Abstract
Purpose
Astragaloside IV (AS-IV) is a natural saponin substance extracted from the plant Radix Astragali with anti-inflammatory, antioxidant, anti-apoptotic, and liver-protecting effects. This study was to evaluate the liver protection effect of AS-IV on mice after acute alcohol stimulation.
Materials and Methods
Mice were orally administrated with AS-IV (50, 150, and 500 mg/kg, respectively), and sodium carboxymethyl cellulose (CMC, 50 mg/kg) daily for 7 days, before giving five alcohol-intragastric injections.
Results
Results suggested that the levels of serum ALT and AST, liver SOD, GSH-PX, 4-HNE, and MDA, serum and liver TNF-α, IL-1β, and IL-6, serum lipopolysaccharide (LPS), lipopolysaccharide binding protein (LBP), diamine oxidase (DAO) and Myeloperoxidase (MPO), the mRNA and protein expression of hepatic NLRP3, Caspase-1, IL-1β, and IL-18 were significantly decreased in AS-IV-treated mice compared with the model group. Moreover, the effect of AS-IV on histopathology of liver tissue confirmed its protective function. Furthermore, AS-IV ameliorated the gut microbiota imbalance and adjusted the abundance of the following dysfunctional bacteria closer to the control group: Butyricicoccus, Turicibacter, Akkermansia, Anaerotruncus, and Mucispirillum. A strong correlation between intestinal bacteria and potential biomarkers was found.
Conclusion
Together, our findings indicated that AS-IV exert the hepatoprotective effect by modulating the gut microbiota imbalance and regulating NLRP3/Caspase-1 signaling pathway.
KEY MESSAGE
Astragaloside IV alleviated liver dysfunction during alcohol-induced liver injury.
Astragaloside IV inhibited LPS, LBP, and DAO translocation in the intestine.
Astragaloside IV attenuated liver dysfunction in mice by modulating gut microbiota and inhibiting NLRP3/Caspase-1 signaling pathway.
Introduction
Excessive alcohol intake has become a global healthcare concern. Globally, about 3.3 million people die of alcoholism every year, accounting for 6% of global deaths [Citation1]. The liver is usually the earliest and the most damaged organ induced by excessive drinking due to it is the main part of alcohol metabolism [Citation2]. Excessive alcohol intake can lead to alcoholic liver diseases (ALD) and progress to fatty liver, alcoholic hepatitis, liver fibrosis, and even alcoholic liver cirrhosis and liver cancer [Citation1]. In recent years, the morbidity of ALD is gradually increasing, and it has become the second major cause of liver injury after viral hepatitis [Citation3]. However, the therapeutic effect of ALD is poor, and there is no effective drug in the clinic.
Emerging evidence suggested that the gut microbiota–gut–liver axis is crucial for alcohol-induced liver inflammation [Citation4]. Disorder of the gut microbiota caused by alcohol can generate various pathogen associated molecular patterns (PAMP), such as lipopolysaccharide (LPS), flagellin, peptidoglycan, and microbial nucleic acids, and increase the permeability of intestinal mucosa, which can cause liver inflammation when these substances enter the blood from the intestinal cavity [Citation5,Citation6]. Previous studies have confirmed that the abundance and composition of gut microbiota in patients with ALD have altered [Citation7]. The exact association between the change of the gut microbiota and ALD pathogenesis is unknown yet and thereby warrants attentions as a key target for prevention and treatment of this disease. Growing evidence has suggested that inflammasomes promote inflammation, and chronic inflammatory responses triggered by a variety of immune cells also play a central role in alcohol-induced liver inflammation [Citation8,Citation9]. Although there are different types of inflammasomes, most studies so far have focused on the nucleotide-binding domain-like receptor protein 3 (NLRP3) in the pathogenesis of acute or chronic liver disease. NLRP3 inflammasomes are macromolecular complexes formed by the oligomerization of the NLRP3 receptors, consisting of NLRP3, apoptotic speck protein (ASC), and pro-caspase-1 [Citation2], which play a vital role in innate immune sensing. When stimulated by LPS or peptidoglycan, the NLRP3 inflammasome promotes activation of caspase-1, followed by the conversion of pro-interleukin-1β (IL-1β) and pro-IL-18 into their mature bioactive forms [Citation10]. Mature IL-1β and IL-18 are then released to extracellular space, and lead to liver inflammation [Citation9].
Astragaloside IV (AS-IV), a natural saponin component isolated from a traditional Chinese medicinal plant Radix astragali, has been reported to exert anti-inflammatory, anti-aging, antioxidant, anti-apoptotic, metabolic regulation, and liver-protecting effects [Citation11]. To date, many studies have shown that AS-IV possesses potent protective effects on liver, cardiovascular system, lung, kidney, and brain [Citation11]. Liu et al. reported that AS-IV improved high-fat diet-induced hepatic steatosis by regulating inflammatory factors level by TLR4/NF-κB signaling pathway [Citation12]. AS-IV has also been reported to effectively attenuate chronic alcoholic fatty liver disease by suppressing oxidative stress [Citation13]. The metabolism of AS-IV by gut microbiota was a crucial step in the disposition and therapeutic effects of AS-IV [Citation11]. Xu et al. found that AS-IV alleviated the autophagy and oxidative stress caused by the intestinal flora in mice with acute ischaemic stroke [Citation14]. AS-IV also modulated the abundance and diversity of gut microbiota in type 2 diabetes mellitus mice, and improved butyric acid levels [Citation15]. However, the potential mechanism of AS-IV in the amelioration of acute alcohol-induced liver injury and restoration of gut microbiota remains unknown and needs to be elucidated.
In the present study, we evaluated the effects of AS-IV by the establishment of a mice model of acute alcohol-induced liver injury and further explored the possible mechanisms related to the regulation of gut microbiota and NLRP3/Caspase-1 signaling pathways. Our study provided a theoretical basis for the further development of AS-IV new drugs for the prevention and treatment of ALD.
Materials and methods
Chemicals and reagents
AS-IV, anhydrous ethanol and carboxymethyl cellulose (CMC) were purchased from Shanghai Acmec Biochemical Co., Ltd. (Shanghai, China). Alanine aminotransferase (ALT), aspartate aminotransferase (AST), superoxide dismutase (SOD), glutathione peroxidase (GSH-PX), and malondialdehyde (MDA) assay kit were obtained from Nanjing JianCheng Bioengineering Institute (Nanjing, China). LPS, lipopolysaccharide binding protein (LBP), diamine oxidase (DAO), TNF-α, IL-6, L-1β, 4-Hydroxynonenal (4-HNE), and Myeloperoxidase (MPO) Ienzyme linked immunosorbent assay (ELISA) kits were purchased from Jiangsu Meimian Industrial Co., Ltd (Jiangsu, China). TRIzol Reagent, PrimeScript™ RT Master Mix reagent kit, and TB Green® Premix Ex Taq™II (Tli RNaseH Plus) were supplied from Takara (Beijing, China). RIPA Lysis Buffer and BCA Protein Assay Kit were gained from Beyotime Institute of Biotechnology (Jiangsu, China). Anti-body (NLRP3: cat.#AF7438, pro-caspase-1: cat.#AF5418, Cleaved-Caspase-1: cat.#AF4022) were purchased from Affinity Biosciences (Jiangsu, China). Anti-body (GAPDH: Cat NO. 10494-1-AP, IL-1β: Cat NO. 29530-1-AP, IL-18: Cat NO. 10663-1-AP, Claudin 4: Cat NO. 16195-1-AP, Occludin: Cat NO. 13409-1-AP, F4/80: Cat NO. 29414-1-AP) and HRP-conjugated Affinipure Goat Anti-Rabbit IgG (H + L) (Cat NO. SA00001-12) were supplied from Proteintech Group, Inc. (Chicago, USA).
Animals and experimental design
Fifty male Kunming mice (24–26 g) were purchased from the Southern Medical University Animal Center (Guangdong, China). All mice were maintained in a standard environment (23 ± 1 °C, 50 ± 5% relative humidity, a 12 h light/dark cycle) with free access to food and water during the experiment. After a week of acclimation, the mice were randomly divided into five groups (n = 10): control, model, AS-IV-L (50 mg kg−1), AS-IV-M (150 mg kg−1), and AS-IV-H (500 mg kg−1). Mice in AS-IV treated groups were given different dose of AS-IV (dissolve in 0.5% CMC) by gavage once daily successively for 7 days, while the control and model mice received the same volume of 0.5% CMC. After the last administration, the mice except the control group were intragastrically given 50% ethanol solution (12 mL/kg) every 12 h five times [Citation16,Citation17].
All the mice were fasted, but with free access to water for 9 h, then anesthetized with pentobarbital and sacrificed for subsequent assays. The body weight was measured every day. Fresh fecal samples were collected from each mouse before dissection, placed into 2 mL sterile tubes, and stored at −80 °C. All fecal, blood, and liver samples were collected and stored at −80 °C. The protocol was approved by the Institutional Animal Care and Use Committee of Guangdong Pharmaceutical University (gdpulac2022120).
Biochemical assays of serum and liver samples
ALT and AST activities as liver function indices were measured using ALT and AST activity assay kits. The OD value was measured and recorded with a microplate reader (BIO-RAD xMark, USA) at 450 nm (AST) or 570 nm (ALT). The contents of LPS, LBP, and DAO in serum and the levels of inflammatory factors, such as TNF-α, IL-1β, IL-6, and MPO in serum and liver tissues were determined using the corresponding ELISA kits. Absorbance at 450 nm was recorded. All assays were performed according to the manufacturers’ instructions.
Histopathological and immunohistochemical analysis
The mice liver were removed and fixed in 4% paraformaldehyde for 48 h, washed in PBS, and eluted with alcohol gradient elution. After dehydration, they were embedded in paraffin according to standard methods. To observe the damage of hepatocytes, the paraffin-embedded tissues were made into 5 μm thick tissue sections, followed by staining with haematoxylin and eosin (H&E). The changes in histopathology were observed under the light microscope (IX73, Olympus).
To investigate the inflammation in the liver, immunohistochemical staining was performed with anti-F4/80 antibody. liver sections were deparaffinized with xylene, rehydrated in alcohol gradient, and then immersed in 3% H2O2. Sections were blocked with serum for 30 min and incubated overnight with primary antibody at 4 °C. The secondary antibody was incubated at room temperature for 1 h and then was restained with hematoxylin. The sections were viewed and images were captured using a Nikon microscope (Nikon, Melville, NY, USA).
Determination of the liver antioxidant capacity
The activities of liver SOD and GSH-Px are used as the indices to measure the liver antioxidant capacity. 4-HNE is one of the end products of lipid peroxidation and is often used as an indicator of lipid peroxidation. MDA reflects the degree of lipid peroxidation damage to cell membrane. The liver tissues were weighed accurately and prepared into 10% tissue homogenate by adding nine times the volume of physiological saline, and the supernatant was obtained by centrifugation at 3000 rpm and 4 °C for 15 min. Liver SOD activity was measured using a water-soluble tetrazole-1 colorimetric (WST-1) method at 450 nm absorbance. The activity of GSH-PX is expressed by the reaction rate of catalyzing GSH, and calculated by determining the consumption of GSH, and the results are expressed in units of per mg protein (U/mg protein). The GSH level was detected by measuring the absorbance of 2-nitro-5-thiobenzoic acid at 412 nm. The 4-HNE was determined using a double antibody one-step sandwich enzyme-linked immunosorbent assay at 450 nm wavelength with an enzyme-labeled instrument. The MDA level of the liver was measured by thiobarbituric acid test at 450 nm absorbance. All assays were conducted according to the manufacturers’ instructions.
Quantitative real-time PCR (qPCR) assay
Total RNA was extracted from liver tissue using Trizol reagent (Takara Bio Inc, Beijing, China) according to the manufacturer’s instructions, and the concentration and quality of mRNA were measured using a Nano-Drop 1000 spectrophotometer (Thermo Scientific, USA). PrimeScript RT Master Mix kit was used for the reverse transcription of mRNA into cDNA. The mRNA expression levels of NLRP3, pro-Caspase1, Caspase-1, IL-1β, and IL-18 were determined by qPCR using TB Green® Premix Ex TaqTMII (Takara Bio Inc, Beijing, China). The amplification reaction conditions were as follows: initial activation at 95 °C for 30 s, followed by 40 cycles of denaturation at 95 °C for 5 s and 60 °C for 31 s, and amplification at 95 °C for 15 s and annealing at 60 °C for 60 s. The relative expression of mRNA was calculated using the 2−ΔΔCT method with the internal reference values of 18s. The primers used in the experiment are shown in .
Table 1. Primer sequence of target gene.
Western blot
Total protein was extracted from liver tissue by RIPA Lysis Buffer containing Phenyl methane sulfonyl fluoride (PMSF) (Beyotime Institute of Biotechnology, Shanghai, China). Protein content was detected by the BCA Protein Assay Kit (Beyotime Institute of Biotechnology, Shanghai, China). Proteins were separated by 10% SDS-PAGE gel electrophoresis and transferred to polyvinylidene difluoride (PVDF) membranes. The blotting membranes were blocked in 5% skim milk for 2 h and then incubated with the corresponding primary antibodies, such as NLRP3 (1:1000), Pro-Caspase-1 (1:1000), IL-1β (1:1000), IL-18 (1:2500), Cleaved-Caspase-1 (1:1000), Claudin 4 (1:750), Occludin (1:8000), F4/80 (1:500), and GAPDH (1:30,000) for 12 h at 4 °C in a shaker. The membranes were washed six times with TBS/Tween Buffer (Epizyme Biomedical Technology, Shanghai, China) for 5 min each, followed by incubation with secondary antibody HRP-conjugated Affinipure Goat Anti-Rabbit IgG (H + L) (1:6000) at room temperature for 1 h. Western blots (WB) were visualized using an electrochemiluminescence system (Tanon, Shanghai, China), and grayscale values were analyzed by Image J software (version 1.8.0, National Institutes of Health, USA).
16S rRNA sequencing and gut microbiota analysis
Total DNA was extracted from the feces using the Cetyltrimethylammonium Bromide(CTAB) method, and the quality of DNA was examined using 1% agarose gel electrophoresis. For each sample, the V3–V4 region of the bacterial 16S ribosomal RNA gene was amplified with the 341 F/806R primer (341 F: 5′-CCTAYGGGRBGCASCAG-3′; 806 R: 5′-GGACTACNNGGGTATCTAAT-3′) and sequenced on the Illumina NovaSeq platform (Illumina, San Diego, CA, USA) by Shenzhen Microbial Technology Co., Ltd (Shenzhen, China). After quality control, pruning, de-noising, splicing, and removal of chimeras, the original base sequences were obtained from the original reads, and all bioinformatics analysis was performed with reference to Qiime 2. Compared with the Greenones Database (version 13.8), the resulting sequences were then clustered into operational taxonomic units (OTUs) with 1% difference (99% similarity). Alpha (α) diversity is used to assess the richness and diversity of the microbiota within an individual, beta (β) diversity is conducted using principal component analysis (PCoA) to evaluate the similarity of microbial composition among individuals. Linear discriminant analysis (LDA) effect size (LEfSe) is used to identify species with significant differences in abundance among groups and LDA score > 3, p < 0.05, and FDR < 0.1 indicated significant differences. Spearman rank correlation analysis was performed to assess the correlation between related environmental factors and microbial species, and heat map was used to display it.
Statistical analysis
The results were expressed as mean ± standard error of mean (SEM). One-way ANOVA and Tukey’s tests were used to distinguish the statistical significance of more than three groups of data. A two-tailed students t-test was used to evaluate the statistical differences between two independent groups. A two-tailed p < 0.05 was considered statistically significant. All statistical analyses were performed using SPSS software (version 23, SPSS, Inc., USA), and all figures were drawn on GraphPad Prism (version 8.0, GraphPad Software, Inc., USA).
Results
AS-IV improved liver function and alleviated liver injury in mice with acute alcohol-induced liver injury
During the treatment, the changes in body weight gain and relative weight of liver in mice are shown in . The body weight gain in the AS-IV-H group was significantly lower than that in the model group (, p < 0.05). The liver index was increased significantly in the model group compared with control group (, p < 0.05), but AS-IV treatment did not prevent these increases (, p > 0.05). ALT and AST levels are important criteria to measure liver function. The serum ALT and AST levels in the model group were significantly higher than those in the control group (p < 0.05), while AS-IV decreased the serum ALT and AST levels of mice under alcohol treatment (p < 0.05, ). In addition, the liver pathology sections indicated obvious cellular degeneration, hepatocyte necrosis, and nucleus pycnosis in the model group than those in the control group, while AS-IV treatment reversed alcohol-induced severe liver injury (). These results indicated that AS-IV could reduce the pathological damage of liver and improved liver function.
Figure 1. AS-IV improved liver function and alleviated liver injury in mice with acute alcohol-induced liver injury (n = 5–10). (A) Body weight gain. (B) Liver index. (C) Serum levels of ALT. (D) Serum levels of AST. (E) H&E staining of liver tissue samples (magnification, 200×, the arrows indicate cell degeneration, hepatocyte necrosis, and nuclear necrosis). The data are represented as means ± SEM. #p < 0.05 vs. control group, *p < 0.05 and **p < 0.01 vs. model group. ns - no significance.
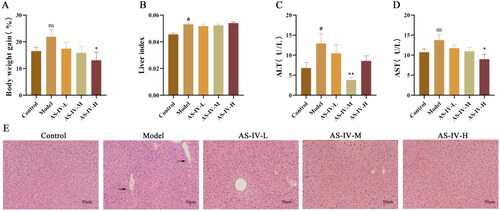
AS-IV ameliorates alcohol-induced hepatic oxidative stress damage in mice with acute alcohol-induced liver injury
The levels of oxidative stress are important indices to evaluate the degree of alcoholic liver injury. As shown in , SOD activities and the GSH-PX content in the liver were inhibited in model group compared with control group (p < 0.05), but AS-IV treatment prevented these decreases (p < 0.05). The levels of 4-HNE and MDA in the liver were increased in model group compared with control group (p < 0.001), but AS-IV treatment prevent increases of 4-HNE level (p < 0.001), and low-dose AS-IV treatment prevent increases of MDA level (p < 0.05, ). Compared with model group, different doses of AS-IV significantly inhibited oxidation stress to various degrees in the AS-IV treated group.
Figure 2. AS-IV alleviates oxidative stress in the liver tissue of ALD mice (n = 9). (A–D) The markers of oxidative stress are SOD, GSH-Px, 4-HNE, and MDA. The data are represented as means ± SEM. #p < 0.05, ##p < 0.01, and ###p < 0.001 vs. control group, *p < 0.05, **p < 0.01, and ***p < 0.001 vs. model group.
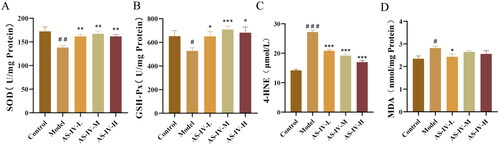
AS-IV attenuated serum and hepatic inflammation in mice with acute alcohol-induced liver injury
Acute alcohol stimulation leads to increased production of Kupffer cells and neutrophils, causes systemic and hepatic inflammation. F4 is an important marker of Kupfer cells. MPO is an important mediator of neutrophil release. TNF-α, IL-1β, and IL-6 are important inflammatory factors that induce host inflammation. As shown in , the results of immunohistochemical staining showed that F4/80 has obvious differences among control, model, and AS-IV treatment group. Western blotting results revealed that acute alcohol stimulation significantly increased the protein levels of F4/80 (p < 0.05), but low and high dose AS-IV treatment can prevent these increases (p < 0.05). The mice in the model group had higher levels of TNF-α, IL-1β, IL-6, and MPO in serum and liver tissue compared with those in the control group (, p < 0.05). AS-IV treatment could significantly decreased the serum and hepatic TNF-α, IL-1β, IL-6, and MPO levels (p < 0.05).
Figure 3. AS-IV reduced the Inflammation in serum and liver tissue of ALD mice (n = 5–10). (A) The expression of F4/80 was detected by immunohistochemical staining (Brown color means positive staining) and western blotting, and presented as fold change relative to control. (B–E) concentration of serum TNF-α, IL-1β, IL-6, and MPO. (F–I) concentration of liver tissue TNF-α, IL-1β, IL-6, and MPO. The data are represented as means ± SEM. #p < 0.05, ##p < 0.01, and ###p < 0.001 vs. control group, *p < 0.05, **p < 0.01, and ***p < 0.001 vs. model group.
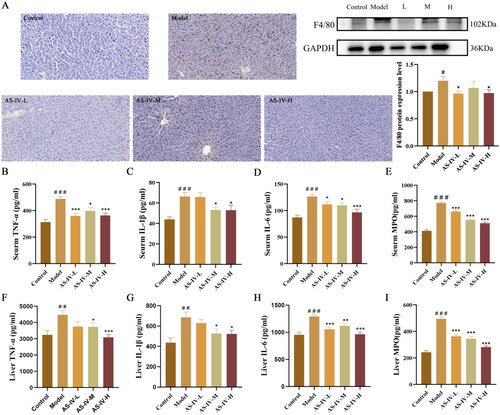
AS-IV ameliorated intestinal leakage and endotoxemia in mice with acute alcohol-induced liver injury
The levels of LPS, LBP, and DAO in blood can reflect the degree of intestinal leakage. As shown in , the levels of LPS, LBP, and DAO in serum substantially increased after acute alcohol stimulation (p < 0.05), but AS-IV administration significantly decreased their levels. As shown in , acute alcohol stimulation significantly decreased the expression of tight junction proteins including Occludin and Claudin 4 in small intestines compared with the control group, but AS-IV treatment can prevent these decreases.
Figure 4. AS-IV reduced the serum levels of DAO, LPS, and LBP and improved intestinal barrier functions. (n = 6–8). (A–C) serum DAO, LPS, and LBP levels. (D–F) protein expression of Occludin and Claudin 4 in the small intestine was detected by western blotting, and presented as fold change relative to control. The data are represented as means ± SEM. ##p < 0.01 and ###p < 0.001 vs. control group, *p < 0.05, **p < 0.01, and ***p < 0.001 vs. model group.
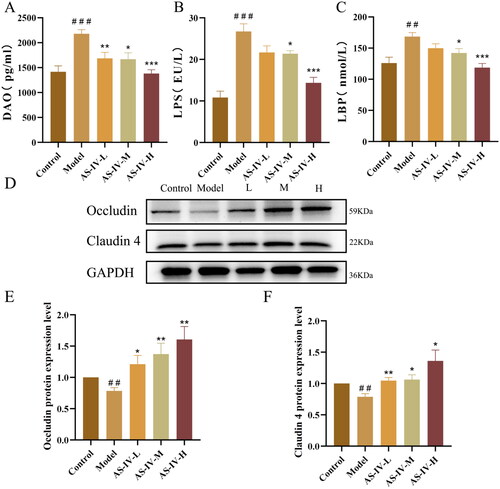
AS-IV modulated gut microbiota in mice with acute alcohol-induced liver injury
Gut microbiota changes are an important environmental factor that determines the occurrence of ALD [Citation4]. An OTU is considered as a species, and the same or unique OTUs among the different samples were calculated based on the abundance of OTU in each sample [Citation18]. The Venn diagram showed that the total OTUs of control, model, AS-IV-L, AS-IV-M, and AS-IV-H group were 1330, 1172, 1504, 1019, and 1238, respectively (). A total of 334 OTUs were shared among the five groups. UniFrac distance-based PCoA was used to assess the similarity and difference of microbial evolution among different samples. As shown in , both weighted and unweighted UniFrac analyses presented that the point distance among the five groups was close, indicating that the microbial structure composition of the samples was similar but slightly different. The composition differences of the intestinal flora among the five groups were further analyzed (). At the phylum level, Bacteroidetes, Firmicutes, and Proteobacteria were found to be the dominant flora of the five groups. The relative abundance of Bacteroidetes in control, model, AS-IV-L, AS-IV-M, and AS-IV-H was (67.68 ± 10.16), (62.46 ± 4.79), (59.68 ± 11.38), (53.69 ± 13.78), and (55.83 ± 20.79)%, respectively, without statistical differences among them. There were also no statistical differences in the relative abundance of Firmicutes and Proteobacteria among five groups. At the genus level, the dominant flora of the five groups were Bacteroides, Lactobacillus, and Prevotella (). The relative abundance of Bacteroides in control, model, AS-IV-L, AS-IV-M, and AS-IV-H was (10.70 ± 4.84), (10.42 ± 5.82), (11.43 ± 4.36), (7.05 ± 3.07), and (7.20 ± 5.35)%, respectively, without statistical differences among them. There were also no statistical differences in the relative abundance of Lactobacillus and Prevotella among the five groups.
Figure 5. AS-IV affects the composition of gut microbiota in mice with acute alcohol-induced liver injury (n = 5). (A) Venn analysis of bacterial OTUs composition between Control, Model, AS-IV-L, AS-IV-M, and AS-IV-H groups. (B,C) Principal component analysis based on weighted and unweighted UniFrac. (D) Distribution of intestinal flora at phylum and genus level, respectively.
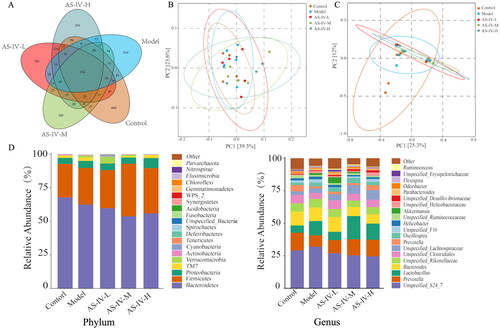
To identify the specific bacterial taxa related to improved acute alcohol-induced liver injury following AS-IV treatment, we compared the intestinal flora for the five groups on the genus level by Lefse analysis (). As shown in , the dominant bacteria in the control group were Phascolarctoobacterium, Enterobacter, Turicibacter, Acetobacter, and Butyricicoccus, while for the model group the dominant bacteria were Verrucomicrobia, Deferribacteres, Akkermansia, Anaerotruncus, Mucispirillum, and Ralstonia, indicating that the gut symbiosis was interrupted in acute alcohol treated mice. In the AS-IV group, the gut microbiota significantly increased in Turicibacter (AS-IV-L), Butyricicoccus (AS-IV-L and AS-IV-M), Akkermansia (AS-IV-L), and decreased in Anaerotruncus (AS-IV-L), Mucispirillum (AS-IV-H) ().
Figure 6. The Lefse analysis among five groups (LDA score > 3, p < 0.05, FDR < 0.1). (A) cladogram in control and model groups at each classification level (Phylum, Class, Order, Family, Genus). (B) LDA results between control and model groups at each classification level (Phylum, Class, Order, Family, Genus). (C) LDA results in all groups at genus level. (D-H) The significantly changed bacteria at the genus level based on LDA results in all groups.
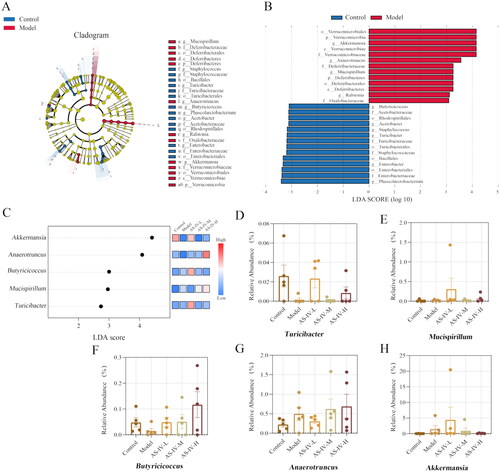
Relationship between related environmental factors and gut microbiota
The relationships between liver and serum inflammatory factors, endotoxemia, and gut microbiota were analyzed using Spearman’s correlation coefficient (). At the phylum level (), Verrucomicrobia was significantly correlated with LPS, DAO, and IL-6 in serum, Deferribacteres was positively associated with LPS, IL-1β, TNF-α in serum, and IL-1β in liver, Spirochaetes and Turicibacter was positively related to liver IL-1β. At the genus level (), Mucispirillum was positively correlated with LPS, IL-1β, TNF-α in serum, and IL-1β in liver, Ralstonia was related to serum IL-6, Anaerotruncus and Helicobacter was positively associated with serum TNF-α, Parabacteroides was correlated with liver IL-1β, Ruminococcaceae was correlated with serum IL-1β. AF12 was inversely related to serum LPS, LBP, DAO, IL-1β, and IL-6, Butyricicoccus, Coprococcus, and Clostridium were negatively associated with serum IL-6. The correlation results indicated that host inflammatory level, endotoxemia, and gut microbiota in the AS-IV-treated environment constitute an interactive relationship.
AS-IV regulated the activation of LPS-mediated NLRP3/caspase-1 signaling pathway in the livers of alcohol-treated mice
In the alcohol-induced liver injury model, inflammatory factors are often abnormal, and the NLRP3 inflammasome is often regarded as an important signaling factor in the inflammatory response [Citation19]. Considering IL-1β and IL-18 are products of activated NLRP3 inflammasome, the effect of AS-IV on these two pro-inflammatory interleukins at both mRNA and protein levels were further detected (). As shown in , high mRNA expression levels of NLRP3, caspase-1, and IL-1β were found in the livers of only alcohol treated mice compared with control group (p < 0.05). A similar effect on the mRNA expression level of IL-18 in the liver was also observed, but there was no statistical significance (p > 0.05). Comparatively, AS-IV administration, especially in AS-IV-M group, inhibited these reactions caused by alcohol intake, as indicated by the decreased mRNA expression levels of NLRP3, IL-1β, and IL-18 (p < 0.05). The protein expression of NLRP3, Pro-caspase-1, Cleaved-Caspase-1, IL-1β, and IL-18 in liver tissue significantly increased after acute alcohol stimulation (, p < 0.05). Compared with model group, the protein expression of NLRP3, Pro-caspase-1, IL-1β, and IL-18 in AS-IV-L, AS-IV-M, and AS-IV-H group significantly decreased (p < 0.05), while there was a decreasing trend of the protein expression of Cleaved-Caspase-1 without statistical significance (p > 0.05).
Figure 8. AS-IV inhibited hepatic inflammation in mice with acute alcohol-induced liver injury through NLRP3/Caspase-1 signaling pathway (n = 6–9). (A–D) the mRNA expression of NLRP3, caspase-1, IL-1β, and IL-18 in liver. (E–J) protein expression of NLRP3, Pro-Caspase-1, IL-1β, IL-18, and Cleaved-Caspase-1 in liver assayed by western blotting, and presented as fold change relative to control. The same internal reference GAPDH bands were used in and . The data are represented as means ± SEM. #p < 0.05, ##p < 0.01, and ###p < 0.001 vs. control group, *p < 0.05, **p < 0.01, and ***p < 0.001 vs. model group.
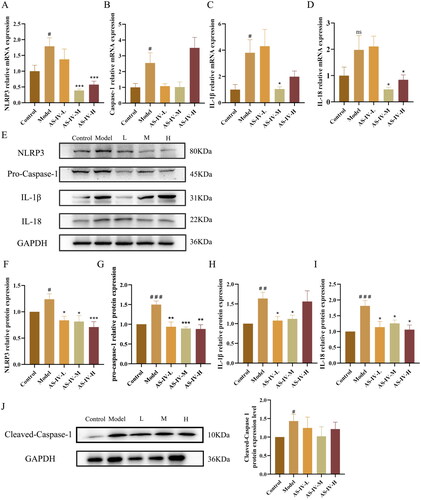
Discussion
ALD is the leading cause of liver-related deaths worldwide [Citation3]. The effect of routine drug treatment for ALD is poor and the side effects are obvious. Nowadays, natural herbs have attracted much attention for their unique advantages, such as natural sources and low toxicity. In this study, we have confirmed the capability of AS-IV to prevent acute alcohol-induced liver injury in mice. The results showed alcohol treatment significantly increased the levels of serum ALT and AST, serum and liver TNF-α, IL-1β, IL-6, and MPO in mice, and AS-IV pretreatment prevented these increases caused by alcohol. Liver pathological sections demonstrated that pretreatment with AS-IV significantly relieved alcohol-induced cellular degeneration, hepatocyte necrosis, and nucleus pycnosis. AS-IV pretreatment also ameliorated alcohol-induced hepatic oxidative stress damage. Besides, AS-IV pretreatment reduced alcohol-induced liver inflammation via modulating the gut microflora imbalance and suppressing LPS, LBP, and DAO translocation, improved intestinal barrier functions, and curbing NLRP3/Caspase-1 inflammatory signaling pathway. In conclusion, these findings revealed that AS-IV has a high potential for preventing alcohol-induced liver injury.
Oxidative stress plays an important role in acute alcohol-induced liver injury, especially when there exist few antioxidants in the liver to resist the excessive reactive oxygen species (ROS) [Citation20]. MDA can reflect the degree of cell injury caused by lipid oxidation. The excessive ROS can activate the lipid peroxidation of liver cells to induce an increase in the production of lipid peroxides, such as MDA, thus destroying the cell membrane structure and subsequently leading to the swelling and necrosis of cells. Antioxidant enzymes, especially SOD and GSH-Px, can mitigate the content of free radicals and the formation of active oxygen in cells, and play a key role in alleviating lipid peroxidation and maintaining the structure and function of cell membranes [Citation21]. AS-IV has been reported to exert antioxidant effects. Li et al. found that AS-IV protects the integrity of blood–brain barrier from LPS-induced disruption by reducing the level of ROS in mice brain microvascular endothelial cells [Citation22]. Wang et al. reported that AS-IV significantly increased the content of antioxidant enzymes SOD, GSH-Px, and CAT, and reduced the ROS production, thereby ameliorating high glucose-induced apoptosis and oxidative stress of the human proximal tubular cell line HK-2 [Citation23]. In our study, the liver content of 4-HNE and MDA increased in model group compared with control group, indicating that acute alcohol treatment causes strong lipid peroxidation in liver tissue, but AS-IV treatment prevent the increases of 4-HNE level. The activity of SOD and the content of GSH-PX in the liver were inhibited in model group compared with control group, but AS-IV treatment could significantly increase these enzyme activities, indicating that the protective effect of AS-IV on acute alcohol liver injury may be related to its antioxidant activity.
The gut-liver axis refers to the two-way relationship between the intestine along with its microbiota and the liver, resulting from the interaction of signals generated by diet, environment, and genetic factors [Citation24]. The control of the microbiota is essential to maintain the homeostasis of the gut-liver axis, and the liver shapes gut microbiota. Growing evidence has demonstrated that gut-liver axis contributes to the pathogenesis of ALD [Citation24,Citation25]. Alcohol and its metabolite acetaldehyde destroy the gut-liver axis at multiple interconnected levels, such as the gut microbiota, intestinal barrier, and antibacterial peptide production, and subsequently increase microbial exposure and the proinflammatory environment in the liver [Citation25]. Bacterial translocation caused by impaired intestinal barrier function is a prerequisite for the development of ALD [Citation26]. In ALD, gut barrier dysfunction is the decisive factor to promote the translocation of PAMP, such as LPS and metabolites of microorganisms from the enteric cavity to the portal vein, where it binds to LBP, triggering a pro-inflammatory cascade that aggravates liver inflammation [Citation27]. Studies have reported that acute alcohol intake or alcoholism can also impair the multilayer defense of the intestinal barrier and increase the level of serum bacterial products in animal and human models [Citation28,Citation29]. Qin et al. found that AS-IV injection prevents alcohol-induced gastric mucosal injury [Citation30]. Xie et al. also confirmed that AS-IV protected intestinal epithelium from sepsis-induced barrier dysfunction by curbing RhoA/NLRP3 inflammasome signal pathway [Citation31]. However, the impact of AS-IV on intestinal barrier function is still unknown. Serum and plasma biomarkers LPS, LBP, and DAO have been used as indicators of impaired intestinal barrier function [Citation32]. In this experiment, we examined the serum levels of LPS, LBP, and DAO, and measured the expression of tight junction proteins including Occludin and Claudin-4 in small intestines, and found that the remission of the intestinal leakage and the decrease of endotoxemia in AS-IV-treated mice, indicating its effective treatment for gut barrier dysfunction.
Alcohol intake has a direct impact on the intestinal microbiota, which has appeared long before liver injury occurs [Citation6]. Growing studies have presented that the intestinal microbiota composition of individuals with chronic alcohol abuse or feeding alcohol to mice is different from healthy controls, such as a lower abundance of Bacteroidetes and Firmicutes, as well as of a greater abundance of Proteobacteria [Citation33,Citation34]. Thus, the restoration of intestinal microbiota could be an effective strategy for preventing and treating alcohol-induced liver injury [Citation24]. As reported by Jin et al. the metabolism of AS-IV by gut microbiota was a crucial step in the disposition of AS-IV, rather than by hepatic and intestinal enzymes. Meanwhile, variations in gut microbiota may alter the disposition of AS-IV and subsequently impact its potential health benefits [Citation11]. Therefore, gut microbiota should be considered as a key factor for AS-IV to exert its bioactivities. In our study, the PCoA analysis showed that the microbial structure composition of the samples had a slight difference, indicating that the therapeutic effects of AS-IV were partly rooted in the effect on intestinal flora. Our results showed that the abundance of Verrucomicrobia, Deferribacteres, Akkermansia, Anaerotruncus, Mucispirillum, and Ralstonia was significantly higher in mice with acute alcohol-induced liver injury, whereas that of Phascolarctoobacterium, Enterobacter, Turicibacter, Acetobacter, and Butyricicoccus was lower in the control group. AS-IV treatment was more effective in modulating the abundance of the following five genera: Butyricicoccus, Turicibacter, Akkermansia, Anaerotruncus, and Mucispirillum. These results of gut microbiota are not completely consistent with previous studies [Citation35,Citation36], which may be attributed to the strain of experimental animals, the duration of alcohol intake, and drug intervention [Citation37].
Butyricicoccus, as an anaerobic, butyrate-producing Gram-positive clostridial cluster IV genus, predominantly colonizes the mucosal-associated surfaces of the colon [Citation38]. Butyrate is a short-chain fatty acid produced during the fermentation of dietary fibre in the colon and is responsible for the maintenance of colonic homeostasis and the control of intestinal epithelial permeability [Citation39]. A study reported a considerable reduction in mucosa-associated genus Butyricicoccus in patients with ulcerative colitis and was correlated with aberrant claudin-1 expression [Citation40]. Accumulating evidence showed that this butyrate-producing bacterium is such a promising potential probiotic candidate for people suffering from intestinal inflammatory bowel disease and colorectal cancer [Citation41,Citation42]. In the current study, the abundance of Butyricicoccus was significantly decreased in model groups, while treatment with AS-IV led to an increase in the abundance of Butyricicoccus. In addition, the inflammatory factor IL-6 in serum was inversely associated with this genus. Turicibacter is widely distributed in mammalian feces and intestines. Although little is known about this genus, increasing studies reported that changes in Turicibacter levels have been related to specific disease states [Citation43]. A previous study has shown that Turicibacter supposedly increases the level of butyrate [Citation44]. The relative abundance of Turicibacter was positively correlated with the intestinal expression of multiple gene pathways, including those important for lipid and steroid metabolism, thereby correspondingly reducing host systemic triglyceride levels and inguinal adipocyte size [Citation45]. In our study, the abundance of this genus decreased in mice with acute alcohol-induced liver injury compared with its abundance in the control mice, whereas AS-IV treatment led to an increase in its relative abundance. Mucispirillum, as a Gram-negative anaerobic bacterium, is widely distributed in the intestinal mucosa of rodents and humans, and has been considered to have the ability to degrade mucin layer. Mucispirillum can trigger colitis by accumulating in the intestinal lumen and mucosa and impeding neutrophil recruitment in immunodeficient mice [Citation46]. A previous study found that Mucispirillum and Anaerotruncus increased in high-fat/high-cholesterol-fed mice, which was corroborated in human hypercholesteremia patients [Citation47]. In our study, the level of Anaerotruncus and Mucispirillum in the model group was significantly higher than that in the control group, and the level of these two bacteria was significantly decreased after the administration of AS-IV. In addition, Mucispirillum was positively correlated with LPS, IL-1β, TNF-α in serum, and IL-1β in liver, and Anaerotruncus was positively associated with serum TNF-α. These results showed that the anti-alcohol and hepato-protective effect of AS-IV could be closely related to its interaction with the gut microbiota.
NLPR3/caspase-1 signaling pathway is one of the key pathways related to the inflammatory response. Its activation can cause the production of a large number of inflammatory cytokines and then lead to a series of inflammatory responses. LPS is the main component of the outer membrane of Gram-negative bacteria, and can increase the production of various inflammatory cytokines and chemokines and subsequently induces innate immunity of liver [Citation48]. After LPS stimulation, the activity of NLRP3 inflammasome increased via various signaling mechanisms. NLRP3 then binds to ASC and recruits caspase-1 through pyrin domain (PYD) and recruitment domain (CARD) domains, ultimately upregulating the levels of inflammation and apoptosis [Citation48,Citation49]. Cui et al. found that the levels of the NLRP3 inflammasome components NLRP3, ASC, and cleaved caspase-1 increased, and the mature IL-1β raised in Kupffer cells (KCs) of alcohol-fed mice [Citation50]. Petrasek et al. demonstrated that the deficiency of Caspase-1, ASC, or IL-1R1 could continuously prevented alcoholic hepatitis and significantly ameliorated alcohol-induced steatosis and liver damage in Casp1−/−, ASC−/−, or IL-1R−/− knockout mice [Citation51]. In addition, it has been found that elevated liver expression of inflammasome components including caspase-1, IL-1β, and IL-18 was associated with liver injury in patients with ALD [Citation52]. Although AS-IV has been proven to regulate the NLRP3 inflammasome, its molecular mechanism remains largely unknown. Feng et al. reported that AS-IV improved renal function and epithelial cell injury in the visceral layer of the renal capsule and delayed the development of diabetic nephropathy in mice by inhibiting the NLRP3/caspase-1 signaling pathway [Citation53]. Sun et al. showed that AS-IV could inhibit monocrotaline-induced pulmonary arterial hypertension by the NLRP3/caspase-1 pathway in vivo and/or in vitro [Citation54]. NLRP3 inflammasome activation could be induced by LPS [Citation19]. Alcohol can cause liver tissue damage via the production of LPS from gut microbiota [Citation28,Citation29]. In this study, we have found that AS-IV treatment inhibited an increase in serum LPS levels, as well as the liver NLRP3, caspase-1, IL-1β, and IL-18 in mice treated with alcohol. In addition, we also found that the levels of Neutrophil marker MPO and proinflammatory cytokine, such as TNF-α, IL-6, and IL-1β were significantly increased after alcohol stimulation in the serum and liver tissue, while AS-IV treatment prevented these increases. In the liver, TNF-α is produced by alcohol-induced LPS-stimulated KCs and is involved in various pathophysiological processes, such as immune inflammation, cell proliferation, and apoptosis [Citation55]. Administration of excessive alcohol to TNF-α knockout mice does not cause liver damage. In patients with alcoholic hepatitis, elevated levels of TNF-inducible cytokines or chemokines, such as IL-6, IL-1β, and IL-18 in serum have also been found [Citation56]. IL-6 is mainly secreted by KCs and exacerbates alcohol-induced liver inflammation by promoting the production of Th17 and IL-17 [Citation57]. These results suggest that AS-IV inhibits alcohol-induced liver injury and systematic inflammatory response by inhibiting the LPS-induced NLRP3/caspase-1 signaling pathway.
In summary, This study reveals that AS-IV is capable of ameliorating hepatic oxidative stress, liver function, and inflammation via modulating gut microbiota and regulating NLRP3/Caspase-1 signaling pathway (). AS-IV could be a natural active substance that prevents acute alcoholic liver injury.
Figure 9. Schematic diagram of the protective effect of Astragaloside IV in alcohol-induced liver injury via modulating gut microbiota and regulating NLRP3/Caspase-1 signaling pathway, resulting in IL-1β and IL-18 reduction, and eventually alleviating liver inflammation. PAMP: pathogen-associated molecular patterns; NLRP3: nucleotide-binding domain-like receptor protein 3; ASC: apoptosis associated speck-like protein containing a caspase recruitment domain.
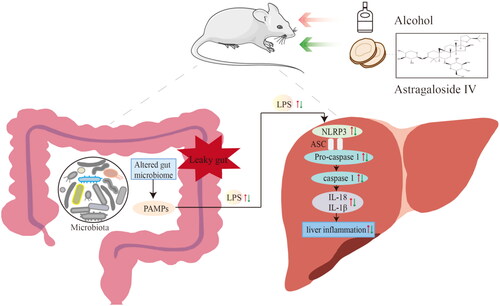
Ethical approval
The animal study was approved by the Institutional Animal Care and Use Committee of Guangdong Pharmaceutical University (Guangzhou, China).
Author contributions
S.W. acquired the financial support, formulated the overarching research goals, and wrote the original draft. F.W. carried out the experiments, analyzed or synthesized the study data, and wrote the original draft. X.Z. and W.D. designed the methodology and carried out the experiments. M.C. and J.W. acquired the financial support and critical review of the manuscript.
Disclosure statement
No potential conflict of interest was reported by the author(s).
Data availability statement
The raw data supporting the conclusions of this article will be made available by the authors, without undue reservation.
Additional information
Funding
References
- Singal AK, Bataller R, Ahn J, et al. ACG clinical guideline: alcoholic liver disease. Am J Gastroenterol. 2018;113(2):1–16.
- Osna NA, Donohue TJ, Kharbanda KK. Alcoholic liver disease: pathogenesis and current management. Alcohol Res. 2017;38:147–161.
- Kong LZ, Chandimali N, Han YH, et al. Pathogenesis, early diagnosis, and therapeutic management of alcoholic liver disease. Int J Mol Sci. 2019;20(11):2712.
- Meroni M, Longo M, Dongiovanni P. Alcohol or gut microbiota: who is the guilty? Int J Mol Sci. 2019;20(18):4568.
- Grander C, Adolph TE, Wieser V, et al. Recovery of ethanol-induced Akkermansia muciniphila depletion ameliorates alcoholic liver disease. Gut. 2018;67(5):891–901.
- Bull-Otterson L, Feng W, Kirpich I, et al. Metagenomic analyses of alcohol induced pathogenic alterations in the intestinal microbiome and the effect of Lactobacillus rhamnosus GG treatment. PLOS One. 2013;8(1):e53028.
- Maccioni L, Gao B, Leclercq S, et al. Intestinal permeability, microbial translocation, changes in duodenal and fecal microbiota, and their associations with alcoholic liver disease progression in humans. Gut Microbes. 2020;12(1):1782157.
- Shang Y, Jiang M, Chen N, et al. Inhibition of HMGB1/TLR4 signaling pathway by digitoflavone: a potential therapeutic role in alcohol-associated liver disease. J Agric Food Chem. 2022;70(9):2968–2983.
- Alegre F, Pelegrin P, Feldstein AE. Inflammasomes in liver fibrosis. Semin Liver Dis. 2017;37(2):119–127.
- Hornung V, Ablasser A, Charrel-Dennis M, et al. AIM2 recognizes cytosolic dsDNA and forms a caspase-1-activating inflammasome with ASC. Nature. 2009;458(7237):514–518.
- Jin Y, Guo X, Yuan B, et al. Disposition of astragaloside IV via enterohepatic circulation is affected by the activity of the intestinal microbiome. J Agric Food Chem. 2015;63(26):6084–6093.
- Liu YL, Zhang QZ, Wang YR, et al. Astragaloside IV improves high-fat diet-induced hepatic steatosis in nonalcoholic fatty liver disease rats by regulating inflammatory factors level via TLR4/NF-κB signaling pathway. Front Pharmacol. 2020;11:605064.
- Jiang ZB, Gao J, Chai YH, et al. Astragaloside alleviates alcoholic fatty liver disease by suppressing oxidative stress. Kaohsiung J Med Sci. 2021;37(8):718–729.
- Xu N, Kan P, Yao X, et al. Astragaloside IV reversed the autophagy and oxidative stress induced by the intestinal microbiota of AIS in mice. J Microbiol. 2018;56(11):838–846.
- Gong P, Xiao X, Wang S, et al. Hypoglycemic effect of astragaloside IV via modulating gut microbiota and regulating AMPK/SIRT1 and PI3K/AKT pathway. J Ethnopharmacol. 2021;281:114558.
- Khan I, Bhardwaj M, Shukla S, et al. Carvacrol inhibits cytochrome P450 and protects against binge alcohol-induced liver toxicity. Food Chem Toxicol. 2019;131:110582.
- Zhao H, Li H, Lai Q, et al. Antioxidant and hepatoprotective activities of modified polysaccharides from Coprinus comatus in mice with alcohol-induced liver injury. Int J Biol Macromol. 2019;127:476–485.
- Kemp PF, Aller JY. Bacterial diversity in aquatic and other environments: what 16S rDNA libraries can tell us. FEMS Microbiol Ecol. 2004;47(2):161–177.
- Schroder K, Tschopp J. The inflammasomes. Cell. 2010;140(6):821–832.
- Wu D, Cederbaum AI. Oxidative stress and alcoholic liver disease. Semin Liver Dis. 2009;29(2):141–154.
- Ma Z, Zhang Y, Li Q, et al. Resveratrol improves alcoholic fatty liver disease by downregulating HIF-1α expression and mitochondrial ROS production. PLOS One. 2017;12(8):e183426.
- Li H, Wang P, Huang F, et al. Astragaloside IV protects blood-brain barrier integrity from LPS-induced disruption via activating Nrf2 antioxidant signaling pathway in mice. Toxicol Appl Pharmacol. 2018;340:58–66.
- Wang J, Guo HM. Astragaloside IV ameliorates high glucose-induced HK-2 cell apoptosis and oxidative stress by regulating the Nrf2/ARE signaling pathway. Exp Ther Med. 2019;17(6):4409–4416.
- Albillos A, de Gottardi A, Rescigno M. The gut-liver axis in liver disease: pathophysiological basis for therapy. J Hepatol. 2020;72(3):558–577.
- Bajaj JS. Alcohol, liver disease and the gut microbiota. Nat Rev Gastroenterol Hepatol. 2019;16(4):235–246.
- Fouts DE, Torralba M, Nelson KE, et al. Bacterial translocation and changes in the intestinal microbiome in mouse models of liver disease. J Hepatol. 2012;56(6):1283–1292.
- Tilg H, Moschen AR, Szabo G. Interleukin-1 and inflammasomes in alcoholic liver disease/acute alcoholic hepatitis and nonalcoholic fatty liver disease/nonalcoholic steatohepatitis. Hepatology. 2016;64(3):955–965.
- Bala S, Marcos M, Gattu A, et al. Acute binge drinking increases serum endotoxin and bacterial DNA levels in healthy individuals. PLOS One. 2014;9(5):e96864.
- Voigt RM, Forsyth CB, Shaikh M, et al. Diurnal variations in intestinal barrier integrity and liver pathology in mice: implications for alcohol binge. Am J Physiol Gastrointest Liver Physiol. 2018;314(1):G131–G141.
- Qin S, Huang K, Fang Z, et al. The effect of astragaloside IV on ethanol-induced gastric mucosal injury in rats: involvement of inflammation. Int Immunopharmacol. 2017;52:211–217.
- Xie S, Yang T, Wang Z, et al. Astragaloside IV attenuates sepsis-induced intestinal barrier dysfunction via suppressing RhoA/NLRP3 inflammasome signaling. Int Immunopharmacol. 2020;78:106066.
- Vancamelbeke M, Vermeire S. The intestinal barrier: a fundamental role in health and disease. Expert Rev Gastroenterol Hepatol. 2017;11(9):821–834.
- Chen Y, Yang F, Lu H, et al. Characterization of fecal microbial communities in patients with liver cirrhosis. Hepatology. 2011;54(2):562–572.
- Yan AW, Fouts DE, Brandl J, et al. Enteric dysbiosis associated with a mouse model of alcoholic liver disease. Hepatology. 2011;53(1):96–105.
- Zhang H, Zuo Y, Zhao H, et al. Folic acid ameliorates alcohol-induced liver injury via gut-liver axis homeostasis. Front Nutr. 2022;9:989311.
- Liu SX, Liu H, Wang S, et al. Diallyl disulfide ameliorates ethanol-induced liver steatosis and inflammation by maintaining the fatty acid catabolism and regulating the gut-liver axis. Food Chem Toxicol. 2022;164:113108.
- Leclercq S, Matamoros S, Cani PD, et al. Intestinal permeability, gut-bacterial dysbiosis, and behavioral markers of alcohol-dependence severity. Proc Natl Acad Sci USA. 2014;111:E4485–E4493.
- Eeckhaut V, Van Immerseel F, Teirlynck E, et al. Butyricicoccus pullicaecorum gen. nov., sp. nov., an anaerobic, butyrate-producing bacterium isolated from the caecal content of a broiler chicken. Int J Syst Evol Microbiol. 2008;58(12):2799–2802.
- Guilloteau P, Martin L, Eeckhaut V, et al. From the gut to the peripheral tissues: the multiple effects of butyrate. Nutr Res Rev. 2010;23(2):366–384.
- Devriese S, Eeckhaut V, Geirnaert A, et al. Reduced mucosa-associated butyricicoccus activity in patients with ulcerative colitis correlates with aberrant claudin-1 expression. ECCOJC. 2017;11(2):229–236.
- Chang SC, Shen MH, Liu CY, et al. A gut butyrate-producing bacterium Butyricicoccus pullicaecorum regulates short-chain fatty acid transporter and receptor to reduce the progression of 1,2-dimethylhydrazine-associated colorectal cancer. Oncol Lett. 2020;20(6):327.
- Geirnaert A, Steyaert A, Eeckhaut V, et al. Butyricicoccus pullicaecorum, a butyrate producer with probiotic potential, is intrinsically tolerant to stomach and small intestine conditions. Anaerobe. 2014;30:70–74.
- Goodrich JK, Davenport ER, Waters JL, et al. Cross-species comparisons of host genetic associations with the microbiome. Science. 2016;352(6285):532–535.
- Zhong Y, Nyman M, Fåk F. Modulation of gut microbiota in rats fed high-fat diets by processing whole-grain barley to barley malt. Mol Nutr Food Res. 2015;59(10):2066–2076.
- Fung TC, Vuong HE, Luna C, et al. Intestinal serotonin and fluoxetine exposure modulate bacterial colonization in the gut. Nat Microbiol. 2019;4(12):2064–2073.
- Caruso R, Mathes T, Martens EC, et al. A specific gene-microbe interaction drives the development of Crohn’s disease-like colitis in mice. Sci Immunol. 2019;4(34):eaaw4341.
- Zhang X, Coker OO, Chu ES, et al. Dietary cholesterol drives fatty liver-associated liver cancer by modulating gut microbiota and metabolites. Gut. 2021;70(4):761–774.
- Li Q, Tan Y, Chen S, et al. Irisin alleviates LPS-induced liver injury and inflammation through inhibition of NLRP3 inflammasome and NF-κB signaling. J Recept Signal Transduct Res. 2021;41(3):294–303.
- Lu A, Magupalli VG, Ruan J, et al. Unified polymerization mechanism for the assembly of ASC-dependent inflammasomes. Cell. 2014;156(6):1193–1206.
- Cui K, Yan G, Xu C, et al. Invariant NKT cells promote alcohol-induced steatohepatitis through interleukin-1β in mice. J Hepatol. 2015;62(6):1311–1318.
- Petrasek J, Bala S, Csak T, et al. IL-1 receptor antagonist ameliorates inflammasome-dependent alcoholic steatohepatitis in mice. J Clin Invest. 2012;122(10):3476–3489.
- Voican CS, Njiké-Nakseu M, Boujedidi H, et al. Alcohol withdrawal alleviates adipose tissue inflammation in patients with alcoholic liver disease. Liver Int. 2015;35(3):967–978.
- Feng H, Zhu X, Tang Y, et al. Astragaloside IV ameliorates diabetic nephropathy in db/db mice by inhibiting NLRP3 inflammasome‑mediated inflammation. Int J Mol Med. 2021;48(2):164.
- Sun Y, Lu M, Sun T, et al. Astragaloside IV attenuates inflammatory response mediated by NLRP-3/calpain-1 is involved in the development of pulmonary hypertension. J Cell Mol Med. 2021;25(1):586–590.
- McClain CJ, Song Z, Barve SS, et al. Recent advances in alcoholic liver disease. IV. Dysregulated cytokine metabolism in alcoholic liver disease. Am J Physiol Gastrointest Liver Physiol. 2004;287(3):G497–G502.
- Hill DB, Marsano L, Cohen D, et al. Increased plasma interleukin-6 concentrations in alcoholic hepatitis. J Lab Clin Med. 1992;119:547–552.
- Kawaratani H, Tsujimoto T, Douhara A, et al. The effect of inflammatory cytokines in alcoholic liver disease. Mediators Inflamm. 2013;2013:495156.