Abstract
Background
Studies demonstrated that outpatient aerobic exercise programs (aEP) can significantly decrease aortic stiffness in people with metabolic syndrome (MetS). There is some limited data that remotely supervised home-based aEP can also improve arterial stiffness in this population. We aimed to evaluate the changes in the arterial wall parameters after the 2-month ambulatory supervised aEP followed by the 6-month home-based aEP with and without targeting of heart rate (HR) by electrocardiogram (ECG) in people with MetS.
Methods
In this prospective study (ClinicalTrials.gov identifier: NCT05592704) 132 MetS subjects (mean age 52.44 ± 6.26 years, 54.55% female) were evaluated. At first, all subjects participated in the 2-month ambulatory supervised aEP, which consisted of 40 individual aerobic training sessions on a cycle ergometer 5 times/week for 40 min and received the recommendations for home-based training. Then the study (n = 66) and the control (n = 66) groups participated in the 6-month home-based aEP, but only the study group subjects targeted their HR using ECG monitor connected to the smartphone during workouts. Arterial stiffness parameters and carotid artery intima-media thickness (cIMT) were evaluated in all participants at baseline and after 8 months.
Results
After 8 months, carotid-femoral pulse wave velocity (c-f PWV) significantly reduced in both groups (−12.22% in the study group vs. −7.85% in the control group, all p < .001) without a significant between-group difference (p = 0.144). A significant improvement of carotid-radial pulse wave velocity (c-r PWV) was observed only in the study group (-11.37%, p < .001, d = −0.671) with significant between-group difference (p < .001). The reduction of c-r PWV after 8 months of aEP occurred when c-r PWV at baseline was in the 2nd quartile (>7.90 m/s). A significant decrease of 3.32% in cIMT was present only in the study group (p = .032, d = −0.288).
Conclusions
The combination of 2-month ambulatory supervised aEP and successive 6-month home-based aEP targeted by HR monitoring using ECG improved arterial properties in MetS subjects more than the same combination without HR targeting, leading to the greater reduction of c-r PWV and cIMT.
Key Messages
The combination of 2-month ambulatory supervised aEP and successive 6-month home-based aEP targeted by HR monitoring using ECG improved arterial properties in MetS subjects more than the same combination without HR targeting, leading to the greater reduction of c-r PWV and cIMT.
This study foregrounds the importance of home-based training with HR targeting using ECG in people with MetS.
1. Introduction
People with metabolic syndrome (MetS), a cluster of elevated blood pressure (BP), dyslipidemia, impaired glucose tolerance and abdominal obesity [Citation1], are at increased risk of cardiovascular (CV) disease and all-cause mortality [Citation2,Citation3]. One of the reasons for the increased CV risk in MetS are changes in arterial parameters, in particular, increased stiffness of large arteries and thickness of common carotid artery intima-media (cIMT) [Citation4]. It was demonstrated that arterial impairment increases with the increase in number of different cardiometabolic risk factors related to MetS [Citation5]. Arterial stiffness itself is an independent and strong predictor of future CV events and all-cause mortality [Citation6]. The predictive value of arterial stiffness parameter, such as aortic pulse wave velocity (PWV), and carotid intima-media thickness (cIMT) for CV risk was also confirmed in people with MetS [Citation7]. Therefore, to reduce the health burden related to MetS is of high importance in primary CV prevention, given that the prevalence of MetS is estimated to be ∼25% among the adult population and is increasing rapidly [Citation8,Citation9].
Physical activity (PA), in particular regular exercise, is a key component in preventing MetS and reducing its negative impact on health [Citation10]. However, the level of PA is reported to be insufficient in individuals with MetS [Citation11]. The lack of adherence to PA recommendations is recognized as a major concern [Citation12]. The recommendations on PA lack information on how to ensure that individuals maintain the proper intensity of PA over time and how to motivate sedentary individuals to exercise more or even start to exercise [Citation12]. Nevertheless, the level of PA can be increased and the appropriate intensity of PA can be maintained by the support of healthcare professionals and by using technological devices [Citation12].
Studies demonstrated the importance of supervised aerobic exercise programs (aEP) in people with MetS, as they can not only improve cardiometabolic profile, cardiorespiratory fitness, health-related quality of life but also significantly decrease aortic stiffness [Citation13–15]. However, supervised exercise programs are usually conducted at the outpatient setting, are short-term and not widely available.
Home-based exercise training using technologies, such as wearable PA tracking devices and mobile phones, is becoming a preferred approach as an intervention due to reduced cost and increased accessibility [Citation16]. It was demonstrated that PA interventions that combine wearable PA trackers with health professional consultations could improve the levels of PA among people with cardiometabolic conditions [Citation17,Citation18]. In addition, wearable PA tracking devices through a smartphone application could serve as an important motivation tool to increase PA and help individuals with MetS to be more engaged in regular PA [Citation19]. However, these trackers as usual are monitoring heart rate (HR) just by pulse wave signals that are not so precise as obtained by electrocardiogram (ECG).
There is some data demonstrating that home-based exercise training improves aortic stiffness and endothelial function in obese people with the increased CV risk [Citation20]. Therefore, we hypothesized that the program consisting of the outpatient ambulatory aEP followed by the ECG targeted HR home-based training could improve arterial stiffness parameters even more than the aEP without ECG HR targeting during home-based training. To the best of our knowledge, there are no studies assessing arterial stiffness changes under such a combination of PA interventions in people with MetS.
The aim of this study was to evaluate the changes in arterial stiffness parameters and cIMT after the 2-month ambulatory supervised aEP followed by the 6-month home-based aEP with and without targeting of HR by ECG in people with MetS.
2. Materials and methods
2.1. Study design and population
This study is a prospective study that was carried out between 2017 and 2019 at the outpatient clinic InMedica, Lithuania. Prior to patient enrollment, the study was approved by the Lithuanian Bioethics Committee (protocol number: PFAEV53, approval number: L-17-05/1, date of approval: 7 July 2017) and conducted according to the guidelines of the Declaration of Helsinki. Written informed consent was obtained from all subjects involved in the study. The study was registered at ClinicalTrials.gov (identifier: NCT05592704).
All individuals recruited for this study were participants of the ongoing Lithuanian High Cardiovascular Risk (LitHiR) primary prevention program [Citation9,Citation21]. Eligible participants were male (40–55 years) and female (50–65 years) having MetS, which was defined according to the National Cholesterol Education Program (NCEP ATP III) criteria [Citation1]. To diagnose MetS at least three of the following five criteria are required: abdominal obesity (waist circumference > 102 cm for men and > 88 cm for women); elevated BP (systolic BP ≥ 130 mmHg and/or diastolic BP ≥ 85 mmHg or current use of antihypertensive drugs); elevated fasting plasma glucose ≥ 5.6 mmol/L; hypertriglyceridemia (triglycerides ≥ 1.7 mmol/L); high-density lipoprotein cholesterol ≤ 1.03 mmol/L for men or ≤ 1.29 mmol/L for women [Citation1]. Patients with overt cardiovascular disease (previous myocardial infarction, coronary artery bypass surgery, percutaneous coronary intervention, previous stroke) as well as having severe heart failure symptoms or uncontrolled hypertension were excluded from the study.
Initially, 208 individuals, who agreed to participate, were allocated to the study (with HR targeting by ECG) and control (conventional care) groups by the study researcher using a random sampling method. The allocation to groups was not blinded. The statistical analysis included data from 132 participants (66 subjects per group) after propensity score matching (1:1).
2.2. Clinical assessment
All study participants were investigated at baseline and after 8 months. Their anthropometric parameters, including body mass index (BMI) and waist circumference were measured. Clinical data such as BP, heart rate (HR) at rest, venous blood sampling for blood lipids, and glucose, were assessed at rest between 7 am and 12 pm after an overnight fast and abstinence from caffeine and at least 2 h after smoking. BP was measured in a sitting position using oscillometric devices in accordance with the 2018 European Society of Cardiology/European Society of Hypertension guidelines [Citation22]. Arterial hypertension was defined as systolic BP ≥ 140 mmHg and/or diastolic BP ≥ 90 mmHg [Citation22] or the use of antihypertensive drugs. Obesity was defined as a BMI of ≥ 30 kg/m2 and overweight as a BMI of 25 − 29.9 kg/m2 [Citation22]. Smoking status was assessed from medical records.
We evaluated cardiorespiratory fitness (CRF) in all study participants by performing an incremental cardiopulmonary exercise test (CPET) on the cycle ergometer [Citation23] at baseline and after 8 months of aEP. An ergometer was set to a ramp mode from 15 to 30 W/min. After 2 min of cycling without resistance, the workload was constantly increased until the participants refused to continue exercise due to fatigue or other withdrawal symptoms. The exercise protocol lasted ∼8–12 min. During CPET, an electrocardiogram and BP were measured continuously. For gas exchange analysis a dedicated metabolic cart (Sensormedics, Vmax ENCORE 229, USA) was used. We evaluated ventilatory equivalents for O2 (VE/VO2, where VE is maximal minute ventilation and VO2 is oxygen uptake) and CO2 (VE/VCO2, where VCO2 is carbon dioxide output) as a function of work rate in order to establish the first ventilatory threshold (VT1) and the second ventilatory threshold (VT2). VT1 was identified at the point when VE/VO2 ratio inverted its trend in the presence of a still decreasing or constant VE/VCO2 and VT2 was identified at the point where the VE/VCO2 ratio inverted its trend [Citation23]. HR registered at VT1 during CPET was taken as the minimum training HR and HR registered at VT2 was the maximum training HR [Citation23–25]. HR at the ventilatory threshold is one of the CPET physiological parameters useful for aerobic exercise training intensity prescription and monitoring [Citation24]. Therefore, the values of minimum and maximum training HR were used for those purposes during our 6-month home-based training program. The CRF of the participant was described by maximal oxygen uptake (VO2 max ml/kg/min) and metabolic equivalents of the task (METs) achieved [Citation23,Citation25]. A criterion of maximal effort during CPET was the respiratory exchange ratio (VCO2/VO2) > 1.
Arterial stiffness parameters and wave reflection were assessed by an applanation tonometry system (SphygmoCor v.8.0; AtCor Medical, Sydney, Australia) with a high-fidelity micromanometer (Millar R; Millar Instruments, Houston, TX, USA), which was placed on the skin surface at the projection of radial, carotid and femoral arteries to obtain pulse pressure wave curves [Citation26]. Before the analysis, the distance between the surface markings of the sternal notch and the femoral artery was measured. Brachial BP and electrocardiogram were recorded simultaneously during the analysis. By using pulse wave curves, the system automatically computed the main parameters of arterial stiffness: aortic arterial stiffness (carotid–femoral pulse wave velocity [c-f PWV]), peripheral arterial stiffness (carotid-radial pulse wave velocity [c-r PWV]), pulse pressure (PP) in the aorta, aortic augmentation index (AIx) and aortic mean blood pressure (MBP).
Common carotid artery (CCA) intima-media thickness (IMT) and non dimensional index Quality Carotid Stiffness (QCS) of CCA were assessed using high-resolution echo-tracking technology (Art. Lab, Esaote Europe B.V.) at 1 cm proximal to the carotid bifurcation along 4 cm arterial segment [Citation27–29]. In this study, the mean values of right and left-side CCA measurements of IMT and QCS were used.
2.3. Ambulatory aerobic exercise program
The 2-month ambulatory aEP was carried out at the outpatient clinic and was supervised by a team of rehabilitation specialist and cardiologist.
Initially, all subjects from both groups participated in this individualized, HR-targeted aEP, which consisted of exercises on a cycle ergometer for 30–40 min/d, 5 d/week for 2 months (a total of 40 individual sessions for each participant). The aerobic training protocol was developed according to the standard recommendations for incremental aerobic exercise [Citation23,Citation25] and was adapted for untrained subjects with MetS.
Each aerobic exercise training on a cycle ergometer session started with a warm-up phase, which lasted 10 min. At first, the exercise intensity was set to 25 W for 5 min and then it was gradually increased until the training HR was reached. During the following phase of training, the intensity of exercise was maintained at such a level that the training HR remained constant. The last aerobic exercise training session phase of cool-down also lasted 10 min, during which the exercise intensity was gradually reduced to 25 W for the first 5 min and then was maintained for the last 5 min.
For the first 2 weeks of aEP, the intensity of exercise was maintained at HR registered at VT1 during CPET for the initial training. The intensity of exercise was gradually increased during the third and fourth week of aEP and reached the target HR, which was registered at VT2 or AT during CPET. The remaining 4 weeks of aEP were dedicated to maintaining the exercise intensity at the target HR. To adjust and maintain the exercise intensity and monitor real-time HR, we used the Ergoline Rehabilitation System-2 (Ergoline GmbH, Bitz, Germany). This system allowed us to control the load of the cycle ergometer so that the patient’s HR would be at a targeted level during the exercise training phase. Targeted HR was carefully monitored and always maintained and the deviation from the target HR was not significant. The pedaling frequency was set to 60 rpm throughout each session. The Borg Perceived Exertion 6–20 scale was used to assess the effort of the participant to exercise [Citation30].
2.4. Intervention tools
For real-time HR measuring during workouts, an electrocardiogram (ECG) HR monitor Polar H10 (Polar Electro Oy, Kempele, Finland) was used. This elastic chest strap with electrodes accurately measures HR during exercise [Citation31,Citation32] and is referred to as a reference device in research studies [Citation33,Citation34]. The Polar H10 monitors in our study were paired with smartphones (Huawei Y5H, Huawei Technologies Co., Ltd, Shenzhen, China) via Bluetooth connection. It allowed each smartphone to receive HR data (from ECG signals) during exercise from the assigned Polar H10 monitor. All HR data were recorded on the smartphone using the application that was specifically created for this study by information technology specialists in cooperation with study researchers. The application was developed for both Android and iOS using Flutter platform and was created for participants to self-monitor PA (physical activity) during the home-based training program with the possibility to review the data after the workout and/or send PA data to study researchers.
The main features of the application for PA self-monitoring included the ability to select the type of aerobic exercises (aerobics, cycling, walking, jogging, team sports), track workout time, and monitor HR in order to maintain it within the training HR range during a workout. The latter feature of the application was used to control the intensity of PA. According to the entered values of minimum and maximum training HR on the settings page, the application responded to the real-time HR during a workout and prompted the user to reduce or increase exercise intensity accordingly by changing the the display color in the main application page: indicating yellow if the real-time HR was too low, green – the HR was within the training HR range, and red – the HR was too high. A corresponding comment and an audio signal also appeared according to the real-time HR. Audible notifications made it possible to use the app during training without constantly monitoring the screen. The text in the application was presented in Lithuanian language. The application’s main page with features are presented in .
2.5. Motivational consultations and 6 months of home-based aerobic exercise training
After 2 months of aEP, all individuals participated in the motivational consultation which lasted about 30 min and was supervised by a rehabilitation specialist and cardiologist from the study. During this motivational consultation, each participant from both groups individually received detailed recommendations on the reduction of cardiovascular risk, focusing on the standard recommendations for PA and home-based training [Citation13,Citation35]. Participants from the study and control groups were encouraged to exercise individually for 6 months by performing aerobic exercises (e.g. walking, jogging, and cycling) for 30 min/d 5 times/week based on the standard recommendations for PA [Citation35].
Only the study group subjects were encouraged to exercise using the study application on the smartphone, which was paired with the Polar H10 monitor. They were also requested to select the type of PA on the main application page before each workout and to maintain the assigned exercise intensity during the workout by keeping the HR within the training HR range at least 50% of the workout time. The importance of using the application and devices during a workout was explained to participants with no expectation or judgment from the researchers. All smartphones were already prepared for use. They were with the latest operating system and had the study application and other necessary applications installed. Before issuing the devices to the participants, supervisors of the motivational consultation filled the application settings by entering the study number and the values of minimum and maximum training HR, which were individually assessed. A suitable size Polar H10 chest strap was selected for the participant. After the Polar H10 monitor was connected to the smartphone, the demonstration on how to use these devices and the study application was carried out. Every participant sent the control PA data to the database during the first motivational consultation. After the demonstration, the aforementioned devices, the instructions for the use and care of the devices, and the contact details of the responsible researcher were issued to each participant of the study group.
During the 6-month home-based training program, study group participants exercised with PA self-monitoring devices as instructed. Every participant was given full technical support during this home-based training program.
2.6. Statistical analysis
Power and sample size calculations were computed a priori using G*Power (Version 3.1.9.6; Universitat Kiel, Kiel, Germany). Based on previous studies, comparing the changes in various variables between the intervention group with aerobic training and the control group, a medium effect is usually achieved [Citation36–38]. To detect at least the medium effect (Cohen’s d = 0.5) between-group differences on the change in arterial parameters with at least 80% power at the 5% significance level (t-test) a sample of 64 individuals per group was required. Cohen’s d effect size of < 0.20 was categorized as small, 0.20–0.79 – as moderate, and > 0.80 – as large [Citation39]. In order to reduce bias and to increase the comparability between the study group and the control group for the statistical analysis, a propensity score matching was performed on baseline data of 208 participants, who were initially enrolled into the study. A propensity score matching was computed using XLSTAT (Version 2021.3.1) with optimal algorithm Mahalanobis distance matching method (variables used: glucose level at baseline, age; Caliper: 0.50 * Sigma; number of matches: 1:1; 95% CI; 0.001 tolerance). A sample of 66 subjects per group was obtained after propensity score matching analysis. The main statistical analysis included data from 132 participants and was performed using Jamovi (Version 2.2.5) [Citation40]. The Shapiro-Wilk test was applied to check for the Gaussian distribution of the data. Descriptive statistics are presented as means ± standard deviations for the continuous variables and as absolute numbers and percentages for the categorical variables. Continuous variables between the groups at baseline were compared by the independent samples t-test for normally distributed data and the Mann-Whitney U-test for non-normally distributed data. Categorical variables at baseline were analyzed with the χ2 test or Fisher’s exact test. To assess the improvement within groups, a paired t-test or the Wilcoxon signed-rank test was used to compare baseline to 8 months in the study group and the control group. Linear multiple regression was conducted to assess the between-group difference after 8 months of the program where the dependent variable (e.g. PP in the aorta at 8 months) is regressed against the group (i.e. study group vs control group) and other covariates (sex, age, systolic blood pressure and dependent variable at baseline) are controlled for. ANCOVA was used to test the dependence of change in c-r PWV on the magnitude of the baseline values stratified by quartiles in the study group adjusted for the change in systolic BP. The level of significance was set at a p value <.05.
The flowchart of the study enrollment and evaluation after propensity score matching analysis is detailed in .
3. Results
3.1. Descriptive statistics
A total of 132 participants with MetS were included into the study analysis (mean age 52.44 ± 6.26 years, 54.55% female). Most of the participants were obese (60.61%). The mean age in the study group was 52.05 ± 6.15 years and in the control group − 52.83 ± 6.38 years. All participants prior to the study indicated exercising less than the recommended 30 min of moderate aerobic physical activity 5 times/week. More detailed baseline characteristics of the participants with the intention to treat are presented in .
Table 1. Intention to treat: baseline characteristics of the participants.
At the baseline, there were no significant differences between the study and control groups according to age, sex, CRF parameters, blood and arterial parameters, main anthropometric parameters, smoking status, except for weight, arterial hypertension status, systolic BP, diastolic BP and HR at rest (p < .05).
Based on the PA data received through the study application, each participant from the study group on average had 22.02 ± 34.32 individual exercise training sessions with HR targeting. The average workout lasted for 30.16 ± 20.16 min.
3.2. Changes in cardiorespiratory fitness parameters
A significant increase in both CRF parameters, VO2 max, and METs achieved, was observed in both groups after 8 months of the program (all p < .001). The between-group differences found in the change of VO2 max and METs achieved were not significant (p = .945 and p = .907, respectively). All changes in CRF parameters after 8 months are presented in .
Table 2. Changes in cardiorespiratory fitness parameters after 8 months of aerobic training program.
3.3. Changes in arterial parameters
After 8 months of the program, statistically significant improvement of c-r PWV was observed only in the study group (a decrease of −11.37%, p < .001, d = −0.671) with a significant between-group difference (p = <.001). There was no statistically significant improvement in c-r PWV in the control group (p = .121, d = −0.251). The significant decrease in c-f PWV was observed in both groups (−12.22% in the study group vs. −7.85% in the control group, all p < .001). However, the between-group difference found in the change of c-f PWV was not significant (p = .144). The decrease in PP in the aorta was not statistically significant within the groups (all p > .05). In both groups, AIx values decreased after 8 months without statistical significance within groups (all p > .05). Only in the control group the decrease in aortic MBP was significant (p = .001, d = −0.528), however between-group difference was not significant (p = .512). The significant decrease of 3.32% in cIMT value was present only in the study group (p = .032, d = −0.288) but between group difference was not significant (p = .472). No statistically significant improvement in carotid stiffness was found in any of the groups (all p > .05). All changes in arterial parameters after 8 months are presented in and .
Figure 3. Percentage changes in arterial parameters after 8 months. Data are presented as a percentage. p value in bold denotes a statistically significant difference between groups (p <.05). * Statistically significant difference within group (p <.05). PP: pulse pressure; c-r PWV: carotid-radial artery pulse wave velocity; c-f PWV: carotid-femoral artery pulse wave velocity; AIXHR75: augmentation index; MBP: mean blood pressure; cIMT: common carotid artery intima–media thickness.
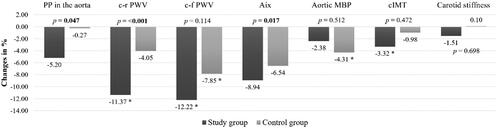
Table 3. Changes in arterial stiffness parameters after 8 months of aerobic training program.
Comparing the dynamics of arterial stiffness among the study participants according to the quartiles of the baseline c-r PWV, we observed that c-r PWV change after 8 months of the program was dependent on the initial magnitude of c-r PWV: the higher value of c-r PWV was at baseline, the greater reduction of peripheral arterial stiffness was achieved. The reduction of c-r PWV after 8 months of the program occurred when c-r PWV at baseline was in the 2nd quartile (> 7.90 m/s) (). Comparing the dynamics of arterial stiffness according to the quartiles of the baseline c-f PWV, we observed that the reduction of c-f PWV after 8 months of the program occurred even in the lowest c-f PWV quartile ().
Table 4. The dependence of change in carotid-radial pulse wave velocity on the magnitude of the baseline values in the study group.
Table 5. The dependence of change in carotid-femoral pulse wave velocity on the magnitude of the baseline values in the study group.
4. Discussion
This study aimed to assess the changes in arterial stiffness parameters and cIMT after the 2-month ambulatory supervised aEP on a cycle ergometer followed by the 6-month home-based aEP with and without targeting of HR by ECG in people with MetS. The analysis demonstrated that this combination of 2-month ambulatory supervised aEP and successive 6-month home-based ECG HR targeted aEP improved arterial stiffness and cIMT even more than only the 2-month ambulatory on a cycle ergometer followed just by PA recommendations during home-based training.
Supervised aEP demonstrated their positive effect on various arterial stiffness parameters among people with MetS in several previously reported studies. In a prospective study with 126 MetS subjects, 84 participants took part in a 2-month HR-targeted supervised aerobic exercise program, which 5% decreased their baseline arterial stiffness, measured as c-f PWV (p = .005) [Citation13]. Mora-Rodriguez et al. demonstrated that 6 months of the intense aerobic interval training improved arterial stiffness by 17% decreasing AIx (p = .048) in people with MetS [Citation41]. Although c-f PWV also decreased in the training group after the aerobic interval training, the between-group difference was not significant [Citation41]. Another study, which analyzed female patients with MetS, showed that the supervised aerobic exercise for 3 months decreased brachial-ankle PWV (p < .05) [Citation14]. However, the aforementioned studies were conducted only on an outpatient basis, with healthcare professionals directly supervising MetS patients during all of their training. In our study with 132 MetS subjects, 8 months of aEP consisted of the ambulatory supervised aEP on a cycle ergometer for 2 months followed by the home-based aEP for 6 months. Both, the study, and the control group, initially participated in the 2-month ambulatory supervised aEP and then only the study group exercised with additional HR targeting by ECG during the 6-month home-based training using wearable devices in order to achieve purposeful improvement of arterial stiffness and IMT parameters. The idea to analyze the changes in arterial parameters between initial and final visit during 8 months of aEP was supported by the previously mentioned study with 126 MetS subjects [Citation13]. The study demonstrated that the positive effect on arterial parameters was absent in the control group, where subjects were asked to exercise individually for 2 months based only on the given PA recommendations without targeting their HR in the outpatient clinic [Citation13]. Since the 2-month ambulatory supervised aEP has demonstrated its positive effect on arterial parameters in MetS subjects [Citation13], we think that it is crucial to apply it before the home-based aEP as an initial PA intervention, and that this combination of aEP can lead to a greater improvement in arterial parameters.
We demonstrated that c-f PWV significantly decreased in both groups (all p < .001) without significant difference between the groups. It can be explained by the fact that the control group subjects also participated in the 2-month ambulatory supervised aEP before the home-based training period without remote supervision. However, a greater reduction of c-f PWV was seen in the study group compared to the control group (−12.22% vs. −7.85%). This result again confirms that supervised outpatient aEP can effectively decrease c-f PWV, as it was demonstrated in previously mentioned our and other studies [Citation13,Citation41]. Also, it shows that the effect on c-f PWV can be residual even for 6 months after the supervised outpatient aEP.
Our main finding was that only in the study group subjects we observed a significant decrease of 11.37% in c-r PWV, (p < .001) with the between-group difference also being significant (p < .001). Only the study group participants used wearable devices and targeted HR additionally to maintain the assigned exercise intensity by keeping their training HR during home-based exercises, just as it was maintained during the outpatient ambulatory aEP. The significant c-r PWV decrease in our study can be explained by the properties of peripheral arteries, as they are more muscular and stiffer than central arteries and can larger adapt in response to exercise interventions as was indicated [Citation42]. Indeed, some studies have demonstrated that aerobic exercise can have even a greater effect on peripheral PWV rather than on central PWV [Citation43,Citation44]. Although the role of c-r PWV in predicting CV disease is not well established, this parameter still has clinical importance. Because of higher smooth muscle content and increased vascular smooth muscle tone in the peripheral arteries, c-r PWV can reflect the functional aspect of arterial stiffness [Citation45]. Moreover, c-r PWV can be recognized as a functional measure of arterial vasodilator reserve and maybe endothelial function [Citation46]. Overall, increased central or peripheral arterial stiffness can indicate the arterial wall damage and the progression of atherosclerosis.
In addition, we observed that the higher values of c-r PWV and c-f PWV were at baseline, the greater reduction of PWV was achieved after the 8-month aEP in the study group. The reduction of c-r PWV after 8 months of the program occurred when c-r PWV value at baseline was in the 2nd quartile (> 7.90 m/s). In line with our results, a meta-analysis involving studies with healthy adults and cardiometabolic patients demonstrated that participants with stiffer arteries at baseline (PWV > 8 m/s) also had a greater reduction in PWV after aerobic exercise interventions [Citation43]. Moreover, longer aerobic exercise interventions (> 10 weeks) tended to result in larger arterial stiffness reduction [Citation43]. Our results highlight the importance of prescribing HR targeted home-based aEP additionally to just provided physical activity recommendations in outpatient aEP in order to achieve a greater impact of a home-based exercise intervention on arterial wall parameters in subjects with MetS.
We have found only two recently published studies, which analyzed the effect of supervised home-based aEP using wearable devices on arterial stiffness in cardiometabolic patients. Scott et al. demonstrated that in obese adults at increased CV risk, 3 months of virtually supervised home-based high-intensity interval training significantly decreased aortic PWV, similarly to fully supervised outpatient aEP [Citation20]. Another study, which involved 89 patients with type 2 diabetes, showed that after the supervised walking program using wearable devices arterial stiffness parameters, measured as cardio-ankle vascular index and PP of the lower extremities, decreased significantly (p = .012, p < .001, respectively) [Citation47]. These studies confirm that virtually supervised home-based exercise can have an independent positive effect on arterial stiffness in people with MetS. Some advantage of our study is that during home-based training we used a precise HR monitoring by ECG instead of usually used pulse wave based HR monitoring.
Another finding of our study was the significant decrease in cIMT only in the study group subjects, who participated not only in the 2-month outpatient aEP but also in the 6-month ECG-targeted HR home-based aEP (p = .032). Although the between-group difference in the change of cIMT was not significant, the change within the study group (–3.32% from the baseline value) still shows some positive influence of the ECG targeted home-based aEP on the cIMT parameter. In support of our finding, it was previously demonstrated that cIMT did not change significantly after 2 months of outpatient aEP only [Citation13,Citation48]. A significant reduction in cIMT was recently demonstrated in patients with type 2 diabetes after 1 year of supervised aerobic exercise combined with resistance training [Citation44]. It seems that longer duration of the supervised aEP may also lead to a greater cIMT reduction in cardiometabolic subjects. It is also important to mention that these changes could be detected only when using high-resolution echo-tracking technology [Citation27,Citation29].
It is worth to highlight that in our study CRF parameters, VO2 max, and METs achieved, significantly improved in both the study and control groups. Therefore, only the monitoring of arterial parameters allowed us to determine the positive effect of 8-month aEP: supervised ambulatory and successive home-based HR-targeted aEP on muscular artery stiffness and cIMT.
The present study has some potential limitations which should be acknowledged. First, in this study, we did not evaluate participants’ medications properly, which always included BP and HR lowering and in most subjects dyslipidemia control by statins. Even after propensity score matching analysis, there were significant differences in weight, systolic BP, diastolic BP and resting HR between the study and control groups at baseline. To add, there was missing data due to the incomplete participation of some subjects in the clinical evaluation during the first and final visit. Moreover, we did not record any exercise training sessions during home-based aEP in the control group subjects because they were not supposed to use any wearable devices or somehow track their exercises. Meanwhile, the study group subjects used wearable devices during home-based aEP but their main purpose of use was to increase the motivation of the subject to exercise and help to maintain the appropriate PA intensity by targeting HR during exercises, not to receive all the PA data from participants. Hence, the comparison of the number of exercise sessions between the study and the control group during home-based aEP was not analyzed in our study. The important difference between groups besides the given PA recommendations was the HR targeting during home-based exercise sessions. Although our 8-month aEP program may not appear to be very cost-effective, it was a relatively short PA intervention and the tools used for self-monitoring were widely available and were intended to maintain the subject’s motivation to exercise. To add, the study cohort was very homogeneous in terms of age and clinical conditions, that limits the generalizability of our findings. Nevertheless, to the authors’ knowledge, this is the first study that evaluated changes after the supervised ambulatory outpatient aEP followed by the home-based HR targeted by ECG aEP in individuals with MetS.
5. Conclusions
The findings of this study suggest that the combination of 2-month ambulatory supervised aEP and successive 6-month home-based aEP targeted by HR monitoring using ECG improved arterial properties in MetS subjects more than the same combination without HR targeting, leading to the greater reduction of c-r PWV and cIMT. Our data foregrounds the importance of home-based training with HR targeting using ECG in people with MetS.
Author contributions
Conception and design: AL, JZ, IL, LR, PN, RK; data curation: JZ, IL, LR, PN, RK; investigation: JZ, IL; formal analysis: JZ, LR; writing – original draft: JZ, AL, LR, RK; critical review and editing: JZ, IL, LR, PN, AL, RK; Final approval of the version to be published: JZ, IL, LR, PN, AL, RK. All authors agree to be accountable for all aspects of the work.
Acknowledgments
We would like to express our gratitude to the outpatient clinic InMedica, where the clinical examination and motivational counseling of our participants were performed. We would also like to show our gratitude to the public institution ‘Informeda’ for its technical support while the course of this study.
Disclosure statement
No potential conflict of interest was reported by the author(s).
Data availability statement
The data that support the findings of this study are available from the corresponding author upon reasonable request.
References
- Expert Panel on Detection, Evaluation, and Treatment of High Blood Cholesterol in Adults. EXecutive summary of the third report of the national cholesterol education program (ncep) expert panel on detection, evaluation, and treatment of high blood cholesterol in adults (adult treatment panel iii). JAMA. 2001;285(19):1–13. doi: 10.1001/jama.285.19.2486.
- Mottillo S, Filion KB, Genest J, et al. The metabolic syndrome and cardiovascular risk: a systematic review and meta-analysis. J Am Coll Cardiol. 2010;56(14):1113–1132. doi: 10.1016/j.jacc.2010.05.034.
- Ju S-Y, Lee J-Y, Kim D-H. Association of metabolic syndrome and its components with All-Cause and cardiovascular mortality in the elderly: a meta-analysis of prospective cohort studies. Medicine. 2017;96(45):e8491. doi: 10.1097/MD.0000000000008491.
- Scuteri A, Najjar SS, Orru M, et al. The central arterial burden of the metabolic syndrome is similar in men and women: the Sardinia study. Eur Heart J. 2010;31(5):602–613. doi: 10.1093/eurheartj/ehp491.
- Sequí-Domínguez I, Cavero-Redondo I, Álvarez-Bueno C, et al. Association between arterial stiffness and the clustering of metabolic syndrome risk factors: a systematic review and meta-analysis. J Hypertens. 2021;39(6):1051–1059. doi: 10.1097/HJH.0000000000002754.
- Vlachopoulos C, Aznaouridis K, Stefanadis C. Prediction of cardiovascular events and all-cause mortality with arterial stiffness: a systematic review and meta-analysis. J Am Coll Cardiol. 2010;55(13):1318–1327. doi: 10.1016/j.jacc.2009.10.061.
- Ryliškytė L, Navickas R, Šerpytis P, et al. Association of aortic stiffness, carotid Intima-Media thickness and endothelial function with cardiovascular events in metabolic syndrome subjects. Blood Press. 2019;28(2):131–138. doi: 10.1080/08037051.2019.1569461.
- IDF Worldwide Definition of the Metabolic Syndrome. 2023. [cited 5 April 2023]. http://www.idf.org/metabolic-syndrome.
- Laucevičius A, Rinkūnienė E, Skorniakov V, et al. High-risk profile in a region with extremely elevated cardiovascular mortality. Hellenic J Cardiol. 2013;54:441–447.
- Myers J, Kokkinos P, Nyelin E. Physical activity, cardiorespiratory fitness, and the metabolic syndrome. Nutrients. 2019;11(7):1652. doi: 10.3390/nu11071652.
- Ekblom-Bak E, Halldin M, Vikström M, et al. Physical activity attenuates cardiovascular risk and mortality in men and women with and without the metabolic syndrome - a 20-year follow-up of a population-based cohort of 60-year-olds. Eur J Prev Cardiol. 2021;28(12):1376–1385. doi: 10.1177/2047487320916596.
- Collado-Mateo D, Lavín-Pérez AM, Peñacoba C, et al. Key factors associated with adherence to physical exercise in patients with chronic diseases and older adults: an umbrella review. Int J Environ Res Public Health. 2021;18(4):2023. doi: 10.3390/ijerph18042023.
- Slivovskaja I, Ryliskyte L, Serpytis P, et al. Aerobic training effect on arterial stiffness in metabolic syndrome. Am J Med. 2018;131(2):148–155. doi: 10.1016/j.amjmed.2017.07.038.
- Kang S-J, Kim E, Ko K-J. Effects of aerobic exercise on the resting heart rate, physical fitness, and arterial stiffness of female patients with metabolic syndrome. J Phys Ther Sci. 2016;28(6):1764–1768. doi: 10.1589/jpts.28.1764.
- Zupkauskiene J, Lauceviciene I, Navickas P, et al. Changes in health-related quality of life, motivation for physical activity, and the levels of anxiety and depression after individualized aerobic training in subjects with metabolic syndrome. Hellenic J Cardiol. 2022;66:41–51. doi: 10.1016/j.hjc.2022.04.003.
- Power S, Rowley N, Flynn D, et al. Home-based exercise for adults with overweight or obesity: a rapid review. Obes Res Clin Pract. 2022;16(2):97–105. doi: 10.1016/j.orcp.2022.02.003.
- Kirk MA, Amiri M, Pirbaglou M, et al. Wearable technology and physical activity behavior change in adults with chronic cardiometabolic disease: a systematic review and Meta-Analysis. Am J Health Promot. 2019;33(5):778–791. doi: 10.1177/0890117118816278.
- Hodkinson A, Kontopantelis E, Adeniji C, et al. Interventions using wearable physical activity trackers among adults with cardiometabolic conditions: a systematic review and meta-analysis. JAMA Netw Open. 2021;4(7):e2116382. doi: 10.1001/jamanetworkopen.2021.16382.
- Huh U, Tak YJ, Song S, et al. Feedback on physical activity through a wearable device connected to a mobile phone app in patients with metabolic syndrome: pilot study. JMIR Mhealth Uhealth. 2019;7(6):e13381. doi: 10.2196/13381.
- Scott SN, Shepherd SO, Hopkins N, et al. Home-Hit improves muscle capillarisation and ENOS/NAD(P)Hoxidase protein ratio in obese individuals with elevated cardiovascular disease risk. J Physiol. 2019;597(16):4203–4225. doi: 10.1113/JP278062.
- Laucevičius A, Rinkūnienė E, Ryliškytė L, et al. Primary prevention strategy for cardiovascular disease in Lithuania. Semin Cardiovasc Med. 2019;25(1):14–39. doi: 10.2478/semcard-2019-0004.
- Williams B, Mancia G, Spiering W, et al. 2018 ESC/ESH guidelines for the management of arterial hypertension: the task force for the management of arterial hypertension of the european society of cardiology (ESC) and the european society of hypertension (ESH). Eur Heart J. 2018;39(33):3021–3104. doi: 10.1093/eurheartj/ehy339.
- Mezzani A, Hamm LF, Jones AM, et al. Aerobic exercise intensity assessment and prescription in cardiac rehabilitation: a joint position statement of the European Association for Cardiovascular Prevention and Rehabilitation, the American Association of Cardiovascular and Pulmonary Rehabilitation and the Canadian Association of Cardiac Rehabilitation. Eur J Prev Cardiol. 2013;20(3):442–467. doi: 10.1177/2047487312460484.
- Mezzani A, Agostoni P, Cohen-Solal A, et al. Standards for the use of cardiopulmonary exercise testing for the functional evaluation of cardiac patients: a report from the exercise physiology section of the European Association for Cardiovascular Prevention and Rehabilitation. Eur J Cardiovasc Prev Rehabil. 2009;16(3):249–267. doi: 10.1097/HJR.0b013e32832914c8.
- Fletcher GF, Ades PA, Kligfield P, et al. Exercise standards for testing and training. Circulation. 2013;128(8):873–934. doi: 10.1161/CIR.0b013e31829b5b44.
- Laurent S, Cockcroft J, Van Bortel L, et al. Expert consensus document on arterial stiffness: methodological issues and clinical applications. Eur Heart J. 2006;27(21):2588–2605. doi: 10.1093/eurheartj/ehl254.
- Engelen L, Ferreira I, Stehouwer CD, et al. Reference values for arterial measurements collaboration reference intervals for common carotid intima-media thickness measured with echotracking: relation with risk factors. Eur Heart J. 2013;34(30):2368–2380. doi: 10.1093/eurheartj/ehs380.
- Palombo C, Kozakova M, Guraschi N, et al. Radiofrequency-based carotid wall tracking: a comparison between two different systems. J Hypertens. 2012;30(8):1614–1619. doi: 10.1097/HJH.0b013e328354dd44.
- Uejima T, Dunstan FD, Arbustini E, et al. Age-specific reference values for carotid arterial stiffness estimated by ultrasonic wall tracking. J Hum Hypertens. 2020;34(3):214–222. doi: 10.1038/s41371-019-0228-5.
- Williams N. The borg rating of perceived exertion (RPE) scale. Occup Med. 2017;67(5):404–405. doi: 10.1093/occmed/kqx063.
- Kingsley M, Lewis MJ, Marson RE. Comparison of polar 810s and an ambulatory ECG system for RR interval measurement during progressive exercise. Int J Sports Med. 2005;26(1):39–44. doi: 10.1055/s-2004-817878.
- Gilgen-Ammann R, Schweizer T, Wyss T. RR interval signal quality of a heart rate monitor and an ECG holter at rest and during exercise. Eur J Appl Physiol. 2019;119(7):1525–1532. doi: 10.1007/s00421-019-04142-5.
- Müller AM, Wang NX, Yao J, et al. Heart rate measures from Wrist-Worn activity trackers in a laboratory and free-living setting: validation study. JMIR Mhealth Uhealth. 2019;7(10):e14120. doi: 10.2196/14120.
- Budig M, Keiner M, Stoohs R, et al. Heart rate and distance measurement of two multisport activity trackers and a cellphone app in different sports: a cross-sectional validation and comparison field study. Sensors. 2021;22(1):180. doi: 10.3390/s22010180.
- Piepoli MF, Hoes AW, Agewall S, et al. 2016 European guidelines on cardiovascular disease prevention in clinical practice: the sixth joint task force of the European Society of Cardiology and Other Societies on Cardiovascular Disease Prevention in Clinical Practice (constituted by representatives of 10 societies and by invited experts) developed with the special contribution of the European Association for Cardiovascular Prevention & Rehabilitation (EACPR). Eur Heart J. 2016;37(29):2315–2381. doi: 10.1093/eurheartj/ehw106.
- Ramos RM, Coelho-Júnior HJ, Asano RY, et al. Impact of moderate aerobic training on physical capacities of hypertensive obese elderly. Gerontol Geriatr Med. 2019;5:2333721419859691. doi: 10.1177/2333721419859691.
- Nagyova I, Jendrichovsky M, Kucinsky R, et al. Effects of nordic walking on cardiovascular performance and quality of life in coronary artery disease. Eur J Phys Rehabil Med. 2020;56(5):616–624. doi: 10.23736/S1973-9087.20.06120-1.
- Hinchman CA, Cabral DF, Ciesla M, et al. Exercise engagement drives changes in cognition and cardiorespiratory fitness after 8 weeks of aerobic training in sedentary aging adults at risk of cognitive decline. Front Rehabil Sci. 2022;3:923141. doi: 10.3389/fresc.2022.923141.
- Cohen J. Statistical power analysis for the behavioral sciences. 2nd ed. New York (NY): Routledge Academic; 1988.
- The Jamovi Project. Jamovi (Version 2.3) [Computer Software]. 2022. [cited 2021 October 25]. https://www.jamovi.org
- Mora-Rodriguez R, Ramirez-Jimenez M, Fernandez-Elias VE, et al. Effects of aerobic interval training on arterial stiffness and microvascular function in patients with metabolic syndrome. J Clin Hypertens. 2018;20(1):11–18. doi: 10.1111/jch.13130.
- Green DJ, Hopman MTE, Padilla J, et al. Vascular adaptation to exercise in humans: role of hemodynamic stimuli. Physiol Rev. 2017;97(2):495–528. doi: 10.1152/physrev.00014.2016.
- Ashor AW, Lara J, Siervo M, et al. Effects of exercise modalities on arterial stiffness and wave reflection: a systematic review and meta-analysis of randomized controlled trials. PLOS One. 2014;9(10):e110034. doi: 10.1371/journal.pone.0110034.
- Magalhães JP, Melo X, Correia IR, et al. Effects of combined training with different intensities on vascular health in patients with type 2 diabetes: a 1-year randomized controlled trial. Cardiovasc Diabetol. 2019;18(1):34. doi: 10.1186/s12933-019-0840-2.
- Badhwar S, Chandran DS, Jaryal AK, et al. Regional arterial stiffness in central and peripheral arteries is differentially related to endothelial dysfunction assessed by brachial flow-mediated dilation in metabolic syndrome. Diab Vasc Dis Res. 2018;15(2):106–113. doi: 10.1177/1479164117748840.
- Liu Y, Beck A, Olaniyi O, et al. Carotid-Radial pulse wave velocity responses following hyperemia in patients with congestive heart failure. J Am Soc Hypertens. 2014;8(10):687–692. doi: 10.1016/j.jash.2014.07.025.
- Alonso-Domínguez R, Recio-Rodríguez JI, Patino-Alonso MC, et al. Acute effect of healthy walking on arterial stiffness in patients with type 2 diabetes and differences by age and sex: a pre-post intervention study. BMC Cardiovasc Disord. 2019;19(1):56. doi: 10.1186/s12872-019-1039-x.
- Donley DA, Fournier SB, Reger BL, et al. Aerobic exercise training reduces arterial stiffness in metabolic syndrome. J Appl Physiol. 2014;116(11):1396–1404. doi: 10.1152/japplphysiol.00151.2014.