Abstract
Introduction. Currently the only treatment for celiac disease is a lifelong gluten-free diet. The diet is, however, often burdensome, and thus new treatment options are warranted. We isolated proteases from germinating wheat grain naturally meant for total digestion of wheat storage proteins and investigated whether these enzymes can diminish toxic effects of gluten in vitro and ex vivo.
Methods. Pepsin and trypsin digested (PT) gliadin was pretreated with proteases from germinating wheat, whereafter the degradation was analyzed by HPLC-MS (high-performance liquid chromatography and mass spectroscopy) and sodium dodecyl sulphate polyacrylamide gel electrophoresis. The toxicity of cleaved PT-gliadin products was assessed in Caco-2 epithelial cells, celiac patient-derived T cells, and in human small intestinal mucosal organ culture biopsies.
Results. Proteases from germinating wheat degraded gliadin into small peptide fragments, which, unlike unprocessed PT-gliadin, did not increase epithelial permeability, induce cytoskeletal rearrangement or changes in ZO-1 expression in Caco-2 cells. Pretreated gliadin did not stimulate T cell proliferation in vitro or enhance the production of autoantibodies to culture supernatants and the activation of CD25+ lymphocytes in the organ culture to the same extent as unprocessed PT-gliadin.
Discussion. Germinating wheat enzymes reduce the toxicity of wheat gliadin in vitro and ex vivo. Further studies are justified to develop an alternative therapy for celiac disease.
Introduction
Celiac disease is a genetically determined intestinal disorder induced by storage proteins of wheat (gliadin), rye (secalin), and barley (hordein). The disease is fairly common, affecting approximately 1% of the population in the US and in Europe Citation[1]. Ingestion of the above-mentioned cereal proteins provokes variable symptoms in susceptible subjects and induces small-bowel mucosal damage characterized by flattening of villi, crypt hyperplasia, and substantial inflammation. Gliadin, secalin, and hordein are rich in proline and glutamine residues and thus only poorly cleaved by digestive proteolytic enzymes even in healthy subjects Citation[2–4]. Incomplete degradation of these dietary proteins in the gastrointestinal tract leads to the appearance of peptides, of which some are toxic Citation[5], Citation[6] and others immunogenic Citation[3], Citation[7] for celiac disease patients. Recent research has revealed that the so-called toxic gliadin peptides act as the trigger to an innate immune response Citation[8]. Of the immunogenic gliadin peptides at least the 33-mer peptide is an excellent substrate for the enzyme transglutaminase 2 (TG2), a key player in the pathogenesis of celiac disease Citation[3]. TG2 is known to deamidate the immunogenic gliadin peptides, increasing their affinity to human leucocyte antigen (HLA) DQ2 or DQ8 molecules and thus activating the T cell-mediated mucosal immune response which eventually leads to small-intestinal mucosal damage, nutrient malabsorption, and appearance of clinical symptoms Citation[9], Citation[10]. Interestingly, Lammers and colleagues recently showed that gliadin binds to a chemokine receptor CXCR3 in small-intestinal epithelial cells Citation[11]. Since the gliadin peptides remain fairly intact when reaching the small-intestinal mucosa, they are recognized by this receptor, this leading to increased intestinal epithelial permeability. Hence full cleavage of gliadin peptides in the gut lumen before binding to intestinal epithelial cells would be extremely important in blocking the activation of deleterious downstream immune responses in the small-bowel mucosa.
Key messages
We introduce a novel strategy for gluten degradation using the entire range of enzymes from germinating wheat grain, naturally meant for total digestion of wheat storage proteins.
The toxicity of PT-gliadin was markedly diminished both in vitro in Caco-2 epithelial cells, celiac patient derived T cells, and ex vivo in celiac disease patients’ small-bowel mucosal organ culture biopsies after pretreatment with germinating wheat enzymes.
Gluten detoxification by germinating wheat enzymes offers a promising alternative means of treating celiac disease in the future.
Currently the only treatment for celiac disease is a lifelong gluten-free diet, excluding all food products containing wheat, rye, and barley. However, due to the particularly restricted nature of the diet, the high cost, and often poor availability of gluten-free foods, compliance with the diet easily remains inadequate Citation[12]. Furthermore, insufficient labeling of gluten-containing foods together with contamination of supposedly gluten-free products may expose celiac patients to disease-inducing dietary antigens. Such exposure might be significant enough to maintain an on-going small-bowel mucosal damage. As the success of the strict gluten-free diet cannot be guaranteed, new treatment options are clearly desirable.
Abbreviations
Several lines of research aiming at the development of novel treatments are already under way. These include preventing gliadin presentation to T cells by blocking the binding of gluten epitopes to HLA-DQ2 molecules Citation[13], downregulation of immune response by antibodies against proinflammatory cytokines Citation[14–16], and promotion of tolerance by other immunomodulatory strategies Citation[17], Citation[18]. In addition, re-establishment of the intestinal epithelial barrier against gluten entry Citation[19], Citation[20] and inhibition of the enzymatic activity of TG2 Citation[21], Citation[22] are being assessed as potential strategies. Based on the knowledge that prolyl endopeptidases (PEP) of certain bacteria, such as Flavobacterium meningosepticumCitation[23], Citation[24], Sphingomonas capsulata, and Myxococcus xanthusCitation[25], Citation[26], as well as the fungi Aspergillus nigerCitation[27], Citation[28] are able to cleave gliadin in vitro, the notion of oral enzyme therapy promoting complete digestion of cereal proteins has emerged Citation[3]. Alone most of these enzymes might in fact prove rather ineffective Citation[23], at least in vivo; in combination with glutamine-specific endoprotease 2 (EP-B2) from barley, however, results have been more promising in destroying immunotoxic gluten epitopes Citation[29]. Interestingly, EP-B2 is one of the barley seed proteases responsible for the degradation of endosperm storage proteins during germination, providing nitrogenous nutrients to support the growth of young seedlings. In addition to EP-B2, a wide variety of endogenous seed proteases in cereals take part in this degradation process Citation[30].
In this study we hypothesized that the full range of enzymes isolated from germinating wheat would be most efficient in detoxifying dietary gluten and could thus be suitable as enzyme supplement therapy in the treatment of celiac disease. The harmfulness of such enzymatically digested gliadin was assessed by several in vitro assays and ex vivo in an organ culture system applied to small-bowel mucosal biopsy samples derived from celiac disease patients.
Materials and methods
Preparation of pepsin and trypsin digested (PT) gliadin and bovine serum albumin (BSA)
Gliadin was extracted from wheat flour (Raisio Oyj, Raisio, Finland) and digested using pepsin and trypsin as previously described Citation[31]. A PT-digest of BSA (Sigma-Aldrich, Seelze, Germany) served as control to distinguish the effects exerted by gliadin itself and possible minor amounts of pepsin and trypsin in the gliadin digests.
Isolation of proteases from germinating wheat and pretreatment of gliadin
Wheat grains were germinated in pilot malting equipment (Joe White Malting System, Melbourne, Australia). The grains were first steeped at 14°C for 48 h (8 h wet, 16 h air, 8 h wet, 16 h air) and then germinated for 8 days at +14°C. Germinated grains were frozen and homogenized in 4×vol of 50 mM Na-acetate buffer, pH 5.0, with Polytron PT1200 homogenizator. Water-soluble proteins including proteases were extracted by incubating the homogenate for 1 h at +4°C under constant shaking and then centrifuged (10,000 g, 20 min, +4°C). The supernatant was used as enzyme source. The gliadin-hydrolyzing activity of water-soluble germinated wheat protein was studied by preincubating gliadin (6 mg/mL) with germinated wheat protein (0.3 mg/mL) for 24 h at +37°C in 50 mM Na-acetate buffer, pH 4.0. Thereafter PT digestion of wheat enzyme-treated gliadin was carried out as with PT-gliadin described above.
High-performance liquid chromatography and mass spectroscopy (HPLC-MS) of PT-gliadin and enzymatically pretreated PT-gliadin
The HPLC-MS data were recorded using an LTQ quadrupole ion trap mass spectrometer connected to a Surveyor MS HPLC system (Finnigan, San Jose, CA, USA). An XTerra C-8 column (20 mm×2.1 mm, 3.5 µm particle) was used to separate the compounds. The gradient was 2%–64% acetonitrile over 46 min; the eluent was rendered acidic using 0.1% formic acid. The flow rate was 150 µL/min. The spray needle was set to at 4 kV, and the spray was stabilized by a nitrogen sheath flow. The inlet capillary temperature was 250°C. The protein hydrolysis products were identified by their molecular mass and MS/MS fragmentation, and by retention times of standard α-gliadin peptides 12-mer (QLQPFPQPQLPY, New England Peptide, Fitchburg, MA, USA) and 33-mer (LQLQPFPQPQLPYPQPQLPYPQPQLPYPQPQPF, New England Peptide). The MS/MS spectra were measured using helium as collision gas, and the collision energy was set at 30% (arbitrary units).
Sodium dodecyl sulphate polyacrylamide gel electrophoresis (SDS-PAGE) of enzymatically pretreated gliadin samples
Gliadin (6 mg/mL) was incubated with or without germinating wheat enzymes (0.3 mg/mL) for 24 h at +37°C in 50 mM Na-acetate buffer, pH 4.0. The reaction was then stopped by heating (>95°C, 10 min), whereafter 2 µL samples were subjected to standard SDS-PAGE analysis on 12% gel.
Cell cultures
Caco-2 cells (passage 25–81, American Type Culture Collection, HTB-37, Rockville, MD, USA) were maintained in Minimum Essential Medium (GIBCO Invitrogen, Paisley, UK) supplemented with 20% fetal bovine serum (FBS) (GIBCO Invitrogen), 50 U/mL penicillin-streptomycin (GIBCO Invitrogen), 1 mM sodium pyruvate (Sigma-Aldrich), 1.5 g/L sodium bicarbonate (GIBCO Invitrogen), and 0.1 mM non-essential amino acids (GIBCO Invitrogen). The cells were grown at 37°C in a 5% CO2 atmosphere and passaged twice a week upon reaching 80% confluence.
Barrier function measurements
Caco-2 cells were plated on Millicell Culture Plate Inserts (Millipore Corporate, Billerica, MA, USA), and culture medium was changed every 3 days. Transepithelial electrical resistance (TER) was measured using a Millicell-ERS volt-ohm meter (Millipore Corporate). Once resistance reached 1,000 ohms*cm2, indicating confluency of the cell layer, the cells were starved overnight in a medium containing 1% FBS, non-essential amino acids, sodium pyruvate, sodium bicarbonate, and penicillin. After starvation, the cells were exposed to 1 mg/mL of PT-BSA, PT-gliadin, or enzymatically pretreated PT-gliadin. TER was measured immediately after change of media as well as after 0.5, 1, 2, 3, 4, 5, and 6 h. Values were expressed as ohms*cm2, taking into account the surface area of the membrane (0.6 mm). The experiments were performed in duplicate at least three times independently.
Immunofluorescence stainings of Caco-2 epithelial cells
To evaluate cytoskeletal actin rearrangement Citation[32] Caco-2 cells were plated onto eight-chamber glass slides (BD Biosciences, Erembodegem, Belgium) and cultured as previously described Citation[31]. After 3–5 days of culture the cells were starved as described above and thereafter cultured for 24 h in the presence of PT-BSA, PT-gliadin, or enzymatically pretreated PT-gliadin. To visualize membrane ruffle formation the cells were stained for intracellular F actin with phalloidin-fluorescein isothiocyanate (1:300, Sigma-Aldrich). The extent of actin cytoskeleton rearrangement, evinced as membrane ruffling, was quantified by measuring the cellular edge covered by membrane ruffles as a percentage of the total length of the cell cluster. The measurements were made with analySIS software (Olympus Soft Imaging System GmbH, Munster, Germany) from at least 60 different cell clusters from each sample group in 6 independent experiments.
The pattern of tight junction-associated protein ZO-1 expression was studied in confluent Caco-2 cell layers, cultured on Transwell polyester membrane inserts (Millipore Corporate) as previously described Citation[31]. Prior to staining with monoclonal mouse anti-ZO-1 antibody (1:100, ZYMED, San Francisco, CA, USA) the cultures were starved and supplemented for 24 h with PT-BSA, PT-gliadin, or enzymatically treated PT-gliadin. Cells were visualized with an Olympus BX60 fluorescence microscope (Olympus, Hamburg, Germany). Samples were analyzed blindly by two independent observers.
T cell proliferation assay
Gliadin-reactive CD4 + T cells cultured in vitro from celiac disease patients’ small-intestinal mucosal biopsies proliferate upon recognition of gliadin epitopes presented on HLA-DQ2 in a dose-dependent manner. A majority of these T cells are reactive to gliadin antigen only after specific deamidation mediated by the enzyme TG2 Citation[33]. PT-gliadin was tested in in vitro T cell proliferation assay with and without TG2 treatment. For TG2 treatment, lyophilized PT-gliadin or PT-BSA were dissolved in 100 mM Tris-HCl pH 7.4 buffer supplemented with 5 mM Ca, adjusted to 1 mg/mL and incubated with 100 µg/mL recombinant human TG2 at 37°C for 2 h. A HLA-DQ2 homozygous Epstein-Barr virus (EBV)-transformed B lymphoblastoid cell line derived from a celiac disease patient (CD114) was used as antigen-presenting cells. Fifty thousand B cells irradiated with 100 Gy were co-incubated overnight at 37°C in a 96-well plate with various concentrations of PT-gliadin antigen in RPMI/10% human serum. Forty thousand gluten-specific T cells derived and expanded from intestinal biopsies of celiac disease patients were added to each well on day 1. The following T cell preparations were used: The T cell clone (TCC) 436.5.6 is specific for the DQ2-α-II epitope (9-mer core region PQPELPYPQ; part of the 33-mer fragment) in a deamidation-dependent manner (data not shown); the TCC 387.19 is specific for the DQ2-γ-VII epitope (QQPEQPFPQ) in a deamidation-dependent manner Citation[34]; the T cell line (TCL) KT.CD.E3 is reactive to the 33-mer as well as the DQ2-γ-IV (SQPEQEFPQ) and DQ2-γ-VI (QQPFPEQPQ) epitopes (data not shown); and the TCL 496.1.2 is not reactive to the 33-mer, but is reactive to the DQ2-γ-II epitope (IQPQ/EQPAQL) and is indifferential to deamidation of this epitope (data not shown). T cell proliferation was measured by the uptake of 3H-thymidine (1 µCi/well) added 20 h prior to harvesting. The cells were harvested after 72 h onto glass fiber paper and 3H-thymidine incorporation was measured by liquid scintillation counting. Each antigen concentration was studied in triplicate.
Small-bowel mucosal biopsy organ culture
Small-bowel mucosal biopsies were taken upon upper gastrointestinal endoscopy from 22 untreated celiac disease patients (median age 44 years, range 17–74 years, females 65%) evincing subtotal villous atrophy with crypt hyperplasia and positive serum TG2 autoantibodies. Six healthy subjects (median age 53 years, range 18–68 years, females 50%) with normal small-bowel mucosal morphology and negative serum TG2 antibody levels served as controls. The study protocol was accepted by the Ethical Committee of Tampere University Hospital and written informed consent was obtained from all patients and controls. Three biopsies from each participant were subjected to culture: one received medium only, one PT-gliadin, and one enzymatically pretreated PT-gliadin. Organ culture was carried out for 24 or 48 h depending on further analyses as previously described Citation[35].
TG2 autoantibodies were detected in culture supernatants after a 48 h culture period, using enzyme-linked immunosorbent assay (ELISA) (Celikey®, Phadia, Freiburg, Germany). CD25-positive cells were stained from snap-frozen 5 µm thick small-bowel mucosal biopsy sections by using an immunofluorescence method previously illustrated Citation[35]. Number of lamina propria CD25-positive T cells were calculated from the samples and presented as number of cells in a total area of 1 mm2 of lamina propria Citation[8], Citation[35].
Statistical analysis
Results are given as mean±standard error of mean (SEM). The two-tailed Mann-Whitney U test or the two-tailed Wilcoxon signed ranks test was used to assess the statistical significance of differences. P-values lower than 0.05 were considered significant.
Results
Enzymatic degradation of gliadin
PT treatment cleaved gliadin into peptide products as expected (A and 1B, red line). However, some high-molecular-weight gliadin proteins that eluted at 32 and 33 minutes remained practically intact. Pretreatment of gliadin with wheat enzymes before PT digestion resulted in total disappearance of these gliadin proteins (B, black line). Comparison of HPLC-MS profiles of PT-gliadin and enzymatically pretreated PT-gliadin clearly shows that wheat enzymes are able to cleave gliadin into smaller peptide products than PT digestion only, as the signal intensity at elution times after 12 minutes had diminished. Correspondingly, signal intensity at elution times before 5 minutes had risen. As the toxic 12-mer (p31–43) and immunodominant 33-mer (p56–p89) peptides eluted at 16.5 and 23.3 minutes (C), it can be estimated that germinating wheat enzymes decreased the fraction of gliadin peptides that are potentially harmful to celiac disease patients. Moreover, we were able to measure the relative levels of 12-mer and 33-mer peptides in PT-gliadin and enzymatically pretreated PT-gliadin samples (D). The toxic 12-mer eluting at 16.5 minutes was completely cleaved in enzymatically pretreated gliadin, contrary to PT-treated gliadin. In addition, the level of intact 33-mer peptide eluting at 23.3 minutes was greatly diminished by germinating wheat enzyme treatment, being reduced to only 17% of that in the unprocessed PT-gliadin sample.
Figure 1. Enzymatic degradation of gliadin analyzed by HPLC-MS (A–D) and SDS-PAGE (E). A: HPLC-MS elution pattern of untreated gliadin, full-range m/z = 480–2000. B: Pepsin-trypsin-digested gliadin (PT-G) and PT-G pretreated with germinating wheat enzymes (PT-G + enzymes), full-range m/z = 480–2000. C: 12-mer and 33-mer standard gliadin peptides eluted at 16.5 and 23.3 min, respectively; m/z = 728–730 for 12-mer and 1304–1306 for 33-mer. D: Pretreatment of PT-G with germinating wheat enzymes reduced the levels of 12-mer and 33-mer significantly when compared to PT-G; m/z = 728–730 for 12-mer and 1304–1306 for 33-mer. E: SDS-PAGE analysis of gliadin degradation by wheat enzymes. Lane 1 = standards; lane 2 = gliadin; lane 3 = gliadin + heat-inactivated germinating wheat enzymes; lane 4 = gliadin + germinating wheat enzymes.
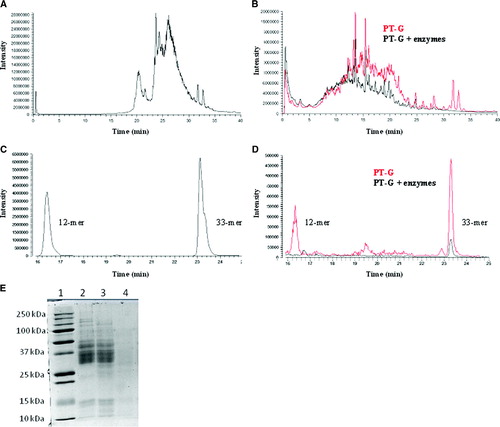
SDS-PAGE of wheat enzyme-treated gliadin
Treatment of gliadin with the germinating wheat enzyme preparation led to the complete disappearance of the SDS-PAGE band pattern typical for gliadin whereas heat-inactivated enzyme preparation (5 min, 100°C) was unable to hydrolyze gliadin (E).
Barrier function
In all Caco-2 cell cultures, TER automatically dropped when the culture medium was changed, as described by Li and colleagues Citation[36] but started to recover after 1 h incubation. In control cultures without any supplementation or with PT-BSA, the TER recovered to near base-line level after 6 h (). In contrast, the TER in cells challenged with PT-gliadin for 6 h remained significantly lower than in the controls. Interestingly, however, when PT-gliadin pretreated with germinating wheat enzymes was administered to Caco-2 cells, the TER recovery resembled that of the controls. After 6 h the TER of the cells receiving germinating wheat enzyme-digested PT-gliadin differed significantly from that of PT-gliadin-treated cells, reaching the level of the PT-BSA control (). Addition of germinating wheat enzymes alone to Caco-2 cell cultures had no effect on TER (data not shown).
Figure 2. Transepithelial resistance of Caco-2 cells treated with study compounds: medium only (open circles), pepsin and trypsin-digested bovine serum albumin (open squares), pepsin and trypsin-digested gliadin (black squares), germinating wheat enzyme-pretreated pepsin-trypsin digest of gliadin (black circles). Data are given as mean values±SEM derived from three independent experiments performed in duplicate. Statistical analyses by two-tailed Mann-Whitney U test. NS = not significant; P<0.05 was considered significant.
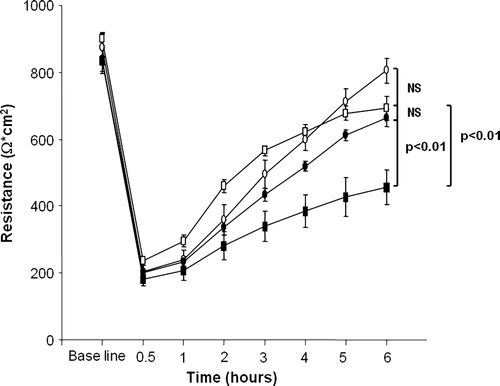
Actin membrane ruffling
When studying the extent of actin cytoskeleton rearrangement as a percentage of Caco-2 cell cluster edge covered by membrane ruffles it was noted that in control cultures with medium only and with PT-BSA supplementation the cell edges remained fairly intact (). In contrast, after PT-gliadin administration large rufflings were observed at the edge of the entire Caco-2 cell clusters. This toxic effect of PT-gliadin was mostly abolished by pretreatment with germinating wheat enzymes (). The extent of membrane ruffling in cell cultures supplemented with germinating wheat enzymes only did not exceed the level of controls (data not shown).
Figure 3. The extent of actin cytoskeleton rearrangement measured as a percentage of Caco-2 cell cluster edge covered by membrane ruffles treated with medium only, pepsin-trypsin-digested bovine serum albumin (PT-BSA), pepsin-trypsin-digested gliadin (PT-G), and germinating wheat enzyme-pretreated pepsin-trypsin-digested gliadin (PT-G + enzymes). A: Representative images of membrane ruffle formation after different treatments. Arrows point to membrane ruffles. Magnification 20×. B: Toxicity of PT-G as measured by the extent of membrane ruffling is abolished by pretreatment of PT-G with germinating wheat enzymes. Data are given as mean values±SEM derived from three independent experiments performed in duplicate. Statistical analyses by two-tailed Mann-Whitney U test. NS = not significant; P<0.05 was considered significant.
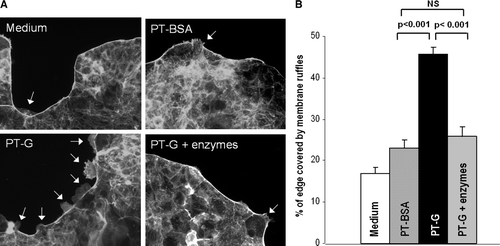
Tight junction-associated protein ZO-1 expression
In cells cultured with medium only and with PT-BSA, tight junction-associated protein ZO-1 expression appeared curly. In cultures challenged with PT-gliadin the junctions clearly straightened. The curly appearance of ZO-1 staining was to some extent preserved in Caco-2 cells receiving germinating wheat enzyme-pretreated PT-gliadin (). Addition of germinating wheat enzymes only did not affect the epithelial junctions (data not shown).
Figure 4. The appearance of Caco-2 cell tight junctions after treatment with medium only, pepsin-trypsin-digested bovine serum albumin (PT-BSA), pepsin-trypsin-digested gliadin (PT-G), and germinating wheat enzyme-pretreated pepsin-trypsin-digested gliadin (PT-G + enzymes). Pictures are representative images from experiments performed in duplicate three independent times. Magnification 100×.
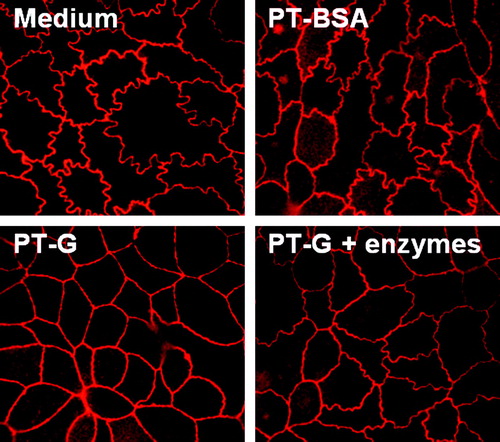
T cell proliferation
When we tested T cell clones which recognize epitopes that differ in their primary sequences as well as two polyclonal T cell lines that are reactive to a broader range of gliadin-derived epitopes, we observed a reduction in T cell proliferation for all TCCs and TCLs tested when they were challenged with enzyme-pretreated PT-gliadin. The extent of the reduction was similar in all cases, and the effect corresponded roughly to a 3-fold shift in gliadin antigen concentration ().
Figure 5. The T cell proliferation as measured by 3H-thymidine incorporation is decreased after germinating wheat enzyme pretreatment of pepsin-trypsin-digested gliadin (PT-G). A: The dose-dependent T cell proliferation of TCC436.5.6 that recognizes the DQ2-αII-epitope found within the 33-mer. B: Proliferation of TCC387.19 that recognizes the DQ2-γVII-epitope. C: The proliferation of TCL496.1.2 that is reactive to the DQ2-γII-epitope but not the 33-mer. D: The proliferation of TCL KT.CD.E3 that is reactive to the 33-mer and the DQ2-γIV and DQ2-γVI-epitopes. Data are given as mean values±SEM (TG2 = transglutaminase 2; PT-BSA = pepsin-trypsin-digested bovine serum albumin; CPM = counts per minute).
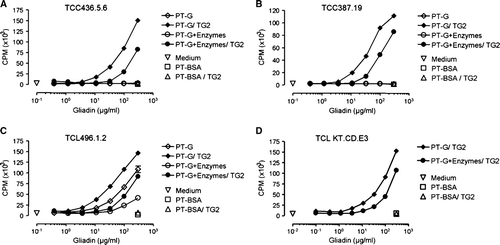
Secretion of TG2 autoantibodies to culture supernatant and the density of CD25+ cells in organ culture of small-bowel mucosal biopsies
In small-bowel mucosal biopsies derived from untreated celiac disease patients, TG2-autoantibody titers were significantly increased in culture supernatants after 48 h PT-gliadin challenge when compared to biopsies cultured with medium only (A). The induction of autoantibody secretion to culture supernatants by PT-gliadin was eliminated when PT-gliadin was pretreated with germinating wheat enzymes. In fact, the autoantibody titers did not significantly differ from those secreted to the supernatant in biopsies cultured in medium only. No autoantibody secretion occurred in any of the organ cultures performed with biopsies derived from healthy controls (A).
Figure 6. A: The secretion of transglutaminase 2 autoantibodies (U) to the organ culture supernatants was significantly increased in small-bowel mucosal explants derived from untreated celiac disease patients after 48 hours’ challenge with pepsin-trypsin-digested gliadin (PT-G). When the explants were incubated with germinating wheat enzyme-pretreated pepsin-trypsin-digested gliadin (PT-G + enzymes), the autoantibody levels remained at control level with medium only. Duodenal explants from healthy controls did not respond to any treatment. B: In the cultured biopsy samples from untreated celiac disease patients, the density of CD25-positive T cells in the mucosal lamina propria increased significantly after PT-G challenge. This toxic effect was markedly diminished when gliadin was pretreated with germinating wheat enzymes. No significant changes were seen in biopsies from healthy controls. Data are given as mean values±SEM. Statistical analyses by the two-tailed Wilcoxon signed ranks test. NS = not significant; P<0.05 was considered significant.
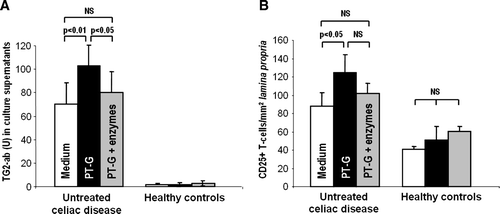
The density of CD25-positive T cells in the mucosal lamina propria increased significantly after 24-h PT-gliadin challenge in biopsies from untreated celiac disease patients (B). However, the number of CD25+ cells in samples cultured in the presence of PT-gliadin pretreated with germinating wheat enzymes remained close to the level of the control cultures. Moreover, different treatments of healthy control biopsies induced no significant changes in CD25+ cells (B).
Discussion
The aim of this work was to show the proof of idea for the concept that the entire range of enzymes from germinated wheat grain can be used to degrade gliadin into harmless products. Indeed, as studied in HPLC-MS and SDS-PAGE, wheat's own enzymes were extremely efficient in degrading the storage protein gliadin. Further mass spectrometric analyses demonstrated that the toxic p31–43 peptide was completely cleaved after enzymatic pretreatment. On the other hand, residual immunogenic 33-mer peptides were still left in the gliadin samples. Although the 24-hour incubation time applied in the current study is far from a physiological time-frame in the gastrointestinal tract, it is important to note that we did not enrich the proteins responsible for the gliadin hydrolysis but instead used simple water-soluble germinating wheat enzyme preparations. Thus it is very likely that the active enzyme concentrations can be increased many-fold by optimizing the extraction process, and hence the incubation times can be shortened respectively.
In the current study, the toxicity of PT-gliadin was markedly diminished both in vitro in cell-based assays and ex vivo in celiac disease patients’ small-bowel mucosal organ culture biopsies after pretreatment with germinating wheat enzymes. However, since the degradation of the 33-mer (and possibly some other toxic) peptides was incomplete it is not surprising that T cell proliferation and activation of CD25+ in the celiac patient organ culture biopsies were only diminished but not completely eliminated after germinating wheat enzyme pretreatment.
Several enzymes have been proposed to be used therapeutically for the degradation of immunotoxic gluten protein. With the exception of barley-derived EP-B2 Citation[29] none of these proteases have been evolutionarily selected for the degradation of cereal storage proteins. Although earlier attempts to detoxify gliadin with different oral proteases such as PEPs from F. meningosepticum, S. capsulata, and M. xanthus have proved promising in in vitro experiments Citation[3], Citation[23], Citation[25], Citation[37], recently Matysiak-Budnik and colleagues Citation[23] showed that fairly high concentrations of bacterial PEP and prolonged exposure are needed to achieve complete digestion of gluten peptides. A further difficulty in using bacteria-derived PEP as an alternative therapy in celiac disease is that it does not completely function in conditions found in the stomach and it is broken down by pepsin Citation[25].
The germinating wheat enzymes used in the current study provide an alternative means to overcome the above-mentioned problems. A considerable advantage of this method lies in the wide variety of different enzymes naturally present in the wheat grain meant for total digestion of full-length wheat storage proteins into amino acids. In the search for a suitable method for gliadin detoxification, it may be assumed that an array of different enzymes can cleave gliadin peptides more efficiently than only one or two specific enzymes. Furthermore, it is known that cereal kernels are colonized by resident bacteria, having their own characteristic set of proteases Citation[38], which again might accelerate gliadin degradation, although their exact role in this process is not known. In addition, germinating wheat enzymes are resistant to digestion with pepsin and trypsin (Venäläinen and associates, unpublished observation) and active over a large pH range Citation[39], being thus effective both in the stomach and in the small intestine. Further benefits of using these enzymes in detoxifying gluten are that they have a good safety profile as they are derived from a naturally safe source, the isolation of these proteases is simple and inexpensive Citation[40], and the method is already widely used in industry in the malting of cereal grains for production of beer, spirits, and baking ingredients.
We conclude that gluten detoxification by germinating wheat enzymes offers a promising alternative means of treating celiac disease in the future. Such enzymes could be administered at meal-times to detoxify ingested gluten, or they could be utilized in food processing in order to eliminate gluten toxicity and to develop high-quality celiac-safe food products. This proof-of-concept study provides a platform for future exploratory investigations.
Acknowledgements
The authors thank Mrs Jaana Leskinen and Mr Jorma Kulmala for technical assistance. The Celiac Disease Study Group and the current work have been financially supported by the Academy of Finland, the Pediatric Research Foundation, the Competitive Research Funding of the Pirkanmaa Hospital District and the European Comission (contract number MRTN-CT-2006-036032). Declaration of interest: The authors report no conflicts of interest. The authors alone are responsible for the content and writing of the paper.
References
- Fasano A, Berti I, Gerarduzzi T, Not T, Colletti RB, Drago S, et al. Prevalence of celiac disease in at-risk and not-at-risk groups in the United States: a large multicenter study. Arch Intern Med. 2003; 163: 286–92
- Bruce G, Woodley JF, Swan CH. Breakdown of gliadin peptides by intestinal brush borders from coeliac patients. Gut. 1984; 25: 919–24
- Shan L, Molberg O, Parrot I, Hausch F, Filiz F, Gray GM, et al. Structural basis for gluten intolerance in celiac sprue. Science. 2002; 297: 2275–9
- Garcia-Horsman JA, Venalainen JI, Lohi O, Auriola IS, Korponay-Szabo IR, Kaukinen K, et al. Deficient activity of mammalian prolyl oligopeptidase on the immunoactive peptide digestion in coeliac disease. Scand J Gastroenterol. 2007; 42: 562–71
- de Ritis G, Auricchio S, Jones HW, Lew EJ, Bernardin JE, Kasarda DD. In vitro (organ culture) studies of the toxicity of specific A-gliadin peptides in celiac disease. Gastroenterology. 1988; 94: 41–9
- Sturgess R, Day P, Ellis HJ, Lundin KE, Gjertsen HA, Kontakou M, et al. Wheat peptide challenge in coeliac disease. Lancet. 1994; 343: 758–61
- Anderson RP, Degano P, Godkin AJ, Jewell DP, Hill AV. In vivo antigen challenge in celiac disease identifies a single transglutaminase-modified peptide as the dominant A-gliadin T-cell epitope. Nat Med. 2000; 6: 337–42
- Maiuri L, Ciacci C, Ricciardelli I, Vacca L, Raia V, Auricchio S, et al. Association between innate response to gliadin and activation of pathogenic T cells in coeliac disease. Lancet. 2003; 362: 30–7
- Sollid LM. Molecular basis of celiac disease. Annu Rev Immunol. 2000; 18: 53–81
- Bethune MT, Khosla C. Parallels between pathogens and gluten peptides in celiac sprue. PLoS Pathog. 2008; 4: e34
- Lammers KM, Lu R, Brownley J, Lu B, Gerard C, Thomas K, et al. Gliadin induces an increase in intestinal permeability and zonulin release by binding to the chemokine receptor CXCR3. Gastroenterology. 2008; 135: 194–204.e3
- Lee A, Newman JM. Celiac diet: its impact on quality of life. J Am Diet Assoc. 2003; 103: 1533–5
- Xia J, Bergseng E, Fleckenstein B, Siegel M, Kim CY, Khosla C, et al. Cyclic and dimeric gluten peptide analogues inhibiting DQ2-mediated antigen presentation in celiac disease. Bioorg Med Chem. 2007; 15: 6565–73
- Chaudhary R, Ghosh S. Infliximab in refractory coeliac disease. Eur J Gastroenterol Hepatol. 2005; 17: 603–4
- Sollid LM, Khosla C. Future therapeutic options for celiac disease. Nat Clin Pract Gastroenterol Hepatol. 2005; 2: 140–7
- Benahmed M, Meresse B, Arnulf B, Barbe U, Mention JJ, Verkarre V, et al. Inhibition of TGF-beta signaling by IL-15: a new role for IL-15 in the loss of immune homeostasis in celiac disease. Gastroenterology. 2007; 132: 994–1008
- Maurano F, Siciliano RA, De Giulio B, Luongo D, Mazzeo MF, Troncone R, et al. Intranasal administration of one alpha gliadin can downregulate the immune response to whole gliadin in mice. Scand J Immunol. 2001; 53: 290–5
- RP Anderson. Development of a vaccine for celiac disease. Frontiers in celiac disease. Pediatric and adolescent medicine. 12. BaselSwitzerland: Karger; 2008.
- Clemente MG, De Virgiliis S, Kang JS, Macatagney R, Musu MP, Di Pierro MR, et al. Early effects of gliadin on enterocyte intracellular signalling involved in intestinal barrier function. Gut. 2003; 52: 218–23
- Paterson BM, Lammers KM, Arrieta MC, Fasano A, Meddings JB. The safety, tolerance, pharmacokinetic and pharmacodynamic effects of single doses of AT-1001 in coeliac disease subjects: a proof of concept study. Aliment Pharmacol Ther. 2007; 26: 757–66
- Hausch F, Halttunen T, Maki M, Khosla C. Design, synthesis, and evaluation of gluten peptide analogs as selective inhibitors of human tissue transglutaminase. Chem Biol. 2003; 10: 225–31
- Siegel M, Khosla C. Transglutaminase 2 inhibitors and their therapeutic role in disease states. Pharmacol Ther. 2007; 115: 232–45
- Matysiak-Budnik T, Candalh C, Cellier C, Dugave C, Namane A, Vidal-Martinez T, et al. Limited efficiency of prolyl-endopeptidase in the detoxification of gliadin peptides in celiac disease. Gastroenterology. 2005; 129: 786–96
- Pyle GG, Paaso B, Anderson BE, Allen DD, Marti T, Li Q, et al. Effect of pretreatment of food gluten with prolyl endopeptidase on gluten-induced malabsorption in celiac sprue. Clin Gastroenterol Hepatol. 2005; 3: 687–94
- Shan L, Marti T, Sollid LM, Gray GM, Khosla C. Comparative biochemical analysis of three bacterial prolyl endopeptidases: implications for coeliac sprue. Biochem J. 2004; 383: 311–8
- Gass J, Ehren J, Strohmeier G, Isaacs I, Khosla C. Fermentation, purification, formulation, and pharmacological evaluation of a prolyl endopeptidase from Myxococcus xanthus: implications for celiac sprue therapy. Biotechnol Bioeng. 2005; 92: 674–84
- Stepniak D, Spaenij-Dekking L, Mitea C, Moester M, de Ru A, Baak-Pablo R, et al. Highly efficient gluten degradation with a newly identified prolyl endoprotease: implications for celiac disease. Am J Physiol Gastrointest Liver Physiol. 2006; 291: G621–9
- Mitea C, Havenaar R, Drijfhout JW, Edens L, Dekking L, Koning F. Efficient degradation of gluten by a prolyl endoprotease in a gastrointestinal model: implications for coeliac disease. Gut. 2008; 57: 25–32
- Gass J, Bethune MT, Siegel M, Spencer A, Khosla C. Combination enzyme therapy for gastric digestion of dietary gluten in patients with celiac sprue. Gastroenterology. 2007; 133: 472–80
- Simpson DJ. Proteolytic degradation of cereal prolamins—the problem with proline. Plant Science. 2001; 161: 825–38
- Lindfors K, Blomqvist T, Juuti-Uusitalo K, Stenman S, Venalainen J, Maki M, et al. Live probiotic Bifidobacterium lactis bacteria inhibit the toxic effects induced by wheat gliadin in epithelial cell culture. Clin Exp Immunol. 2008; 152: 552–8
- Barone MV, Gimigliano A, Castoria G, Paolella G, Maurano F, Paparo F, et al. Growth factor-like activity of gliadin, an alimentary protein: implications for coeliac disease. Gut. 2007; 56: 480–8
- Molberg O, McAdam SN, Korner R, Quarsten H, Kristiansen C, Madsen L, et al. Tissue transglutaminase selectively modifies gliadin peptides that are recognized by gut-derived T cells in celiac disease. Nat Med. 1998; 4: 713–7
- Qiao SW, Bergseng E, Molberg O, Jung G, Fleckenstein B, Sollid LM. Refining the rules of gliadin T cell epitope binding to the disease-associated DQ2 molecule in celiac disease: importance of proline spacing and glutamine deamidation. J Immunol. 2005; 175: 254–61
- Stenman SM, Lindfors K, Korponay-Szabo IR, Lohi O, Saavalainen P, Partanen J, et al. Secretion of celiac disease autoantibodies after in vitro gliadin challenge is dependent on small-bowel mucosal transglutaminase 2-specific IgA deposits. BMC Immunol. 2008; 9: 6
- Li N, DeMarco VG, West CM, Neu J. Glutamine supports recovery from loss of transepithelial resistance and increase of permeability induced by media change in Caco-2 cells. J Nutr Biochem. 2003; 14: 401–8
- Cerf-Bensussan N, Matysiak-Budnik T, Cellier C, Heyman M. Oral proteases: a new approach to managing coeliac disease. Gut. 2007; 56: 157–60
- Laitila A, Kotaviita E, Peltola P, Home S, Wilhelmson A. Indigenous microbial community of barley greatly influences grain germination and malt quality. J Inst Brew. 2007; 113: 9–20
- Hartmann G, Koehler P, Wieser H. Rapid degradation of gliadin peptides toxic for celiac disease patients by proteases from germinating cereals. J Cereal Sci. 2006; 44: 368–71
- Siegel M, Bethune MT, Gass J, Ehren J, Xia J, Johannsen A, et al. Rational design of combination enzyme therapy for celiac sprue. Chem Biol. 2006; 13: 649–58