Abstract
The prevalence of atopic diseases has increased abruptly in recent years in most Westernized societies, making the question why this happened the topic of a heated debate. The best paradigm available to date to explain this steep rise, the ‘hygiene hypothesis’, supports that it is the excess ‘cleanliness’ of our environments that has led to the decline in the number of infectious stimuli that are necessary for the proper development of our immune system. Recent findings support that it is the combined effect that not only pathogenic, but also non-pathogenic microorganisms, and even their structural components, can exert on the immune system that deters from the development of atopic responses. Adding to these results are intriguing new findings on the effect different gene polymorphisms can have on an individual's predisposition to allergic diseases. The most important linkages produced, to date, include those among the genes for IL-4, IL-13, HLA-DRB, TNF, LTA, FCER1B, IL-4RA, ADAM33, TCR α/δ, PHF11, GPRA, TIM, p40, CD14, DPP10, T-bet, GATA-3, and FOXP3 and allergic disorders. The two parallel research efforts, epidemiologic and genetic, are only recently starting to converge, producing fascinating results on the effect particular gene-environment interactions might have in the development of atopy. The most important lesson learned through this tremendous research effort is that not only a small number but thousands and millions of separate risk factors act in concordance in the production of the allergic phenotype.
Introduction
Over the last 50 years the prevalence of diseases such as hay fever, atopic dermatitis, and allergic asthma has increased significantly in the developed world Citation1–3. The 2002 surveillance for asthma in the United States reported a 76% increase of the estimated annual prevalence of self-reported asthma during the period 1980–1995, its prevalence reaching an impressive 5.5% in 1995 Citation4. Allergic diseases are now epidemic in most Westernized societies, affecting approximately 20%–30% of their populations, and ranking first among the causes of chronic disease in children. Despite the fact that some recent evidence suggests a stabilizing trend of their prevalence Citation5–9, the question why these diseases have spread so rapidly remains hot.
Allergy is an immunological disorder. It is defined as a disease that follows an immune system response towards an otherwise innocuous antigen (Ag) and is usually equated with type I hypersensitivity reaction (immediate type hypersensitivity). The condition that underlies allergic diseases is atopy, which is characterized by raised immunoglobulin E (IgE) levels. Multiple pathways have been identified that can lead to the production of IgE in response to external stimuli Citation10, Citation11.
Key messages
The presence of particular gene variations modifies an individual's likelihood to develop allergic diseases.
Exposure to infections during the maturation of a child's immune response may be crucial to reduce reactivity to innocuous environmental particles.
More than any specific infection, it is the overall microbial burden early in life that seems to protect against the development of allergies.
Mechanisms of IgE production
Most allergens are small, highly soluble, proteins presented to the immune system at very low doses. They seem to induce immune responses that are very similar to those induced by helminthic infections. Several cell types detect allergens and can trigger a response against them ().
Figure 1. The three main pathways of antigen presentation and the effector mechanisms induced during the allergic reaction.
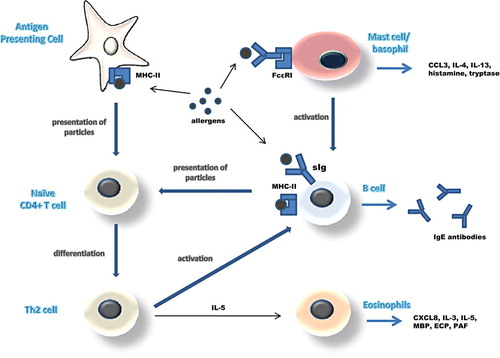
Allergens that end up in local lymph nodes are mostly taken up by dendritic cells which present them to naive CD4+ T cells through their class II major histocompatibility complexes (MHC-II). This signals CD4+ T cells to become activated and differentiate into a predominantly T helper 2 (Th2) phenotype. Th2 cells are thought to be the key organizers of the allergic response from then on. They produce interleukins IL-4 and IL-13 and express costimulatory molecules, like the ligand for the CD40 molecule (CD40L), on their surface. CD40L binds to CD40 on the surface of B cells signaling them to initiate class switching to IgE production Citation12, Citation13. Concurrently, Th2 cells produce IL-5, an activating factor that stimulates eosinophils to release their various inflammatory mediators and toxic proteins. B cells can also take up antigen through their surface immunoglobulin receptors, process it, and present it to T helper cells as peptide-MHC class II complex. This T-B cell interaction promotes the transcription and translation of the IL-4 gene in T cells and in this way the induction of IgE production from B cells Citation11, Citation12.
Both of these pathways depend on the presence of antigen and are referred to as ‘cognate’ (antigen-driven). However, non-cognate pathways also seem to occur. Mast cells and basophils express on their surface FcεRI, the high-affinity receptor for IgE. When the FcεRI-bound IgE antibodies are cross-linked by antigen, CD40L expression and IL-4 secretion are induced signaling B cells to produce IgE. Mast cells and basophils also release vasoactive mediators, chemotactic factors and cytokines which, together with the substances released by eosinophils, trigger the inflammatory cascade that ultimately leads to allergic symptomatology Citation10, Citation14.
Whatever the cell type primarily involved in the initiation of the immune response against an allergen, it seems that the activation of Th2 cells and the consequent B cell signaling are events central to the pathogenesis of atopy. What remains a mystery is why some individuals oversecrete IgE antibodies and overreact to innocuous molecules, while others do not. In order to answer this question one has to examine both the genetic and the environmental factors that can influence the expression of the allergic phenotype.
Genetic factors
Research into genetic factors contributing to an allergic phenotype in humans has proved to be quite complicated. Monozygotic twins are known to be more concordant for any type of allergy than are dizygotic twins. However, even in monozygotic twin pairs (where at least one member is allergic) concordance averages only around 50%–60% Citation15. A second confusing finding is the parent of origin effect. It has been observed that the risk of transmission of an allergic disorder from an affected mother is approximately four times higher than from an affected father Citation16. The mechanisms by which these phenomena occur remain largely unknown.
Allergy is considered to be a genetically determined complex disease, which means it does not obey the classical Mendelian laws of recessive, dominant, or codominant inheritance. The allergic clinical phenotype is not only determined by interactions between multiple major and minor genes but is also modulated by non-genetic factors, like the environment. Adding to this complexity is the fact that under the term ‘allergy’ numerous clinical entities are encompassed, most of which are not very clearly demarcated.
Nevertheless, numerous genetic and genomic studies have been performed to date, already producing a significant number of candidate susceptibility genes. The eight most cited atopy-associated genes until 2003 included the ones encoding for IL-4, IL-13, β2 adrenergic receptor (ADRB2), human leukocyte antigen DRB1 (HLA-DRB1), tumor necrosis factor (TNF), lymphotoxin-alpha (LTA), high-affinity IgE receptor (FCER1B), and the alpha chain of the IL-4 receptor (IL-4RA) Citation17. Apart from these, a number of other genes have received significant attention more recently and are also discussed below.
Allergy susceptibility genes
The MHC class II region shows strong evidence for linkage with allergy in many studies Citation18–21, as this would have been expected since HLA specificities are the ones that restrict the immune response to particular allergens. Associations between several HLA haplotypes and immune responses to specific allergens have been observed Citation22, Citation23. Haplotypes containing the HLA class II DRB1*1501 allele, for example, have been associated to responses against ragweed pollen allergens Citation24. Certain HLA-DQB1*03 alleles, on the other hand, are associated with higher levels of total serum IgE and an increased susceptibility to allergy and asthma Citation25–27 ().
Table I. Major candidate susceptibility genes for allergic diseases discovered to date.
A linkage between specific IgE responses and the T cell receptor α/δ (TCR-α/δ) locus on chromosome 14 has been proved by many researchers as well Citation28–30. It has been shown that allele 2 of the Vα8,1 polymorphism (Vα8,1*2) is associated with higher IgE titers against Der p II, a house dust mite antigenic component Citation29. Certain germ-like elements of the TCR-Va region are even thought to interact with particular HLA-DR types to produce in common a modified response to particular foreign antigens Citation29.
Another candidate for an allergy-susceptibility gene is the tumor necrosis factor gene encoding for TNF, a potent proinflammatory cytokine found in excess in asthmatic airways Citation31, Citation32. Polymorphisms in the gene encoding for TNF have been associated with variation in the expression of TNF-α, in particular, and the presence of asthma. The TNF-308 promoter allele has shown a consistent association with asthma in many studies Citation33–35.
Closely related to TNF, located on band 21 of the short (p) arm of chromosome 6 (6p21), is the gene that encodes for lymphotoxin-alpha (LTα). LTα's main function seems to be to regulate the expression of the TNF gene. As a consequence, polymorphisms in this area have also been linked to the development of asthma. The LtαNcoI polymorphism, in particular, is associated with an increased risk of asthma and bronchial hyperresponsiveness in many studies Citation33, Citation36–38.
Located on band 13 of the long (q) arm of chromosome 11 (11q13), the gene that encodes for the β chain of the high-affinity receptor for IgE (FcεRI-β) is also being associated to atopy in several trials Citation39–42. The FcεRI-β chain stabilizes the receptor's expression on the surface of mast cells and amplifies almost 7-fold its response to activation. Consequently, polymorphisms in this area modify the strength of the immune response against allergens. Allergy, asthma, and bronchial hyperresponsiveness have all been associated with certain FcεRI-β polymorphisms Citation43, Citation44.
Bands 23–35 on chromosome 5q seem to contain multiple allergy susceptibility candidate genes, including IL-4, IL-13, granulocyte-macrophage colony-stimulating factor (GM-CSF) and IL-9. Collectively these genes are known as the cytokine cluster Citation45. IL-4 gene polymorphisms are only weakly linked to asthma Citation46, Citation47, whereas a far stronger link has been established between IL-13 polymorphisms, increased serum IgE levels, asthma and atopy Citation48–51. A second set of genes found in this area is the TIM (T cell immunoglobulin and mucin domain) family genes Citation52. TIM encodes for various surface proteins expressed on CD4+ T cells. In mouse strains, TIM genes have been correlated with the production of IL-4 and IL-13 and the degree of airway hypersensitivity. In humans, certain TIM-1 allelic variants have been associated with protection from atopy, but this protection only seems to be conferred to individuals with a hepatitis A virus exposure history Citation53. This finding is of particular importance since it provides molecular evidence of the hygiene hypothesis theory (discussed in the next section).
On the same area of chromosome 5q, the CD14, ADRB2, and p40 genes are found, all of which are associated with the development of atopic disorders. CD14 encodes for a protein that forms part of the cell surface bacterial lipopolysaccharide (LPS) receptor. Polymorphisms in the CD14 promoter region have been linked to the presence of atopy in some populations Citation54, Citation55, an example being the CD14-159CC genotype which has been associated with an increased risk of early-onset atopy and airway hyperresponsiveness Citation56. The p40 gene, encoding for one of the two subunits of IL-12, has been associated to an increased susceptibility to asthma, atopic dermatitis, and psoriasis vulgaris Citation57–59. Associations between the gene encoding for the β2-adrenergic receptor (ADRB2) and variations in the airway's smooth muscle responsiveness to allergens and asthma have also been reported Citation60–64.
Alpha disintegrin and metalloproteinase-33 (ADAM33) found on chromosome 20p13 is yet another gene that has been linked to atopy. ADAM33 is expressed ubiquitously in muscle cells of every type, particularly in lung smooth muscle cells, and in bronchial fibroblasts. The proteins encoded by ADAM33 have been implicated in processes like proteolysis of extracellular matrix and extracellular communication and signaling. It has thus been suggested that the ADAM33 gene might encode for a protein that plays a role in airway remodeling by fibroblasts and in bronchial smooth muscle contraction. Mutations in the ADAM33 region have been linked to both bronchial hyperreactivity and airway remodeling in asthma Citation65–67.
The DPP10 gene on chromosome 2q14 is also linked to allergy in several studies Citation19, Citation20, Citation68–70. DPP10 encodes for a dipeptidyl peptidase, a peptidase involved in cleaving terminal dipeptides from chemokines and cytokines. Through this process dipeptidyl peptidases can regulate the activity of certain chemokines and cytokines. The exact role of DPP10 proteins is not yet entirely clear, however, some researchers supporting that these proteins are not at all linked with atopy but are in fact associated with type A K+ channels in neurons Citation71.
Associated with asthma as well, the gene for the neuropeptide S receptor 1 (NSR1), also called the G-protein-coupled receptor for asthma susceptibility (GPRA), is located on chromosome 7p15 Citation72, Citation73. Out of the two receptor isoforms encoded by this gene, NSR1-B is differentially expressed in bronchial epithelial and smooth muscle cells of asthmatic individuals compared to healthy controls, suggesting that a dysregulation in NSR1 expression could predispose to asthma Citation73–76.
Another candidate for an allergy susceptibility gene, IL-4RA gene, is encoding for the IL-4 receptor alpha chain that forms a component of, not only the IL-4, but also the IL-13 receptor Citation41, Citation77. Variations in this gene are linked with allergic inflammatory disorders, including hyper-IgE syndrome and atopic dermatitis Citation78, Citation79.
One of the most consistent linkage findings in genetic studies is the one of PHF11 gene on chromosome 13q14, asthma and atopy Citation41, Citation80–82. PHF11 is mainly expressed in the stomach, tonsils, and B lymphocytes, this finding leading to the assumption that PHF11 could be involved in the regulation of lymphocyte activation and immunoglobulin synthesis. The exact functional role of PHF11, however, still remains unclear.
Finally, the transcription factors involved in Th1 and Th2 response polarization and the development of T regulatory (Treg) cells are also thought to be associated with allergy Citation13, Citation83–85. GATA-3, the transcription factor necessary for the development of Th2 cytokine responses and T-bet, and the corresponding transcription factor for Th1 cytokine gene expression have both been implicated in the development of atopy. Since Treg cells are thought to be responsible for controlling the Th1/Th2 response balance, their respective transcription factor, FOXP3, is plausibly linked with atopy as well.
Environmental factors
The fact that the prevalence of allergic disorders has increased rapidly during the last 50 years, and that this increase was primarily experienced in the developed world, both strongly suggest the involvement of environmental factors in their pathogenesis. In an effort to identify these factors it is useful to consider the time window during which atopic diseases most frequently manifest for the first time. The greatest incidence of wheeze occurs in children under the age of 4 years, and it is during the same period that asthma starts in most patients Citation86, Citation87. It is thus logical to assume that atopic sensitization could take place, either during early childhood or, even earlier, during pregnancy. Indeed, T cell responses to common environmental allergens can be detected in almost all newborn babies Citation88.
The probable effect maternal behavior could have on the gestating embryo has been the focus of intense research lately Citation89–92. It is speculated that maternally originating allergenic peptides could be presented to the fetal immune system, either directly, passing inside the fetal circulation, or on the surface of maternal antigen-presenting cells at the maternal-fetal interface Citation88, Citation93. The purpose of this priming could supposedly be to preadapt the fetal immune system to current environmental conditions Citation94, Citation95. Alternatively, maternal exposures might influence directly the genetic profile of the embryo through a process called epigenetic inheritance Citation96, Citation97.
This early sensitization could also contribute to the Th2 cell polarization that is most usually seen in newborn babies Citation88, Citation98, Citation99. The Th2 cell predominance helps reduce the maternal immune system's reactivity against the fetal allograft Citation100; however, at the same time, it confers a predisposing effect for atopic diseases. This is probably the reason why normally, during the first 2 years of life, this Th2 polarization gradually gives way to a Th1 bias. This transition, however, does not seem to happen in atopic children, and they are found to exhibit the Th2 shift for the rest of their lives. Various theories have been proposed to explain why this happens; the most exciting of all and, at the same time, the one that has received the greatest attention is called the ‘hygiene hypothesis’.
The hygiene hypothesis
This hypothesis was formulated about 20 years ago, based on data showing that the prevalence of hay fever and eczema in children is proportional to the number of their younger and older siblings Citation101. What it essentially postulates is that infections during infancy (which purportedly occur more often in larger families) might in fact protect against allergic disorders. At a cellular level, microbial infections might be necessary in order to help shift the Th1/Th2 balance.
Following the development of the ‘hygiene hypothesis’ numerous epidemiologic studies have emerged in support of its main thesis (). Individuals that present with a positive tuberculin response Citation102 or a positive serology to hepatitis A Citation103–105 have been found to possess a lower risk of exhibiting allergic disorders. An inverse relationship between atopy, asthma and allergic rhinitis, and infections of the gastrointestinal tract caused by Helicobacter pylori or Toxoplasma gondii has also been established Citation103, Citation106–108. Measles infection early in life has also been found to be negatively associated with the development of atopy Citation109. Finally, Epstein-Barr virus (EBV), Cytomegalovirus (CMV) and herpes infections have all shown a protective effect against allergy Citation110, Citation111. All these studies essentially support the notion that exposure to pathogens, like hepatitis A virus, early in life helps in the prevention of allergy, probably through a Th1 upregulation mechanism.
Table II. Epidemiologic studies in support of the ‘hygiene hypothesis’. For a more extensive catalogue of relevant epidemiologic studies see reference Citation175.
Using a more indirect approach to support the role of infectious diseases in the prevention of allergy, it has been found that the exposure of young children to older children at home Citation112, Citation113 or to other children at day care Citation114, Citation115 can confer protection against the development of asthma and wheezing. Likewise, living in a farm environment during childhood seems to protect against the development of allergy Citation116–119. Also protective against atopy are those vaccines that process Th1-stimulating properties, like Bacille Calmette-Guérin (BCG) Citation120.
Despite the significant number of studies in support of the hygiene hypothesis, there is an equally high number of studies that has emerged with results that are contradictory to its main thesis. In contrast to other infections, respiratory viral infections early in life have been associated with an increased risk of wheeze Citation121, Citation122. Respiratory syncytial virus (RSV) infection during infancy, in particular, is strongly associated with the development of asthma and allergic sensitization Citation123, Citation124. On the other hand, the protective effect purportedly conferred by measles or hepatitis A infections has been disputed by a number of studies where either no effect or a positive association between these infections and allergy has been found Citation125–129. Children growing up in a farm environment, although having a lower prevalence of atopy, present a paradoxically high prevalence of asthma Citation130, Citation131. Controversial data have emerged on the role mycobacterial infections might have in the development of atopic diseases as well Citation132. Similarly, the protective role of BCG vaccination against atopy has been questioned by some researchers Citation133–136.
The immune regulation theory
The finding that has puzzled scientists the most, however, is that allergies can present in parallel with both helminthic infections and autoimmune diseases in the same individual. The paradox in this is that parasitism with helminths confers a protective effect against allergy, despite the fact that parasitisms are known to provoke a, predominantly, Th2 immune response Citation137–139. It thus seems that the Th1/Th2 paradigm is insufficient to explain this protective effect. In an attempt to explain this paradox and at the same time encompass the conflicting results that different epidemiologic studies have produced, under a single pathogenetic mechanism, van den Biggelaar and colleagues proposed in 2000 an intriguing theory Citation140. What they hypothesized was that parasites could be responsible of inducing the production of IL-10 from T cells that can subsequently downregulate Th2 responses and IgE production. IL-10, mainly produced by CD4+CD25+ regulatory T cell (Treg) populations, is known to be able to dampen immune responses in general. What this theory essentially suggested was that infections with either parasites or with microbial agents might boost those regulatory networks that are necessary to dampen responses as soon as the infection is controlled. These same regulatory responses could be necessary in order to avoid immune reactivity against innocuous environmental particles ().
Figure 2. Schematic representation of the immune-regulation network theory. This network is believed to be responsible for preventing the development of both autoimmune and atopic diseases.
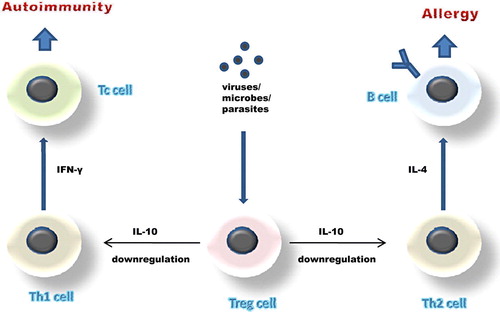
This ‘immune-regulation theory’, apart from explaining the presence of parasitic infections in atopic individuals, can also provide a satisfactory basis for the explanation of the type 1 diabetes/allergy coexistence that is seen in some patients Citation141, Citation142. This coexistence suggests that the root cause for the increase in atopic diseases could also be responsible for the recent rise in the prevalence of autoimmune disorders Citation143, despite the fact that allergy is a Th2- and autoimmune diseases a Th1-associated disease. In this case again, infections by pathogens that induce the production of IL-10 might be responsible for the downregulation of both the Th2 and the Th1 responses that are seen in allergic and autoimmune disorders, respectively. This theory further explains why it is so difficult to generate autoimmune diseases in ‘dirty’ animal colonies Citation143, Citation144.
The role of innate immunity
Based on the above framework it has been proposed more recently that, not only contact with pathogens that manifests themselves clinically, but even contact with non-pathogenic microorganisms, or merely with their components, might be enough to modulate the immune system's reactivity Citation145, Citation146. Attention has mainly focused on the effects that five particular bacterial components might be in a position to exert on the proper education of our immune system. These include the cell wall components LPS (endotoxin), teichoic acid and peptidoglycan, the bacterial DNA component CpG oligonucleotide, and the fungal and helminthic structural constituent, chitin Citation147–149.
Experimental evidence suggests that it is the innate immune system that first comes into contact with these bacterial particles, their recognition relying on pattern recognition receptors, like toll-like receptors (TLRs) and caspase recruitment domains (CARDs). These receptors are mainly found in dendritic cells, and their primary function is thought to be to detect the presence of ‘danger’ signals Citation150. Signaling through TLRs can induce the maturation of dendritic cells into cells that are able to initiate the adaptive immune response cascade. In the absence of these essential ‘danger’ signals, dendritic cells are unable to support the further maturation of responses and, instead, regulatory pathways are activated Citation151. Intermittent contact with microbial particles might, in this manner, be necessary in order to properly ‘educate’ our immune system to become tolerant to non-hazardous particles and allergens. Similarly, chronic infections, like those caused by parasites and Mycobacterium tuberculosis, could be of value to our immune system in that they can provide a constant stimulus for the production of anti-inflammatory cytokines. Indeed, repeated or continuous exposure to an antigen is one of the primary pathways that can lead to the induction of tolerance Citation152, Citation153.
Do we know which is the major source or sources of bacterial stimuli that was present in the past, and that are dramatically reduced in today's developed world? Soil, almost entirely absent from most modern societies, contains a large number of bacteria, including mycobacteria and actinomycetes. Studies performed in North Karelia, Finland and the Republic of Karelia in Russia show a large variation in the prevalence of allergic diseases between these two regions, despite their close geographical proximity Citation154, Citation155. This is attributed to the different environmental exposures that are present among these two populations, including exposure to soil and its products. Even among populations that originate from the same country, large differences in the frequency of atopic symptoms can be found. The large, cross-sectional, PARSIFAL (Prevention of Allergy Risk factors for Sensitization In children related to Farming and Anthroposophic Life-Style) study has recently addressed this particular issue, concluding that exposure to farm environment early in life protects against the development of atopic diseases Citation156–158. Both these studies show that it is not specific infections but rather what urbanization has caused to our environments in general, that has led to the explosion of allergies.
Bacterial populations are also present in large quantities inside our own gut, making them an intriguing candidate for the missing bacterial pool. The molecular ‘signatures’ these bacteria carry allow them to be recognized as weak ‘danger’ signals, thus not inducing proper immune reactions, but triggering the development of regulatory responses Citation151. The role this indigenous bacterial flora plays in the proper development of our immune system is illustrated in trials where the administration of antibiotics early in life has been found to correlate with the frequency of allergic diseases Citation159–161. Antibiotics can disrupt the physiologic gut bacterial flora, thus reducing its influence on the development of our immune system. In support of this view, the patterns of bacterial colonization during early childhood have been found to exhibit significant differences both between the developed and the developing world Citation162 and between allergic and non-allergic children Citation163–165. Reduction of the number of infections occurring early in life could be another way through which frequent antibiotic use might contribute to the development of atopy. In contrast, children from families with an anthroposophic life-style that avoids frequent antibiotic use exhibit a lower prevalence of atopy Citation156, Citation166, Citation167.
Gene-environment and gene-gene interactions
For a disease like allergy that manifests itself in so many different ways and in such diverse populations, it is only natural to assume that it is the combined effect of a constellation of different risk factors that act together in its pathogenesis. It is has recently become clear that these allergy susceptibility factors can interact with each other in many different ways in the production of the allergic phenotype.
In particular, a constantly increasing number of gene-environment interactions are being reported. The CD14-159 polymorphism that was mentioned earlier has been shown to either confer a protective or a predisposing effect to the development of asthma, depending on the levels of LPS present in the environment of the carriers Citation168, Citation169. Other studies have shown that the protective effect this polymorphism confers can only be manifested during mid-childhood, whereas later in life it is lost Citation56. Analysis of a subsample of children that participated to the PARSIFAL study, on the other hand, showed that maternal exposure to a farm environment during pregnancy is not only associated with protection from atopic sensitization, but also with an increased expression of the genes for the microbial receptors TLR2, TLR4, and CD14 in children Citation95.
Different genes have also been shown to be able to interact with each other in the production of a specific phenotypic variant. For example, the combined presence of the 590C allelic variant of the gene encoding for IL-4 together with the Arg551 allele of the IL-4RA gene significantly increases susceptibility to asthma Citation170. Similar interactions have been detected among the IL4RA-S478P and IL13-1111C/T gene polymorphisms, their common presence in an individual increasing susceptibility to asthma by as much as five times Citation171.
Future directions
Owing to the dramatic increase of atopic disorders, research effort has greatly intensified recently, leading to the discovery of fascinating new findings. Despite the tremendous progress achieved, however, large questions on the how and why allergies arise still remain to be answered.
An example is the Th1/Th2 paradigm, which, although providing a basic framework for the molecular mechanisms that might underlie the development of atopy, seems inadequate to explain many of the present experimental findings. The Th2 polarization that is reported to be found in atopic individuals, for example, is primarily detected in peripheral blood, which leaves us the question whether similar imbalances are present in other tissues as well, or whether this phenomenon is restricted there. What is even more unsatisfying about this paradigm is the fact it has been used in the past to explain the pathogenesis of a vast array of entirely different immune disorders. It thus seems more plausible to hypothesize that the Th1/Th2 paradigm must be an oversimplification of the true molecular mechanisms that underlie the development of atopic diseases.
Another area that future studies are expected to shed some light upon is the question of the primary tissue (or tissues) where the protective effect conferred by microorganisms originates. Both the respiratory and the gastrointestinal tract represent plausible candidates. Some evidence in support of the role the latter might play in the development of allergies has already been produced Citation172, although more research on this issue is still necessary.
Finally, one of the greatest issues still unsettled is the issue on the definition of atopic diseases themselves. There is as yet no single instrument to identify asthma, for example, and its diagnosis remains largely clinical. In the past different studies, both genetic and epidemiologic, have used totally different ways in order to define atopic disorders. Even more, a clear distinction on the relationship among atopy and atopic diseases is not yet available, an increasing number of studies suggesting that this relationship might not be as clear-cut as previously thought Citation139, Citation154, Citation173, Citation174. Finally, the distinction among risk factors that are responsible for the onset and those that are responsible for the exacerbation of atopic diseases has not always been obvious in the past and needs to be clarified in future studies.
References
- Beasley R, Crane J, Lai CK, Pearce N. Prevalence and etiology of asthma. J Allergy Clin Immunol. 2000; 105: S466–72
- Crater DD, Heise S, Perzanowski M, Herbert R, Morse CG, Hulsey TC, et al. Asthma hospitalization trends in Charleston, South Carolina, 1956 to 1997: twenty-fold increase among black children during a 30-year period. Pediatrics. 2001; 108: E97
- Smith JM. The prevalence of asthma and wheezing in children. Br J Dis Chest. 1976; 70: 73–7
- Mannino DM, Homa DM, Akinbami LJ, Moorman JE, Gwynn C, Redd SC. Surveillance for asthma—United States, 1980–1999. MMWR Surveill Summ. 2002; 51: 1–13
- Anderson HR, Ruggles R, Strachan DP, Austin JB, Burr M, Jeffs D, et al. Trends in prevalence of symptoms of asthma, hay fever, and eczema in 12–14 year olds in the British Isles, 1995–2002: questionnaire survey. BMJ. 2004; 328: 1052–3
- Braun-Fahrlander C, Gassner M, Grize L, Takken-Sahli K, Neu U, Stricker T, et al. No further increase in asthma, hay fever and atopic sensitisation in adolescents living in Switzerland. Eur Respir J. 2004; 23: 407–13
- Toelle BG, Ng K, Belousova E, Salome CM, Peat JK, Marks GB. Prevalence of asthma and allergy in schoolchildren in Belmont, Australia: three cross sectional surveys over 20 years. BMJ. 2004; 328: 386–7
- Asher MI, Montefort S, Bjorksten B, Lai CK, Strachan DP, Weiland SK, et al. Worldwide time trends in the prevalence of symptoms of asthma, allergic rhinoconjunctivitis, and eczema in childhood: ISAAC Phases One and Three repeat multicountry cross-sectional surveys. Lancet. 2006; 368: 733–43
- Williams H, Stewart A, von Mutius E, Cookson W, Anderson HR. Is eczema really on the increase worldwide? J Allergy Clin Immunol. 2008;121:947–54 e15.
- Janeway CA, Travers P, Walport M, Shlomchik M. Allergy and hypersensitivity6th ed. Garland Science, New YorkUSA 2005
- Benjamini E. Hypersensitivity reactions: antibody-mediated (type I) reactions4th ed. Wiley-Liss, New York, Chichester 2000
- Romagnani S. The role of lymphocytes in allergic disease. J Allergy Clin Immunol. 2000; 105: 399–408
- Akdis M, Verhagen J, Taylor A, Karamloo F, Karagiannidis C, Crameri R, et al. Immune responses in healthy and allergic individuals are characterized by a fine balance between allergen-specific T regulatory 1 and T helper 2 cells. J Exp Med. 2004; 199: 1567–75
- Piccinni MP, Maggi E, Romagnani S. Environmental factors favoring the allergen-specific Th2 response in allergic subjects. Ann N Y Acad Sci. 2000; 917: 844–52
- Barnes KC, Marsh DG. The genetics and complexity of allergy and asthma. Immunol Today. 1998; 19: 325–32
- Halonen M, Stern D, Taussig LM, Wright A, Ray CG, Martinez FD. The predictive relationship between serum IgE levels at birth and subsequent incidences of lower respiratory illnesses and eczema in infants. Am Rev Respir Dis. 1992; 146: 866–70
- Hoffjan S, Nicolae D, Ober C. Association studies for asthma and atopic diseases: a comprehensive review of the literature. Respir Res. 2003; 4: 14
- Haagerup A, Bjerke T, Schiotz PO, Binderup HG, Dahl R, Kruse TA. Asthma and atopy—a total genome scan for susceptibility genes. Allergy. 2002; 57: 680–6
- Koppelman GH, Stine OC, Xu J, Howard TD, Zheng SL, Kauffman HF, et al. Genome-wide search for atopy susceptibility genes in Dutch families with asthma. J Allergy Clin Immunol. 2002; 109: 498–506
- Wjst M, Fischer G, Immervoll T, Jung M, Saar K, Rueschendorf F, et al. A genome-wide search for linkage to asthma. German Asthma Genetics Group. Genomics. 1999; 58: 1–8
- Xu J, Meyers DA, Ober C, Blumenthal MN, Mellen B, Barnes KC, et al. Genomewide screen and identification of gene-gene interactions for asthma-susceptibility loci in three U.S. populations: collaborative study on the genetics of asthma. Am J Hum Genet. 2001; 68: 1437–46
- Marsh DG, Meyers DA, Bias WB. The epidemiology and genetics of atopic allergy. N Engl J Med. 1981; 305: 1551–9
- Young RP, Dekker JW, Wordsworth BP, Schou C, Pile KD, Matthiesen F, et al. HLA-DR and HLA-DP genotypes and immunoglobulin E responses to common major allergens. Clin Exp Allergy. 1994; 24: 431–9
- Zwollo P, Ehrlich-Kautzky E, Scharf SJ, Ansari AA, Erlich HA, Marsh DG. Sequencing of HLA-D in responders and nonresponders to short ragweed allergen, Amb a V. Immunogenetics. 1991; 33: 141–51
- Gao J, Lin Y, Qiu C, Liu Y, Ma Y. Association between HLA-DQA1, -DQB1 gene polymorphisms and susceptibility to asthma in northern Chinese subjects. Chin Med J (Engl) 2003; 116: 1078–82
- Juhn YJ, Kita H, Lee LA, Swanson RJ, Smith R, Bagniewski SM, et al. Childhood asthma and measles vaccine response. Ann Allergy Asthma Immunol. 2006; 97: 469–76
- Parapanissiou E, Papastavrou T, Deligiannidis A, Adam K, Kanakoudi F, Daniilidis M, et al. HLA antigens in Greek children with allergic bronchial asthma. Tissue Antigens. 2005; 65: 481–4
- Moffatt MF, Hill MR, Cornelis F, Schou C, Faux JA, Young RP, et al. Genetic linkage of T-cell receptor alpha/delta complex to specific IgE responses. Lancet. 1994; 343: 1597–600
- Moffatt MF, Schou C, Faux JA, Cookson WO. Germline TCR-A restriction of immunoglobulin E responses to allergen. Immunogenetics. 1997; 46: 226–30
- Cornelis F, Pile K, Loveridge J, Moss P, Harding R, Julier C, et al. Systematic study of human alpha beta T cell receptor V segments shows allelic variations resulting in a large number of distinct T cell receptor haplotypes. Eur J Immunol. 1993; 23: 1277–83
- Broide DH, Lotz M, Cuomo AJ, Coburn DA, Federman EC, Wasserman SI. Cytokines in symptomatic asthma airways. J Allergy Clin Immunol. 1992; 89: 958–67
- Munthe-Kaas MC, Carlsen KL, Carlsen KH, Egeland T, Haland G, Devulapalli CS, et al. HLA Dr-Dq haplotypes and the TNFA-308 polymorphism: associations with asthma and allergy. Allergy. 2007; 62: 991–8
- Albuquerque RV, Hayden CM, Palmer LJ, Laing IA, Rye PJ, Gibson NA, et al. Association of polymorphisms within the tumour necrosis factor (TNF) genes and childhood asthma. Clin Exp Allergy. 1998; 28: 578–84
- Winchester EC, Millwood IY, Rand L, Penny MA, Kessling AM. Association of the TNF-alpha-308 (G→A) polymorphism with self-reported history of childhood asthma. Hum Genet. 2000; 107: 591–6
- Witte JS, Palmer LJ, O'Connor RD, Hopkins PJ, Hall JM. Relation between tumour necrosis factor polymorphism TNFalpha-308 and risk of asthma. Eur J Hum Genet. 2002; 10: 82–5
- Moffatt MF, Cookson WO. Tumour necrosis factor haplotypes and asthma. Hum Mol Genet. 1997; 6: 551–4
- Trabetti E, Patuzzo C, Malerba G, Galavotti R, Martinati LC, Boner AL, et al. Association of a lymphotoxin alpha gene polymorphism and atopy in Italian families. J Med Genet. 1999; 36: 323–5
- Wang TN, Chen WY, Wang TH, Chen CJ, Huang LY, Ko YC. Gene-gene synergistic effect on atopic asthma: tumour necrosis factor-alpha-308 and lymphotoxin-alpha-NcoI in Taiwan's children. Clin Exp Allergy. 2004; 34: 184–8
- Young RP, Sharp PA, Lynch JR, Faux JA, Lathrop GM, Cookson WO, et al. Confirmation of genetic linkage between atopic IgE responses and chromosome 11q13. J Med Genet. 1992; 29: 236–8
- Cookson WO, Sharp PA, Faux JA, Hopkin JM. Linkage between immunoglobulin E responses underlying asthma and rhinitis and chromosome 11q. Lancet. 1989; 1: 1292–5
- Daniels SE, Bhattacharrya S, James A, Leaves NI, Young A, Hill MR, et al. A genome-wide search for quantitative trait loci underlying asthma. Nature. 1996; 383: 247–50
- Dizier MH, Besse-Schmittler C, Guilloud-Bataille M, Annesi-Maesano I, Boussaha M, Bousquet J, et al. Genome screen for asthma and related phenotypes in the French EGEA study. Am J Respir Crit Care Med. 2000; 162: 1812–8
- Sandford AJ, Shirakawa T, Moffatt MF, Daniels SE, Ra C, Faux JA, et al. Localisation of atopy and beta subunit of high-affinity IgE receptor (Fc epsilon RI) on chromosome 11q. Lancet. 1993; 341: 332–4
- Shirakawa T, Li A, Dubowitz M, Dekker JW, Shaw AE, Faux JA, et al. Association between atopy and variants of the beta subunit of the high-affinity immunoglobulin E receptor. Nat Genet. 1994; 7: 125–9
- Marsh DG, Neely JD, Breazeale DR, Ghosh B, Freidhoff LR, Ehrlich-Kautzky E, et al. Linkage analysis of IL4 and other chromosome 5q31.1 markers and total serum immunoglobulin E concentrations. Science. 1994; 264: 1152–6
- Rosenwasser LJ, Klemm DJ, Dresback JK, Inamura H, Mascali JJ, Klinnert M, , et al Promoter polymorphisms in the chromosome 5 gene cluster in asthma and atopy. Clin Exp Allergy. 1995;25 Suppl 2:74–8; discussion 95–6.
- Walley AJ, Cookson WO. Investigation of an interleukin-4 promoter polymorphism for associations with asthma and atopy. J Med Genet. 1996; 33: 689–92
- Graves PE, Kabesch M, Halonen M, Holberg CJ, Baldini M, Fritzsch C, et al. A cluster of seven tightly linked polymorphisms in the IL-13 gene is associated with total serum IgE levels in three populations of white children. J Allergy Clin Immunol. 2000; 105: 506–13
- Howard TD, Whittaker PA, Zaiman AL, Koppelman GH, Xu J, Hanley MT, et al. Identification and association of polymorphisms in the interleukin-13 gene with asthma and atopy in a Dutch population. Am J Respir Cell Mol Biol. 2001; 25: 377–84
- Leung TF, Tang NL, Chan IH, Li AM, Ha G, Lam CW. A polymorphism in the coding region of interleukin-13 gene is associated with atopy but not asthma in Chinese children. Clin Exp Allergy. 2001; 31: 1515–21
- Noguchi E, Nukaga-Nishio Y, Jian Z, Yokouchi Y, Kamioka M, Yamakawa-Kobayashi K, et al. Haplotypes of the 5′ region of the IL-4 gene and SNPs in the intergene sequence between the IL-4 and IL-13 genes are associated with atopic asthma. Hum Immunol. 2001; 62: 1251–7
- McIntire JJ, Umetsu SE, Akbari O, Potter M, Kuchroo VK, Barsh GS, et al. Identification of Tapr (an airway hyperreactivity regulatory locus) and the linked Tim gene family. Nat Immunol. 2001; 2: 1109–16
- McIntire JJ, Umetsu DT, DeKruyff RH. TIM-1, a novel allergy and asthma susceptibility gene. Springer Semin Immunopathol. 2004; 25: 335–48
- Baldini M, Lohman IC, Halonen M, Erickson RP, Holt PG, Martinez FD. A Polymorphism* in the 5′ flanking region of the CD14 gene is associated with circulating soluble CD14 levels and with total serum immunoglobulin E. Am J Respir Cell Mol Biol. 1999; 20: 976–83
- Koppelman GH, Reijmerink NE, Colin Stine O, Howard TD, Whittaker PA, Meyers DA, et al. Association of a promoter polymorphism of the CD14 gene and atopy. Am J Respir Crit Care Med. 2001; 163: 965–9
- O'Donnell AR, Toelle BG, Marks GB, Hayden CM, Laing IA, Peat JK, et al. Age-specific relationship between CD14 and atopy in a cohort assessed from age 8 to 25 years. Am J Respir Crit Care Med. 2004; 169: 615–22
- Morahan G, Huang D, Wu M, Holt BJ, White GP, Kendall GE, et al. Association of IL12B promoter polymorphism with severity of atopic and non-atopic asthma in children. Lancet. 2002; 360: 455–9
- Noguchi E, Yokouchi Y, Shibasaki M, Kamioka M, Yamakawa-Kobayashi K, Matsui A, et al. Identification of missense mutation in the IL12B gene: lack of association between IL12B polymorphisms and asthma and allergic rhinitis in the Japanese population. Genes Immun. 2001; 2: 401–3
- Tsunemi Y, Saeki H, Nakamura K, Sekiya T, Hirai K, Fujita H, et al. Interleukin-12 p40 gene (IL12B) 3′-untranslated region polymorphism is associated with susceptibility to atopic dermatitis and psoriasis vulgaris. J Dermatol Sci. 2002; 30: 161–6
- Hopes E, McDougall C, Christie G, Dewar J, Wheatley A, Hall IP, et al. Association of glutamine 27 polymorphism of beta 2 adrenoceptor with reported childhood asthma: population based study. BMJ. 1998; 316: 664
- Kotani Y, Nishimura Y, Maeda H, Yokoyama M. Beta2-adrenergic receptor polymorphisms affect airway responsiveness to salbutamol in asthmatics. J Asthma. 1999; 36: 583–90
- Martinez FD, Graves PE, Baldini M, Solomon S, Erickson R. Association between genetic polymorphisms of the beta2-adrenoceptor and response to albuterol in children with and without a history of wheezing. J Clin Invest. 1997; 100: 3184–8
- Reihsaus E, Innis M, MacIntyre N, Liggett SB. Mutations in the gene encoding for the beta 2-adrenergic receptor in normal and asthmatic subjects. Am J Respir Cell Mol Biol. 1993; 8: 334–9
- Turki J, Pak J, Green SA, Martin RJ, Liggett SB. Genetic polymorphisms of the beta 2-adrenergic receptor in nocturnal and nonnocturnal asthma. Evidence that Gly16 correlates with the nocturnal phenotype. J Clin Invest. 1995; 95: 1635–41
- Cakebread JA, Haitchi HM, Holloway JW, Powell RM, Keith T, Davies DE, et al. The role of ADAM33 in the pathogenesis of asthma. Springer Semin Immunopathol. 2004; 25: 361–75
- Umland SP, Garlisi CG, Shah H, Wan Y, Zou J, Devito KE, et al. Human ADAM33 messenger RNA expression profile and post-transcriptional regulation. Am J Respir Cell Mol Biol. 2003; 29: 571–82
- Van Eerdewegh P, Little RD, Dupuis J, Del Mastro RG, Falls K, Simon J, et al. Association of the ADAM33 gene with asthma and bronchial hyperresponsiveness. Nature. 2002; 418: 426–30
- Qi SY, Riviere PJ, Trojnar J, Junien JL, Akinsanya KO. Cloning and characterization of dipeptidyl peptidase 10, a new member of an emerging subgroup of serine proteases. Biochem J. 2003; 373: 179–89
- Allen M, Heinzmann A, Noguchi E, Abecasis G, Broxholme J, Ponting CP, et al. Positional cloning of a novel gene influencing asthma from chromosome 2q14. Nat Genet. 2003; 35: 258–63
- Ewart SL, Kuperman D, Schadt E, Tankersley C, Grupe A, Shubitowski DM, et al. Quantitative trait loci controlling allergen-induced airway hyperresponsiveness in inbred mice. Am J Respir Cell Mol Biol. 2000; 23: 537–45
- Nadal MS, Ozaita A, Amarillo Y, Vega-Saenz de Miera E, Ma Y, Mo W, et al. The CD26-related dipeptidyl aminopeptidase-like protein DPPX is a critical component of neuronal A-type K+ channels. Neuron. 2003; 37: 449–61
- Laitinen T, Daly MJ, Rioux JD, Kauppi P, Laprise C, Petays T, et al. A susceptibility locus for asthma-related traits on chromosome 7 revealed by genome-wide scan in a founder population. Nat Genet. 2001; 28: 87–91
- Laitinen T, Polvi A, Rydman P, Vendelin J, Pulkkinen V, Salmikangas P, et al. Characterization of a common susceptibility locus for asthma-related traits. Science. 2004; 304: 300–4
- Feng Y, Hong X, Wang L, Jiang S, Chen C, Wang B, et al. G protein-coupled receptor 154 gene polymorphism is associated with airway hyperresponsiveness to methacholine in a Chinese population. J Allergy Clin Immunol. 2006; 117: 612–7
- Melen E, Bruce S, Doekes G, Kabesch M, Laitinen T, Lauener R, et al. Haplotypes of G protein-coupled receptor 154 are associated with childhood allergy and asthma. Am J Respir Crit Care Med. 2005; 171: 1089–95
- Pulkkinen V, Haataja R, Hannelius U, Helve O, Pitkanen OM, Karikoski R, et al. G protein-coupled receptor for asthma susceptibility associates with respiratory distress syndrome. Ann Med. 2006; 38: 357–66
- Ober C, Leavitt SA, Tsalenko A, Howard TD, Hoki DM, Daniel R, et al. Variation in the interleukin 4-receptor alpha gene confers susceptibility to asthma and atopy in ethnically diverse populations. Am J Hum Genet. 2000; 66: 517–26
- Hershey GK, Friedrich MF, Esswein LA, Thomas ML, Chatila TA. The association of atopy with a gain-of-function mutation in the alpha subunit of the interleukin-4 receptor. N Engl J Med. 1997; 337: 1720–5
- Oiso N, Fukai K, Ishii M. Interleukin 4 receptor alpha chain polymorphism Gln551Arg is associated with adult atopic dermatitis in Japan. Br J Dermatol. 2000; 142: 1003–6
- Beyer K, Nickel R, Freidhoff L, Bjorksten B, Huang SK, Barnes KC, et al. Association and linkage of atopic dermatitis with chromosome 13q12-14 and 5q31-33 markers. J Invest Dermatol. 2000; 115: 906–8
- Kimura K, Noguchi E, Shibasaki M, Arinami T, Yokouchi Y, Takeda K, et al. Linkage and association of atopic asthma to markers on chromosome 13 in the Japanese population. Hum Mol Genet. 1999; 8: 1487–90
- Zhang Y, Leaves NI, Anderson GG, Ponting CP, Broxholme J, Holt R, et al. Positional cloning of a quantitative trait locus on chromosome 13q14 that influences immunoglobulin E levels and asthma. Nat Genet. 2003; 34: 181–6
- Karagiannidis C, Akdis M, Holopainen P, Woolley NJ, Hense G, Ruckert B, et al. Glucocorticoids upregulate FOXP3 expression and regulatory T cells in asthma. J Allergy Clin Immunol. 2004; 114: 1425–33
- Akdis CA, Blaser K, Akdis M. Genes of tolerance. Allergy. 2004; 59: 897–913
- Wahl SM, Vazquez N, Chen W. Regulatory T cells and transcription factors: gatekeepers in allergic inflammation. Curr Opin Immunol. 2004; 16: 768–74
- Morgan WJ, Stern DA, Sherrill DL, Guerra S, Holberg CJ, Guilbert TW, et al. Outcome of asthma and wheezing in the first 6 years of life: follow-up through adolescence. Am J Respir Crit Care Med. 2005; 172: 1253–8
- Celedon JC, Litonjua AA, Ryan L, Platts-Mills T, Weiss ST, Gold DR. Exposure to cat allergen, maternal history of asthma, and wheezing in first 5 years of life. Lancet. 2002; 360: 781–2
- Prescott SL, Macaubas C, Holt BJ, Smallacombe TB, Loh R, Sly PD, et al. Transplacental priming of the human immune system to environmental allergens: universal skewing of initial T cell responses toward the Th2 cytokine profile. J Immunol. 1998; 160: 4730–7
- Kihlstrom A, Lilja G, Pershagen G, Hedlin G. Exposure to high doses of birch pollen during pregnancy, and risk of sensitization and atopic disease in the child. Allergy. 2003; 58: 871–7
- McKeever TM, Lewis SA, Smith C, Hubbard R. The importance of prenatal exposures on the development of allergic disease: a birth cohort study using the West Midlands General Practice Database. Am J Respir Crit Care Med. 2002; 166: 827–32
- Schonberger HJ, Dompeling E, Knottnerus JA, Kuiper S, van Weel C, Schayck CP. Prenatal exposure to mite and pet allergens and total serum IgE at birth in high-risk children. Pediatr Allergy Immunol. 2005; 16: 27–31
- Shaheen SO, Newson RB, Henderson AJ, Headley JE, Stratton FD, Jones RW, et al. Prenatal paracetamol exposure and risk of asthma and elevated immunoglobulin E in childhood. Clin Exp Allergy. 2005; 35: 18–25
- Holloway JA, Warner JO, Vance GH, Diaper ND, Warner JA, Jones CA. Detection of house-dust-mite allergen in amniotic fluid and umbilical-cord blood. Lancet. 2000; 356: 1900–2
- Martinez FD, Holt PG. Role of microbial burden in aetiology of allergy and asthma. Lancet. 1999; 354 Suppl 2: SII12–5
- Ege MJ, Bieli C, Frei R, van Strien RT, Riedler J, Ublagger E, et al. Prenatal farm exposure is related to the expression of receptors of the innate immunity and to atopic sensitization in school-age children. J Allergy Clin Immunol. 2006; 117: 817–23
- Vercelli D. Genetics, epigenetics, and the environment: switching, buffering, releasing. J Allergy Clin Immunol. 2004;113:381–6; quiz 387.
- Warner JO. The early life origins of asthma and related allergic disorders. Arch Dis Child. 2004; 89: 97–102
- Barrios C, Brawand P, Berney M, Brandt C, Lambert PH, Siegrist CA. Neonatal and early life immune responses to various forms of vaccine antigens qualitatively differ from adult responses: predominance of a Th2-biased pattern which persists after adult boosting. Eur J Immunol. 1996; 26: 1489–96
- Piccinni MP, Giudizi MG, Biagiotti R, Beloni L, Giannarini L, Sampognaro S, et al. Progesterone favors the development of human T helper cells producing Th2-type cytokines and promotes both IL-4 production and membrane CD30 expression in established Th1 cell clones. J Immunol. 1995; 155: 128–33
- Wegmann TG, Lin H, Guilbert L, Mosmann TR. Bidirectional cytokine interactions in the maternal-fetal relationship: is successful pregnancy a TH2 phenomenon?. Immunol Today. 1993; 14: 353–6
- Strachan DP. Hay fever, hygiene, and household size. BMJ. 1989; 299: 1259–60
- Shirakawa T, Enomoto T, Shimazu S, Hopkin JM. The inverse association between tuberculin responses and atopic disorder. Science. 1997; 275: 77–9
- Linneberg A, Ostergaard C, Tvede M, Andersen LP, Nielsen NH, Madsen F, et al. IgG antibodies against microorganisms and atopic disease in Danish adults: the Copenhagen Allergy Study. J Allergy Clin Immunol. 2003; 111: 847–53
- Matricardi PM, Rosmini F, Ferrigno L, Nisini R, Rapicetta M, Chionne P, et al. Cross sectional retrospective study of prevalence of atopy among Italian military students with antibodies against hepatitis A virus. BMJ. 1997; 314: 999–1003
- Matricardi PM, Rosmini F, Panetta V, Ferrigno L, Bonini S. Hay fever and asthma in relation to markers of infection in the United States. J Allergy Clin Immunol. 2002; 110: 381–7
- Kosunen TU, Hook-Nikanne J, Salomaa A, Sarna S, Aromaa A, Haahtela T. Increase of allergen-specific immunoglobulin E antibodies from 1973 to 1994 in a Finnish population and a possible relationship to Helicobacter pylori infections. Clin Exp Allergy. 2002; 32: 373–8
- Matricardi PM, Rosmini F, Riondino S, Fortini M, Ferrigno L, Rapicetta M, et al. Exposure to foodborne and orofecal microbes versus airborne viruses in relation to atopy and allergic asthma: epidemiological study. BMJ. 2000; 320: 412–7
- McCune A, Lane A, Murray L, Harvey I, Nair P, Donovan J, et al. Reduced risk of atopic disorders in adults with Helicobacter pylori infection. Eur J Gastroenterol Hepatol. 2003; 15: 637–40
- Shaheen SO, Aaby P, Hall AJ, Barker DJ, Heyes CB, Shiell AW, et al. Measles and atopy in Guinea-Bissau. Lancet. 1996; 347: 1792–6
- Nilsson C, Linde A, Montgomery SM, Gustafsson L, Nasman P, Blomberg MT, et al. Does early EBV infection protect against IgE sensitization?. J Allergy Clin Immunol. 2005; 116: 438–44
- Janson C, Asbjornsdottir H, Birgisdottir A, Sigurjonsdottir RB, Gunnbjornsdottir M, Gislason D, et al. The effect of infectious burden on the prevalence of atopy and respiratory allergies in Iceland, Estonia, and Sweden. J Allergy Clin Immunol. 2007; 120: 673–9
- von Mutius E, Martinez FD, Fritzsch C, Nicolai T, Reitmeir P, Thiemann HH. Skin test reactivity and number of siblings. BMJ. 1994; 308: 692–5
- Cardoso MR, Cousens SN, de Goes Siqueira LF, Alves FM, D'Angelo LA. Crowding: risk factor or protective factor for lower respiratory disease in young children?. BMC Public Health. 2004; 4: 19
- Ball TM, Castro-Rodriguez JA, Griffith KA, Holberg CJ, Martinez FD, Wright AL. Siblings, day-care attendance, and the risk of asthma and wheezing during childhood. N Engl J Med. 2000; 343: 538–43
- Celedon JC, Wright RJ, Litonjua AA, Sredl D, Ryan L, Weiss ST, et al. Day care attendance in early life, maternal history of asthma, and asthma at the age of 6 years. Am J Respir Crit Care Med. 2003; 167: 1239–43
- Eduard W, Douwes J, Omenaas E, Heederik D. Do farming exposures cause or prevent asthma? Results from a study of adult Norwegian farmers. Thorax. 2004; 59: 381–6
- Elliott L, Yeatts K, Loomis D. Ecological associations between asthma prevalence and potential exposure to farming. Eur Respir J. 2004; 24: 938–41
- Ernst P, Cormier Y. Relative scarcity of asthma and atopy among rural adolescents raised on a farm. Am J Respir Crit Care Med. 2000; 161: 1563–6
- Portengen L, Sigsgaard T, Omland O, Hjort C, Heederik D, Doekes G. Low prevalence of atopy in young Danish farmers and farming students born and raised on a farm. Clin Exp Allergy. 2002; 32: 247–53
- Erb KJ, Holloway JW, Sobeck A, Moll H, Le Gros G. Infection of mice with Mycobacterium bovis-Bacillus Calmette-Guerin (BCG) suppresses allergen-induced airway eosinophilia. J Exp Med. 1998; 187: 561–9
- Rusconi F, Galassi C, Corbo GM, Forastiere F, Biggeri A, Ciccone G, et al. Risk factors for early, persistent, and late-onset wheezing in young children. SIDRIA Collaborative Group. Am J Respir Crit Care Med. 1999; 160: 1617–22
- Mallia P, Johnston SL. Respiratory viruses: do they protect from or induce asthma?. Allergy. 2002; 57: 1118–29
- Illi S, von Mutius E, Lau S, Bergmann R, Niggemann B, Sommerfeld C, et al. Early childhood infectious diseases and the development of asthma up to school age: a birth cohort study. BMJ. 2001; 322: 390–5
- Sigurs N, Bjarnason R, Sigurbergsson F, Kjellman B, Bjorksten B. Asthma and immunoglobulin E antibodies after respiratory syncytial virus bronchiolitis: a prospective cohort study with matched controls. Pediatrics. 1995; 95: 500–5
- Lewis SA, Britton JR. Measles infection, measles vaccination and the effect of birth order in the aetiology of hay fever. Clin Exp Allergy. 1998; 28: 1493–500
- Paunio M, Heinonen OP, Virtanen M, Leinikki P, Patja A, Peltola H. Measles history and atopic diseases: a population-based cross-sectional study. JAMA. 2000; 283: 343–6
- Bodner C, Anderson WJ, Reid TS, Godden DJ. Childhood exposure to infection and risk of adult onset wheeze and atopy. Thorax. 2000; 55: 383–7
- Cullinan P, Harris JM, Newman Taylor AJ, Jones M, Taylor P, Dave JR, et al. Can early infection explain the sibling effect in adult atopy?. Eur Respir J. 2003; 22: 956–61
- Jarvis D, Luczynska C, Chinn S, Burney P. The association of hepatitis A and Helicobacter pylori with sensitization to common allergens, asthma and hay fever in a population of young British adults. Allergy. 2004; 59: 1063–7
- Chai SK, Nga NN, Checkoway H, Takaro TK, Redding GJ, Keifer MC, et al. Comparison of local risk factors for children's atopic symptoms in Hanoi, Vietnam. Allergy. 2004; 59: 637–44
- Merchant JA, Naleway AL, Svendsen ER, Kelly KM, Burmeister LF, Stromquist AM, et al. Asthma and farm exposures in a cohort of rural Iowa children. Environ Health Perspect. 2005; 113: 350–6
- Obihara CC, Bollen CW, Beyers N, Kimpen JL. Mycobacterial infection and atopy in childhood: a systematic review. Pediatr Allergy Immunol. 2007; 18: 551–9
- Alm JS, Lilja G, Pershagen G, Scheynius A. Early BCG vaccination and development of atopy. Lancet. 1997; 350: 400–3
- Annus T, Montgomery SM, Riikjarv MA, Bjorksten B. Atopic disorders among Estonian schoolchildren in relation to tuberculin reactivity and the age at BCG vaccination. Allergy. 2004; 59: 1068–73
- Gruber C, Meinlschmidt G, Bergmann R, Wahn U, Stark K. Is early BCG vaccination associated with less atopic disease? An epidemiological study in German preschool children with different ethnic backgrounds. Pediatr Allergy Immunol. 2002; 13: 177–81
- Krause TG, Hviid A, Koch A, Friborg J, Hjuler T, Wohlfahrt J, et al. BCG vaccination and risk of atopy. JAMA. 2003; 289: 1012–5
- Lynch NR, Hagel I, Perez M, Di Prisco MC, Lopez R, Alvarez N. Effect of anthelmintic treatment on the allergic reactivity of children in a tropical slum. J Allergy Clin Immunol. 1993; 92: 404–11
- Cooper PJ, Chico ME, Rodrigues LC, Ordonez M, Strachan D, Griffin GE, et al. Reduced risk of atopy among school-age children infected with geohelminth parasites in a rural area of the tropics. J Allergy Clin Immunol. 2003; 111: 995–1000
- Yemaneberhan H, Bekele Z, Venn A, Lewis S, Parry E, Britton J. Prevalence of wheeze and asthma and relation to atopy in urban and rural Ethiopia. Lancet. 1997; 350: 85–90
- van den Biggelaar AH, van Ree R, Rodrigues LC, Lell B, Deelder AM, Kremsner PG, et al. Decreased atopy in children infected with Schistosoma haematobium: a role for parasite-induced interleukin-10. Lancet. 2000; 356: 1723–7
- Kero J, Gissler M, Hemminki E, Isolauri E. Could TH1 and TH2 diseases coexist? Evaluation of asthma incidence in children with coeliac disease, type 1 diabetes, or rheumatoid arthritis: a register study. J Allergy Clin Immunol. 2001; 108: 781–3
- Simpson CR, Anderson WJ, Helms PJ, Taylor MW, Watson L, Prescott GJ, et al. Coincidence of immune-mediated diseases driven by Th1 and Th2 subsets suggests a common aetiology. A population-based study using computerized general practice data. Clin Exp Allergy. 2002; 32: 37–42
- Bach JF. The effect of infections on susceptibility to autoimmune and allergic diseases. N Engl J Med. 2002; 347: 911–20
- Pozzilli P, Signore A, Williams AJ, Beales PE. NOD mouse colonies around the world—recent facts and figures. Immunol Today. 1993; 14: 193–6
- Eder W, Ege MJ, von Mutius E. The asthma epidemic. N Engl J Med. 2006; 355: 2226–35
- von Hertzen L, Haahtela T. Disconnection of man and the soil: reason for the asthma and atopy epidemic?. J Allergy Clin Immunol. 2006; 117: 334–44
- Reese TA, Liang HE, Tager AM, Luster AD, Van Rooijen N, Voehringer D, et al. Chitin induces accumulation in tissue of innate immune cells associated with allergy. Nature. 2007; 447: 92–6
- Braun-Fahrlander C, Riedler J, Herz U, Eder W, Waser M, Grize L, et al. Environmental exposure to endotoxin and its relation to asthma in school-age children. N Engl J Med. 2002; 347: 869–77
- Schaub B, Campo M, He H, Perkins D, Gillman MW, Gold DR, et al. Neonatal immune responses to TLR2 stimulation: influence of maternal atopy on Foxp3 and IL-10 expression. Respir Res. 2006; 7: 40
- Matzinger P. The danger model: a renewed sense of self. Science. 2002; 296: 301–5
- Gale EA. A missing link in the hygiene hypothesis?. Diabetologia. 2002; 45: 588–94
- Hall G, Houghton CG, Rahbek JU, Lamb JR, Jarman ER. Suppression of allergen reactive Th2 mediated responses and pulmonary eosinophilia by intranasal administration of an immunodominant peptide is linked to IL-10 production. Vaccine. 2003; 21: 549–61
- Takabayashi K, Libet L, Chisholm D, Zubeldia J, Horner AA. Intranasal immunotherapy is more effective than intradermal immunotherapy for the induction of airway allergen tolerance in Th2-sensitized mice. J Immunol. 2003; 170: 3898–905
- Pekkarinen PT, von Hertzen L, Laatikainen T, Makela MJ, Jousilahti P, Kosunen TU, et al. A disparity in the association of asthma, rhinitis, and eczema with allergen-specific IgE between Finnish and Russian Karelia. Allergy. 2007; 62: 281–7
- Vartiainen E, Petays T, Haahtela T, Jousilahti P, Pekkanen J. Allergic diseases, skin prick test responses, and IgE levels in North Karelia, Finland, and the Republic of Karelia, Russia. J Allergy Clin Immunol. 2002; 109: 643–8
- Alfven T, Braun-Fahrlander C, Brunekreef B, von Mutius E, Riedler J, Scheynius A, et al. Allergic diseases and atopic sensitization in children related to farming and anthroposophic lifestyle—the PARSIFAL study. Allergy. 2006; 61: 414–21
- Ege MJ, Frei R, Bieli C, Schram-Bijkerk D, Waser M, Benz MR, et al. Not all farming environments protect against the development of asthma and wheeze in children. J Allergy Clin Immunol. 2007; 119: 1140–7
- Karadag B, Ege MJ, Scheynius A, Waser M, Schram-Bijkerk D, van Hage M, et al. Environmental determinants of atopic eczema phenotypes in relation to asthma and atopic sensitization. Allergy. 2007; 62: 1387–93
- Farooqi IS, Hopkin JM. Early childhood infection and atopic disorder. Thorax. 1998; 53: 927–32
- Wickens K, Pearce N, Crane J, Beasley R. Antibiotic use in early childhood and the development of asthma. Clin Exp Allergy. 1999; 29: 766–71
- Cohet C, Cheng S, MacDonald C, Baker M, Foliaki S, Huntington N, et al. Infections, medication use, and the prevalence of symptoms of asthma, rhinitis, and eczema in childhood. J Epidemiol Community Health. 2004; 58: 852–7
- Adlerberth I, Carlsson B, de Man P, Jalil F, Khan SR, Larsson P, et al. Intestinal colonization with Enterobacteriaceae in Pakistani and Swedish hospital-delivered infants. Acta Paediatr Scand. 1991; 80: 602–10
- Bjorksten B, Naaber P, Sepp E, Mikelsaar M. The intestinal microflora in allergic Estonian and Swedish 2-year-old children. Clin Exp Allergy. 1999; 29: 342–6
- Bottcher MF, Nordin EK, Sandin A, Midtvedt T, Bjorksten B. Microflora-associated characteristics in faeces from allergic and nonallergic infants. Clin Exp Allergy. 2000; 30: 1590–6
- Kalliomaki M, Kirjavainen P, Eerola E, Kero P, Salminen S, Isolauri E. Distinct patterns of neonatal gut microflora in infants in whom atopy was and was not developing. J Allergy Clin Immunol. 2001; 107: 129–34
- Alm JS, Swartz J, Lilja G, Scheynius A, Pershagen G. Atopy in children of families with an anthroposophic lifestyle. Lancet. 1999; 353: 1485–8
- Floistrup H, Swartz J, Bergstrom A, Alm JS, Scheynius A, van Hage M, et al. Allergic disease and sensitization in Steiner school children. J Allergy Clin Immunol. 2006; 117: 59–66
- Eder W, Klimecki W, Yu L, von Mutius E, Riedler J, Braun-Fahrlander C, et al. Opposite effects of CD 14/-260 on serum IgE levels in children raised in different environments. J Allergy Clin Immunol. 2005; 116: 601–7
- Zambelli-Weiner A, Ehrlich E, Stockton ML, Grant AV, Zhang S, Levett PN, et al. Evaluation of the CD14/-260 polymorphism and house dust endotoxin exposure in the Barbados Asthma Genetics Study. J Allergy Clin Immunol. 2005; 115: 1203–9
- Lee SG, Kim BS, Kim JH, Lee SY, Choi SO, Shim JY, et al. Gene-gene interaction between interleukin-4 and interleukin-4 receptor alpha in Korean children with asthma. Clin Exp Allergy. 2004; 34: 1202–8
- Howard TD, Koppelman GH, Xu J, Zheng SL, Postma DS, Meyers DA, et al. Gene-gene interaction in asthma: IL4RA and IL13 in a Dutch population with asthma. Am J Hum Genet. 2002; 70: 230–6
- Wilson AF, Novey HS, Berke RA, Surprenant EL. Deposition of inhaled pollen and pollen extract in human airways. N Engl J Med. 1973; 288: 1056–8
- Leung R, Ho P. Asthma, allergy, and atopy in three south-east Asian populations. Thorax. 1994; 49: 1205–10
- von Mutius E, Weiland SK, Fritzsch C, Duhme H, Keil U. Increasing prevalence of hay fever and atopy among children in Leipzig, East Germany. Lancet. 1998; 351: 862–6
- Bloomfield SF, Stanwell-Smith R, Crevel RW, Pickup J. Too clean, or not too clean: the hygiene hypothesis and home hygiene. Clin Exp Allergy. 2006; 36: 402–25