Abstract
Background
Although abdominal aortic aneurysm (AAA) is associated with life-threatening complications, there are still limited reliable biomarkers for diagnostic purpose. MicroRNAs (miRNAs) have been proposed as the potential diagnostic and risk stratification markers of AAA patients, and we aim to evaluate the serum level of miR-1-3p and its diagnostic value in AAA.
Methods
This study included 200 AAA patients and 200 controls. Demographic data and clinical information were collected from the subjects’ medical records. Individual image analyses of AAA morphology were determined based on computed tomography angiography (CTA). The levels of serum miRNA expression were detected by quantitative real-time PCR. Bioinformatics tools were used to identify the target genes of miR-1-3p and their potential biological functions were further enriched.
Results
Serum miR-1-3p levels in the AAA group were significantly lower when compared with those in the control group in overall and subgroup comparisons. It was negatively related to WBC, CRP, maximal aneurysm diameter, area, and volume in AAA patients. Circulating miR-1-3p levels could significantly discriminate between AAA patients and healthy individuals with an area under the curve (AUC) of 0.672 (95% CI = 0.619-0.724, p < 0.001), a sensitivity of 84.5% and a specificity of 45.5%. Serum miR-1-3p was associated with a reduced risk of AAA even after adjustment for possible risk factors (OR = 0.440 per unit increase, 95% CI = 0.301-0.643, p < 0.001). And low levels of serum miR-1-3p could significantly elevate the risk of AAA in both univariate and multivariate logistic regression analyses with ORs of 4.076 and 4.136, respectively (all p < 0.001). Further GO enrichment analysis revealed that miR-1-3p was mainly involved in negative regulation of apoptotic process, sprouting angiogenesis, angiogenesis, positive regulation of blood vessel endothelial cell migration, positive regulation of cell proliferation, regulation of cell shape, etc.
Conclusions
MiR-1-3p can be used as a promising circulating biomarker in the development of AAA, and it may participate in multiple biological processes to play a crucial role in AAA pathogenesis.
1. Introduction
Cardiovascular diseases have gradually become a prevalent healthcare concern, of which abdominal aortic aneurysm (AAA) is an insidious but potentially life-threatening vascular disorder [Citation1,Citation2]. AAA is characterized by progressive structural impairment of the abdominal aorta, accompanied with accumulation of immune cells, inflammation, extracellular matrix degradation, and apoptosis of vascular smooth muscle cell (VSMC) [Citation3]. Patients with AAA are at a higher risk of developing severe aortic complications, and it’s crucial to recognize that the growth of aneurysm can increase the risk of acute aortic rupture, leading to internal bleeding and urgent conditions. Unfortunately, in most patients, AAA is asymptomatic and rarely causes pain before rupture [Citation4]. It is often diagnosed during routine cardiovascular screenings or in the course of investigating other medical conditions. Therefore, sensitive and effective biomarkers are needed to identify high-risk patients, improve disease stratification, and aid clinical decision-making [Citation5].
MicroRNAs (miRNAs) are a class of small non-coding RNAs with lengths 19-24 nts, which can regulate protein expression by binding to the 3′-untranslated region (3’UTR) of target genes [Citation6,Citation7]. They are stable and can be detected in blood or urine samples, making them a potentially valuable candidate as promising biomarkers for different disease conditions [Citation8,Citation9]. Mounting evidence suggested that miRNAs could modulate multiple cellular processes and signaling pathways, thereby exerting regulatory effects on the development of cardiovascular diseases [Citation10–12]. In human hypertrophic cardiomyopathy, downregulation of miR-1-3p has been found to be correlated with cardiac function [Citation13]. Novel findings from recent investigations suggest that the expression of miR-1-3p has the potential to be utilized as a valuable biomarker in the pathogenesis of cardiovascular events [Citation14,Citation15]. Our previous study found that miR-1-3p could participate in Ang II-mediated VSMC phenotypic transformation and exhibited a down-regulated expression pattern in human AAA tissues [Citation16]. However, as far as we know, there is still a lack of available information regarding the precise role of circulating miR-1-3p in the diagnosis of AAA.
In this case-control study, we analyzed the expression and clinical value of serum miR-1-3p in AAA patients, aiming to assess the effectiveness of circulating miR-1-3p in the identification of AAA. In addition, bioinformatics methods were used to explore the possible mechanisms of miR-1-3p in the development of AAA.
2. Materials and methods
2.1. Study subjects
Between August 2017 and April 2020, 200 patients diagnosed with AAA and a matched control group of 200 individuals were enrolled from the First Hospital of China Medical University. The determination of AAA was established utilizing computed tomography angiography (CTA), and AAA was defined as aortic enlargement with a diameter of 3.0 cm or larger [Citation17]. Inclusion criteria were as follows: patients were diagnosed as AAA through CTA; patients had not been treated with AAA therapy before this study; patients had complete clinical data, and the patients or their families signed informed consent form. This investigation excluded individuals with traumatic abdominal aortic pseudoaneurysm, Marfan syndrome, prior aortic surgeries, malignant neoplasms, infectious disorders, hematological diseases, autoimmune conditions, or significant organ failure. The controls were continuously recruited from subjects for routine check-ups with imaging examination to confirm the absence of AAA, and frequency-matched to AAA cases on the basis of gender and age (±5 years).
Fasting venous blood samples (5 mL each) were collected from each control subject undergoing a regular routine blood test, as well as from AAA patients before their surgeries. The serum was separated by centrifugation for 10 min at 3000 g within 30 min after blood collection, and subsequently preserved at −80 °C until required for analysis. Clinical information and demographic data were gathered from medical records of the subjects. The Ethics Committee of the First Hospital of China Medical University (Shenyang, China) reviewed and approved this study (Shenyang, China, Ethical Approval Number: [2020]146).
2.2. Image analyses
Individual morphometric AAA analyses were determined by CTA using GE AW4.7 software (GE HealthCare, USA). All measurements were completed by an experienced radiology expert to obtain image parameters including maximal diameter, area, length and volume of AAA and intraluminal thrombus (ILT). The maximal diameter and area were measured on cross-sections perpendicular to the aorta centerline. The length was performed parallel to the aortic centerline on curved planar reformat images. And the volume was generated by fully automatic calculation after automatic identification of the outline. In case of inaccurate recognition, the outline was manually adjusted by the radiology expert. The experienced radiology expert and another independent expert conducted repeated measurements of CTA images in twenty randomly selected patients to assess intra- and inter-observer variability using the intraclass correlation coefficient (ICC). An ICC value of >0.80 indicated excellent agreement. In our study, the mean ICC values for intra- and inter-observer variability in CTA image measurements were 0.95 and 0.92, respectively.
2.3. MiRNA isolation and quantification
The isolation of total RNA from serum was accomplished using Trizol reagent (Thermo Fisher Scientific, Waltham, USA) in accordance with the manufacturer’s instructions. Following isolation, the total RNA was subjected to reverse transcription to generate cDNA, utilizing the miRcute miRNA First-Strand cDNA Synthesis Kit (Tiangen, Beijing, China). To quantify the expression of miRNA, quantitative real-time polymerase chain reaction (qRT-PCR) was performed using the miRcute miRNA SYBR Green qPCR Detection Kit (Tiangen, Beijing, China). U6 was adopted as the internal control to determine the relative expression of miR-1-3p with the 2−ΔΔCT method. The primer sequences were as follows: U6, forward 5′-CTCGCTTCGGCAGCACA-3′ and reverse 5′-AACGCTTCACGAATTTGCGT-3′; miR-1-3p, forward 5′-TGGAATGTAAAGAAGTATGTAT-3′ and reverse 5′-CGCTTCACGAATTTGCGTG-3′. To ensure comparability of datasets that were generated between 2017 and 2020, the same internal control was run in every reverse transcription and qRT-PCR. Data on mRNA expression were presented in relation to this control (=1). Efficiency was checked for each pair of primers and was >90%.
2.4. Bioinformatics analyses
The miRTarBase database (version 9.0) (https://mirtarbase.cuhk.edu.cn/) [Citation18] and TargetScan database (version 8.0) (http://www.targetscan.org) [Citation19] were used to predict the putative targets of miR-1-3p. The overlapped mRNAs were selected as common target genes of miR-1-3p. To investigate the specific biological processes enriched by miR-1-3p target genes, Gene Ontology (GO) enrichment analysis was conducted. The visualization of predicted interaction network was realized with Cytoscape software (version 3.9.1) (https://cytoscape.org/).
2.5. Statistical analysis
The statistical analyses were performed utilizing the SPSS 23.0 software. The data were presented as mean ± standard deviation (SD) or number (percentage), and comparisons of continuous variables were conducted using either Student’s t-test or Mann-Whitney U test. The chi-square (χ2) test was applied to evaluate the differences in the categorical variables. Spearman correlation analysis was utilized to identify the relationship of serum miR-1-3p expression with AAA-related circulating biomarkers and image parameters. Receiver operating characteristics (ROC) curves were utilized to evaluate the diagnostic efficacy of serum miR-1-3p. Univariate and multivariate logistic regression analyses were conducted to assess the association between miR-1-3p expression and the risk of AAA, with odds ratios (ORs) and their corresponding 95% confidence intervals (95% CIs) calculated. Statistical significance was defined as a two-sided p-value < 0.05.
3. Results
3.1. Baseline characteristics of study subjects
The baseline characteristics and AAA image features are presented in . AAA patients and control subjects showed significant differences in terms of heart rate, dyslipidemia, white blood cell (WBC), hemoglobin (Hb), homocysteine (Hcy) and C-reactive protein (CRP) (all p < 0.05). Maximal aneurysm diameter, area, length and volume of AAA patients were 5.93 ± 1.66 cm, 27.09 ± 16.62 cm2, 12.15 ± 10.11 cm and 365.82 ± 409.50 ml, respectively.
Table 1. Clinical characteristics of AAA patients and control subjects.
3.2. MiR-1-3p expression in the human serum cohort
Overall, the levels of miR-1-3p were found to be significantly lower in the AAA group compared to the control group (0.82 ± 0.58 vs. 1.23 ± 1.00, p < 0.001) () (). Further stratified analysis showed that AAA patients displayed much lower levels of serum miR-1-3p than controls in age > 65 years, male or female, smoking or non-smoking, hypertension or non-hypertension, dyslipidemia or non-dyslipidemia, and non-diabetes subgroups ().
Table 2. Relative quantitative expression values of serum miR-1-3p between AAA patients and controls in the general and stratified comparisons.
3.3. Association of serum miR-1-3p with AAA-related biomarkers and image parameters
Correlation analyses indicated that serum miR-1-3p was negatively related to circulating WBC and CRP in total AAA patients and aneurysm >5.0 cm group (). Of note, we also observed a negative association of serum miR-1-3p expression with maximal aneurysm diameter, area and volume in AAA patients, while the association remained significant in either aneurysm > 5.0 cm group or 3.0 cm ≤ aneurysm ≤ 5.0 cm group (all p < 0.05) ().
Table 3. Relationship of serum miR-1-3p expression with circulating biomarkers and image parameters in AAA patients.
3.4. Diagnostic accuracy of serum miR-1-3p as a predictor for AAA
ROC analysis was used to assess the diagnostic efficacy of serum miR-1-3p (). Circulating miR-1-3p levels could significantly discriminate between AAA patients and healthy individuals with an area under the curve (AUC) of 0.672 (95% CI = 0.619-0.724, p < 0.001), a sensitivity of 84.5% and a specificity of 45.5%. Additionally, in the univariate analysis, a higher serum miR-1-3p level was found to be associated with decreased probability of AAA incidence (OR = 0.451 per unit increase, 95% CI = 0.315-0.645, p < 0.001), and this association remained statistically significant after adjusting for age, sex, smoking, heart rate, hypertension, diabetes, and dyslipidemia (OR = 0.440 per unit increase, 95% CI = 0.301-0.643, p < 0.001) (). Subsequently, using the optimal cut-off value of miR-1-3p levels determined by the ROC curve as a basis for discriminating AAA risk, the subjects were stratified into two groups: the low level group (≤0.62) and the high level group (>0.62). Regarding this classification, the low serum miR-1-3p levels were significantly associated with an increased risk of AAA in both univariate and multivariate logistic regression analyses, with ORs of 4.076 and 4.136, respectively (all p < 0.001) ().
Figure 2. Receiver Operator characteristic curve to assess the performance of serum miR-1-3p for AAA diagnosis.
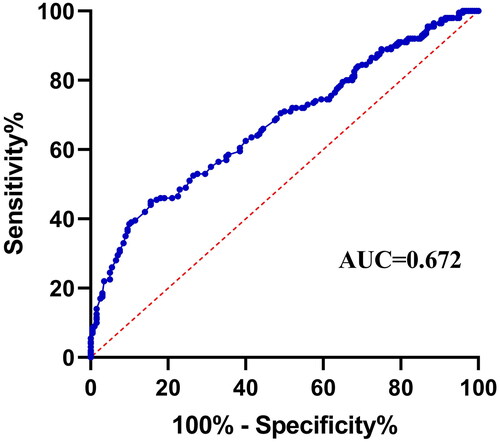
Table 4. Logistic regression analysis of the association between serum miR-1-3p expression and AAA risk.
3.5. Functional enrichment analysis
Subsequently, bioinformatics tools were utilized to identify the predicted target genes of miR-1-3p and then explore the potential biological function enriched characteristics associated with AAA pathogenesis. Based on GO enrichment analysis, miR-1-3p was identified to be mainly involved in negative regulation of apoptotic process, sprouting angiogenesis, angiogenesis, positive regulation of blood vessel endothelial cell migration, positive regulation of cell proliferation, regulation of cell shape and so on (). The miR-1-3p-related interaction network is constructed and presented in .
Figure 3. The hsa-miR-1-3p-mRNA-biological process interaction network. The network displays the association between hsa-miR-1-3p, predicted mRNAs and vital biological processes. Blue circle, mRNA. Green hexagon, hsa-miR-1-3p. Orange rectangle, biological process, the larger rectangle indicates a smaller P-value. Dash line, interaction between hsa-miR-1-3p and mRNAs. Solid line, interaction between mRNAs and biological processes.
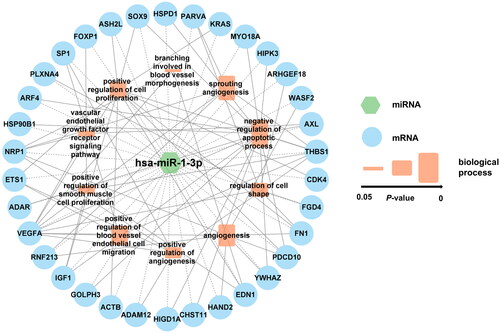
Table 5. Functional enrichment analysis of miR-1-3p target genes.
4. Discussion
Due to their stable character in circulating blood, miRNAs are recognized as potential diagnostic biomarkers. Recently, reports have been published regarding the association between circulating miRNAs and aneurysm [Citation20,Citation21]. Our prior investigation revealed that miR-1-3p might be implicated in the pathology of AAA by influencing VSMC phenotypic switching [Citation16]. In this study, for the first time, we revealed a significant down-regulation of serum miR-1-3p in AAA patients, and serum miR-1-3p could serve as a reliable circulating indicator for the recognition of AAA. Moreover, bioinformatics analysis indicated that miR-1-3p might participate in multiple biological processes to play a crucial role in the pathogenesis of AAA.
In the present study, it was firstly demonstrated that patients with AAA exhibited significantly lower levels of serum miR-1-3p compared to controls in both general and subgroup analyses. Hence, our findings suggested that serum miR-1-3p had the potential to function as a distinguishing biomarker between normal and AAA individuals. AAA is a consequence of sustained vascular remodeling and also strongly associated with both regional and systemic inflammatory responses. MiR-1-3p has been reported to play a role in regulating inflammatory responses in various biological processes and pathological conditions [Citation22,Citation23]. For instance, miR-1-3p regulates cell proliferation, apoptosis, and invasion by targeting chemokines and cytokines, and it has been regarded as a significant regulator of gene expression and cell contractility in VSMCs and plays a crucial role in pulmonary vascular remodeling [Citation24,Citation25]. Furthermore, according to an earlier investigation, LncRNA H19 could attenuate proliferation, promote apoptosis, and facilitate extracellular matrix (ECM) degradation of VSMC via miR-1-3p/ADAM10 axis in thoracic aortic aneurysm [Citation26]. In particular, our previous research has illuminated that the modulation of cell phenotypic transformation through the miR-1-3p/TLR4 axis can significantly influence the risk of AAA [Citation16]. Therefore, it is a plausible assumption that miR-1-3p may relevant to aneurysm development in the abdominal aorta as well.
In addition, we evaluated the correlation between serum miR-1-3p and various AAA-related biomarkers and image parameters. The correlation analyses revealed that serum miR-1-3p levels had a negative correlation with circulating WBC and CRP in patients with AAA. WBC and CRP are widely used in routine clinical practice as acute phase reactants to evaluate systemic inflammation, and several studies have demonstrated their close association with the development of AAA [Citation27,Citation28]. The observed correlation may indicate their potential interaction in response to aortic injury in the pathogenesis of AAA. Interestingly, we also observed a negative association of serum miR-1-3p expression with maximal aneurysm diameter, area and volume in either aneurysm > 5.0 cm group or 3.0 cm ≤ aneurysm ≤ 5.0 cm group. It is widely acknowledged that aortic size is a highly predictive indicator of aneurysm expansion, and serves as a clinical marker employed in the follow-up and management of AAA patients [Citation29,Citation30]. Our results indicated that regardless of the size of the aneurysm, serum miR-1-3p could change with it stably and synchronously, providing potential value of miR-1-3p as a biomarker for monitoring aortic dilation and predicting the progression of AAA, which could potentially aid in preventing the development of severe aortic complications.
Further, our study demonstrated that measuring serum miR-1-3p could serve as an independent risk factor for AAA diagnosis, and this may prove helpful in clinical decision-making and screening of patients with AAA. Based on ROC curve, reduced miR-1-3p expression showed a high degree of sensitivity in the discovery of AAA. In fact, several miRNAs have been identified as stable serum biomarkers with significant potential for clinical diagnosis, or for providing precise prognosis concerning patients with cardiovascular disorders [Citation31,Citation32]. Unlike conventional imaging methods, utilizing serum-based biomarkers for diagnosis requires minimal time and expense, and is available at bedside [Citation33]. After controlling for potential confounders, the results of multiple logistic regression analyses revealed a robust correlation between the expression of serum miR-1-3p and the presence of AAA. Participants with lower levels of miR-1-3p were found to have an approximately four-fold greater risk of developing AAA compared to those with higher levels. These findings suggest that serum miR-1-3p may have a significant clinical utility in predicting the onset of AAA, and thus represents a promising biomarker for further investigation. In future studies, with the development of detection technologies and the decreasing costs associated with such tests, the inclusion of miR-1-3p quantification may enhance risk stratification for individuals with AAA, presuming that its diagnostic efficacy could be confirmed in large patient cohorts.
The bioinformatics analysis indicated that miR-1-3p may potentially participate in the negative regulation of apoptotic process, sprouting angiogenesis, angiogenesis, positive regulation of blood vessel endothelial cell migration, positive regulation of cell proliferation, regulation of cell shape and so on. These prediction results are consistent with the features of increased VSMC apoptosis, dysfunction and phenotype changes, which leads to the destruction of aortic wall associated with AAA pathogenesis [Citation34,Citation35]. By potentially targeting genes involved in apoptosis regulation, miR-1-3p may play a critical role in modulating VSMC survival and function in AAA development. Furthermore, angiogenesis has been conclusively identified as a hallmark of AAA in both animal and human models [Citation36,Citation37]. Mural neovascularization is a prominent pathological characteristic of human AAA and has the potential to accelerate clinical progression [Citation38]. Our findings suggested that decreased expression of miR-1-3p might promote neovascularization, which was corroborated by observed low expression levels of miR-1-3p in AAA patients. Collectively, these data provided compelling evidence that miR-1-3p may play a crucial role in the formation of AAA by modulating processes such as VSMC apoptosis, neovascularization, and vascular remodeling. Further research into the origin and functional impact of miR-1-3p in AAA mechanisms could significantly enhance our comprehension of this complex disease. Understanding the specific targets and downstream effects of miR-1-3p may open up new avenues for therapeutic interventions and personalized treatment strategies for AAA patients. Our study sheds light on the potential role of miR-1-3p in AAA pathogenesis and highlights the importance of further investigation to unravel its precise molecular mechanism and functional impact on this multifaceted disease.
It should be acknowledged that the current study has several limitations. First, the sample size was relatively small, which may impede the generalizability and interpretation of results. Second, our experimental design did not permit for sequential determinations of miR-1-3p levels during aneurysm initiation and progression, precluding us from establishing causality. Third, although our bioinformatics analysis strongly implicated a functional association between miR-1-3p and AAA pathogenesis, further investigation was warranted to explore and confirm this complex association.
5. Conclusions
The current study identified a significant relationship between serum miR-1-3p levels and AAA risk. Compared to the control group, miR-1-3p levels were significantly down-regulated in both overall and stratified analyses. Serum miR-1-3p expression was negatively related to maximal aneurysm diameter, area and volume in AAA patients. Multivariate logistic analyses further suggested that miR-1-3p was a potential predictor of AAA risk, and circulating miR-1-3p levels demonstrated a significant discriminatory ability between AAA patients and healthy individuals. We also proposed that miR-1-3p may play a significant role in the pathogenesis of AAA by actively participating in several biological processes. However, further in-depth studies are required to firmly establish the molecular mechanism underlying our observations.
Author contributions
Jingjing Jing drafted the manuscript. Miao Chang performed the experiments. Shuyi Jiang interpreted the results. Tianlong Wang contributed to bioinformatics analysis. Qiuyan Sun and Jun Yang collected the clinical data. Chunyan Ma coordinated blood sample collection. Tan Li designed the research, analyzed the data and revised the manuscript. All authors have read and approved the final manuscript.
Competing interests
The authors declare no competing interests.
Ethics approval and consent to participate
Ethics approval was approved by the ethics committee of the First Hospital of China Medical University and written informed consent was obtained from each patient.
Acknowledgements
We thank all the patients and researchers for participating in this study.
Disclosure statement
No potential conflict of interest was reported by the author(s).
Data availability statement
The data that support the findings of this study are available on request from the corresponding author upon reasonable request.
Additional information
Funding
References
- Gao J, Cao H, Hu G, et al. The mechanism and therapy of aortic aneurysms. Signal Transduct Target Ther. 2023;8(1):1.
- Moll FL, Powell JT, Fraedrich G, et al. Management of abdominal aortic aneurysms clinical practice guidelines of the European society for vascular surgery. Eur J Vasc Endovasc Surg. 2011;41(Suppl 1):S1–9. doi:10.1016/j.ejvs.2010.09.011.
- Nordon IM, Hinchliffe RJ, Loftus IM, et al. Pathophysiology and epidemiology of abdominal aortic aneurysms. Nat Rev Cardiol. 2011;8(2):92–102. doi:10.1038/nrcardio.2010.180.
- Smidfelt K, Nordanstig J, Davidsson A, et al. Misdiagnosis of ruptured abdominal aortic aneurysms is common and is associated with increased mortality. J Vasc Surg. 2021;73(2):476–483 e473. doi:10.1016/j.jvs.2020.06.047.
- Memon AA, Zarrouk M, Agren-Witteschus S, et al. Identification of novel diagnostic and prognostic biomarkers for abdominal aortic aneurysm. Eur J Prev Cardiol. 2020;27(2):132–142. doi:10.1177/2047487319873062.
- Agarwal V, Bell GW, Nam JW, et al. Predicting effective microRNA target sites in mammalian mRNAs. Elife. 2015;4:e05005. doi:10.7554/eLife.05005.
- Rouleau S, Glouzon JS, Brumwell A, et al. 3’ UTR G-quadruplexes regulate miRNA binding. RNA. 2017;23(8):1172–1179. doi:10.1261/rna.060962.117.
- Mitchell PS, Parkin RK, Kroh EM, et al. Circulating microRNAs as stable blood-based markers for cancer detection. Proc Natl Acad Sci U S A. 2008;105(30):10513–10518. doi:10.1073/pnas.0804549105.
- Su X, Nie M, Zhang G, et al. MicroRNA in cardio-metabolic disorders. Clin Chim Acta. 2021;518:134–141. doi:10.1016/j.cca.2021.03.024.
- Kumar S, Boon RA, Maegdefessel L, et al. Role of noncoding RNAs in the pathogenesis of abdominal aortic aneurysm. Circ Res. 2019;124(4):619–630. doi:10.1161/CIRCRESAHA.118.312438.
- Zhou SS, Jin JP, Wang JQ, et al. miRNAS in cardiovascular diseases: potential biomarkers, therapeutic targets and challenges. Acta Pharmacol Sin. 2018;39(7):1073–1084. doi:10.1038/aps.2018.30.
- Tang Y, Fan W, Zou B, et al. TGF-beta signaling and microRNA cross-talk regulates abdominal aortic aneurysm progression. Clin Chim Acta. 2021;515:90–95. doi:10.1016/j.cca.2020.12.031.
- Li M, Chen X, Chen L, et al. MiR-1-3p that correlates with left ventricular function of HCM can serve as a potential target and differentiate HCM from DCM. J Transl Med. 2018;16(1):161. doi:10.1186/s12967-018-1534-3.
- Badacz R, Kleczyński P, Legutko J, et al. Expression of miR-1-3p, miR-16-5p and miR-122-5p as possible risk factors of secondary cardiovascular events. Biomedicines. 2021;9(8):1055. doi:10.3390/biomedicines9081055.
- Gao M, Yu T, Liu D, et al. Sepsis plasma-derived exosomal miR-1-3p induces endothelial cell dysfunction by targeting SERP1. Clin Sci (Lond). 2021;135(2):347–365. doi:10.1042/CS20200573.
- Li T, Jing J, Sun L, et al. The SNP rs4591246 in pri-miR-1-3p is associated with abdominal aortic aneurysm risk by regulating cell phenotypic transformation via the miR-1-3p/TLR4 axis. Int Immunopharmacol. 2023;118:110016. doi:10.1016/j.intimp.2023.110016.
- Owens DK, Davidson KW, Krist AH, et al. Screening for abdominal aortic aneurysm: US preventive services task force recommendation statement. JAMA. 2019;322(22):2211–2218. doi:10.1001/jama.2019.18928.
- Huang HY, Lin YC, Cui S, et al. miRTarBase update 2022: an informative resource for experimentally validated miRNA-target interactions. Nucleic Acids Res. 2022;50(D1):D222–D230. doi:10.1093/nar/gkab1079.
- McGeary SE, Lin KS, Shi CY, et al. The biochemical basis of microRNA targeting efficacy. Science. 2019;366(6472):eaav1741. doi:10.1126/science.aav1741.
- Boileau A, Lino Cardenas CL, Courtois A, et al. MiR-574-5p: a circulating marker of thoracic aortic aneurysm. Int J Mol Sci. 2019;20(16):3924.
- Thanigaimani S, Iyer V, Bingley J, et al. Association between serum MicroRNAs and abdominal aortic aneurysm diagnosis and growth. Eur J Vasc Endovasc Surg. 2023;65(4):573–581. doi:10.1016/j.ejvs.2022.12.028.
- Ginckels P, Holvoet P. Oxidative stress and inflammation in cardiovascular diseases and cancer: role of non-coding RNAs. Yale J Biol Med. 2022;95(1):129–152.
- Sun TY, Li YQ, Zhao FQ, et al. MiR-1-3p and MiR-124-3p synergistically damage the intestinal barrier in the ageing Colon. J Crohns Colitis. 2022;16(4):656–667. doi:10.1093/ecco-jcc/jjab179.
- Jiang Y, Yin H, Zheng XL. MicroRNA-1 inhibits myocardin-induced contractility of human vascular smooth muscle cells. J Cell Physiol. 2010;225(2):506–511. doi:10.1002/jcp.22230.
- Sysol JR, Chen J, Singla S, et al. Micro-RNA-1 is decreased by hypoxia and contributes to the development of pulmonary vascular remodeling via regulation of sphingosine kinase 1. Am J Physiol Lung Cell Mol Physiol. 2018;314(3):L461–L472. doi:10.1152/ajplung.00057.2017.
- Fan Z, Liu S, Zhou H. LncRNA H19 regulates proliferation, apoptosis and ECM degradation of aortic smooth muscle cells via miR-1-3p/ADAM10 axis in thoracic aortic aneurysm. Biochem Genet. 2022;60(2):790–806. doi:10.1007/s10528-021-10118-y.
- De Haro J, Bleda S, Acin F. C-reactive protein predicts aortic aneurysmal disease progression after endovascular repair. Int J Cardiol. 2016;202:701–706. doi:10.1016/j.ijcard.2015.09.122.
- Folsom AR, Yao L, Alonso A, et al. Circulating biomarkers and abdominal aortic aneurysm incidence: the atherosclerosis risk in communities (ARIC) study. Circulation. 2015;132(7):578–585. doi:10.1161/CIRCULATIONAHA.115.016537.
- Wanhainen A, Mani K, Golledge J. Surrogate markers of abdominal aortic aneurysm progression. Arterioscler Thromb Vasc Biol. 2016;36(2):236–244. doi:10.1161/ATVBAHA.115.306538.
- Yavarimanesh M, Cheng HM, Chen CH, et al. Abdominal aortic aneurysm monitoring via arterial waveform analysis: towards a convenient point-of-care device. NPJ Digit Med. 2022;5(1):168. doi:10.1038/s41746-022-00717-3.
- Kablak-Ziembicka A, Badacz R, Przewlocki T. Clinical application of serum microRNAs in atherosclerotic coronary artery disease. J Clin Med. 2022;11(22):6849.
- Parizadeh SM, Ferns GA, Ghandehari M, et al. The diagnostic and prognostic value of circulating microRNAs in coronary artery disease: a novel approach to disease diagnosis of stable CAD and acute coronary syndrome. J Cell Physiol. 2018;233(9):6418–6424. doi:10.1002/jcp.26324.
- Peyronnel C, Totoson P, Martin H, et al. Relevance of circulating markers of endothelial activation for cardiovascular risk assessment in rheumatoid arthritis: a narrative review. Life Sci. 2023;314:121264. doi:10.1016/j.lfs.2022.121264.
- Mao N, Gu T, Shi E, et al. Phenotypic switching of vascular smooth muscle cells in animal model of rat thoracic aortic aneurysm. Interact Cardiovasc Thorac Surg. 2015;21(1):62–70. doi:10.1093/icvts/ivv074.
- Quintana RA, Taylor WR. Cellular mechanisms of aortic aneurysm formation. Circ Res. 2019;124(4):607–618. doi:10.1161/CIRCRESAHA.118.313187.
- Trollope AF, Golledge J. Angiopoietins, abdominal aortic aneurysm and atherosclerosis. Atherosclerosis. 2011;214(2):237–243. doi:10.1016/j.atherosclerosis.2010.08.051.
- Zhang M, Sui W, Cheng C, et al. Erythropoietin promotes abdominal aortic aneurysms in mice through angiogenesis and inflammatory infiltration. Sci Transl Med. 2021;13(603):eaaz4959. doi:10.1126/scitranslmed.aaz4959.
- Tedesco MM, Terashima M, Blankenberg FG, et al. Analysis of in situ and ex vivo vascular endothelial growth factor receptor expression during experimental aortic aneurysm progression. Arterioscler Thromb Vasc Biol. 2009;29(10):1452–1457. doi:10.1161/ATVBAHA.109.187757.