Abstract
Diabetic nephropathy (DN) is a severe complication of diabetes mellitus, causing a substantive threat to the public, which receives global concern. However, there are limited drugs targeting the treatment of DN. Owing to this, it is highly crucial to investigate the pathogenesis and potential therapeutic targets of DN. The process of ferroptosis is a type of regulated cell death (RCD) involving the presence of iron, distinct from autophagy, apoptosis, and pyroptosis. A primary mechanism of ferroptosis is associated with iron metabolism, lipid metabolism, and the accumulation of ROS. Recently, many studies testified to the significance of ferroptosis in kidney tissue under diabetic conditions and explored the drugs targeting ferroptosis in DN therapy. Our review summarized the most current studies between ferroptosis and DN, along with investigating the significant processes of ferroptosis in different kidney cells, providing a novel target treatment option for DN.
1. Introduction
Diabetic nephropathy (DN) is a severe complication of diabetes mellitus, the prevalence of which is now estimated to reach 34.2 million individuals, poses a tremendous threat to the public and causes lots of concerns [Citation1]. In addition, DN is also the leading cause of end-stage renal disease (ESRD), which is hugely burdensome for patients and their families. However, most of the DN mechanism is still unknown, and there aren’t enough medications specifically targeting DN to slow down the DN progression [Citation2]. In response to persistently high blood glucose levels, advanced glycation end-products (AGEs), cytokines, growth factors, and inflammation factors are produced, breaking down the internal environment homeostasis and triggering oxidative stress (OS), endoplasmic reticulum stress, inflammation, mitochondrial dysfunction, ultimately inducing various regulated cell death forms, which make up the primary pathogenesis of DN [Citation3–5].
Recently, one type of regulated cell death (RCD) named ferroptosis, characterized by iron-dependent lipid peroxidation, glutathione (GSH) depletion, and glutathione peroxidase 4 (GPX4) inactivation, has gained tremendous popularity in DN events [Citation6]. In the context of DN, therapeutic approaches targeting ferroptosis prevent inflammation and fibrotic processes brought on by specific cell death modalities, blazing new trails in DN therapy [Citation7]. However, most of the current ferroptosis research in DN is still conducted on animals or cells, and our knowledge of humans is still quite limited. Therefore, in this review, we presented the modern view on ferroptosis in the pathogenesis of DN and demonstrated the potential benefit of ferroptosis in DN therapy to offer a foundation for further research ().
2. Background of ferroptosis
The term ‘ferroptosis’, which was coined in 2012, characterizes an abnormal form of RCD that is distinguishable from apoptosis, autophagy, as well as pyroptosis by exhibiting smaller mitochondria, disappearing mitochondrial crest, and increased mitochondrial membrane density but not chromatin condensation and margination, cytoplasmic and organelle expansion, plasma membrane rupture, or double-membrane contained vesicles [Citation8]. The emergence of OS and extensive membrane lipid peroxidation that resulted in the loss of the plasma membrane selective permeability triggered these abnormal cell responses. In this regard, based on the ongoing in-depth study of ferroptosis, the primary regulatory pathways of ferroptosis might be summarized as the following: the influence of lipid metabolism, ROS accumulation, and iron metabolism, as illustrated in [Citation9].
Figure 2. Overview of the ferroptosis mechanism. Primary regulatory pathways of ferroptosis might be summarized as the influence of lipid metabolism, ROS accumulation, and iron metabolism. GSH, glutathione; GPX4, glutathione peroxidase 4; LIP, labile iron pool; Nrf2, nuclear-factor- erythroid2-related factor 2; TRF, transferrin; LPO, lipid peroxide; H2O2, hydrogen peroxide; · OH, producing toxic hydroxyl radicals; Fe2+, divalent iron ionization; PUFAs, polyunsaturated fatty acids; ROS, reactive oxygen species; Cys, cystine; Glu, glutamate; PRMT4, Protein Arginine Methyltransferase 4; KLF2, Kruppel-like factor 2; This figure is created with BioRender.com.
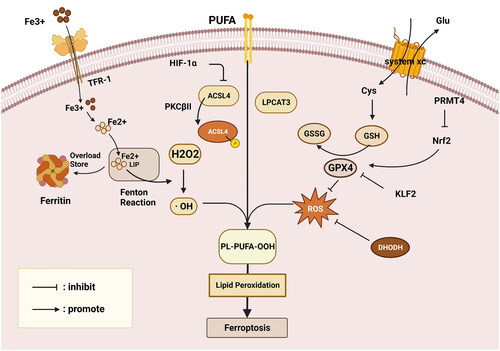
3. The mechanism of ferroptosis
3.1. Iron metabolism: cornerstone
Ferroptosis, as its name implies, relies on iron, the metabolism of which is essential for preserving homeostasis [Citation10], and in which ferritin, which serves to store extracellular iron, is the primary contributor [Citation11]. Moreover, another strong motivator of ferroptosis is the increased intracellular labile iron pool (LIP), as evidenced by the findings of NFE2L2/nuclear-factor-E2-related factor (Nrf2) deletion, which leads to apoferritin accumulation, an enlarged LIP, and enhanced sensitivity to ferroptosis [Citation12]. In addition, it is not surprising that transferrin (TFR) is vital in maintaining systemic iron homeostasis and ferroptosis. For example, the hepatocyte-specific TFR has the potential ability to transport Fe3+ into the tissues for utilization, thereby inhibiting ferroptosis to alleviate fibrosis in liver tissue [Citation13]. Owing to the impact of ferritin and transferrin, the concentration of intracellular free ionic iron alteration influences the occurrence of the Fenton reaction, the essence of which is that the divalent iron ionization (Fe2+) catalyzes hydrogen peroxide (H2O2), producing toxic hydroxyl radicals (· OH), thereby sparking cellular lipid peroxidation, ultimately provoking ferroptosis [Citation14]. Conversely, a Fenton-independent reaction contributes to ferroptosis due to a novel lipid peroxide (LPO) generator associated with GSH and iron redox couple [Citation15].
3.2. Lipid metabolism: bracket
Despite the fact that unrestrained lipid peroxidation is a trademark of ferroptosis, current findings have disproved the theory that free fatty acids are the root cause of the condition. As demonstrated by the interaction between ROS and polyunsaturated fatty acids (PUFAs) on the lipid membrane, PUFA activation is more critical, leading to lipid hydroperoxides generation and cell ferroptosis [Citation9]. Along the same line, exogenous administration of monounsaturated fatty acids (MUFAs) reduces the levels of PUFAs to perform as an anti-ferroptosis agent to suppress the buildup of lipid peroxides [Citation16]. Notably, a fascinating study on the dietary exogenous intake of MUFAs or n-3 long-chain PUFA reveals that the latter exhibits lipid peroxidation and accelerates ferroptosis to delay tumor progression [Citation17].
The thorough studies on PUFA have revealed that several vital enzymes govern the activation of PUFAs, such as lysophosphatidylcholine acyltransferase 3 (LPCAT3) and acyl-coenzyme A (CoA) synthetase long-chain family member 4 (ACSL4) [Citation18]. For example, PKCβII, a molecule that can sense early lipid peroxides, can increase lipid peroxidation to stimulate ferroptosis by phosphorylating and activating ACSL [Citation19]. Another research revealed that ACSL4 expression was suppressed by HIF-1α, reducing lipid peroxidation and inhibiting ferroptosis in ischemic stroke [Citation20]. Except for ACSL4, a novel form of anti-ferroptosis induced by iPLA2β must be taken into consideration since it performs a protective role by liberating oxidized fatty acids from phospholipids and forcing the acyl tails to separate from the lipid glycerol backbone [Citation21].
3.3. Reactive oxygen species (ROS) accumulation: crossbeam
ROS accumulation is another crucial aspect of lipid peroxidation that is relevant to GPX4, which is a GSH-dependent, selenocysteine-containing enzyme that catalyzes the conversion of certain lipid hydroperoxides into lipid alcohols to detoxicate and makes use of the selenium to halt hydroperoxide-induced ferroptosis [Citation22]. Under the condition of GSH, GPX4 continuously reduces the generation of PLOOHs. In contrast, this phenomenon will be revised by either GPX4 inactivation or GSH depletion, leading to lipid peroxidation and ferroptosis [Citation23]. Furthermore, GPX4 is tightly associated with a specialized cystine transporter named system xc, which influences cysteine content and GSH synthesis by exchanging extracellular cystine (Cys) for intracellular glutamate (Glu), eventually affecting the activity of GPX4 to reduce high levels of ROS [Citation24]. Alternatively, the degree of GPX4 transcription also has a significant role. A thorough investigation revealed that Protein Arginine Methyltransferase 4 (PRMT4) interfered with Nrf2 nuclear translocation by interaction, which decreased GPX4 transcription and ultimately aided in ferroptosis [Citation25]. Likewise, GPX4 was coupled to Kruppel-like factor 2 (KLF2), a transcription factor family member with conserved zinc-finger domains, which affected its transcriptional control to encourage ferroptosis in clear cell renal cell carcinoma [Citation26]. An additional strategy for lowering the accumulation of ROS is dihydroorotate dehydrogenase (DHODH), which is responsible for converting ubiquinone (CoQ) into ubiquinol (CoQH2), an antioxidant that traps free radicals and has anti-ferroptosis activity [Citation27]. All these processes serve to maintain homeostasis and inhibit the buildup of ROS.
4. Ferroptosis in kidney disease
Generally speaking, when exposed to stress circumstances such as hypoxia, OS, and ER stress, kidney cells are apt to undergo different forms of regulated cell death, especially ferroptosis. An avalanche of studies identifies the role of ferroptosis in kidney diseases, such as acute kidney injury (AKI), renal fibrosis, and renal clear-cell carcinoma. According to previous studies, the proximal tubular enzyme Myo-inositol oxygenase (MIOX), highly expressed in the proximal tubule, had a pro-ferroptosis effect in AKI [Citation28]. In addition to MIOX, heme scavenging protein, Hemopexin, accumulated in the proximal tubules, resulting in a higher level of heme oxygenase-1 (HO-1) and stimulating ferroptosis in AKI [Citation29]. Ferroptosis suppressor protein 1 (FSP1) depletion contributed to acute tubular necrosis, which is the morphological characteristic of AKI. A newly synthesized small molecule inhibitor (Nec-1f) also showed its function in anti-ferroptosis [Citation30]. HIF-1α had a low expression in HK-2 cells and NRK-52E cells under AKI conditions, while the ASCL4 showed the opposite results. Later test experiments demonstrated that HIF-1α is capable of binding to the promoter of ACSL4 and regulating it in the reverse direction, finally triggering ferroptosis [Citation31]. Furthermore, activated farnesoid X receptor (FXR) could modulate the transcription and expression of ferroptosis-related genes, inhibiting ferroptosis and reducing kidney injury [Citation32].
What’s more, studies in HEK-293 cells demonstrating that HIF-2α stimulated the lipid droplet-associated protein (HILPDA) and specifically enriched rate-limiting substrates for lipid peroxidation to trigger ferroptosis to indicate that ferroptosis is still essential for the pathophysiology of renal clear cell carcinoma [Citation33]. Similar to this, investigations revealed that ferroptosis related to the pathological process of renal fibrosis, an outcome of many kidney disorders, as demonstrated by the studies in unilateral ureteral obstruction mice that Lip-1 exerts the function of anti-fibrosis by inhibiting ferroptosis in HK-2 cells [Citation34]. Based on these data, we find a close connection between ferroptosis and kidney diseases. As for the significance of DN in kidney diseases, many scientists focus on the ferroptosis in DN.
5. Ferroptosis in diabetic nephropathy
DN, a microvascular complication of diabetes, alters the ultrastructure and function of the kidneys due to constant stimulation of the blood vessels of the kidneys by high blood glucose, which is connected to multiple kidney cells such as tubular epithelial cells (TECs), mesangial cells, podocytes, and endothelial cells [Citation35]. In the face of the interaction of metabolic derangements, oxidative stress, dyslipidemia and hemodynamic alterations, and immune dysregulation, kidney cells exhibit RCD, including autophagy, apoptosis, pyroptosis, and ferroptosis. Iron homeostasis is essential for kidney cells to function correctly, so it is not surprising to see the appearance of ferroptosis in DN [Citation36]. In 2020, researchers first looked into the connection between ferroptosis and DN. In the face of the prominent expression of ferroptosis-related marker ACSL4 and the increased iron content in DN, they decided to examine their presumptions. They discovered that ACSL4 knockdown or overexpression could alter renal tubular cell sensitivity to ferroptosis through both in vivo and in vitro tests. Moreover, the ACSL4 inhibitor rosiglitazone ameliorates DN by blocking ferroptosis in renal tubular cells, highlighting the significance of ferroptosis in DN [Citation7]. Moreover, HIF-1α and HO-1 levels increased in db/db animals, but this was countered by the ferroptosis inhibitor ferrostatin-1, which resulted in decreased iron levels in ferritin and transferrin. This suggests that HIF-1α/HO-1 signaling is another way to induce ferroptosis in diabetic conditions [Citation37]. Based on this, researchers investigate the key molecules exclusively expressed in kidney tubules and the intercellular communication during ferroptosis within the kidney through advanced single-cell RNA sequencing (scRNA-seq) [Citation38].
5.1. Tubular epithelial cell (TEC) ferroptosis in DN
Kidney biopsy samples from DN patients commonly show low levels of the ferroptosis-related biomarkers solute carrier family 7, member 11 (SLC7A11), and GPX4. The phenomenon has been proven by further in vitro and in vivo experiments. Similar characteristics of mitochondria in the group of TGF-β1 and Erastin are seen on transmission electron microscopy, including shrinking of the mitochondria and disappearance of mitochondrial cristae. Parallel to this, it has been discovered that the TGF-β1-induced ferroptosis in NRK-52E cells can be abolished when treated with Ferrostatin-1, a kind of ferroptosis inhibitor [Citation39]. From then on, scientists began investigating the particular mechanisms that drive ferroptosis in renal tubular epithelial cells.
5.1.1. Nrf2 signaling
The transcription factor Nrf2, which is a crucial regulator of the cellular antioxidant response to governing the expression of genes that combat oxidative and electrophilic stressors, can regulate downstream target genes to resist peroxidation and ferroptosis [Citation40]. Notably, Nrf2 was downregulated in high glucose (HG)-induced HK-2 cells, along with iron overload, massive ROS, and lipid peroxidation. One study revealed two anti-ferroptosis pathways based on Nrf2 as shown by that Nrf2 knockdown functioned its role in antioxidant stress by inhibiting GPX4 and SLC7A11 as well as its role in regulating iron metabolism by stimulating FTH-1 and TFR-1.[Citation41]. Consistently, a derivative of coumarin called umbelliferone reversed the Nrf2 downregulation in HK-2 cells and diabetic mice, increasing HO-1 expression, a crucial antioxidant enzyme, ultimately inhibiting the onset of ferroptosis and delaying DN progression [Citation42]. A similar result was obtained after treatment with empagliflozin, which activated AMPK and Nrf2, as well as ferroptosis markers like GPX4, SLC7A11, and FTH1, indicating the function of AMPK/Nrf2 signaling in suppressing ferroptosis [Citation43]. In addition, there is a relationship between endoplasmic reticulum stress (ERS) and ferroptosis, as shown by the fact that ERS triggers the high expression of Hrd1 and the interaction between Hrd1 and Nrf2, which promote the ubiquitylation of Nrf2 to accelerate ferroptosis[Citation44]. As a bioactive peptide, salicin-β is abundantly expressed in the kidneys to inactivate Nrf-2 signaling, thus contributing to HG-induced ferroptosis[Citation45]. Furthermore, CAR inhibits ferroptosis and inflammation by regulating the Nrf2 axis [Citation46].
5.1.2. GPX4 signaling
Compared to the healthy controls, GPX4 had a lower expression in kidney biopsy of DN patients, which is primarily expressed in kidney tubulointerstitium, especially in TECs, confirming its position as a standalone predictor of developing ESKD [Citation47]. Similar GPX4 and SLC7A11 downregulation were also observed in renal biopsy samples taken from DN patients compared to non-diabetic renal disease patients [Citation43]. Recent experimental evidence indicated that the natural ingredient Platycodin D (PD) promoted the levels of GPX4 to inhibit ferroptosis in HK-2 cells, relieving the accumulation of lipid ROS production and Fe2+ levels [Citation48]. Similar outcomes are seen in dog renal TECs (Madin-darby canine kidney, or MDCK) under high glucose conditions, which exhibit reduced GPX4 expression and improved expression of TXNIP, high-mobility group box-1 (HMGB1), and ferritin. Further experiments demonstrated that N-acetylcysteine (NAC) and insulin combination therapy had an anti-ferroptosis ability in renal TEC, likely by binding Sirt3 and increasing the expression of Sirt3 and GPX4 expression while inhibiting that of sod2, indicating a novel signaling SIRT3-SOD2-Gpx4 in ameliorating DN through preserving the mitochondrial homeostasis and alleviating ferroptosis [Citation49]. In mice RTECs, Bone morphogenetic protein-7 (BMP7), an antagonist of TGF-β, has been shown to accelerate the regeneration of pancreatic beta cells to inhibit ferroptosis through GPX4 signaling [Citation50].
5.1.3. SLC40A1 signaling
Depending on the contribution of the solute carrier family to ferroptosis, the latest findings showed that some drugs targeting SLC40A1 inhibited ferroptosis in DKD. One of these demonstrated that for HK-2 cells treated with DAPA, the high levels of intracellular iron and GSH, along with the low expression of GPX4 and SCL7A11 brought on by ferroptosis, could be reversed. Interestingly, SLC40A1, the only iron export protein discovered in mammals, displayed higher expression in the DAPA group compared to the HG group. The additional experiment proved that SLC40A1 ubiquitination was markedly elevated by HG administration in HK-2 cells but decreased by DAPA therapy, a drug that could directly combine and stabilize SLC40A1 through bioinformatics verification [Citation51].
Additionally, some studies revealed some other regulatory mechanisms of ferroptosis in DKD. ZRT/IRT-like protein 14 (ZIP14), a transport protein that facilitates cellular iron uptake, is significantly increased in DN. This increase promoted the ferrous iron (Fe2+) levels both in vivo and in vitro, as well as the expression of malondialdehyde (MDA), whereas it decreased the levels of GPX4 and glutathione. Nevertheless, Fer-1 administration can interrupt the process, revealing a unique pro-ferroptosis function for ZIP14 in DN [Citation52]. In the rat renal tubular epithelial cells (NRK-52E), a form of traditional Chinese medicine glabridin (Glab) exhibited its anti-ferroptosis capability by increasing GSH activity, GPX4, SLC7A11, and SLC3A2 expression, lowering MDA and iron concentrations, and upregulating TFR1 expression. Further research supports the theory that VEGF/Akt/ERK is the primary mechanism through which glab improves the progress of DN [Citation53]. The latest study discovered that Aspirin, a classical medicine to make it function by inhibiting cyclooxygenase-2 (COX2), exhibited its protective role in inhibiting ferroptosis in DN. Owing to the overexpression of COX-2, which is an enzyme expressed in the proximal tubule, causing cellular redox damage, while COX-2 knockdown reversed the alterations in the sensors of ferroptosis metabolism, such as GPX4 activity, lipid hydroperoxidation, and GSH levels [Citation54]. Moreover, some natural extracts like total flavones (TFA) from Abelmoschus manihot and Calycosin (C16H12O5) from Astragali Radix (AR) showed the protective role of anti-ferroptosis to ameliorate diabetic kidney injury in HK-2 cells similar to dapagliflozin administration in DN treatment via antioxidation and improving iron deposition [Citation55, Citation56].
5.2. Podocytes ferroptosis in DN
Studies have looked into the process of ferroptosis in podocytes and the damage mechanism in renal tubules. Ferroptosis and podocytes may be related, as shown by the GSH depletion in podocytes and the rise in ROS levels. Germacrone mitigated mitochondria damage and ferroptosis in HG-induced podocytes by promoting upregulation of mmu_mmu_circRNA_0000309, a novel identified circular RNA. This study revealed a ceRNA regulatory network among mmu_mmu_circRNA_0000309, miR-188-3p, and GPX4 [Citation57]. Furthermore, Ginkgolide B (GB), the active component of the herbal remedy Ginkgo biloba, was used in a recent investigation on mouse podocytes and DN mice to explore another mechanism of GPX4. GB performed its anti-ferroptosis function by inhibiting GPX4 ubiquitination and promoting its expression and role in renal fibrosis by suppressing α-SMA and Collagen 1 expression, indicating an innovative strategy to alleviate the progression of DN [Citation58].
In addition to GPX4 signaling, researchers discovered that antioxidant enzymes peroxiredoxin 6 (Prdx6) contributed to podocyte ferroptosis. Low levels of Prdx6 and the transcription factor specificity protein 1 (Sp1) are expressed in response to HG stimulation. This expression diminished with rising blood glucose levels and was time-dependent. Subsequent investigations confirmed that the overexpression of prdx6 combined with sp1 to reverse the HG-induced oxidative stress and ferroptosis [Citation59]. Another study revealed a Gpx4/ferroptosis suppressor protein 1/CoQ10 axis in podocytes, which meliorate renal ferroptosis. Then, utilizing systems pharmacology and ‘multi-omics’ analysis, researchers discovered the role of mangiferin monosodium salt (MGM), which inhibits lipid driver ACSL-4-mediated pro-ferroptosis synthesis in the kidney [Citation60].
5.3. Glomerular endothelial cells (GECs) ferroptosis in DN
Under the stimulation of 20 mmol/L D-glucose in human renal glomerular endothelial cells, there is a low expression level of circular ASAP2, which sponges and upregulates miR-770-5p, regulating SOX11/SLC770A5 pathway to increase inflammation, OS, and ferroptosis in DN, thereby providing a novel network of circ ASAP2 to ameliorate kidney injury in DN [Citation61]. According to the latest scientific investigations, Nrf2 showed a significant role in ferroptosis by activating the downstream antioxidant enzyme genes transcription, such as HO-1, SOD2, and GPX4. Furthermore, this axis can be regulated by the AdipoR1/AMPK signaling and AdipoR1 ubiquitination. Hence, Schisandrin A is a potential therapeutic option for DN by modulating AdipoR1 ubiquitination [Citation62]. These findings suggest the close connection between ferroptosis and diabetic nephropathy, which is involved in renal TECs, mesangial cells, podocytes, and GECs.
5.4. Mesangial cells ferroptosis in DN
Although the evidence is scarce, ferroptosis is also observed in mesangial cells. Taking notice of the ferroptosis markers ferritin and LDH upregulation in the serum of DN patients, researchers began to look into the connection between ferroptosis and DN in mesangial cells. Their findings suggested that the downregulation of HMGB1 was inversely linked with Nrf2 and promoted ferroptosis through the Nrf2 signaling pathway [Citation63].
5.4.1. Drugs targeting ferroptosis in DN
Despite numerous studies showing the significance of ferroptosis in DN, there are few medications that specifically target ferroptosis (). Some of them are Chinese traditional medicine. For instance, the complex medication San-Huang-Yi-Shen (SHYS) promotes the cystine/GSH/GPX4 axis to prevent ferroptosis in db/db mice [Citation64]. Similar to this, Emodin, a key component of rhubarb that is useful against DN, demonstrated its renal protective action by preventing ferroptosis by increasing Nrf2 [Citation65]. In addition, some extracts from fruits and plants are useful. Mangiferin monosodium salt (MGM), a special component of mango, has also demonstrated a systemic protective role in DN by regulating lipid metabolism derangements and arachidonic acid (AA) metabolism [Citation60]. A few plant extracts, like as Vitexin, are significant bioactive flavonoid monomers that demonstrate their anti-ferroptosis functions via stimulating GPX4 signaling [Citation66]. In renal tubular epithelial cells, quercetin (QCT), a naturally occurring flavonoid derived from fruits and vegetables, increased the Nrf2/Heme oxygenase-1(HO-1) signaling to anti-ferroptosis [Citation67]. Furthermore, some organic compounds have also demonstrated anti-ferroptosis properties. The capacity of reducing sugars like 2-deoxy-D-ribose (dRib) to prevent the xCT protein from being produced by the ubiquitin-proteasome system (UPS), which reduces intracellular cystine absorption, is another indication that they are implicated in ferroptosis in DN [Citation68]. Additionally, aspirin, a class of nonsteroidal anti-inflammatory drugs (NSAIDs), has reno-protective properties by preventing ferroptosis in PTECs by decreasing COX2 signaling [Citation54].
Table 1. Drugs targeting ferroptosis in DN.
6. Conclusion and perspectives
DN is one of the most severe complications of DM, which lacks efficient therapy to inhibit its progression. Ferroptosis is a brand-new kind of cell death form with unique traits from other RCDs. Its induction mechanisms have been concluded in three parts: iron metabolism, lipid metabolism, and accumulation of ROS. Nowadays, ferroptosis shows its role in DN from the recent studies mentioned above, which may offer a fresh approach to DN treatment. Ferroptosis involves the kidneys’ central cells, such as TECs, podocytes, GECs, and glomerular mesangial cells, and the main pathological changes in these cells lead to the deterioration of the kidneys and the progression of DN. Although several medications that target iron death have also been discovered that decrease the progression of diabetic nephropathy, these findings have only been confirmed in vitro and in vivo, which have not been confirmed in clinical. Besides, further research is required because ferroptosis does not occur alone and is frequently accompanied by other forms of cell death. A prospective observational study revealed that ferroptosis marker GPX4 in venous blood was independently associated with massive proteinuria and was an elevated prediction value for DN. In conclusion, ferroptosis is a novel cell death and plays an important role in DN, providing a new therapeutic direction for patients.
Author contributions
QW wrote and revised the manuscript. FH critically revised the manuscript. Both authors read and approved the final manuscript.
Acknowledgements
We would like to thank BioRender, all figures are created with BioRender.com
Disclosure statement
The authors have no relevant financial or non-financial interests to disclose.
Data availability statement
Data sharing is not applicable to this article as no new data were created or analyzed in this study.
Additional information
Funding
References
- Federation I. IDF Diabetes Atlas, tenth. International Diabetes. 2021.
- Jager KJ, Kovesdy C, Langham R, et al. A single number for advocacy and communication-worldwide more than 850 million individuals have kidney diseases. Kidney Int. 2019;96(5):1–11. doi: 10.1016/j.kint.2019.07.012.
- Anders H-J, Huber TB, Isermann B, et al. CKD in diabetes: diabetic kidney disease versus nondiabetic kidney disease. Nat Rev Nephrol. 2018;14(6):361–377. doi: 10.1038/s41581-018-0001-y.
- Rico-Fontalvo J, Aroca G, Cabrales J, et al. Molecular mechanisms of diabetic kidney disease. Int J Mol Sci. 2022;23(15):8668. doi: 10.3390/ijms23158668.
- Volpe CMO, Villar-Delfino PH, Dos Anjos PMF, et al. Cellular death, reactive oxygen species (ROS) and diabetic complications. Cell Death Dis. 2018;9(2):119. doi: 10.1038/s41419-017-0135-z.
- Wu Y, Chen Y. Research progress on ferroptosis in diabetic kidney disease. Front Endocrinol (Lausanne). 2022;13:945976. doi: 10.3389/fendo.2022.945976.
- Wang Y, Bi R, Quan F, et al. Ferroptosis involves in renal tubular cell death in diabetic nephropathy. Eur J Pharmacol. 2020;888:173574. doi: 10.1016/j.ejphar.2020.173574.
- Dixon SJ, Lemberg KM, Lamprecht MR, et al. Ferroptosis: an iron-dependent form of nonapoptotic cell death. Cell. 2012;149(5):1060–1072. doi: 10.1016/j.cell.2012.03.042.
- Stockwell BR. Ferroptosis turns 10: emerging mechanisms, physiological functions, and therapeutic applications. Cell. 2022;185(14):2401–2421. doi: 10.1016/j.cell.2022.06.003.
- Fang X, Ardehali H, Min J, et al. The molecular and metabolic landscape of iron and ferroptosis in cardiovascular disease. Nat Rev Cardiol. 2023;20(1):7–23. doi: 10.1038/s41569-022-00735-4.
- Fang X, Cai Z, Wang H, et al. Loss of cardiac ferritin H facilitates cardiomyopathy via Slc7a11-Mediated ferroptosis. Circ Res. 2020;127(4):486–501. doi: 10.1161/CIRCRESAHA.120.316509.
- Anandhan A, Dodson M, Shakya A, et al. NRF2 controls iron homeostasis and ferroptosis through HERC2 and VAMP8. Sci Adv. 2023;9(5):eade9585. doi: 10.1126/sciadv.ade9585.
- Yu Y, Jiang L, Wang H, et al. Hepatic transferrin plays a role in systemic iron homeostasis and liver ferroptosis. Blood. 2020;136(6):726–739. doi: 10.1182/blood.2019002907.
- Shen Z, Liu T, Li Y, et al. Fenton-reaction-acceleratable magnetic nanoparticles for ferroptosis therapy of orthotopic brain tumors. ACS Nano. 2018;12(11):11355–11365. doi: 10.1021/acsnano.8b06201.
- He YJ, Liu XY, Xing L, et al. Fenton reaction-independent ferroptosis therapy via glutathione and iron redox couple sequentially triggered lipid peroxide generator. Biomaterials. 2020;241:119911. doi: 10.1016/j.biomaterials.2020.119911.
- Das UN. Saturated fatty acids, MUFAs and PUFAs regulate ferroptosis. Cell Chem Biol. 2019;26(3):309–311. doi: 10.1016/j.chembiol.2019.03.001.
- Dierge E, Debock E, Guilbaud C, et al. Peroxidation of n-3 and n-6 polyunsaturated fatty acids in the acidic tumor environment leads to ferroptosis-mediated anticancer effects. Cell Metab. 2021;33(8):1701–1715.e5. doi: 10.1016/j.cmet.2021.05.016.
- Friedmann Angeli JP, Xavier da Silva TN, Schilling B. CD8(+) T cells PUF(A)ing the flames of cancer ferroptotic cell death. Cancer Cell. 2022;40(4):346–348. doi: 10.1016/j.ccell.2022.03.003.
- Zhang HL, Hu BX, Li ZL, et al. PKCβII phosphorylates ACSL4 to amplify lipid peroxidation to induce ferroptosis. Nat Cell Biol. 2022;24(1):88–98. doi: 10.1038/s41556-021-00818-3.
- Cui Y, Zhang Y, Zhao X, et al. ACSL4 exacerbates ischemic stroke by promoting ferroptosis-induced brain injury and neuroinflammation. Brain Behav Immun. 2021;93:312–321. doi: 10.1016/j.bbi.2021.01.003.
- Chen D, Chu B, Yang X, et al. iPLA2β-mediated lipid detoxification controls p53-driven ferroptosis independent of GPX4. Nat Commun. 2021;12(1):3644. doi: 10.1038/s41467-021-23902-6.
- Ingold I, Berndt C, Schmitt S, et al. Selenium utilization by GPX4 is required to prevent Hydroperoxide-Induced ferroptosis. Cell. 2018;172(3):409–422.e21. e421. doi: 10.1016/j.cell.2017.11.048.
- Ye Y, Chen A, Li L, et al. Repression of the antiporter SLC7A11/glutathione/glutathione peroxidase 4 axis drives ferroptosis of vascular smooth muscle cells to facilitate vascular calcification. Kidney Int. 2022;102(6):1259–1275. doi: 10.1016/j.kint.2022.07.034.
- Parker JL, Deme JC, Kolokouris D, et al. Molecular basis for redox control by the human cystine/glutamate antiporter system xc. Nat Commun. 2021;12(1):7147. () doi: 10.1038/s41467-021-27414-1.
- Wang Y, Yan S, Liu X, et al. PRMT4 promotes ferroptosis to aggravate doxorubicin-induced cardiomyopathy via inhibition of the Nrf2/GPX4 pathway. Cell Death Differ. 2022;29(10):1982–1995. doi: 10.1038/s41418-022-00990-5.
- Lu Y, Qin H, Jiang B, et al. KLF2 inhibits cancer cell migration and invasion by regulating ferroptosis through GPX4 in clear cell renal cell carcinoma. Cancer Lett. 2021;522:1–13. doi: 10.1016/j.canlet.2021.09.014.
- Mao C, Liu X, Zhang Y, et al. DHODH-mediated ferroptosis defence is a targetable vulnerability in cancer. Nature. 2021;593(7860):586–590. doi: 10.1038/s41586-021-03539-7.
- Deng F, Sharma I, Dai Y, et al. Myo-inositol oxygenase expression profile modulates pathogenic ferroptosis in the renal proximal tubule. J Clin Invest. 2019;129(11):5033–5049. doi: 10.1172/JCI129903.
- Fan X, Zhang X, Liu LC, et al. Hemopexin accumulates in kidneys and worsens acute kidney injury by causing hemoglobin deposition and exacerbation of iron toxicity in proximal tubules. Kidney Int. 2022;102(6):1320–1330. doi: 10.1016/j.kint.2022.07.024.
- Tonnus W, Meyer C, Steinebach C, et al. Dysfunction of the key ferroptosis-surveilling systems hypersensitizes mice to tubular necrosis during acute kidney injury. Nat Commun. 2021;12(1):4402. doi: 10.1038/s41467-021-24712-6.
- Wang Y, Zhang M, Bi R, et al. ACSL4 deficiency confers protection against ferroptosis-mediated acute kidney injury. Redox Biol. 2022;51:102262. doi: 10.1016/j.redox.2022.102262.
- Kim DH, Choi HI, Park JS, et al. Farnesoid X receptor protects against cisplatin-induced acute kidney injury by regulating the transcription of ferroptosis-related genes. Redox Biol. 2022;54:102382. doi: 10.1016/j.redox.2022.102382.
- Zou Y, Palte MJ, Deik AA, et al. A GPX4-dependent cancer cell state underlies the clear-cell morphology and confers sensitivity to ferroptosis. Nat Commun. 2019;10(1):1617. doi: 10.1038/s41467-019-09277-9.
- Zhang B, Chen X, Ru F, et al. Liproxstatin-1 attenuates unilateral ureteral obstruction-induced renal fibrosis by inhibiting renal tubular epithelial cells ferroptosis. Cell Death Dis. 2021;12(9):843. doi: 10.1038/s41419-021-04137-1.
- Oshima M, Shimizu M, Yamanouchi M, et al. Trajectories of kidney function in diabetes: a clinicopathological update. Nat Rev Nephrol. 2021;17(11):740–750. doi: 10.1038/s41581-021-00462-y.
- van Swelm RPL, Wetzels JFM, Swinkels DW. The multifaceted role of iron in renal health and disease. Nat Rev Nephrol. 2020;16(2):77–98. doi: 10.1038/s41581-019-0197-5.
- Feng X, Wang S, Sun Z, et al. Ferroptosis enhanced diabetic renal tubular injury via HIF-1α/HO-1 pathway in db/db mice. Front Endocrinol (Lausanne). 2021;12:626390. doi: 10.3389/fendo.2021.626390.
- Tsai YC, Kuo MC, Huang JC, et al. Single-cell transcriptomic profiles in the pathophysiology within the microenvironment of early diabetic kidney disease. Cell Death Dis. 2023;14(7):442. doi: 10.1038/s41419-023-05947-1.
- Kim S, Kang SW, Joo J, et al. Characterization of ferroptosis in kidney tubular cell death under diabetic conditions. Cell Death Dis. 2021;12(2):160. doi: 10.1038/s41419-021-03452-x.
- Dodson M, Castro-Portuguez R, Zhang DD. NRF2 plays a critical role in mitigating lipid peroxidation and ferroptosis. Redox Biol. 2019;23:101107. doi: 10.1016/j.redox.2019.101107.
- Li S, Zheng L, Zhang J, et al. Inhibition of ferroptosis by up-regulating Nrf2 delayed the progression of diabetic nephropathy. Free Radic Biol Med. 2021;162:435–449. doi: 10.1016/j.freeradbiomed.2020.10.323.
- Jin T, Chen C. Umbelliferone delays the progression of diabetic nephropathy by inhibiting ferroptosis through activation of the nrf-2/HO-1 pathway. Food Chem Toxicol. 2022;163:112892. doi: 10.1016/j.fct.2022.112892.
- Lu Q, Yang L, Xiao JJ, et al. Empagliflozin attenuates the renal tubular ferroptosis in diabetic kidney disease through AMPK/NRF2 pathway. Free Radic Biol Med. 2023;195:89–102. doi: 10.1016/j.freeradbiomed.2022.12.088.
- Liu Z, Nan P, Gong Y, et al. Endoplasmic reticulum stress-triggered ferroptosis via the XBP1-Hrd1-Nrf2 pathway induces EMT progression in diabetic nephropathy. Biomed Pharmacother. 2023;164:114897. doi: 10.1016/j.biopha.2023.114897.
- Wang WJ, Jiang X, Gao CC, et al. Salusin‑β participates in high glucose‑induced HK‑2 cell ferroptosis in a nrf‑2‑dependent manner. Mol Med Rep. 2021;24(3):674. doi: 10.3892/mmr.2021.12313.
- Zhang S, Li Y, Liu X, et al. Carnosine alleviates kidney tubular epithelial injury by targeting NRF2 mediated ferroptosis in diabetic nephropathy. Amino Acids. 2023;55(9):1141–1155. doi: 10.1007/s00726-023-03301-5.
- Wang YH, Chang DY, Zhao MH, et al. Glutathione peroxidase 4 is a predictor of diabetic kidney disease progression in type 2 diabetes mellitus. Oxid Med Cell Longev. 2022;2022:2948248–2948210. doi: 10.1155/2022/2948248.
- Huang J, Chen G, Wang J, et al. Platycodin D regulates high glucose-induced ferroptosis of HK-2 cells through glutathione peroxidase 4 (GPX4). Bioengineered. 2022;13(3):6627–6637. doi: 10.1080/21655979.2022.2045834.
- Li Q, Liao J, Chen W, et al. NAC alleviative ferroptosis in diabetic nephropathy via maintaining mitochondrial redox homeostasis through activating SIRT3-SOD2/Gpx4 pathway. Free Radic Biol Med. 2022;189:1–170. doi: 10.1016/j.freeradbiomed.2022.05.024.
- Song SH, Han D, Park K, et al. Bone morphogenetic protein-7 attenuates pancreatic damage under diabetic conditions and prevents progression to diabetic nephropathy via inhibition of ferroptosis. Front Endocrinol (Lausanne). 2023;14:1172199. doi: 10.3389/fendo.2023.1172199.
- Huang B, Wen W, Ye S. Dapagliflozin ameliorates renal tubular ferroptosis in diabetes via SLC40A1 stabilization. Oxid Med Cell Longev. 2022;2022:9735555–9735517. doi: 10.1155/2022/9735555.
- Wu K, Fei L, Wang X, et al. ZIP14 is involved in iron deposition and triggers ferroptosis in diabetic nephropathy. Metallomics. 2022;14(7):mfac034. doi: 10.1093/mtomcs/mfac034.
- Tan H, Chen J, Li Y, et al. Glabridin, a bioactive component of licorice, ameliorates diabetic nephropathy by regulating ferroptosis and the VEGF/akt/ERK pathways. Mol Med. 2022;28(1):58. doi: 10.1186/s10020-022-00481-w.
- Wu Z, Li D, Tian D, et al. Aspirin mediates protection from diabetic kidney disease by inducing ferroptosis inhibition. PLoS One. 2022;17(12):e0279010. doi: 10.1371/journal.pone.0279010.
- Huang D, Shen P, Wang C, et al. Calycosin plays a protective role in diabetic kidney disease through the regulation of ferroptosis. Pharm Biol. 2022;60(1):990–996. doi: 10.1080/13880209.2022.2067572.
- Wang MZ, Cai YF, Fang QJ, et al. Inhibition of ferroptosis of renal tubular cells with total flavones of abelmoschus manihot alleviates diabetic tubulopathy. Anat Rec (Hoboken). 2022;306(12):3199–3213. doi: 10.1002/ar.25123.
- Jin J, Wang Y, Zheng D, et al. A novel identified circular RNA, mmu_mmu_circRNA_0000309, involves in Germacrone-Mediated improvement of diabetic nephropathy through regulating ferroptosis by targeting miR-188-3p/GPX4 signaling axis. Antioxid Redox Signal. 2022;36(10-12):740–759. doi: 10.1089/ars.2021.0063.
- Chen J, Ou Z, Gao T, et al. Ginkgolide B alleviates oxidative stress and ferroptosis by inhibiting GPX4 ubiquitination to improve diabetic nephropathy. Biomed Pharmacother. 2022;156:113953. doi: 10.1016/j.biopha.2022.113953.
- Zhang Q, Hu Y, Hu JE, et al. Sp1-mediated upregulation of Prdx6 expression prevents podocyte injury in diabetic nephropathy via mitigation of oxidative stress and ferroptosis. Life Sci. 2021;278:119529. doi: 10.1016/j.lfs.2021.119529.
- Zhao C, Pu Z, Gao J, et al. Multiomics" analyses combined with systems pharmacology reveal the renoprotection of mangiferin monosodium salt in rats with diabetic nephropathy: focus on improvements in renal ferroptosis, renal inflammation, and podocyte insulin resistance. J Agric Food Chem. 2023;71(1):358–381. doi: 10.1021/acs.jafc.2c05595.
- Li Q, Meng X, Hua Q. Circ ASAP2 decreased inflammation and ferroptosis in diabetic nephropathy through SOX2/SLC7A11 by miR-770-5p. Acta Diabetol. 2023;60(1):29–42. doi: 10.1007/s00592-022-01961-5.
- Wang X, Li Q, Sui B, et al. Schisandrin a from schisandra chinensis attenuates ferroptosis and NLRP3 Inflammasome-Mediated pyroptosis in diabetic nephropathy through mitochondrial damage by AdipoR1 ubiquitination. Oxid Med Cell Longev. 2022;2022:5411462. doi: 10.1155/2022/5411462.
- Wu Y, Zhao Y, Yang HZ, et al. HMGB1 regulates ferroptosis through Nrf2 pathway in mesangial cells in response to high glucose. Biosci Rep. 2021;41(2):41.
- Lv S, Li H, Zhang T, et al. San-Huang-Yi-Shen capsule ameliorates diabetic nephropathy in mice through inhibiting ferroptosis. Biomed Pharmacother. 2023;165:115086. doi: 10.1016/j.biopha.2023.115086.
- Ji J, Tao P, Wang Q, et al. Emodin attenuates diabetic kidney disease by inhibiting ferroptosis via upregulating Nrf2 expression. Aging (Albany NY). 2023;15:7673–7688.
- Zhang S, Zhang S, Wang H, et al. Vitexin ameliorated diabetic nephropathy via suppressing GPX4-mediated ferroptosis. Eur J Pharmacol. 2023;951:175787. doi: 10.1016/j.ejphar.2023.175787.
- Feng Q, Yang Y, Qiao Y, et al. Quercetin ameliorates diabetic kidney injury by inhibiting ferroptosis via activating Nrf2/HO-1 signaling pathway. Am J Chin Med. 2023;51(4):997–1018. doi: 10.1142/S0192415X23500465.
- Kim M, Bae JY, Yoo S, et al. 2-Deoxy-d-ribose induces ferroptosis in renal tubular epithelial cells via ubiquitin-proteasome system-mediated xCT protein degradation. Free Radic Biol Med. 2023;208:384–393. doi: 10.1016/j.freeradbiomed.2023.08.027.