Abstract
Background
Delayed diagnosis and inadequate treatment caused by limited biomarkers are associated with the outcomes of COVID-19 patients. It is necessary to identify other promising biomarkers and candidate targets for defining dysregulated inflammatory states.
Methods
The triggering receptors expressed on myeloid cell (TREM)-1 and TREM-2 expression from hospitalized COVID-19 patients were characterized using ELISA and flow cytometry, respectively. Their correlation with disease severity and contrast with the main clinical indicators were evaluated.
Results
Increased expression of soluble TREM-1 and TREM-2 in the plasma of COVID-19 patients was found compared to the control group. Moreover, membrane-bound TREM-1 and TREM-2 expression was upregulated on the cell surface of circulating blood T cells from COVID-19 patients. Correlation analysis showed that sTREM-2 levels were negatively correlated with PaO2/FiO2, but positively correlated with C-reactive protein (CRP), procalcitonin (PCT) and interleukin (IL)-6 levels. Receiver operating characteristic curve analysis indicated that the predictive efficacy of sTREM-1 and sTREM-2 was equivalent to CRP and IL-6, and a little better than absolute leukocyte or neutrophil count and PCT in distinguishing disease severity.
Conclusion
TREM-2 and TREM-1 are critical host immune factors that response to SARS-COV-2 infection and could serve as potential diagnostic biomarkers and therapeutic targets for COVID-19.
KEY MESSAGES
The expression of soluble TREM-1 and TREM-2 in plasma and membrane-bound TREM-1 and TREM-2 on the cell surface was upregulated in COVID-19 patients.
sTREM-2 level was negatively correlated with PaO2/FiO2, but positively correlated with CRP, PCT and IL-6 level, respectively.
sTREM-1 and sTREM-2 exhibited potential predictive abilities, and their expression was equivalent to CRP and IL-6, and better than the absolute leukocytes or neutrophil counts and PCT in distinguishing disease severity.
Keywords:
Introduction
Severe acute respiratory syndrome coronavirus-2 (SARS-CoV-2), which causes coronavirus disease 2019 (COVID-19), is still a health problem worldwide [Citation1–3]. Severe COVID-19 is far more than a serious febrile respiratory illness, and growing evidence suggests that it is a complex multisystem disease that usually involves damage to other vital organs (e.g. lungs, heart, kidneys) [Citation4,Citation5]. Although several vaccines have been developed to diminish the effects of SARS-CoV-2 infection, the elderly and those with defective or suppressed immune systems are still at particularly high risk [Citation6]. At least 10–20% of patients experience a range of medium- and long-term effects after recovering from the acute stage of the disease, which is also called ‘long COVID-19’ [Citation7]. Therefore, the chronic course of the disease complicates subsequent treatment.
Cytokine storms are described as excessive immune responses to external stimuli. It plays a significant role in the progression from acute viral infection to acute respiratory distress syndrome (ARDS) or multiorgan failure [Citation8]. Some laboratory parameters, such as C-reactive protein (CRP), procalcitonin (PCT), D-dimer and ferritin, showed marked alterations after SARS-COV-2 infection [Citation9–11]. In addition, proinflammatory cytokines and chemokines such as interleukin (IL)‐6, IL-1, IL-8, IL-18, tumour necrosis factor (TNF) and granulocyte-colony stimulating factor have been detected at high levels in COVID-19 patients [Citation2,Citation12–15].
Severe COVID-19 has some unique features, but many of its acute manifestations are similar to sepsis caused by other pathogens, and many severe patients meet the diagnostic criteria for sepsis according to the Sepsis-3 International Consensus [Citation16]. Although viral aetiology has become more acceptable as an important cause of sepsis, viral sepsis remains underdiagnosed [Citation17]. Etiological evidence is necessary for diagnosis; however, blood culture sampling often yields a low positivity rate, while the clinical symptoms of viral infection are often nonspecific. With the cumulative efforts of modern practices, many patients who met the viral sepsis criteria but had weak evidence of infection received excessive antimicrobial therapy. Delayed diagnosis and inadequate treatment continue to affect the outcomes of patients with severe COVID-19. Therefore, other promising biomarkers and candidate targets for the dysregulated inflammatory state should be detected and established, besides the typical biomarkers and drug targets that have been used clinically.
Triggering receptors expressed on myeloid cells (TREMs) are a family of cell surface receptors that participate in innate and adaptive immunity, including inflammatory responses, coagulation, metabolism, neuroplasticity [Citation18–20]. The most widely studied members of the TREM family are TREM-1 and TREM-2, which signal through the same adapter molecule. Their soluble forms (sTREM-1 and sTREM-2) result from shedding of the TREM-1 or TREM-2 ectodomain. TREM-1 can amplify the inflammatory response during infection [Citation21]. Patients admitted to the ICU with septic shock have higher TREM-1 expression [Citation22]. Conversely, TREM-2 was initially believed to be the anti-inflammatory counterpart of TREM-1 [Citation23]. However, further investigations have shown that TREM-2 is associated with the induction of a type 2 immune response to viral infections, which might lead to the development of some chronic lung or respiratory infectious diseases [Citation24–26].
To date, in respiratory infection, especially in viral sepsis caused by SARS-COV-2, the roles of TREM-1 and TREM-2 have not been extensively investigated. This study aimed to provide a preliminary demonstration that TREM-2 and TREM-1 could serve as promising biomarkers or therapeutic targets for severe COVID-19, which is extremely meaningful for improving patient management and benefitting clinical translational research.
Methods and materials
Study design
The expression levels of TREM-1 and TREM-2 were retrospectively evaluated and analysed along with the inflammatory marker levels obtained from 78 adult patients of both genders with confirmed COVID-19 from December 2022 to February 2023 admitted in Department of Pulmonary and Critical Care Medicine of Shandong Provincial Hospital, Jinan, China. All patients were diagnosed with pneumonia using chest computed tomography (CT). In addition, throat swab samples were tested by reverse real-time polymerase chain reaction to detect the presence of SARS-CoV-2. Based on the New Coronavirus Pneumonia Diagnosis and Treatment Program (10th edition) published by the National Health Commission of China, severe pneumonia was defined as dyspnoea, respiratory frequency ≥30/min, blood oxygen saturation ≤93%, partial pressure of arterial oxygen (PaO2)-to-fraction of inspired oxygen (FiO2) ratio ≤300 and/or lung infiltrates >50% within 24–48 h, or respiratory failure, septic shock and/or multiple organ dysfunction or failure. Moderate pneumonia was defined as fever or respiratory tract infection symptoms, with imaging findings indicating pneumonia. Fifty peripheral blood samples from asymptomatic individuals or donors who recovered from mild infection (minor clinical symptoms without imaging manifestations) were used as controls. White blood cells (WBCs) and plasma were isolated from whole blood samples, flash-frozen and stored in liquid nitrogen at −80 °C until use.
Enzyme-linked immunosorbent assay
Soluble TREM-1 and TREM-2 are secretory proteins. The concentrations of human sTREM-1 and sTREM-2 (J&l Biological, Shanghai, China) in the plasma samples were measured according to the manufacturer’s instructions. The clinical samples included 78 patients with COVID-19 (34 Moderate pneumonia and 44 with severe pneumonia) and 50 non-pneumonia controls. The differences in sTREM-1 and sTREM-2 expression between non-pneumonia donors and patients with different severities were analysed. Meanwhile, R packages (‘pROC’ and ‘verification’) were used to draw receiver operating characteristic (ROC) curves and calculate the P values of sTREM-1, sTREM-2 and clinical parameters.
Flow cytometric analysis
WBCs were thawed and analysed using a panel of antibodies as follows: FITC anti-human CD3 (clone UCHT1, TONBO), APC-Cyanine7 anti-human CD4 (clone RPA-T4, TONBO), PerCP-Cyanine5.5 anti-human CD8a (clone OKT8, TONBO), PE/Dazzle™ 594 anti-human CD19 (clone HIB19, BioLegend), PE-Cyanine7 anti-human CD14 (clone 61D3, TONBO), PE anti-human CD354 (TREM-1, BioLegend) and human/mouse TREM2 APC-conjugated antibody (clone 237920, R&D Systems). The cell number was generally at least 5 × 106 cells/tube. The WBCs were washed twice, resuspended in 100 μl of phosphate-buffered saline and stained with Ghost Dye™ Red 710 (TONBO) for cell viability testing. The sections were then stained with appropriately diluted concentrations of the antibodies. Cells were resuspended, acquired using CytExpert software (Beckman Coulter) and analysed using FlowJo software (Treestar).
Statistical analysis
Statistical analysis was performed using GraphPad Prism software (version 5.0), and statistical significance was calculated by Student’s t-test, Mann–Whitney U test, chi-square test or Fisher’s exact test, as appropriate. *P < 0.05, **P < 0.01 and ***P < 0.001 represent significant differences.
Results
Clinical cohorts
COVID-19 patients were recruited in 2022–2023 (n = 78). Out of this total cohort of 78 subjects, 34 were classified as ‘Moderate’ and 44 as ‘Severe’ according to criteria described in Methods and materials section. The asymptomatic individuals or donors who recovered from a mild infection were classified as ‘non-pneumonia’. The three groups were similar in terms of sex and weight. All COVID-19 patients enrolled had at least one comorbidity, with the most common being pneumonia. Typical thoracic CT imaging is shown in Figure S1. The highest-level respiratory support strategy was selected and summarized. Among all patients, 89.74% of patients (70 of 78) required supplemental oxygen. In the severe group, 34.09% (15 of 44) needed superior respiratory support (such as mechanical ventilatory support). The in-hospital mortality rate in severe cases of pneumonitis was 15.91% (7 of 44). The severe group had a much higher neutrophil count and CRP and PCT levels, but significantly lower lymphocyte counts and partial pressure of arterial oxygen (PaO2)-to-fraction of inspired oxygen (FiO2) ratio levels compared to the moderate group. Cohort characteristics are listed in and Supplementary Table 1.
Table 1. Demographics and clinical characteristics of patients with COVID-19.
Expression of sTREM-1 and sTREM-2 was higher in plasma of patients with COVID-19
To investigate and distinguish the roles of sTREM-1 and sTREM-2 during SARS-COV-2 infection, plasma was isolated from asymptomatic individuals or donors recovered from mild, moderate, and severe patients with COVID-19 within one month from the onset of clinical symptoms. sTREM-2 expression was higher in the plasma of patients with COVID-19 than that in the non-pneumonia group, with the highest level observed in the severe group ( and S1A). A similar situation was observed in the sTREM-1 levels in patients with pneumonia and controls ( and S1B). Considering the prominent role of the inflammatory/anti-inflammatory balance and its relevance to clinical processes and outcomes, the relationship between the sTREM-1/sTREM-2 ratio and disease severity were explored. The sTREM-1/sTREM-2 ratio was higher in the moderate group than that in the control group. However, the sTREM-2/sTREM-1 ratio did not differ significantly between the patient and control groups (). To further evaluate whether sTREM-1 and sTREM-2 expression levels could predict illness outcomes, a comparative analysis were performed to test their levels in patients with severe COVID-19. The results showed that sTREM-2 and sTREM-1 levels, but not their ratio, were significantly higher in patients who died (Figure S2C,D). To explore the putative effect of age and gender on the production of sTREM-1 and sTREM-2, their concentration levels were also analysed in different age and gender groups. In addition, no significant statistical differences were observed in the respective groups (Figure S2E–H). Altogether, these data indicated that sTREM-1 and sTREM-2 expression was upregulated after SARS-COV-2 infection, suggesting their potential function in inflammatory regulation in patients with COVID-19.
Figure 1. Comparison of soluble TREM-1, TREM-2 and their ratios between COVID-19 patients and non-pneumonia controls. Concentrations of soluble TREM-2 (A) and TREM-1 (B) in plasma obtained from non-pneumonia donors (non-P, n = 50) and moderate (M, n = 34) and severe (S, n = 44) patients with COVID-19. Comparison of the sTREM-1/sTREM-2 ratio (C) and sTREM-1/sTREM-2 ratio (D) from non-pneumonia donors and COVID-19 patients with different illness severities. Data are displayed as the mean ± SD. Statistical significance was determined by an unpaired t-test. *p < 0.05; ***p < 0.001; ns, not significant.
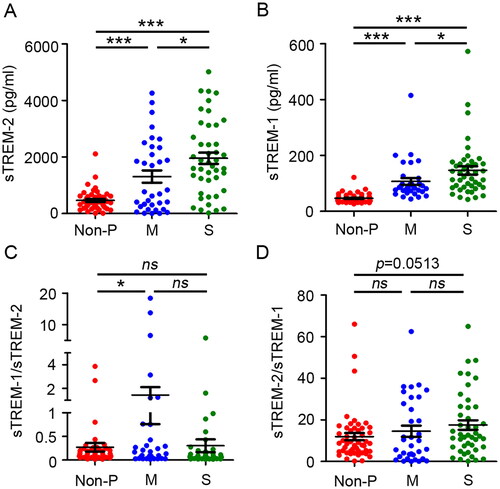
sTREM-2 level was negatively correlated with PaO2/FiO2, but positively correlated with CRP, PCT and IL-6 level, respectively
Many laboratory parameters, such as CRP, PCT and IL-6, have been reported to be associated with the morbidity and mortality of COVID-19. The association between sTREM-1 and sTREM-2 expression, clinical course and laboratory parameters was further assessed by examining the relationship between sTREM-1, sTREM-2, PaO2/FiO2 ratio, CRP, PCT and IL-6 levels. The results showed that sTREM-2 levels were positively correlated with CRP, PCT and IL-6 (CRP: R = 0.3832, P = 0.0007; PCT: R = 0.4184, P = 0.0002; IL-6: R = 0.3495, P = 0.002) and negatively correlated with PaO2/FiO2 (R = −0.2478, P = 0.0309; ). However, sTREM-1 levels were only positively correlated with plasma CRP and PCT levels (CRP: R = 0.2484, P = 0.0316; PCT: R = 0.3031, P = 0.0074), and no significant correlation was found between sTREM-1 and the major indicators of inflammation and severity (IL-6 and PaO2/FiO2 ratio; ). Overall, these data demonstrated that sTREM-2, rather than sTREM-1, was most closely associated with the clinical course of patients with COVID-19.
Figure 2. Correlation analysis between soluble TREM-2, TREM-1 and major clinical parameters. Relationship between soluble TREM-2 and the PaO2/FiO2 ratio (A), CRP (B), PCT (C) and IL-6 (D) in patients with moderate and severe COVID-19. Relationship between soluble TREM-1 and PaO2/FiO2 ratio (E), CRP (F), PCT (G) and IL-6 (H) in patients with COVID-19.
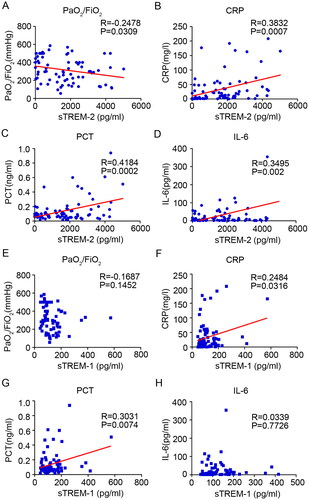
TREM-1 and TREM-2 expression was increased on T cells in patients with COVID-19
Flow cytometry was used to analyse the expression of TREM-1 and TREM-2 on the surface of cell subpopulations, including monocytes, T cells and B cells. The gated strategy is shown in Figure S3. The results showed that TREM-2 was equally expressed in CD14+ monocytes from moderate, severe and control groups. However, the percentage of TREM-1+CD14+ monocytes was slightly higher in the non-pneumonia group than in the COVID-19 (). In contrast, TREM-1 and TREM-2 were selectively expressed on the surfaces of CD4+ and CD8+ T cells. The percentage of TREM-1+CD4+, TREM-2+CD4+, TREM-1+CD8+ and TREM-2+CD8+ T cell subsets was significantly increased in patients with COVID-19 compared to the low levels in controls. However, there was no difference between the moderate and severe patients (). In addition, the percentage of TREM-1+CD19+ cells were also upregulated in severe but not moderate patients with COVID-19 compared to the non-pneumonia group (). Surface TREM-2 was significantly induced in CD19+ cells of moderate COVID-19 (). Collectively, these data suggest that there is an increase in TREM-1+ and TREM-2+ T cells in patients with COVID-19 during the acute phase of SARS-COV-2 infection.
Figure 3. Expression of TREM-1 and TREM-2 on the surface of cell subpopulations. Flow cytometric analysis of TREM-1 expression on the surface of monocytes (A), CD4+ T cells (C), CD8+ T cells (E) and B cells (G) from non-pneumonia donors (n = 50) and patients with moderate (n = 34) and severe COVID-19 (n = 44). Flow cytometric analysis of TREM-2 expression on the surfaces of monocytes (B), CD4+ T cells (D), CD8+ T cells (F) and B cells (H) from non-pneumonia donors or patients with COVID-19. Data are displayed as the mean ± SD. Statistical significance was determined by an unpaired t-test. *p < 0.05; **p < 0.01; ***p < 0.001.
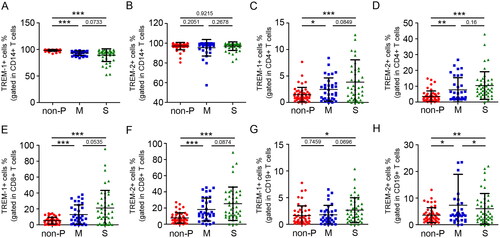
sTREM-1 and sTREM-2 expression was equal to or better than some clinical indicators in distinguishing disease severity
Since sTREM-1 and sTREM-2 play prominent roles in the procession of SARS-COV-2 infection, the authors believe that their expression levels could reflect disease status. ROC curves were used to evaluate the predictive value of sTREM-1 and sTREM-2 for severe infection. Indeed, sTREM-1 and sTREM-2 expression distinguished severe cases a little better than leukocyte and neutrophil subgroup absolute counts and PCT; moreover, it had the same predictive value as traditional inflammatory indicators, such as CRP and IL-6 (sTREM-1: AUC 0.656; sTREM-2: AUC 0.650; ). The positive predictive value (PPV) and negative predictive value (NPV) are shown in Supplementary Table 2. TREM-1 has a PPV of 74.36% and an NPV of 60.87%; TREM-2 has a PPV of 69.57% and an NPV of 63.64%. Collectively, these findings showed that TREM-1 and TREM-2 exhibited potential predictive abilities and indicated that inflammatory and anti-inflammatory imbalance was linked to a poor outcome in SARS-COV-2 infection.
Discussion
To date, anti‐inflammatory treatments have yielded positive results and increased survival in patient with COVID‐19, especially in ‘viral sepsis’ patients. This benefitted from the precise description of the inflammatory response. The present study focused on TREM-1 and TREM-2 levels and showed that both of these parameters, in the plasma or on the cell surface, were increased in patients with COVID-19 compared with the control group. To the best of their knowledge, this study explored the novel roles of TREM family members in the immune response, which might serve as potential diagnostic biological markers or immunotherapeutic targets of COVID-19.
The TREM-1 and TREM-2 had been identified as a reliable indicator of inflammation intensity associated with many lung diseases [Citation27,Citation28]. In the present study, sTREM-2 and sTREM-1 levels that were upregulated in patients with COVID-19 were found, particularly in those who died. However, the sTREM‐1/sTREM‐2 ratio did not exhibit a similar trend. Of note was that sTREM‐1/sTREM‐2 ratio was higher in patients with moderate COVID‐19 compared with those with severe illness and non-pneumonia group. A previous study suggested that the balance between TREM-1 and TREM-2 regulates the progression of acute lung injury and reflects immune status [Citation29]. Similar to influenza infection, COVID-19 with different illness severities or at different times has remarkable heterogeneity in antiviral and inflammatory responses [Citation2,Citation30–32]. Thus, the elevated sTREM-1/sTREM-2 ratio in the plasma indicates that enhanced systemic inflammation may occur in patients with moderate pneumonia. These results showed that the inability of soluble TREM‐2, which plays a role in anti-inflammatory balance, to adequately balance soluble TREM‐1 might be related to moderate disease progression.
A previous report described that a considerable proportion of patients with COVID-19 developed severe disease with respiratory failure, and some of them had ARDS, which used the PaO2/FiO2 to classify the severity [Citation33]. In addition, IL-6, CRP and PCT are significant biomarkers of COVID-19 inflammation [Citation34]. Soluble TREM-2 levels that were positively correlated with CRP, PCT and IL-6 levels but negatively correlated with PaO2/FiO2 were found. Although soluble TREM-1 levels were enhanced in COVID-19 patients, the total expression level at baseline was lower than that of TREM-2. The correlation between sTREM-1 and the inflammatory response or clinical course was also much weaker than that of sTREM-2. Previous research has emphasized that soluble TREM-1 may function as a decoy receptor to downmodulate inflammation, especially in bacterial infections [Citation35]. In contrast, some experimental studies have found that both membrane-bound and soluble forms of TREM-2 contribute to macrophage survival and differentiation after viral infection. Acute viral infection led to an increase in surface TREM-2 expression, which was augmented by IL-13 [Citation36]. IL-13 is a driver of COVID-19 severity [Citation37], which in turn could contribute to the cleavage and formation of soluble TREM-2, which helps to promote the perpetuation of the disease.
TREM-2 and TREM-1 were initially identified as innate myeloid cell receptors. A recent study reported that TREM-2 is expressed in several cell types, including macrophages, microglia, granulocytes, lymphocytes, dendritic cells and endothelial cells [Citation38]. In this study, TREM-1 and TREM-2 were widely expressed on monocytes but were partially and selectively expressed on human T cells and B cells, which was consistent with a previous study [Citation39]. TREM-2 expression has been reported to be altered in the in vitro stimulation of inflammatory contexts, including IFN-γ, TNF, IL-1β and IL-4, and independent of TCR stimulation [Citation40,Citation41]. Some plasma components, such as inflammatory cytokines, may induce TREM-1 or TREM-2 expression in COVID-19 patients.
Some laboratory parameters, such as WBC counts, lymphocyte counts, neutrophil counts, CRP, PCT, IL-6 and PaO2/FiO2, have been extensively investigated and found to be related to illness severity, even mortality of COVID‐19 in previous studies [Citation34]. This study focused on soluble TREM‐1 and TREM‐2 levels and observed that their expression might be more efficient in identifying patients with a severe course than WBC counts, neutrophil counts and PCT levels. Given that elevated PCT levels in individuals are primarily caused by bacterial coinfections, the application value of distinguishing severity after early SARS-COV-2 infection may be weakened [Citation42]. Thus, plasma concentrations of sTREM-1 and sTREM-2 might be potential independent predictors of severe illness in patients with COVID-19.
However, this study had several limitations. Firstly, owing to the relatively small sample size, their grouping was heterogeneous with regard to the underlying disease. Further large-scale prospective studies that provide corroborative evidence may have substantial clinical implications. Secondly, the kinetics of TREM-1, TREM-2, IL-6, CRP and PCT measurements were deficient because of the retrospective design of the study. Incorporating these dynamic data may provide more power for this study. Finally, unmeasured confounders, such as related primary disease, therapy delay and prior corticosteroid use, could have resulted in residual confounding.
Authors contributions
Ruyue Fan, Zuowang Cheng and Shuai Liu conceived of the project. Ruyue Fan, Zuowang Cheng, Zhisheng Huang, Bo Liu and Shuai Liu discussed and designed the experiments. Ying Yang, Peibin Hou and Ruyue Fan conducted experiments. Shuai Liu, Na Sun, Bin Hu and Chuanjun Huang performed statistical analyses. Ruyue Fan and Shuai Liu prepared the manuscript. All authors interpreted the results and edited the manuscript accordingly. All authors have read and approved the final manuscript.
Ethics statement
Experiments involving human participants were conducted in accordance with the Declaration of Helsinki and approved by the Shandong Provincial Hospital Affiliated to Shandong First Medical University Ethics Committee (Approval No. 2023-805) in accordance with the guidelines for the protection of human volunteers. All participants provided written informed consent to participate in the study.
Supplemental Material
Download Zip (1.8 MB)Acknowledgements
The authors thank the National Natural Science Foundation of China for its support.
Disclosure statement
No potential conflict of interest was reported by the author(s).
Data availability statement
The data used in the current study are accessible from the corresponding authors upon reasonable request.
Additional information
Funding
References
- Huang L, Li X, Gu X, et al. Health outcomes in people 2 years after surviving hospitalisation with COVID-19: a longitudinal cohort study. Lancet Respir Med. 2022;10(9):1–9. doi: 10.1016/S2213-2600(22)00126-6.
- Zhou F, Yu T, Du R, et al. Clinical course and risk factors for mortality of adult inpatients with COVID-19 in Wuhan, China: a retrospective cohort study. Lancet. 2020;395(10229):1054–1062. doi: 10.1016/S0140-6736(20)30566-3.
- Li J, Lai S, Gao GF, et al. The emergence, genomic diversity and global spread of SARS-CoV-2. Nature. 2021;600(7889):408–418. doi: 10.1038/s41586-021-04188-6.
- Hu B, Guo H, Zhou P, et al. Characteristics of SARS-CoV-2 and COVID-19. Nat Rev Microbiol. 2021;19(3):141–154. doi: 10.1038/s41579-020-00459-7.
- Wiersinga WJ, Rhodes A, Cheng AC, et al. Pathophysiology, transmission, diagnosis, and treatment of coronavirus disease 2019 (COVID-19): a review. JAMA. 2020;324(8):782–793. doi: 10.1001/jama.2020.12839.
- Palmieri L, Vanacore N, Donfrancesco C, et al. Clinical characteristics of hospitalized individuals dying with COVID-19 by age group in Italy. J Gerontol A Biol Sci Med Sci. 2020;75(9):1796–1800. doi: 10.1093/gerona/glaa146.
- Davis HE, McCorkell L, Vogel JM, et al. Long COVID: major findings, mechanisms and recommendations. Nat Rev Microbiol. 2023;21(3):133–146. doi: 10.1038/s41579-022-00846-2.
- Mehta P, McAuley DF, Brown M, et al. COVID-19: consider cytokine storm syndromes and immunosuppression. Lancet. 2020;395(10229):1033–1034. doi: 10.1016/S0140-6736(20)30628-0.
- Liu F, Li L, Xu M, et al. Prognostic value of interleukin-6, C-reactive protein, and procalcitonin in patients with COVID-19. J Clin Virol. 2020;127:104370. doi: 10.1016/j.jcv.2020.104370.
- Tan C, Huang Y, Shi F, et al. C-reactive protein correlates with computed tomographic findings and predicts severe COVID-19 early. J Med Virol. 2020;92(7):856–862. doi: 10.1002/jmv.25871.
- Lippi G, Plebani M. Procalcitonin in patients with severe coronavirus disease 2019 (COVID-19): a meta-analysis. Clin Chim Acta. 2020;505:190–191. doi: 10.1016/j.cca.2020.03.004.
- Huang C, Wang Y, Li X, et al. Clinical features of patients infected with 2019 novel coronavirus in Wuhan, China. Lancet. 2020;395(10223):497–506. doi: 10.1016/S0140-6736(20)30183-5.
- Ruan Q, Yang K, Wang W, et al. Clinical predictors of mortality due to COVID-19 based on an analysis of data of 150 patients from Wuhan, China. Intensive Care Med. 2020;46(5):846–848. doi: 10.1007/s00134-020-05991-x.
- Wilson JG, Simpson LJ, Ferreira AM, et al. Cytokine profile in plasma of severe COVID-19 does not differ from ARDS and sepsis. JCI Insight. 2020;5(17):e140289. doi: 10.1172/jci.insight.140289.
- Qin R, He L, Yang Z, et al. Identification of parameters representative of immune dysfunction in patients with severe and fatal COVID-19 infection: a systematic review and meta-analysis. Clin Rev Allergy Immunol. 2023;64(1):33–65. doi: 10.1007/s12016-021-08908-8.
- Singer M, Deutschman CS, Seymour CW, et al. The third international consensus definitions for sepsis and septic shock (sepsis-3). JAMA. 2016;315(8):801–810. doi: 10.1001/jama.2016.0287.
- Ljungström LR, Jacobsson G, Claesson BEB, et al. Respiratory viral infections are underdiagnosed in patients with suspected sepsis. Eur J Clin Microbiol Infect Dis. 2017;36(10):1767–1776. doi: 10.1007/s10096-017-2990-z.
- Colonna M. The biology of TREM receptors. Nat Rev Immunol. 2023;23(9):580–594. doi: 10.1038/s41577-023-00837-1.
- Klesney-Tait J, Turnbull IR, Colonna M. The TREM receptor family and signal integration. Nat Immunol. 2006;7(12):1266–1273. doi: 10.1038/ni1411.
- Deczkowska A, Weiner A, Amit I. The physiology, pathology, and potential therapeutic applications of the TREM2 signaling pathway. Cell. 2020;181(6):1207–1217. doi: 10.1016/j.cell.2020.05.003.
- Arts RJ, Joosten LA, van der Meer JW, et al. TREM-1: intracellular signaling pathways and interaction with pattern recognition receptors. J Leukoc Biol. 2013;93(2):209–215. doi: 10.1189/jlb.0312145.
- Matos AO, Dantas P, Silva-Sales M, et al. TREM-1 isoforms in bacterial infections: to immune modulation and beyond. Crit Rev Microbiol. 2021;47(3):290–306. doi: 10.1080/1040841X.2021.1878106.
- Liu D, Dong Y, Liu Z, et al. Impact of TREM-2 gene silencing on inflammatory response of endotoxin-induced acute lung injury in mice. Mol Cell Biochem. 2014;394(1-2):155–161. doi: 10.1007/s11010-014-2091-6.
- Hoogendijk AJ, Blok DC, Garcia Laorden MI, et al. Soluble and cell-associated triggering receptor expressed on myeloid cells-1 and -2 in patients with pulmonary tuberculosis. J Infect. 2015;71(6):706–709. doi: 10.1016/j.jinf.2015.09.002.
- Zhu Z, Zhang X, Dong W, et al. TREM2 suppresses the proinflammatory response to facilitate PRRSV infection via PI3K/NF-κB signaling. PLoS Pathog. 2020;16(5):e1008543. doi: 10.1371/journal.ppat.1008543.
- Hiremath J, Renu S, Tabynov K, et al. Pulmonary inflammatory response to influenza virus infection in pigs is regulated by DAP12 and macrophage M1 and M2 phenotypes. Cell Immunol. 2020;352:104078. doi: 10.1016/j.cellimm.2020.104078.
- Suchankova M, Urban J, Ganovska M, et al. TREM-1 and TREM-2 expression on CD14(+) cells in bronchoalveolar lavage fluid in pulmonary sarcoidosis and hypersensitivity pneumonitis in the context of T cell immune response. Mediators Inflamm. 2020;2020:9501617–9501619. doi: 10.1155/2020/9501617.
- Wang TY, Feng JY, Shu CC, et al. Plasma concentrations of sTREM-1 as markers for systemic adverse reactions in subjects treated with weekly rifapentine and isoniazid for latent tuberculosis infection. Front Microbiol. 2022;13:821066. doi: 10.3389/fmicb.2022.821066.
- Sun GY, Guan CX, Zhou Y, et al. Vasoactive intestinal peptide re-balances TREM-1/TREM-2 ratio in acute lung injury. Regul Pept. 2011;167(1):56–64. doi: 10.1016/j.regpep.2010.11.008.
- Ren X, Wen W, Fan X, et al. COVID-19 immune features revealed by a large-scale single-cell transcriptome atlas. Cell. 2021;184(7):1895–1913.e19. doi: 10.1016/j.cell.2021.01.053.
- Zhang F, Gan R, Zhen Z, et al. Adaptive immune responses to SARS-CoV-2 infection in severe versus mild individuals. Signal Transduct Target Ther. 2020;5(1):156.
- Zhang JY, Wang XM, Xing X, et al. Single-cell landscape of immunological responses in patients with COVID-19. Nat Immunol. 2020;21(9):1107–1118. doi: 10.1038/s41590-020-0762-x.
- Ranieri VM, Rubenfeld GD, Thompson BT, et al. Acute respiratory distress syndrome: the Berlin definition. JAMA. 2012;307(23):2526–2533. doi: 10.1001/jama.2012.5669.
- Ponti G, Maccaferri M, Ruini C, et al. Biomarkers associated with COVID-19 disease progression. Crit Rev Clin Lab Sci. 2020;57(6):389–399. doi: 10.1080/10408363.2020.1770685.
- Jiyong J, Tiancha H, Wei C, et al. Diagnostic value of the soluble triggering receptor expressed on myeloid cells-1 in bacterial infection: a meta-analysis. Intensive Care Med. 2009;35(4):587–595. doi: 10.1007/s00134-008-1333-z.
- Wu K, Byers DE, Jin X, et al. TREM-2 promotes macrophage survival and lung disease after respiratory viral infection. J Exp Med. 2015;212(5):681–697. doi: 10.1084/jem.20141732.
- Donlan AN, Sutherland TE, Marie C, et al. IL-13 is a driver of COVID-19 severity. JCI Insight. 2021;6(15):e150107. doi: 10.1172/jci.insight.150107.
- Matos AO, Dantas P, Queiroz H, et al. TREM-2: friend or foe in infectious diseases? Crit Rev Microbiol. 2022;20(1):1–19. doi: 10.1080/1040841X.2022.2146481.
- Wu Y, Wu M, Ming S, et al. TREM-2 promotes Th1 responses by interacting with the CD3ζ-ZAP70 complex following Mycobacterium tuberculosis infection. J Clin Invest. 2021;131(17):e137407.
- Jay TR, von Saucken VE, Landreth GE. TREM2 in neurodegenerative diseases. Mol Neurodegener. 2017;12(1):56.
- Wu Y, Wang M, Yin H, et al. TREM-2 is a sensor and activator of T cell response in SARS-CoV-2 infection. Sci Adv. 2021;7(50):eabi6802. doi: 10.1126/sciadv.abi6802.
- Williams P, McWilliams C, Soomro K, et al. The dynamics of procalcitonin in COVID-19 patients admitted to intensive care unit—a multi-centre cohort study in the South West of England, UK. J Infect. 2021;82(6):e24–e26. doi: 10.1016/j.jinf.2021.03.011.