying diseases and health status.
In brief, TMAO is linearly associated with all-cause mortality in non-dialysis CKD patients and non-black dialysis patients, and also linearly associated with cardiovascular mortality in non-black dialysis patients. Therefore, attention should be paid to controlling the circulating TMAO concentrations of target patients in clinical practice. Because dietary intake is the main source of circulating TMAO, dietary adjustments are considered important. Protein intake at twice the recommended dietary allowance increases the circulating TMAO in healthy older males [Citation63], while a low protein diet declines it in non-dialysis patients [Citation64]. Supplement of oats β-glucan has been demonstrated to be safe and effective in reducing TMAO levels with well tolerated in patients with CKD stages 3-4 [Citation65] [ In addition, elevated plasma TMAO levels are detected in CKD rats transplanted with microbiota obtained from CKD patients [Citation66], so dysbiosis of the intestinal microbiome also plays a key role in the production of TMAO.
Animal experiments confirmed that treatment with butyrate (a short-chain fatty acid originated from dietary fiber) [Citation66], ranitidine, finasteride [Citation67] and rhubarb enema [Citation68] can significantly increase the richness and diversity of some symbiotic bacteria and probiotics, while reduce the abundance of some potential pathogens, thus inhibit the synthesis and release of TMAO, eventually delaying the progression of CKD. However, clinical trials indicate that short-term probiotic supplementation for 3 months has no effect on TMAO levels in HD patients [Citation69]. Long-term clinical observation should be performed to obtain more reliable conclusions. In general, reducing circulating TMAO concentrations by modifying diet and modulating gut microbiota may be a safe and effective therapeutic target to delay CKD progression.
In conclusion, we found a significant positive dose-dependent association between circulating TMAO concentrations and increased all-cause mortality in non-dialysis and non-black dialysis CKD patients. Likewise, TMAO levels are positively correlated with the cardiovascular mortality risk in a dose-dependent manner in patients with non-black dialysis. However, more prospective studies are still needed to elucidate the relationship between TMAO concentration and mortality in CKD patients with different dialysis status and different races, to help make better clinical decisions.
Limitations
The current study has several limitations. First, because dialysis and non-dialysis patients were studied separately, the meta-analysis for each effect size included less than 10 articles, which may result in large errors in the publication bias. In addition, the loss of some data could increase publication bias. Second, the heterogeneity of studies in some subgroups remains high, and we cannot explain the full sources of heterogeneity. Third, there is too little data on the relationship between TMAO concentrations and the risk of cardiovascular mortality in non-dialysis patients, and the underlying diseases are relatively single, hence more researches are needed for a more reliable meta-analysis. Fourth, in dose-response meta-analyses, the death risk associated with the highest level of exposure is typically analyzed [Citation70]. However, as the highest exposure levels vary among studies, prospective studies with larger samples are needed to demonstrate values of TMAO concentrations that significantly increase the risk of death in CKD patients. Fifth, there are too few studies in the literature on the correlation between TMAO concentration and inflammation in dialysis patients to draw objective results, and because of this, subgroup analysis was not performed. In a word, these results should be interpreted with caution and treated as hypotheses generating.
Author contributions
YC. Li, HM. Lu and HJ. Zheng were responsible for the quality assessment of included articles. J. Guo and ML. Zhang were responsible for data extraction. YC. Li was responsible for data synthesis and analysis. When YC. Li and HM. Lu drafted the manuscript, YN. Liu and WJ. Liu were responsible for revising it critically for intellectual content. All authors participate in the research and agree to be accountable for all aspects of the work.
Supplemental Material
Download PDF (268.2 KB)Disclosure statement
No potential conflict of interest was reported by the author(s).
Data availability statement
The raw data supporting the conclusions of this article will be made available by the authors, without undue reservation.
Additional information
Funding
References
- Levin A, Tonelli M, Bonventre J, et al. Global kidney health 2017 and beyond: a roadmap for closing gaps in care, research, and policy. Lancet. 2017;390(10105):1–16.
- Bikbov B, Purcell CA, Levey AS, et al. Global, regional, and national burden of chronic kidney disease, 1990–2017: a systematic analysis for the global burden of disease study 2017. The Lancet. 2020;395(10225):709–733.
- Go AS, Chertow GM, Fan D, et al. Chronic kidney disease and the risks of death, cardiovascular events, and hospitalization. N Engl J Med. 2004;351(13):1296–1305.
- Huang Y, Xin W, Xiong J, et al. The intestinal microbiota and metabolites in the gut-kidney-heart axis of chronic kidney disease. Front Pharmacol. 2022;13:837500.
- Bennett B, Vallim T, Wang Z, et al. Trimethylamine-N-oxide, a metabolite associated with atherosclerosis, exhibits complex genetic and dietary regulation. Cell Metab. 2013;17(1):49–60. 10.1016/j.cmet.2012.12.011.
- Sumida K, Kovesdy CP. The gut-kidney-heart axis in chronic kidney disease. Physiol Int. 2019;106(3):195–206.
- Zhao J, Ning X, Liu B, et al. Specific alterations in gut microbiota in patients with chronic kidney disease: an updated systematic review. Ren Fail. 2021;43(1):102–112.
- Cosola C, Rocchetti MT, Sabatino A, et al. Microbiota issue in CKD: how promising are gut-targeted approaches? J Nephrol. 2019;32(1):27–37.
- Noce A, Marchetti M, Marrone G, et al. Link between gut microbiota dysbiosis and chronic kidney disease. Eur Rev Med Pharmacol Sci. 2022;26(6):2057–2074.
- Wu R, Ruan X-L, Ruan D-D, et al. Differences in gut microbiota structure in patients with stages 4-5 chronic kidney disease. Am J Transl Res. 2021;13(9):10056–10074.
- Koeth RA, Wang Z, Levison BS, et al. Intestinal microbiota metabolism of L-carnitine, a nutrient in red meat, promotes atherosclerosis. Nat Med. 2013;19(5):576–585.
- Tang WHW, Wang Z, Levison BS, et al. Intestinal microbial metabolism of phosphatidylcholine and cardiovascular risk. N Engl J Med. 2013;368(17):1575–1584.
- Xu K-Y, Xia G-H, Lu J-Q, et al. Impaired renal function and dysbiosis of gut microbiota contribute to increased trimethylamine-N-oxide in chronic kidney disease patients. Sci Rep. 2017;7(1):1445.
- Lau WL, Savoj J, Nakata MB, et al. Altered microbiome in chronic kidney disease: systemic effects of gut-derived uremic toxins. Clin Sci (Lond). 2018;132(5):509–522.
- Bain MA, Faull R, Fornasini G, et al. Accumulation of trimethylamine and trimethylamine-N-oxide in end-stage renal disease patients undergoing hemodialysis. Nephrol Dial Transplant. 2006;21(5):1300–1304.
- Boini KM, Hussain T, Li P-L, et al. Trimethylamine-N-oxide instigates NLRP3 inflammasome activation and endothelial dysfunction. Cell Physiol Biochem. 2017;44(1):152–162.
- Ma G, Pan B, Chen Y, et al. Trimethylamine N-oxide in atherogenesis: impairing endothelial self-repair capacity and enhancing monocyte adhesion. Biosci Rep. 2017;37(2):BSR20160244.
- Seldin MM, Meng Y, Qi H, et al. Trimethylamine N-oxide promotes vascular inflammation through signaling of mitogen-activated protein kinase and nuclear factor-κB. J Am Heart Assoc. 2016;5(2):e002767.
- Sun X, Jiao X, Ma Y, et al. Trimethylamine N-oxide induces inflammation and endothelial dysfunction in human umbilical vein endothelial cells via activating ROS-TXNIP-NLRP3 inflammasome. Biochem Biophys Res Commun. 2016;481(1-2):63–70.
- Zheng Y, Tang Z, You L, et al. Trimethylamine-N-oxide is an independent risk factor for hospitalization events in patients receiving maintenance hemodialysis. Ren Fail. 2020;42(1):580–586.
- Robinson-Cohen C, Newitt R, Shen DD, et al. Association of FMO3 variants and trimethylamine N-oxide concentration, disease progression, and mortality in CKD patients. PLoS One. 2016;11(8):e0161074.
- Wells G, Shea B, O’Connell D, et al. The Newcastle-Ottawa Scale (NOS) for assessing the quality of nonrandomized studies in meta-analyses. [Internet]. Ottawa: Ottawa Hospital Research Institute; 2000. [Cited 2020 Dec 31]. Available from: http://www.ohri.ca/programs/clinical_epidemiology/oxford.asp.
- Rostom A, Dubé C, Cranney A, et al. Celiac disease (evidence reports/technology assessments, No. 104). Appendix D. Quality assessment forms. [Internet]. Rockville (MD): Agency for Healthcare Research and Quality; 2004. [Cited 2020 Dec 31]. Available from: https://www.ncbi.nlm.nih.gov/books/NBK35156/.
- Cooper H, Hedges LV, editors. Handbook of research synthesis. New York (NY): Russell Sage Foundation; 1994.
- Riley RD, Higgins JPT, Deeks JJ. Interpretation of random effects meta-analyses. BMJ. 2011;342:d549.
- Julian PT Higgins and Sally Green. Cochrane handbook for systematic reviews of interventions. Version 5.1.0. Vol. 2011;2:126–130. editor
- Tang WHW, Wang Z, Kennedy DJ, et al. Gut microbiota-dependent trimethylamine N-oxide (TMAO) pathway contributes to both development of renal insufficiency and mortality risk in chronic kidney disease. Circ Res. 2015;116(3):448–455.
- Kaysen GA, Johansen KL, Chertow GM, et al. Associations of trimethylamine N-oxide with nutritional and inflammatory biomarkers and cardiovascular outcomes in patients new to dialysis. J Ren Nutr. 2015;25(4):351–356.
- Stubbs JR, House JA, Ocque AJ, et al. Serum trimethylamine-N-oxide is elevated in CKD and correlates with coronary atherosclerosis burden. J Am Soc Nephrol. 2016;27(1):305–313.
- Missailidis C, Hällqvist J, Qureshi AR, et al. Serum trimethylamine-N-oxide is strongly related to renal function and predicts outcome in chronic kidney disease. PLoS ONE. 2016;11(1):e0141738.
- Mafune A, Iwamoto T, Tsutsumi Y, et al. Associations among serum trimethylamine-N-oxide (TMAO) levels, kidney function and infarcted coronary artery number in patients undergoing cardiovascular surgery: a cross-sectional study. Clin Exp Nephrol. 2016;20(5):731–739.
- Kim RB, Morse BL, Djurdjev O, et al. Advanced chronic kidney disease populations have elevated trimethylamine N-oxide levels associated with increased cardiovascular events. Kidney Int. 2016;89(5):1144–1152.
- Al-Obaide MAI, Singh R, Datta P, et al. Gut microbiota-dependent trimethylamine-N-oxide and serum biomarkers in patients with T2DM and advanced CKD. J Clin Med. 2017;6(9):86.
- Gruppen EG, Garcia E, Connelly MA, et al. TMAO is associated with mortality: impact of modestly impaired renal function. Sci Rep. 2017;7(1):13781.
- Zhang L, Xie F, Tang H, et al. Gut microbial metabolite TMAO increases peritoneal inflammation and peritonitis risk in peritoneal dialysis patients. Transl Res. 2022;240:50–63.
- Zhang P, Zou J-Z, Chen J, et al. Association of trimethylamine N-oxide with cardiovascular and all-cause mortality in hemodialysis patients. Ren Fail. 2020;42(1):1004–1014.
- Fu D, Shen J, Li W, et al. Elevated serum trimethylamine N-oxide levels are associated with mortality in male patients on peritoneal dialysis. Blood Purif. 2021;50(6):837–847.
- Chang D, Xu X, Yang Z, et al. Trimethylamine-N-oxide (TMAO) and clinical outcomes in patients with end-stage kidney disease receiving peritoneal dialysis. Perit Dial Int. 2022;42(6):622–630.
- Shafi T, Powe NR, Meyer TW, et al. Trimethylamine N-oxide and cardiovascular events in hemodialysis patients. J Am Soc Nephrol. 2017;28(1):321–331.
- Stubbs JR, Stedman MR, Liu S, et al. Trimethylamine N-oxide and cardiovascular outcomes in patients with ESKD receiving maintenance hemodialysis. Clin J Am Soc Nephrol. 2019;14(2):261–267.
- Sapa H, Gutiérrez OM, Shlipak MG, et al. Association of uremic solutes with cardiovascular death in diabetic kidney disease. Am J Kidney Dis. 2022;80(4):502–512.e1.
- Winther SA, Øllgaard JC, Tofte N, et al. Utility of plasma concentration of trimethylamine N-oxide in predicting cardiovascular and renal complications in individuals with type 1 diabetes. Diabetes Care. 2019;42(8):1512–1520.
- Winther SA, Øllgaard JC, Hansen TW, et al. Plasma trimethylamine N-oxide and its metabolic precursors and risk of mortality, cardiovascular and renal disease in individuals with type 2-diabetes and albuminuria. PLoS One. 2021;16(3):e0244402.
- Hernandez L, Ward LJ, Arefin S, et al. Blood-brain barrier and gut barrier dysfunction in chronic kidney disease with a focus on circulating biomarkers and tight junction proteins. Sci Rep. 2022;12(1):4414.
- El-Deeb OS, Atef MM, Hafez YM. The interplay between microbiota-dependent metabolite trimethylamine N-oxide, transforming growth factor β/SMAD signaling and inflammasome activation in chronic kidney disease patients: a new mechanistic perspective. J Cell Biochem. 2019;120(9):14476–14485.
- Li D, Lu Y, Yuan S, et al. Gut microbiota-derived metabolite trimethylamine-N-oxide and multiple health outcomes: an umbrella review and updated meta-analysis. Am J Clin Nutr. 2022;116(1):230–243.
- Zeng Y, Guo M, Fang X, et al. Gut microbiota-derived trimethylamine N-oxide and kidney function: a systematic review and meta-analysis. Adv Nutr. 2021;12(4):1286–1304.
- Zhou Z, Jin H, Ju H, et al. Circulating trimethylamine-N-oxide and risk of all-cause and cardiovascular mortality in patients with chronic kidney disease: a systematic review and meta-analysis. Front Med. 2022;9:828343.
- Gupta N, Buffa JA, Roberts AB, et al. Targeted inhibition of gut microbial trimethylamine N-Oxide production reduces renal tubulointerstitial fibrosis and functional impairment in a murine model of chronic kidney disease. Arterioscler Thromb Vasc Biol. 2020;40(5):1239–1255.
- Zhang W, Miikeda A, Zuckerman J, et al. Inhibition of microbiota-dependent TMAO production attenuates chronic kidney disease in mice. Sci Rep. 2021;11(1):518.
- Kapetanaki S, Kumawat AK, Persson K, et al. The fibrotic effects of TMAO on human renal fibroblasts is mediated by NLRP3, caspase-1 and the PERK/akt/mTOR pathway. Int J Mol Sci. 2021;22(21):11864.
- Din AU, Hassan A, Zhu Y, et al. Amelioration of TMAO through probiotics and its potential role in atherosclerosis. Appl Microbiol Biotechnol. 2019;103(23-24):9217–9228.
- Janeiro MH, Ramírez MJ, Milagro FI, et al. Implication of trimethylamine N-oxide (TMAO) in disease: potential biomarker or new therapeutic target. Nutrients. 2018;10(10):1398.
- Yang S, Li X, Yang F, et al. Gut microbiota-dependent marker TMAO in promoting cardiovascular disease: inflammation mechanism, clinical prognostic, and potential as a therapeutic target. Front Pharmacol. 2019;10:1360.
- Zhang L, Nie J, Zhang X. Fo044gut microbiota metabolite trimethylamine-N-oxide aggravates peritoneal inflammation via activating nlrp3-caspase 1 pathway. Nephrol Dial Transplant. 2019;34(Supplement_1):i20–i22.
- Xie Y, Hu X, Li S, et al. Pharmacological targeting macrophage phenotype via gut-kidney axis ameliorates renal fibrosis in mice. Pharmacol Res. 2022;178:106161.
- Lai Y, Tang H, Zhang X, et al. Trimethylamine-N-oxide aggravates kidney injury via activation of p38/MAPK signaling and upregulation of HuR. Kidney Blood Press Res. 2022;47(1):61–71.
- Zhu W, Gregory JC, Org E, et al. Gut microbial metabolite TMAO enhances platelet hyperreactivity and thrombosis risk. Cell. 2016;165(1):111–124.
- Makrecka-Kuka M, Volska K, Antone U, et al. Trimethylamine N-oxide impairs pyruvate and fatty acid oxidation in cardiac mitochondria. Toxicol Lett. 2017;267:32–38.
- Ku E, Yang W, McCulloch CE, et al. Race and mortality in CKD and dialysis: findings from the chronic renal insufficiency cohort (CRIC) study. Am J Kidney Dis. 2020;75(3):394–403.
- Mitchell SM, Milan AM, Mitchell CJ, et al. Protein intake at twice the RDA in older men increases circulatory concentrations of the microbiome metabolite Trimethylamine-N-Oxide (TMAO). Nutrients. 2019;11(9):2207.
- Mafra D, Black Veiga AP, Saraiva dos Anjos J, et al. Mp449effect of low protein diet on trimethylamine-N-oxide plasma levels in non-dialysis chronic kidney disease patients. Nephrol Dial Transplant. 2017;32(suppl_3):iii593–iii594.
- Borges NA, Stenvinkel P, Bergman P, et al. Effects of probiotic supplementation on trimethylamine-N-Oxide plasma levels in hemodialysis patients: a pilot study. Probiotics Antimicrob Proteins. 2019;11(2):648–654.
- Wang S, Lv D, Jiang S, et al. Quantitative reduction in short-chain fatty acids, especially butyrate, contributes to the progression of chronic kidney disease. Clin Sci. 2019;133(17):1857–1870.
- Liu J, Lai L, Lin J, et al. Ranitidine and finasteride inhibit the synthesis and release of trimethylamine N-oxide and mitigates its cardiovascular and renal damage through modulating gut microbiota. Int J Biol Sci. 2020;16(5):790–802.
- Ji C, Li Y, Mo Y, et al. Rhubarb enema decreases circulating trimethylamine N-oxide level and improves renal fibrosis accompanied with gut microbiota change in chronic kidney disease rats. Front Pharmacol. 2021;12:780924.
- Liu X, Tan Z, Huang Y, et al. Relationship between the triglyceride-glucose index and risk of cardiovascular diseases and mortality in the general population: a systematic review and meta-analysis. Cardiovasc Diabetol. 2022;21(1):124. Jul 1
- Hill E, Sapa H, Negrea L, et al. Effect of oat β-glucan supplementation on chronic kidney disease: a feasibility study. J Ren Nutr. 2020;30(3):208–215.
- Yang W, Zhang S, Zhu J, et al. Gut microbe-derived metabolite trimethylamine N-oxide accelerates fibroblast-myofibroblast differentiation and induces cardiac fibrosis. J Mol Cell Cardiol. 2019;134:119–130.
- Integrative HMP (iHMP) Research Network Consortium. The integrative human microbiome project. Nature. 2019;569(7758):641–648.