Abstract
Background
Bile acids play crucial roles in various metabolisms, as well as Lactobacillus in the intestine. But studies on their roles in acute coronary syndrome (ACS) are still insufficient. The aim of this study was to investigate their role and potential association with the severity of coronary lesions and the prognosis of ACS.
Methods
Three hundred and sixty ACS patients were selected. Detection of gut Lactobacillus levels was done through 16S rDNA sequence analysis. Evaluation of the extent of lesions was done using the SYNTAX (SS) score. Mediation analysis was used to assess the relationship between serum total bile acid (TBA), Lactobacillus, atherosclerotic lesions and prognosis of ACS.
Results
Logistic regressive analysis disclosed that serum TBA and Lactobacillus were independent predictors of coronary lesions (high vs. low SS: serum TBA adjusted odds ratio (aOR) = 0.8, 95% confidence interval (CI): 0.6–0.9, p < .01; Lactobacillus: aOR = 0.9, 95% CI: 0.9–1.0, p = .03). According to multivariate Cox regression analysis, they were negatively correlated with the overall risk of all-cause death (serum TBA: adjusted hazard ratio (aHR) = 0.1, 95% CI: 0.0–0.6, p = .02; Lactobacillus: aHR = 0.6, 95% CI: 0.4–0.9, p = .01), especially in acute myocardial infarction (AMI) but not in unstable angina pectoris (UAP). Ulteriorly, mediation analysis showed that serum TBA played an important role as a mediation effect in the following aspects: Lactobacillus (17.0%, p < .05) → SS association (per 1 standard deviation (SD) increase), Lactobacillus (43.0%, p < .05) → all-cause death (per 1 SD increase) and Lactobacillus (45.4%, p < .05) → cardiac death (per 1 SD increase).
Conclusions
The lower serum TBA and Lactobacillus level in ACS patients, especially in AMI, was independently linked to the risk of coronary lesions, all-cause death and cardiac death. In addition, according to our mediation model, serum TBA served as a partial intermediate in predicting coronary lesions and the risk of death by Lactobacillus, which is paramount to further exploring the mechanism of Lactobacillus and bile acids in ACS.
Lower level of serum total bile acid (TBA) was highly associated with the severity of coronary lesions, myocardial damage, inflammation and gut Lactobacillus in acute coronary syndrome (ACS) patients, especially in acute myocardial infarction (AMI).
Lower level of serum TBA was highly associated with mortality (including all-cause death and cardiac death) in patients with ACS, especially with AMI.
Serum TBA had a partial mediating effect rather than regulating effect between gut Lactobacillus and coronary lesions and prognosis of ACS.
KEY MESSAGES
1. Introduction
As one of the main metabolites of cholesterol, bile acids play qualities of momentous roles in lipid metabolism, glucose metabolism and energy metabolism [Citation1–3]. Bile acids are classified into primary and secondary bile acids. In the liver, primary bile acids are synthesized from cholesterol, while in the intestine, secondary bile acids are synthesized from primary bile acids as substrates by the intestinal microbiota. The entero-hepatic cycle – a persistent loop connecting bile acid secretion in the gut lumen, microbial transformation, ileal reabsorption and vascular transit through the portal circulation to the liver – is a link between the microbiota and the host [Citation3]. Animal studies have shown that in the absence of hepatobiliary system disease, increased excretion of bile acids counteracted the cholesterol overload caused by a high-cholesterol diet and delayed atherosclerotic lesions [Citation4]. Pharmacological studies have shown that bile acid chelators could reduce cholesterol levels in the blood, especially LDL cholesterol levels, in patients with various metabolic diseases [Citation5,Citation6]. Therefore, an imbalance of bile acid metabolism will lead to disorders of lipid metabolism, especially cholesterol metabolism, which will lead to the occurrence and progression of atherosclerosis [Citation7,Citation8].
Several recent investigations suggested that coronary artery disease (CAD) patients had lower bile acid faecal excretion than non-CAD [Citation7,Citation8], indicating an imbalance in bile acid metabolism during the CAD process. However, these studies regarding the elimination of TBA in the stool of a limited sample of CAD patients did not further investigate serum TBA. Understanding the relationship between serum TBA and atherosclerosis still stemmed from research from the last century, these studies suggested that the amassed bile acids in the body indicated cholesterol overloading, which might be a risk factor for CAD [Citation7]. Until now, TBA serum is more easily obtained in CAD patients, especially in ACS patients. However, consensus on the role or mechanism of serum TBA in atherosclerosis has still not been reached.
The composition of bile acids is influenced by a variety of microbiota [Citation9]. Previous studies have shown that a diversity of bacteria take part in the complex biotransformation process of bile acids [Citation10], including Lactobacillus [Citation11], Bifidobacterium, Bacteroides and Enterococcus. Only a few studies have shown a correlation between Lactobacillus and AMI. Zhou et al. found that gut Lactobacillus in AMI patients was associated with inflammatory response and cardiovascular events [Citation12]. Lam et al. demonstrated that supplementation with Lactobacillus plantarum 299v reduced acute myocardial injury after ischemia–reperfusion [Citation13]. Haghikia et al. found that gut microbial metabolites predict the risk of cardiovascular events in patients with chest pain [Citation14]. In previous studies by our team, we revealed for the first time the altered genus distribution of ACS intestinal flora by 16S rDNA sequencing analysis of intestinal bacteria [Citation15]. By further exploration, we found that reduced levels of the intestinal probiotic Lactobacillus were closely associated with ACS occurrence, development and prognosis at the same time [Citation16]. Bile acids are one of the fermented metabolites of gut microbiota. Wang et al.’s study showed that bile acids may influence the degree of inflammatory response in AMI patients [Citation17]. Fan et al. showed a decrease in primary bile acids in patients with ACS [Citation18], but did not explore whether this correlated with gut microbiota and coronary lesions. There are no studies showing an association between TBA and Lactobacillus in patients with ACS, or whether this association is related to coronary lesions and prognosis. The application of findings from animal models is limited by significant interspecies metabolic differences [Citation19]. Considering the bridging role of bile acid between gut microbiota and hosts, we attempted for the first time to use analyses of mediation and moderation in ACS patients for exploring the potential link between gut microbiota, bile acid, coronary lesions and prognosis. This study provided a new research direction to further explore the metabolic mechanisms of coronary lesions and also provided evidence for individualized treatment plans for diseases.
2. Materials and methods
2.1. Study population
All hospitalized patients diagnosed with acute coronary syndrome (ACS) were eligible for participation in this study that was conducted between June 2017 and December 2018. The diagnostic criteria of ACS were under ESC guidelines [Citation20]. The detailed diagnostic criteria of ACS were: (1) unstable symptoms of myocardial ischemia occurring at rest or with any exertion within 72 h before admission, including but not limited to persistent ischemic chest pain or recurrent ischemic chest pain; (2) elevated cardiac biomarkers, especially cardiac troponin (cTn) I/T, creatine kinase (CK) isoenzyme; (3) resting ECG changes consistent with ischemia or infarction; (4) ≥50% epicardial coronary stenosis by angiography. The exclusion criteria: (1) age <18-year-old; (2) complicated with structural heart disease; (3) uncontrolled heart failure and hemodynamic instability; (4) history of cerebrovascular disease, kidney disease, respiratory diseases, or gastrointestinal diseases or history of gastrointestinal surgery; (5) infectious or infectious diseases; (6) smoking more than five cigarettes per day [Citation21], intaking more than 140 mg of ethanol per week [Citation22], or those who quit smoking and drinking within the last three months; (7) malignant tumours; (8) prescriptions for bile acid sequestrants, or drugs affecting bile acid metabolism; (9) prescriptions for probiotic, antacid or antibiotic 3 months before or during the study; (10) pregnancy or lactation; (11) no serum total bile acid (TBA) measurement.
This research was conducted under the requirement of the Helsinki Declaration, and the research plan was reviewed and sanctioned by Tianjin Chest Hospital’s Ethics Committee (no. 2018KY-010-01). After being apprised of the procedures and hazards involved, all patients agreed to take part in the study and signed the informed consent. Every procedure followed complied with the ethical requirements of the authorities (institutional and national) responsible for human experimentation, as well as the 1975 Declaration of Helsinki (updated in 2000).
This study ultimately included 360 patients. Among them, 310 were diagnosed with acute myocardial infarction (AMI), and 50 with unstable angina pectoris (UAP). Detailed information for each patient, such as demographic, clinical history, history of present illness, hematologic and angiographic data, were sourced from medical records.
2.2. Specimens
Fasting blood specimens were taken from patients within 12 h of presentation, and serum fasting TBA was measured immediately on a Roche Cobas c701 using reagents for enzymatic cycling assay (TBA Kit, Meikang Biotechnology Co., Ltd., Shanghai, China) as required by the protocols. Quality control procedures were followed as well. The normal range of serum TBA was 0–10 μmol/L. The total mutation coefficients were all less than 5%. On the first morning in the hospital, samples of fresh faecal (more than 300 mg) were gathered from all participators, stored in airtight sample containers, and refrigerated at 4 °C in the laboratory. One part was used for normal inspection, and the other part was placed in a sterile container, external circulation refrigeration tube, which was stored in a sealed environment of −156 °C.
2.3. SYBR Green II Real-time fluorescence quantitative PCR for Lactobacillus detection
Extract faecal DNA from sent faecal samples. After extracting bacterial DNA, SYBR Green II real-time fluorescence quantitative PCR was utilized to determine the total bacterial load and the bacterial load of the Lactobacillus in the intestine. The reaction reference materials were designed based on literature and made by Thermo Fisher Scientific Ltd (Waltham, MA) [Citation23,Citation24]. Use TB Green™ Premier Ex Taq™ II (Tli RNaseH Plus) as a reagent. The reaction system included 10 μL of Real Master Mix, 0.8 μL each of upstream and downstream primers, 0.4 μL of ROX II, 2 μL of template DNA and 6 μL of DEPC water. The reaction was carried out under the following conditions: 95 °C for 5 min before degradation, 95 °C variance for 15 s (total bacterial load 58 °C, Lactobacillus 58 °C), melting for 34 s, 72 °C expansion for 60 s, 40 cycles. The melting curve after the reaction was used to evaluate the specificity of PCR products. The 7500 System SDS software automatically evaluated relative quantitation values (Ct) based on the fluorescence data and developed a standard curve.
2.4. Evaluation of coronary artery atherosclerotic lesions
All subjects underwent coronary angiography, with at least one coronary artery stenosis greater than 50%, and percutaneous coronary intervention (PCI) was performed within 72 h. Following that, SYNTAX scores (SS) were calculated, which were commonly used for assessing the severity of coronary lesions [Citation7,Citation8]. Vessels with a diameter greater than 1.5 mm and 50% stenosis were evaluated. The weight of each coronary segment was set according to the location and severity of the lesion. Use a calculator, SYNTAX calculator (https://syntaxscore.org/), to summarize comprehensive closure, trifurcation, bifurcation, calcification, curvature, length > 20mm, thrombosis, and diffuse or small vessel disease. The results of SS reflected the weight values related to the degree of coronary artery stenosis.
2.5. Follow up
Every 6-month interval after employment, prospective visits were conducted through visits to clinics or phone calls. All enrolled patients were contacted and reminded to come to the coronary heart disease (CAD) clinic on time. Outpatient physicians provided professional advice based on the patient’s current state, including laboratory testing, imaging tests, and the most appropriate measures. Based on follow-up, the cardiologist determined the time of the first MACE (major adverse cardiovascular event) and considered it an endpoint event for the subject. Death, stroke, recurrent myocardial infarction, recurrent coronary revascularization and acute exacerbation of heart failure were all recognized as MACEs.
2.6. Statistical analysis
All data were entered into the SPSS version 23.0 statistical software (SPSS Inc., IBM, Armonk, NY) and analysed. The measurement data were displayed as quartiles, and the categorical data were expressed in percentages. Multiple logistic regression analysis was used to distinguish factors that affect coronary lesions. The multivariate regression analysis included the variables with p value not greater than .05 in univariate analysis. To achieve as much survival homogeneity and heterogeneity between groups as feasible [Citation25], serum TBA was classified using X-tile analysis (X-tile software 3.6.1, Yale University, Cambridge, MA). The level of Lactobacillus was also divided by the above method. The survival time of different serum TBA and Lactobacillus levels was compared using the Kaplan–Meier method. A statistical significance of p < .05 was considered to be present. Multivariate Cox regression was a predictor used to establish the primary outcomes. The regulation model and mediation model were used to test the relationship between Lactobacillus and SS and between Lactobacillus and risk of death. The risk of all-cause death was expressed by the natural logarithm of the ratio of cumulative risk function value and survival analysis function value based on the Cox risk model, as was cardiac death. Hayes’ PROCESS macro tool for SPSS (process version 3.4, IBM, Armonk, NY), based on the method with 5000 bootstraps bias-corrected 95% confidence intervals (CIs), was chosen as recommended by Hayes [Citation26]. All figures were created by GraphPad Prism version 8.0.2 (GraphPad Software, La Jolla, CA).
3. Results
3.1. Patient characteristics
According to the long-standing follow-up consequences of previous studies on the revascularization of patients with ACS and the relationship between SS and treatment strategies and prognosis shown in the 2018 ESC/ESCT revascularization guidelines, the SS was divided into three groups, such as low SS (SS < 23), medium SS (23 ≤ SS ≤ 32) and high SS (SS > 32) [Citation27,Citation28]. The basic characteristics of all subjects are shown in . There were 112 (31.1%) in the low SS group, 78 patients (21.7%) in the medium SS group and 170 patients (42.2%) in the high SS group. The average age of 360 ACS patients was 63.5-year-old, of which 62.8% were men. As shown in , age, CK max, creatine kinase isoenzyme-MB (CK-MB) max, cardiac troponin T (cTNT), B-type natriuretic peptide (BNP) and left ventricular ejection fraction (LVEF) were notably positively associated with SS (all, p < .05). The median of serum TBA of all subjects was 1.7 μmol/L, and of Lactobacillus was 7.4 log10 copies/g, both of them decreased with the increase of SS (p < .05).
Table 1. Demographic and biochemical characteristics of patients grouped according to the SYNTAX score.
3.2. Serum TBA levels were related to coronary lesions, myocardial injury
Univariate logistic regression analysis indicated that age, CK, CKMB, cTNT and CRP were the risk factors for coronary lesion severity, while LVEF, serum TBA and Lactobacillus were protective factors (all, p < .05, ). After adjusting for the above variables with p values less than .05, multivariate analysis as shown in indicated that higher levels of LVEF, serum TBA and Lactobacillus were associated with a reduced risk of the coronary lesion severity (TBA: high vs. low SS: adjusted odds ratio (aOR) = 0.8, 95% CI: 0.6–0.9, p < .01; Lactobacillus: high vs. low SS: aOR = 0.9, 95% CI: 0.9–1.0, p = .03). Further subgroup analysis was stratified according to the types of ACS (UAP, AMI). In the AMI group, age, diabetes, LVEF, HDL, TBA and Lactobacillus were associated with SSs by univariate analysis. By adjusting those variables, serum TBA remained significantly correlated with SSs (TBA: high vs. low SS: aOR = 0.8, 95% CI: 0.6–1.0, p = .04; Lactobacillus: high vs. low SS: aOR = 0.9, 95% CI: 0.8–1.0, p = .03). In the UAP group, although only serum TBA and Lactobacillus were correlated with SS in univariate analysis, this correlation was not significant in multivariate analysis ().
Figure 1. Multivariate logistic regression analysis of serum TBA and Lactobacillus on coronary lesions severity. (A) Heat map to show or and p value for the risk of a high SYNTAX score (the green and yellow blocks represent negative and positive correlations, respectively); (B) multivariate logistic regression model adjusted for age, left ventricular ejection fraction (LVEF), CK max, CK-MB max, cTNT max, C-reactive protein (CRP), serum total bile acids (TBA) and Lactobacillus between SYNTAX score medium group and low group (Ref.); (c) multivariate logistic regression model adjusted for age, left ventricular ejection fraction (LVEF), CK max, CK-MB max, cTNT max, C-reactive protein (CRP), scrum total bile acids (TBA) and Lactobacillus between SYNTAX score high group and low group (Ref.); *p value < .05; **p value < .01.
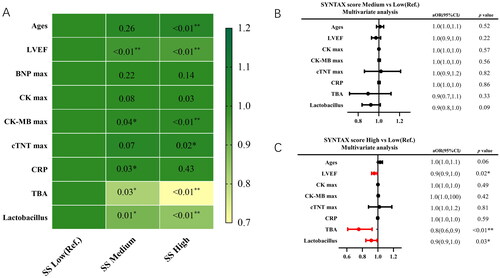
Figure 2. Subgroup analysis in different type of ACS (AMI and UAP). (A,B) Multivariate logistic regression analysis adjusting for ages, diabetes, LVEF, HDL, total bile acid (TBA) and Lactobacillus in AMI group; (C, D) multivariate logistic regression analysis adjusting for total bile acid (TBA) and Lactobacillus in UAP group.
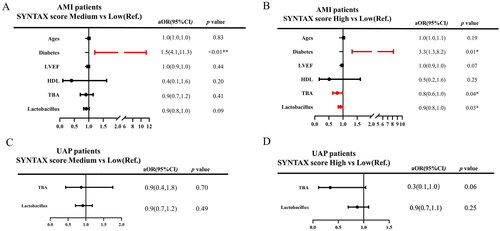
All subjects were assigned into three groups, such as low level group (<1 μmol/L), medium level group (1–2 μmol/L) and high level group (>2 μmol/L) of serum TBA by X-tile analysis (Supplementary Figure). By using the Kruskal–Wallis test (Supplementary Table 1; ), serum TBA was significantly correlated with biomarkers such as LVEF white blood cells (WBC), alanine transaminase (ALT), serum creatinine (Scr), CK max, CKMB max, cTNT max, BNP max, C-reactive protein (CRP), SS and Lactobacillus. Correlation analysis showed serum TBA was passively correlated with WBC, ALT, Scr, CK max, CK-MB max, cTNT max, CRP, BNP and SS, but was positively correlated with LVEF and Lactobacillus ().
Figure 3. The correlation between serum total bile acid (TBA) and biomarkers. (A) The Kruskal–Wallis test of the relationship between TBA and biomarkers; (B) correlation analysis between TBA and biomarkers (the green and orange blocks represent negative and positive correlations, the numbers in each block represent the p value).
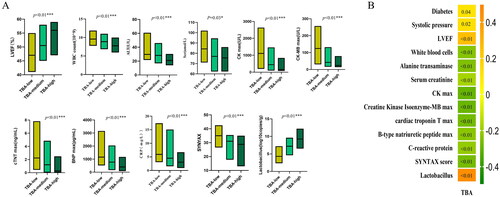
3.3. Serum TBA levels were related to the prognosis of ACS
Only one patient was lost to follow-up because of the wrong mobile phone number. During the 626.0 (540.0, 758.0) days of follow-up, 16 (4.5%) patients had an event of all-cause death, of which 15 had an event of cardiac death. The basic characteristics of patients were grouped based on all-cause death and cardiac death ().
Table 2. Demographic and biochemical characteristics of patients grouped according to the all-cause death and cardiac-cause death.
Age, smoking, old myocardial infarction, systolic pressure, LVEF, Scr, BNP, serum TBA and Lactobacillus were significantly correlated with all-cause death and cardiac death by Wilcoxon Mann–Whitney’s test (). Gut Lactobacillus was split into the low level group (<3.6 copies/g), the medium level group (3.6–7.1 copies/g) and the high level group (>7.1 copies/g) by X-tile analysis. Kaplan–Meier’s curve analyses showed that both a lower serum TBA level and a lower Lactobacillus level could distinguish the patient with a higher risk of all-cause death (). After adjusting for the above variables that were statistically significant, multi-COX regression analysis showed an independent relationship between serum TBA, Lactobacillus, and a reduced risk of all-cause death (serum TBA: adjusted hazard ratio (aHR) = 0.1, 95% CI: 0.0–0.6, p = .02; Lactobacillus: aHR = 0.6, 95% CI: 0.4–0.9, p = .01) (). Subgroup analysis showed that in the AMI subgroup, COX analysis adjusting for the above variables showed that serum TBA and Lactobacillus were significantly correlated with all-cause death (serum TBA: aHR = 0.1, 95% CI: 0.0–0.6, p = .02; Lactobacillus aHR = 0.6, 95% CI: 0.4–0.9, p = .01) (). However, in the UAP subgroup, no such correlation was found.
Figure 4. Kaplan–Meier’s survival curve with all-cause death and cardiac death as endpoint events. (A) The Kaplan–Meier curve between serum TBA and all-cause death. (B) The Kaplan–Meier curve between serum TBA and cardiac death. (C) The Kaplan–Meier curve between Lactobacillus and all-cause death. (D) The Kaplan–Meier curve between Lactobacillus and cardiac death.
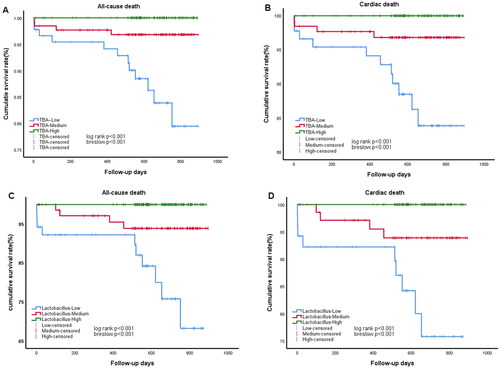
Figure 5. Multivariate COX hazard model adjusted for age, smoking, old myocardial infarction (OMI), systolic pressure, left ventricular ejection fraction (LVEF), serum creatinine (Scr), B-type natriuretic peptide (BNP) max, serum total bile acid (TBA) and Lactobacillus. HR: hazard ratio. (A) Left panel represents the results of serum total bile acid (TBA) and Lactobacillus for all-cause death in ACS patients; right panel represents the results of serum total bile acid (TBA) and Lactobacillus in AMI patients (upper) and UAP patients (lower). (B) Left panel represents the results of serum total bile acid (TBA) and Lactobacillus for all-cardiac death in ACS patients; right panel represents the results of serum total bile acid (TBA) and Lactobacillus in AMI patients (upper) and UAP patients (lower).
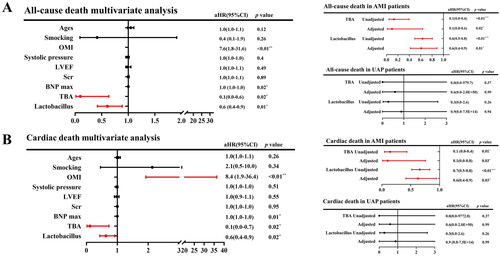
The same procedure was used to analyse the variables associated with cardiac death. multi-COX regression analysis showed an independent relationship between serum TBA, Lactobacillus, and a reduced risk of cardiac death (serum TBA: aHR = 0.1, 95% CI: 0.0–0.7, p = .02; Lactobacillus aHR = 0.6, 95% CI: 0.4–0.9, p = .02) (). Subgroup analysis showed that in the AMI subgroup, COX analysis showed that serum TBA and Lactobacillus were significantly correlated with all-cause death (serum TBA: aHR = 0.1, 95% CI: 0.0–0.8, p = .03; Lactobacillus aHR = 0.6, 95% CI: 0.4–0.9, p = .03) () by adjusting the same variables as above.
3.4. The serum TBA moderated interaction between gut Lactobacillus and SYNTAX scores and cardiac mortality
Multivariate logistic regression analysis showed a significant relationship between gut Lactobacillus levels and serum TBA levels (). Lactobacillus possesses several active enzymes related to bile acid metabolism. Changes in the level of Lactobacillus may lead to changes in bile acids levels and composition. Our recent work demonstrated Lactobacillus was a predictor for coronary lesions and mortality. Next, we attempted to test: can serum TBA explain or mediate this relationship through regulatory analysis and mediation? By using regulation and mediation analysis, we found although Lactobacillus and TBA were correlated with SSs, their interaction terms were not significantly correlated with SSs and prognosis (p > .05, Supplementary Table 2). However, we identified a significant moderated effect of Lactobacillus predicting SSs and prognosis. Serum TBA played an important role as a mediation effect in the following aspects: Lactobacillus (17.0%, p < .05) → SS association (per 1 standard deviation (SD) increase), Lactobacillus (43.0%, p < .05) → all-cause death (per 1 SD increase) and Lactobacillus (45.4%, p < .05) → cardiac death (per 1 SD increase). Statistical values demonstrating the significance of the pathways are presented in and Supplementary Table 3.
Figure 6. The coefficients relating Lactobacillus with SYNTAX score, the risk of all-cause death and the risk of cardia death, and the mediation effect of serum TBA. (A) Association between Lactobacillus and SYNTAX score mediated by serum TBA. (B) Association between Lactobacillus and the risk of allcause death mediated by serum TBA. (C) Association between Lactobacillus and the risk of cardiac death mediated by serum TBA. β: coefficients in regression analysis; TE: total effect; ADE: average direct effect; ACME: average causal mediation effect; CI: confidence interval.
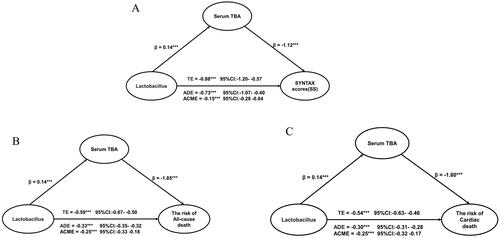
4. Discussion
In recent years, cardiovascular disease, particularly CAD, had remained the leading cause of mortality and morbidity [Citation29]. ACS is one of the more severe types of CAD. In order to prevent exacerbation and administer the appropriate treatments, it is imperative to further explore the clinical characteristics of patients with ACS.
Three hundred and sixty ACS patients were included in this study to explore the relationship between serum TBA and the severity, prognosis and Lactobacillus. The overall characteristics of the patients were consistent with previous clinical studies of obstructive CAD [Citation30]. Our research mainly found that: (1) lower level of serum TBA was highly correlated with the coronary lesion severity, myocardial damage, inflammation and gut Lactobacillus levels in ACS patients, especially in AMI; (2) lower level of serum TBA was highly associated with mortality (including all-cause mortality and cardiac mortality) after PCI in ACS, especially in AMI; (3) serum TBA had a partial mediating effect rather than regulating effect between gut Lactobacillus and coronary lesions and prognosis in ACS.
Bile acid is an endogenous compound derived from cholesterol. Previous studies have suggested that bile acid metabolism participates in the progress of atherosclerosis by regulating cholesterol levels [Citation1]. The amassing of bile acid in the organism was associated with cholesterol overload and was a risk factor for atherosclerosis [Citation6,Citation7]. Some studies currently recommend the combination of cholesterol-lowering therapy with bile acid sequestrants to increase patient benefit [Citation6]. However, several recent research findings had come to different conclusions. Charach et al. [Citation31] showed that the reduction of bile acid production (measured by bile acid in faeces) could result in atherosclerosis and increase the morbidity and mortality of cardiovascular diseases. Unfortunately, the validation of bile acid levels in vivo was lacking in this study. A recent study showed a relationship between serum TBA and the development of coronary lesions [Citation32], and the higher level of serum TBA was a protective factor for atherosclerosis. This finding was clearly inconsistent with the previous association of serum TBA with atherosclerosis. At present, there is no study focused on serum TBA levels in ACS patients. Our study found for the first time that serum TBA was an independent protective factor for coronary lesions and the risk of mortality. The same findings were confirmed in the AMI subgroup. It should be noted that in this study, there was no statistically significant difference in lipids between different groups of coronary lesion severity. These results suggested that bile acid metabolism might have a mechanism that was independent of the cholesterol metabolism of atherosclerosis.
The size of the circulating bile acid pool in ACS patients has not received enough attention. There were many reasons for this phenomenon. One reason was that bile acid levels were related to liver metabolic function, and some ACS patients had an acute liver injury, which may lead to abnormal bile acid metabolism. Another reason was that serum bile acid levels in most ACS patients were in the normal range, leading to a lack of awareness of it. In addition, the composition of bile acids was complex, and some studies focused on the relationship between a single component of bile acids and atherosclerosis. It was noteworthy these studies mostly used additional supplementation with a specific bile acid component, which was inconsistent with the absence of hyperbileacidemia in ACS. In our study, patients with combined hepatobiliary system disease were excluded. There was no difference in liver function among patients with different severity of coronary lesions. Our results suggested that lower levels of serum bile acid may indicate patients with more severe coronary lesions and a worse prognosis.
Emerging evidence had identified the association between gut microbiota and CAD. Malik et al. showed that supplementing Lactobacillus Plantarum 299v to male atherosclerosis patients could improve the function of vascular endothelial and reduce the severe systemic reaction [Citation33]. Lam et al. found that supplementing Lactobacillus Plantarum 299v could reduce the myocardial infarction area of mice with ischemia–reperfusion [Citation34]. The research on the mechanism of Lactobacillus in ACS is still under study. Previous studies by our team had found that Lactobacillus was associated with coronary lesions and prognosis in ACS [Citation16]. Bile acids were one of the fermented metabolites of gut microbiota. However, no clinical studies have discussed the association of serum bile acid with coronary lesions and prognosis in patients with ACS, and no studies have further discussed whether this relationship is associated with Lactobacillus.
There was an interaction between Lactobacillus and bile acid. Lactobacillus can influence the size and composition of the bile acid pool. Lactobacillus colonizing in the upper small intestine affected the absorption of cholesterol, which was the main material for the synthesis of bile acid [Citation10]. Lactobacillus was rich in active bile salt hydrolase (BSH) enzymes, which catalysed the deconjugation of bile acid in the intestine. This resulted in higher excretion of unconjugated bile acid, an amount that must be replenished by de novo synthesis from cholesterol [Citation10]. Deconjugation was the first step in the synthesis of intestinal bile acid, the products of which were used in the synthesis of secondary bile acid. Secondary bile acids not only consumed primary bile acid, but they also altered the size of the bile acid pool by regulating the activity of the rate-limiting enzyme in the bile acid synthetic pathway in the liver [Citation35]. Lactobacillus also regulated the ratio of bile acid components, which was related to its possession of several enzymes involved in bile acid synthesis. In addition to BSH enzymes, the hydroxysteroid dehydrogenases (HSDH) were involved in the oxidation and epimerization of the 3-, 7- or 12-hydroxyl groups of bile acid. Lactobacillus also participated in the esterification of bile acid to reduce solubility and regulate the reabsorption of intestinal bile acid [Citation36]. Animal experiments showed that Lactobacillus rhamnosus GG (LGG) supplementation decreased hepatic bile acid suppression of bile acid de novo synthesis in the liver of mice and enhanced bile acid excretion [Citation37]. L. plantarum CCFM8661 induced hepatic bile acid synthesis and increased faecal bile acid excretion in the mice [Citation38]. Lactobacillus gasseri RW2014 improved the abundance of bile acids, especially secondary bile acid components, in hyperlipidaemic rats [Citation39]. However, the relationship between bile acids and coronary lesions and prognosis in patients with ACS has not been adequately studied. There is a lack of investigation on whether the effect of Lactobacillus on bile acids is related to coronary lesions and prognosis in ACS patients. This study was the first to explore the relationship between serum TBAs and coronary lesions and prognosis in patients with ACS. We also found that there was a correlation between serum TBAs and gut Lactobacillus in ACS patients. Therefore, we further explored the potential association between serum TBAs, gut Lactobacillus, and coronary lesions and prognosis.
We hypothesized that gut Lactobacillus may exert a protective effect against atherosclerosis through its involvement in bile acid metabolism. We used a moderating effect model to verify whether there was an interaction between gut Lactobacillus and serum TBA. The results showed that serum TBA partially mediated, rather than modulated, the association between Lactobacillus and coronary artery lesions and risk of death. This meant that Lactobacillus exerted a protective effect on atherosclerosis by participating in bile acid metabolism, not that Lactobacillus acted differently roles at different bile acid levels.
Although the individual components of bile acids, such as cholic acid (CA), chenodeoxycholic acid (CDCA), deoxycholic acid (DCA) and lithocholic acid (LCA), have different relationships with atherosclerosis, vascular endothelial dysfunction and cardiomyocyte injury, each with different and complex mechanisms of action [Citation40,Citation41]. The mediated regulation of the protective effect of serum TBA on gut Lactobacillus in atherosclerosis was a combination of these complex effects. This was the first time that the involvement of bile acids as metabolites of gut Lactobacillus in atherosclerotic lesions had been proposed and verified in ACS. This finding, on the one hand, provided a research direction to explore the mechanism of action of Lactobacillus in atherosclerosis and also showed that the exploration of bile acid anabolism and bile acid composition was more significant than the study of bile acid levels for the ACS treatment.
5. Limitations
There were still some limitations and shortcomings in this study. For starters, the single-centre study only included Chinese subjects with a confirmed diagnosis of ACS. More patients with ACS and investigations of diverse ethnic groups are needed to back up our findings. Second, just serum TBA was tested in our investigation, leaving out the particular bile acid components. More metabolomic research is needed to back up our findings. Last but not least, the relationship between bile acid composition and gut microbiota was not explored in our study and needs further in-depth investigation.
6. Conclusions
Serum TBA levels were highly and independently correlated with the coronary lesion severity and the probability of occurrence of all-cause death and cardiac death in ACS. Serum TBA could be a therapeutically relevant and low-cost biomarker for predicting coronary artery lesion severity and guiding therapy of ACS. Additionally, our mediation model revealed that the TBA level mediated the protective effect of gut Lactobacillus in ACS patients.
Author contributions
Ting-Ting Liu, Jie Wang, Yan Liang, Xiao-Yuan Wu, Wen-Qing Li, Yu-Hang Wang, An-Ran Jing, Miao-Miao Liang, Li Sun, Jing Dou and Jing-Yu Liu participated in the collection, analysis, and interpretation of the data and drafting of the manuscript. Yin Liu and Jing Gao designed this research. Jing Gao participated in the conception and design of this study. Jing Gao and Yin Liu contributed to the critical revision of the manuscript. All authors agree to be responsible for all aspects of the study. All authors read and approve the final version of the manuscript. All the authors meet the criteria for authorship as per the ICMJE criteria.
Supplemental Material
Download MS Word (33.1 KB)Acknowledgements
The authors wish to thank Tianjin Chest Hospital Biobank for providing standardized frozen plasma samples, and special appreciation to the patients for their participation.
Disclosure statement
The authors Ting-Ting Liu, Jie Wang, Yan Liang, Xiao-Yuan Wu, Wen-Qing Li, Yu-Hang Wang, An-Ran Jing, Miao-Miao Liang, Li Sun, Jing Dou, Jing-Yu Liu, Yin Liu, Zhuang Cui and Jing Gao declare that they have no conflict of interest for this article. Part of the abstract of this article was previously published in the 2023 ACC Annual Meeting. All authors confirm that this work is original and the full article has not been published elsewhere, nor is it currently under consideration for publication elsewhere.
Data availability statement
The authors confirm that the data supporting the findings of this study are available within the article and its supplementary materials.
Additional information
Funding
References
- Zheng X, Chen T, Jiang R, et al. Hyocholic acid species improve glucose homeostasis through a distinct TGR5 and FXR signaling mechanism. Cell Metab. 2021;33(4):1–13. doi:10.1016/j.cmet.2020.11.017.
- Wahlström A, Sayin SI, Marschall HU, et al. Intestinal crosstalk between bile acids and microbiota and its impact on host metabolism. Cell Metab. 2016;24(1):41–50. doi:10.1016/j.cmet.2016.05.005.
- De Aguiar Vallim TQ, Tarling EJ, Edwards PA. Pleiotropic roles of bile acids in metabolism. Cell Metab. 2013;17(5):657–669. doi:10.1016/j.cmet.2013.03.013.
- Murakami S, Fujita M, Nakamura M, et al. Taurine ameliorates cholesterol metabolism by stimulating bile acid production in high-cholesterol-fed rats. Clin Exp Pharmacol Physiol. 2016;43(3):372–378. doi:10.1111/1440-1681.12534.
- Zhang B, Kuipers F, de Boer JF, et al. Modulation of bile acid metabolism to improve plasma lipid and lipoprotein profiles. J Clin Med. 2021;11(1):4. doi:10.3390/jcm11010004.
- Alder M, Bavishi A, Zumpf K, et al. A meta-analysis assessing additional LDL-C reduction from addition of a bile acid sequestrant to statin therapy. Am J Med. 2020;133(11):1322–1327. doi:10.1016/j.amjmed.2020.03.056.
- Mach F, Baigent C, Catapano AL, et al. 2019 ESC/EAS Guidelines for the management of dyslipidaemias: lipid modification to reduce cardiovascular risk [published correction appears in Eur Heart J. 2020;41(44):4255]. Eur Heart J. 2020;41(1):111–188. doi:10.1093/eurheartj/ehz455.
- Mohr FW, Morice MC, Kappetein AP, et al. Coronary artery bypass graft surgery versus percutaneous coronary intervention in patients with three-vessel disease and left main coronary disease: 5-year follow-up of the randomized, clinical SYNTAX trial. Lancet. 2013;381(9867):629–638. doi:10.1016/S0140-6736(13)60141-5.
- Ridlon JM, Harris SC, Bhowmik S, et al. Consequences of bile salt biotransformations by intestinal bacteria [published correction appears in Gut Microb. 2016;7(3):262]. Gut Microbes. 2016;7(1):22–39. doi:10.1080/19490976.2015.1127483.
- Kriaa A, Bourgin M, Potiron A, et al. Microbial impact on cholesterol and bile acid metabolism: current status and future prospects. J Lipid Res. 2019;60(2):323–332. doi:10.1194/jlr.R088989.
- Lye HS, Rusul G, Liong MT. Mechanisms of cholesterol removal by lactobacilli under conditions that mimic the human gastrointestinal tract. Int Dairy J. 2010;20(3):169–175. doi:10.1016/j.idairyj.2009.10.003.
- Zhou X, Li J, Guo J, et al. Gut-dependent microbial translocation induces inflammation and cardiovascular events after ST-elevation myocardial infarction. Microbiome. 2018;6(1):66. doi:10.1186/s40168-018-0441-4.
- Lam V, Su J, Koprowski S, et al. Intestinal microbiota determine severity of myocardial infarction in rats. FASEB J. 2012;26(4):1727–1735. doi:10.1096/fj.11-197921.
- Haghikia A, Li XS, Liman TG, et al. Gut microbiota-dependent trimethylamine N-oxide predicts risk of cardiovascular events in patients with stroke and is related to proinflammatory monocytes. Arterioscler Thromb Vasc Biol. 2018;38(9):2225–2235. doi:10.1161/ATVBAHA.118.311023.
- Gao J, Yan KT, Wang JX, et al. Gut microbial taxa as potential predictive biomarkers for acute coronary syndrome and post-STEMI cardiovascular events. Sci Rep. 2020;10(1):2639. doi:10.1038/s41598-020-59235-5.
- Gao J, Wang J, Zhao LL, et al. Gut Lactobacillus level is a predictive marker for coronary atherosclerotic lesions progress and prognosis in patients with acute coronary syndrome. Front Cell Infect Microbiol. 2021;11:687827. doi:10.3389/fcimb.2021.687827.
- Wang J, Zhang J, Lin X, et al. DCA-TGR5 signaling activation alleviates inflammatory response and improves cardiac function in myocardial infarction. J Mol Cell Cardiol. 2021;151:3–14. doi:10.1016/j.yjmcc.2020.10.014.
- Fan Y, Li Y, Chen Y, et al. Comprehensive metabolomic characterization of coronary artery diseases. J Am Coll Cardiol. 2016;68(12):1281–1293. doi:10.1016/j.jacc.2016.06.044.
- Babu AF, Koistinen VM, Turunen S, et al. Identification and distribution of sterols, bile acids, and acylcarnitines by LC–MS/MS in humans, mice, and Pigs-A qualitative analysis. Metabolites. 2022;12(1):49. doi:10.3390/metabo12010049.
- Ibanez B, James S, Agewall S, et al. 2017 ESC Guidelines for the management of acute myocardial infarction in patients presenting with ST-segment elevation: the Task Force for the Management of Acute Myocardial Infarction in patients presenting with ST-segment elevation of the European Society of Cardiology (ESC). Eur Heart J. 2018;39(2):119–177. doi:10.1093/eurheartj/ehx393.
- Lee SH, Yun Y, Kim SJ, et al. Association between cigarette smoking status and composition of gut microbiota: population-based cross-sectional study. J Clin Med. 2018;7(9):282. doi:10.3390/jcm7090282.
- Martino C, Zaramela LS, Gao B, et al. Acetate reprograms gut microbiota during alcohol consumption. Nat Commun. 2022;13(1):4630. doi:10.1038/s41467-022-31973-2.
- Heilig HG, Zoetendal EG, Vaughan EE, et al. Molecular diversity of Lactobacillus spp. and other lactic acid bacteria in the human intestine as determined by specific amplification of 16S ribosomal DNA. Appl Environ Microbiol. 2002;68(1):114–123. doi:10.1128/AEM.68.1.114-123.2002.
- Nadkarni MA, Martin FE, Jacques NA, et al. Determination of bacterial load by real-time PCR using a broad-range (universal) probe and primers set. Microbiology. 2002;148(Pt 1):257–266. doi:10.1099/00221287-148-1-257.
- Camp RL, Dolled-Filhart M, Rimm DL. X-tile: a new bio-informatics tool for biomarker assessment and outcome-based cut-point optimization. Clin Cancer Res. 2004;10(21):7252–7259. doi:10.1158/1078-0432.CCR-04-0713.
- Hayes AF. Introduction to mediation, moderation, and conditional process analysis: a regression-based approach. New York: Guilford Press; 2018.
- Sousa-Uva M, Neumann FJ, Ahlsson A, et al. 2018 ESC/EACTS Guidelines on myocardial revascularization. Eur J Cardiothorac Surg. 2019;55(1):4–90. doi:10.1093/ejcts/ezy289.
- Thuijs DJFM, Kappetein AP, Serruys PW, et al. Percutaneous coronary intervention versus coronary artery bypass grafting in patients with three-vessel or left main coronary artery disease: 10-year follow-up of the multicentre randomised controlled SYNTAX trial [published correction appears in Lancet. 2020;395(10227):870]. Lancet. 2019;394(10206):1325–1334. doi:10.1016/S0140-6736(19)31997-X.
- Weiwei C, Runlin G, Lisheng L, et al. Outline of the report on cardiovascular diseases in China, 2014. Eur Heart J Suppl. 2016;18(Suppl. F):F2–F11. doi:10.1093/eurheartj/suw030.
- Han Z, Lai L, Pu Z, et al. A nomogram to predict patients with obstructive coronary artery disease: development and validation. Cardiovasc Innov Appl. 2021;5(4):245–255. doi:10.15212/CVIA.2021.0001.
- Charach G, Argov O, Geiger K, et al. Diminished bile acids excretion is a risk factor for coronary artery disease: 20-year follow up and long-term outcome. Therap Adv Gastroenterol. 2018;11:1756283X17743420. doi:10.1177/1756283X17743420.
- Li W, Shu S, Cheng L, et al. Fasting serum total bile acid level is associated with coronary artery disease, myocardial infarction and severity of coronary lesions. Atherosclerosis. 2020;292:193–200. doi:10.1016/j.atherosclerosis.2019.11.026.
- Malik M, Suboc TM, Tyagi S, et al. Lactobacillus plantarum 299v supplementation improves vascular endothelial function and reduces inflammatory biomarkers in men with stable coronary artery disease. Circ Res. 2018;123(9):1091–1102. doi:10.1161/CIRCRESAHA.118.313565.
- Lam V, Su J, Koprowski S, et al. Intestinal microbiota determine severity of myocardial infarction in rats. FASEB J. 2012;26(4):1727–1735. doi:10.1096/fj.11-197921
- Sayin SI, Wahlström A, Felin J, et al. Gut microbiota regulates bile acid metabolism by reducing the levels of tauro-beta-muricholic acid, a naturally occurring FXR antagonist. Cell Metab. 2013;17(2):225–235. doi:10.1016/j.cmet.2013.01.003.
- Larabi AB, Masson HLP, Bäumler AJ. Bile acids as modulators of gut microbiota composition and function. Gut Microbes. 2023;15(1):2172671. doi:10.1080/19490976.2023.2172671.
- Liu Y, Chen K, Li F, et al. Probiotic Lactobacillus rhamnosus GG prevents liver fibrosis through inhibiting hepatic bile acid synthesis and enhancing bile acid excretion in mice. Hepatology. 2020;71(6):2050–2066. doi:10.1002/hep.30975.
- Zhai Q, Liu Y, Wang C, et al. Lactobacillus plantarum CCFM8661 modulates bile acid enterohepatic circulation and increases lead excretion in mice. Food Funct. 2019;10(3):1455–1464. doi:10.1039/c8fo02554a.
- Li X, Xiao Y, Huang Y, et al. Lactobacillus gasseri RW2014 ameliorates hyperlipidemia by modulating bile acid metabolism and gut microbiota composition in rats. Nutrients. 2022;14(23):4945. doi:10.3390/nu14234945.
- Rivard AL, Steer CJ, Kren BT, et al. Administration of tauroursodeoxycholic acid (TUDCA) reduces apoptosis following myocardial infarction in rat. Am J Chin Med. 2007;35(2):279–295. doi:10.1142/S0192415X07004813.
- Gan XT, Ettinger G, Huang CX, et al. Probiotic administration attenuates myocardial hypertrophy and heart failure after myocardial infarction in the rat. Circ Heart Fail. 2014;7(3):491–499. doi:10.1161/CIRCHEARTFAILURE.113.000978.