Abstract
Background:
Systemic immune-inflammatory markers such as neutrophil-to-lymphocyte ratio (NLR), platelet-to-lymphocyte ratio (PLR), lymphocyte-to-monocyte ratio (LMR) and systemic immune-inflammatory index (SII) are associated with the prognosis of many cardiovascular and neoplastic diseases. Moyamoya disease (MMD) is associated with inflammation, but the relationship between systemic immune-inflammatory markers between MMD is unclear. The aim of our study was to analyse the association between systemic immune-inflammatory markers and the risk of MMD and its subtypes.
Methods:
We consecutively recruited 360 patients with MMD and 89 healthy control subjects in a case-control study to calculate and analyse the association of systemic immune-inflammatory markers with the risk of MMD and its subtypes.
Results:
The risk of MMD increased with higher levels of NLR (OR 1.237, 95% CI [1.008, 1.520], p = .042). When NLR and SII were assessed as quartile-spaced subgroups, the third quartile grouping of NLR and SII had a higher risk of MMD than the first quartile grouping (NLR: OR 3.206, 95% CI [1.271, 8.088], p = .014; SII: OR 3.074,95% CI [1.232,7.672], p = .016). When NLR was combined with SII, the highest subgroup had a higher risk of MMD than the lowest subgroup (OR2.643, 95% CI [1.340, 5.212], p = .005). The risk of subtypes also increased with higher levels of NLR and SII. The association between the levels of NLR and SII with the staging of the Suzuki stage follows an inverted U-shape. The highest levels of NLR and SII were found in patients with MMD at Suzuki stages 3–4.
Conclusion:
The risk of MMD increases with elevated systemic immune-inflammatory markers. This study analysed the association of systemic immune-inflammatory markers with the risk of developing MMD and its subtypes, and identified novel inflammatory markers for MMD.
Key Messages
Systemic immune-inflammatory markers such as neutrophil–lymphocyte ratio and systemic immune-inflammatory index were higher in moyamoya disease (MMD) patients than in normal people.
Systemic immune-inflammatory markers may be an independent risk factor for the onset of MMD.
Systemic immune-inflammatory markers were associated with the progression of MMD, and their levels showed an inverted U shape with imaging stages.
Introduction
Moyamoya disease (MMD) is a rare disease characterized by progressive bilateral terminal occlusion of the carotid arteries with collateral vascularization at the base of the brain [Citation1]. The RNF213 variant has been identified as a susceptibility gene for MMD, and studies in the Japanese population have shown that the p.4810k mutation in RNF213 accounts for 73% of non-familial MMD [Citation2], but this percentage is far less in China. Moyamoya disease is therefore considered to be a multifactorial disease, caused by a combination of genetic, immune, inflammatory and other factors. Numerous studies have proven that the systemic chronic inflammatory response plays a crucial role in the pathophysiology of MMD [Citation3].
The neutrophil-to-lymphocyte ratio (NLR), platelet-to-lymphocyte ratio (PLR), systemic immune-inflammatory index (SII) and lymphocyte-to-monocyte ratio (LMR) are readily available as a class of inexpensive inflammatory indicators that provide a better picture of the degree of systemic inflammation than neutrophil (NEUT) or lymphocyte (LY) counts alone. Recently, the NLR has emerged as a strong predictor of poor prognosis in patients with cardiovascular disease [Citation4]. And PLR, LMR and SII have recently been frequently used to predict patient prognosis in cancers such as breast [Citation5,Citation6]. As a disease closely associated with systemic inflammation, no studies have systematically described the association between these systemic inflammatory indices and MMD.
Our study recruited patients with MMD and normal healthy controls and calculated their systemic inflammatory indices. We analysed the correlation of the systemic immune-inflammatory markers with MMD and subgroups. This study will help identify new potential biomarkers for managing MMD.
Methods
Study design and participants
Patients with MMD who attended Tiantan Hospital from September 2020 to December 2021 were recruited for our study. Patients diagnosed with MMD by digital subtraction angiography (DSA) according to the Japanese guidelines for the management of MMD were included in the study and excluded patients with serious infection events within 1 month. Paediatric patients and elderly patients older than 60 years were also excluded. Finally, 360 patients with MMD were included in our study, 259 with ischemic MMD and 101 with haemorrhagic MMD. We then recruited 89 age-sex-matched healthy controls who underwent a routine health check-up. Healthy controls had few comorbidities. Enrolled subjects were screened according to the flowchart in Supplementary Figure 1. We collected baseline demographic data on all enrolled subjects, including age, sex, heart rate, BMI and previous medical history including diabetes, hypertension, hyperlipidaemia, smoking and alcohol consumption. All baseline data collection was done by a dedicated researcher through questionnaires to ensure data credibility.
Baseline data collection
Pre-operative blood samples were collected from all patients with MMD using a rapid clinical analyser for white blood cell (WBC) count, LY count, NEUT count, monocyte (MONO) count, platelet (PLT) count, alanine aminotransferase (ALT), aspartate aminotransferase (AST), glucose (GLU), urea, uric acid (UA), triglycerides (TGs), total cholesterol, high-density lipoprotein (HDL), low-density lipoprotein (LDL), homocysteine. Systemic immune-inflammatory markers were calculated, including NLR, PLR, LMR and SII. RNF213 p.R4810K variant was detected. The primers were designed as follows: RNF213-4810F (rs112735431): 5′-GCCCTCCATTTCTAGCACAC-3′; and RNF213-4810R: 5′-AGCTGTGGCGAAAGCTTCTA-3′. Bilateral angiographic Suzuki staging and the p.R4810K mutation of RNF213 of patients with MMD were collected for within-group comparison.
Statistical analysis
All statistical analyses were performed using SPSS version 25.0 (IBM Corporation, Armonk, NY) and EMPOWER(R) (www.empower stats.com, X&Y Solutions, Inc., Boston, MA). The baseline differences were compared between the MMD group and healthy controls. Continuous variables are expressed as mean with standard deviation (SD) or median with interquartile range (IQR) and categorical variables are expressed as frequencies. NLR and SII are evaluated as continuous variables based on quartiles as follows: NLR: quartile 1 (Q1): ≤1.642; quartile 2 (Q2): 1.642–2.059; quartile 3 (Q3): 2.059–2.784; quartile 4 (Q4): >2.784; SII: Q1: ≤373.893; Q2: 373.893–505.348; Q3: 505.348–750.169; Q4: >750.169. The combination markers were used to classify subjects into four groups based on the median of NLR and SII. Group 1: NLR ≤2.784 and SII ≤373.893; group 2: NLR ≤2.784 and SII >373.893; group 3: NLR >2.784 and SII ≤373.893; group 4: NLR >2.784 and SII >373.893.
Differences between continuous variables were compared using two-tailed Student’s t-tests or Mann–Whitney’s U-tests and differences between categorical variables were compared using χ2 test or Fisher’s exact test. The whole-body inflammatory index was compared as a continuous variable and then grouped at interquartile spacing and differences were compared using χ2 tests. Logistic regression models were performed to identify independent factors for MMD and its subtypes. The crude model was an unadjusted regression model for the systemic inflammatory index. Model 1 was adjusted for covariates including age, gender and clinical tests. Model 2 was further adjusted for BMI and previous risk factors. In addition, we assessed the model’s predictive performance for the risk of MMD and its subtypes by creating receiver operating characteristic (ROC) curves and calculating the area under the curve (AUC).
Results
Baseline characteristics of participants
Our study ultimately included 449 subjects, including 360 patients with MMD and 89 normal subjects. Of these, 192 (40.5%) were male and 282 (59.5%) were female. There were 259 ischemic presentations and 101 haemorrhagic presentations in the patients with MMD. In the group of MMD and its subgroups, WBC count, LY count, NEUT count, glutamate alanine transaminase, GLU, TGs, cholesterol, homocysteine, BMI, NLR, SII and PLR levels were higher than in the HC group and the prevalence of hypertension, diabetes mellitus, hyperlipidaemia, smoking and alcohol consumption was higher. The baseline characteristics of the participants are shown in . The differences in NLR, SII and PLR between the various subgroups of MMD and healthy controls are shown in .
Figure 1. Comparison of the differences in the levels of systemic inflammatory indices between the subtypes of MMD and normal subjects. ns: not significant; *p < .05; **p < .01.
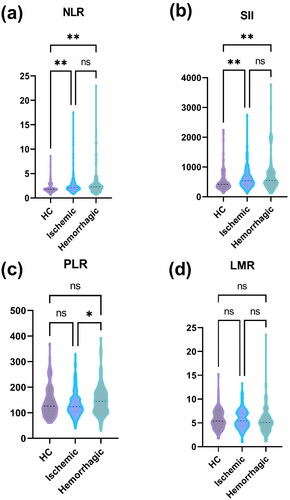
Table 1. Baseline characteristics of MMD and normal subjects.
In addition, patients with MMD were grouped according to NLR quartile spacing, SII quartile spacing and combination markers. Overall, patients with higher NLR and SII levels had a higher prevalence of hypertension and diabetes. The baseline characteristics of subjects grouped according to the IQR of NLR and SII are shown in Supplementary Tables 1 and 2. Baseline characteristics of subjects grouped by combined markers are shown in Supplementary Table 3.
Association of NLR and SII with the risk of MMD and its subtypes
Continuous variables and quartile grouping for NLR and SII were included in the logistic regression model and the overall risk of MMD remained elevated with higher levels of the NLR continuous variable after adjustment for model 2 (OR 1.237, 95% CI [1.008, 1.520], p = .042). The level of the SII continuous variable was not associated with the overall risk of MMD. The risk of MMD was higher in Q3 than in Q1 in the quartile-spaced subgroups of the NLR and SII (NLR: OR 3.206, 95% CI [1.271, 8.088], p = .014; SII: OR 3.074, 95% CI [1.232, 7.672], p = .016). In the subgroup analysis of MMD, the NLR continuous variable was a risk factor for the haemorrhagic type in model 2 (OR 1.435, 95% CI [1.110, 1.854], p = .006). In the NLR quartile-spaced subgroups, the risk of ischemic type was higher in Q3 than in Q1 (OR 2.919, 95% CI [1.008, 8.450], p = .048) and the risk of haemorrhagic type was higher in Q3 and Q4 than in Q1 (OR 5.503, 95% CI [1.718, 17.632], p = .004; OR 2.844, 95% CI [1.000, 8.091], p = .05). In the SII quartile-spaced subgroups, the risk of ischemic type was higher in Q3 than in Q1 (OR 4.033, 95% CI [1.442, 11.284], p = .008) and the risk of haemorrhagic type was higher in Q4 than in Q1 (OR 3.789, 95% CI [1.296, 11.078], p = .044). The details are shown in and . A risk forest plot of NLR and SII versus MMD and its subtypes is shown in .
Figure 2. Risk association of NLR and SII with MMD and its subtypes. Risk forest plots and ROC curves of NLR with MMD overall (a), ischemic type (b) and haemorrhagic type (c). Risk forest plots of SII with MMD overall (d), ischemic type (e) and haemorrhagic type (f).
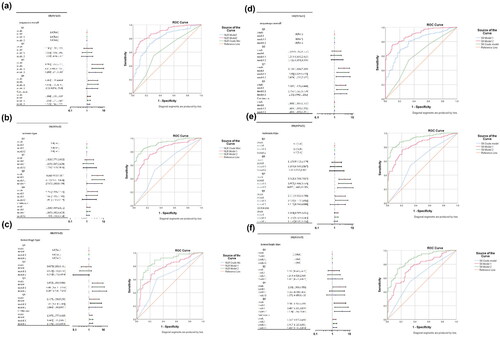
Table 2. Association of NLR with the risk of MMD and its subtypes.
Table 3. Association of SII with the risk of MMD and its subtypes.
The grouping of the combination markers was more consistent with the risk of MMD and its subtypes. After adjustment for model 2, the combination markers had a higher risk of both MMD and ischemia, and haemorrhage in G4 than in G1 (MMD: OR 2.643, 95% CI [1.340, 5.212], p = .005; ischemic type: OR 2.204, 95% CI [1.020, 4.763], p = .044; haemorrhagic type: OR 5.271, 95% CI [2.011, 13.817], p = .001). The details are shown in Supplementary Table 4. A risk forest plot of combination markers versus MMD and its subtypes is shown in Supplementary Figure 2. In the ROC curves, model 2 predicted the risk of MMD and both ischemic and haemorrhagic subtypes better than the original model and model 1.
Improvement of risk prediction models for MMD and its subtypes by systemic immune-inflammatory markers
With the addition of NLR, SII and combination markers, we compared the improvement of the risk prediction model for the prediction of MMD and its subtypes. After adjustment of model 2, the prediction of the haemorrhagic subtype of MMD remained improved by adding NLR. After adding NLR, the NRI was 7.8 (95% CI [0.5, 15.1], p = .036) and the IDI was 7.8 (95% CI [0.5, 15.1], p = .037) for the haemorrhagic subtype of MMD. The addition of combination markers also showed a significant improvement in the prediction of the haemorrhagic subtype of MMD. The NRI for the haemorrhagic subtype of MMD was 11.2 (95% CI [0.6, 21.7], p = .038) and the IDI was 11.2 (95% CI [0.5, 21.8], p = .039) after the addition of the combination markers. The details are shown in .
Table 4. Improvement of the prediction effect of adding systemic inflammation index for the prediction model of MMD and its subtype risk.
NLR and SII correlate with the imaging classification of MMD
We compared all patients with MMD individually using the hemispheres as the basic unit and investigated the relationship between imaging manifestations and systemic inflammatory factors. The 720 hemispheres of 360 patients with MMD were classified into three grades according to Suzuki angiographic staging: Suzuki staging at 0–2 as grade 1, Suzuki staging at 3–4 as grade 2 and Suzuki staging at 5–6 as grade 3. Baseline characteristics of patients with MMD grouped by imaging grade are shown in Supplementary Table 5. The results suggest that the trend of the relationship between the severity of MMD imaging and NLR follows an inverted U-shape. The highest levels of NLR and SII were found in patients with MMD at Suzuki stages 3–4, while lower levels of NLR and SII were found at Suzuki stages 0–2 and 5–6. The relationship between NLR and SII and imaging grade is shown in .
Discussion
Our study investigated 449 subjects and found that elevated NLR and SII were associated with an increased risk of developing MMD, which is an independent risk factor for MMD. Moreover, NLR and SII contribute to the reclassification and differentiation of the risk of ischemic and haemorrhagic subtypes of MMD. The association between the levels of NLR and SII with the staging of the Suzuki stage follows an inverted U-shape.
MMD is an important cause of stroke in young and middle-aged patients in Southeast Asia. The detailed mechanisms underlying the onset and progression of MMD remain unknown, and its etiology is currently thought to be usually integrative, involving genetics, immunity and angiogenesis [Citation7]. Infectious factors are often an easily overlooked cause of MMD. Many studies have previously reported cases of meningitis in combination with MMD to cases of MMD in combination with varicella zoster [Citation8,Citation9], suggesting a close relationship between inflammation and MMD. The possible mechanism by which inflammation triggers MMD is by promoting endothelial vascular smooth muscle cell proliferation and endothelial cell proliferation, causing vascular proliferation, which leads to vascular stenosis and collateral vessel formation [Citation10,Citation11].
The NLR is an inexpensive indicator of systemic inflammation that characterizes inflammation more consistently than NEUT count and LY count and is less susceptible to dehydration and fluid overload [Citation12,Citation13]. In one study, NLR was found to be associated with enhanced imaging of the intracranial aneurysm wall, indicating the presence of a local inflammatory response in the inner wall. Therefore, it is now considered that NLR is a pro-inflammatory indicator, and its elevation represents an increased level of systemic inflammation. A study on tumours showed that elevated NLR levels were accompanied by elevated levels of certain angiogenic factors [Citation14] and matrix metalloproteinases (MMPs) [Citation15]. VEGF and MMP-9 have been reported to be elevated in MMD. VEGF may play a role in the recruitment of vascular progenitors and in the formation of collateral vessels [Citation16]. MMP-9 breaks down intercellular tight junction proteins, leading to the degradation of the vascular basement membrane and remodelling of the extracellular matrix, thereby promoting endothelial cell migration. MMP-9 contributes to the role of VEGF in MMD in promoting intimal thickening and extensive collateral angiogenesis [Citation17]. In addition, it has recently been shown that NEUT extracellular traps produced by NEUT lysis activate local pro-inflammatory and pro-angiogenic responses in pulmonary vascular endothelial cells via ROS-induced TLR4-mediated signalling pathways, with a potential pathogenic role in pulmonary hypertension [Citation18]. The pathology of pulmonary hypertension and MMD is similar, both manifesting as vascular proliferation and narrowing. NLR, as an inflammatory indicator closely associated with NEUTs, may play a role in the pathogenesis of MMD by promoting angiogenesis through the above two pathway.
The SII has a more comprehensive role in the evaluation of systemic inflammation levels because it incorporates PLT counts. Platelets are also capable of secreting pro-inflammatory factors that contribute to the development of MMD through the above pathways. Platelets also secrete chemokines [Citation19,Citation20], and elevated PLTs cause a state of systemic hypercoagulation, leading to intravascular micro thrombosis. Hypercoagulation is another easily overlooked cause of MMD. Of 22 autopsy findings in patients with MMD with bilateral internal carotid arteries, a thrombus was present in 16 cases [Citation21]. This suggests that thrombosis is closely associated with the development of intracranial artery intimal thickening [Citation22]. In addition, PLTs also secrete PLT-derived growth factor (PDGF), which also promotes collateral angiogenesis. It has been shown that PDGF stimulates vascular smooth muscle cells of MMD origin to accelerate DNA synthesis and promote proliferation [Citation10]. Moreover, PLTs act as carriers of peripheral 5-hydroxytryptamine and can secrete 5-hydroxytryptamine to act on the vascular endothelium when activated [Citation23]. 5-Hydroxytryptamine stimulates ROS via Nox, down-regulates protective antioxidant mechanisms and promotes extracellular matrix remodelling [Citation24] and proliferation of vascular endothelial cells and smooth muscle cells. This process eventually triggers constriction and narrowing of the blood vessels [Citation25]. Therefore, PLTs are closely associated with vascular remodelling in MMD.
Interestingly, systemic immune-inflammatory markers were highest in patients with Suzuki stages 3–4 and did not simply rise with Suzuki stage. We assume that systemic inflammation promotes smoky vascular proliferation, so the period with the highest level of systemic inflammation should be the most significant period of smoky vessels. It is known that smoky vessels in MMD are most luxuriant at Suzuki stages 3–4. The results we obtained are fully consistent with the progressive presentation of MMD, and this result suggests a correlation between systemic immune-inflammatory markers and smoky vessels. Previous studies have reported that the prognosis after indirect bypass surgery for MMD correlates with smoky vessels because of the relatively high angiogenic capacity at this time [Citation26]. It means the angiogenic capacity was elevated at the stage of higher systemic inflammation levels. This further validates our hypothesis that systemic inflammation plays a role in MMD through pro-angiogenesis. In addition, it suggests a possible association between systemic inflammation and prognosis. Further clinical studies are needed to analyse the relationship between systemic inflammation and the prognosis of MMD.
There are several limitations of this study. First, this study is a single-centre, small-sample study with inevitable selection bias. Second, NLR and SII, which are systemic immune-inflammatory markers, are influenced by too many complex factors to avoid interference, although patients with infectious diseases were excluded from the study. Third, the study could only demonstrate the association of systemic inflammatory markers with the development of MMD. Due to the lack of animal models of MMD, the underlying mechanisms by which inflammatory markers contribute to MMD remain unclear. Further experiments are needed to confirm the pathways of inflammatory indicators in MMD.
Conclusions
Our study concluded that systemic immune inflammatory markers such as NLR and SII were associated with the risk of MMD, and the relationship with the Suzuki stage of MMD was inverted U-shaped.
Author contributions
Dong Zhang and Peicong Ge conceived the idea; Wei Liu conducted the analyses and wrote the paper; Chenglong Liu, Xiaofan Yu, Yuanren Zhai, Qiheng He and Junsheng Li provided the data; all authors contributed to the revisions.
Ethical approval
The study was conducted in accordance with the Declaration of Helsinki, and approved by the Ethics Committee of Beijing Tiantan Hospital, Capital Medical University (protocol code KY2022-051-02 and date of 2022/06/15).
Patient consent
Informed consent was obtained from all subjects involved in the study. Written informed consent has been obtained from the patients to publish this paper.
Supplemental Material
Download Zip (263.3 KB)Acknowledgments
We acknowledge all individuals for their participation in this study.
Disclosure statement
The authors declare no conflict of interest.
Data availability statement
The raw data supporting the conclusions of this article will be made available by the authors, without undue reservation.
Additional information
Funding
References
- Scott RM, Smith ER. Moyamoya disease and moyamoya syndrome. N Engl J Med. 2009;360(12):1–9. doi:10.1056/NEJMra0804622.
- Kamada F, Aoki Y, Narisawa A, et al. A genome-wide association study identifies RNF213 as the first moyamoya disease gene. J Hum Genet. 2011;56(1):34–40. doi:10.1038/jhg.2010.132.
- Mikami T, Suzuki H, Komatsu K, et al. Influence of inflammatory disease on the pathophysiology of moyamoya disease and quasi-moyamoya disease. Neurol Med Chir. 2019;59(10):361–370. doi:10.2176/nmc.ra.2019-0059.
- Ferro D, Matias M, Neto J, et al. Neutrophil-to-lymphocyte ratio predicts cerebral edema and clinical worsening early after reperfusion therapy in stroke. Stroke. 2021;52(3):859–867.
- Zhu Y, Zhou S, Liu Y, et al. Prognostic value of systemic inflammatory markers in ovarian cancer: a PRISMA-compliant meta-analysis and systematic review. BMC Cancer. 2018;18(1):443.
- Graziano V, Grassadonia A, Iezzi L, et al. Combination of peripheral neutrophil-to-lymphocyte ratio and platelet-to-lymphocyte ratio is predictive of pathological complete response after neoadjuvant chemotherapy in breast cancer patients. Breast. 2019;44:33–38.
- Shang S, Zhou D, Ya J, et al. Progress in moyamoya disease. Neurosurg Rev. 2020;43(2):371–382. doi: 10.1007/s10143-018-0994-5.
- Nagel MA, Niemeyer CS, Bubak AN. Central nervous system infections produced by varicella zoster virus. Curr Opin Infect Dis. 2020;33:272–278.
- Maramattom BV. Postcryptococcal moyamoya syndrome: case report and review of literature. Neurol India. 2020;68(3):660–664.
- Aoyagi M, Fukai N, Sakamoto H, et al. Altered cellular responses to serum mitogens, including platelet-derived growth factor, in cultured smooth muscle cells derived from arteries of patients with moyamoya disease. J Cell Physiol. 1991;147(2):191–198.
- Yoshimoto T, Houkin K, Takahashi A, et al. Angiogenic factors in moyamoya disease. Stroke. 1996;27:2160–2165.
- Azab B, Zaher M, Weiserbs KF, et al. Usefulness of neutrophil to lymphocyte ratio in predicting short- and long-term mortality after non-ST-elevation myocardial infarction. Am J Cardiol. 2010;106:470–476.
- Tekesin A, Tunç AA-O. Inflammatory markers are beneficial in the early stages of cerebral venous thrombosis. Arq Neuropsiquiatr. 2019;77(2):101–105.
- Hoshimaru M, Takahashi JA, Kikuchi H, et al. Possible roles of basic fibroblast growth factor in the pathogenesis of moyamoya disease: an immunohistochemical study. J Neurosurg. 1991;75:267–270.
- Schaider H, Oka M, Bogenrieder T, et al. Differential response of primary and metastatic melanomas to neutrophils attracted by IL-8. Int J Cancer. 2003;103(3):335–343.
- Kang H-S, Kim JH, Phi JH, et al. Plasma matrix metalloproteinases, cytokines and angiogenic factors in moyamoya disease. J Neurol Neurosurg Psychiatry. 2010;81(6):673–678. doi:10.1136/jnnp.2009.191817.
- Shamamian P, Schwartz JD, Pocock BJ, et al. Activation of progelatinase A (MMP-2) by neutrophil elastase, cathepsin G, and proteinase-3: a role for inflammatory cells in tumor invasion and angiogenesis. J Cell Physiol. 2001;189(2):197–206.
- Aldabbous L, Abdul-Salam V, McKinnon T, et al. Neutrophil extracellular traps promote angiogenesis: evidence from vascular pathology in pulmonary hypertension. Arterioscler Thromb Vasc Biol. 2016;36(10):2078–2087. doi:10.1161/ATVBAHA.116.307634.
- Kyaw TA-O, Peter K, Li Y, et al. Cytotoxic lymphocytes and atherosclerosis: significance, mechanisms and therapeutic challenges. Br J Pharmacol. 2017;174(22):3956–3972.
- Mulholland M, Jakobsson G, Lei Y, et al. IL-2Rβγ signalling in lymphocytes promotes systemic inflammation and reduces plasma cholesterol in atherosclerotic mice. Atherosclerosis. 2021;326:1–10.
- Weinberg DG, Arnaout OM, Rahme RJ, et al. Moyamoya disease: a review of histopathology, biochemistry, and genetics. Neurosurg Focus. 2011;30(6):E20.
- Yamashita M, Oka K, Tanaka K. Cervico-cephalic arterial thrombi and thromboemboli in moyamoya disease – possible correlation with progressive intimal thickening in the intracranial major arteries. Stroke. 1984;15(2):264–270. doi:10.1161/01.str.15.2.264.
- MacLean MR, Dempsie Y. Serotonin and pulmonary hypertension—from bench to bedside? Curr Opin Pharmacol. 2009;9:281–286.
- Hood KY, Mair KM, Harvey AP, et al. Serotonin signaling through the 5-HT1B receptor and NADPH oxidase 1 in pulmonary arterial hypertension. Arterioscler Thromb Vasc Biol. 2017;37(7):1361–1370.
- Hervé P, Launay JM, Scrobohaci ML, et al. Increased plasma serotonin in primary pulmonary hypertension. Am J Med. 1995;99(3):249–254.
- Zhao Y, Li J, Lu J, et al. Predictors of neoangiogenesis after indirect revascularization in moyamoya disease: a multicenter retrospective study. J Neurosurg. 2019;1–11. doi:10.3171/2018.9.JNS181562.