Abstract
All stages of the embryonic and larval development of Phascolosoma agassizii from Peter the Great Bay (Sea of Japan) were studied and illustrated using light and electron microscopy. The eggs of P. agassizii have the form of an ellipsoid (long and short axes about 100 and 70 µm, respectively). Egg cleavage is typical, spiral, and unequal. Gastrulation occurs by epiboly. This species possesses two pelagic larval stages, a lecithotrophic trochophore and a planktotrophic pelagosphera. The transformation of trochophore into pelagosphera occurs 80–90 h after fertilization. After 120–180 h, the larva has developed all systems of organs characteristic of the pelagosphera and is capable of feeding. At day 10, pelagospheras can settle, for some time, on the aquarium bottom and move on a ciliated lip, collecting food with the aid of a buccal organ. In addition, the larvae periodically attach themselves to the aquarium bottom or to the surface film of the water by means of a terminal organ. The trunk of the larva elongates by enlargement of the region behind the dorsal anal opening, which is located almost in the middle of the trunk region in the 15-day old larva. In the laboratory, 1-month old larvae spend the greater part of time in the attached state. Being attached by a glandular terminal organ to the aquarium bottom, they characteristically bend the body, actively feeding on microalgae from the substratum surface. The differences in the development of P. agassizii in the isolated West-Pacific and East-Pacific populations are shown and discussed.
Introduction
Sipunculans are a group of trochophore animals that includes about 150 species within the phylum Sipuncula.
To date, the development and reproductive biology have been studied, in a varying degree of detail, for 22 sipunculan species. Four main patterns of development can be distinguished: (1) direct lecithotrophic development without pelagic larval stages (Nephasoma minutum – Åkesson Citation1958; Phascolion cryptus – Rice Citation1975; Themiste pyroides – Rice Citation1967); (2) indirect development with one pelagic larva – a lecithotrophic trochophore (Phascolion strombi – Akesson, Citation1961; Phascolopsis gouldi – Gerould Citation1907); (3) indirect development with two pelagic larval stages – a lecithotrophic trochophore and a lecithotrophic pelagosphera that is short lived (2–14 days) (Golfingia elongata – Åkesson Citation1961; Golfingia pugettensis (=Thysanocardia nigra) – Rice Citation1967; Golfingia vulgaris – Gerould Citation1907; T. nigra – Maiorova and Adrianov Citation2007a; Themiste alutacea – Rice Citation1976; Themiste lageniformis – Williams Citation1972; T. pyroides – Maiorova and Adrianov Citation2007a, Citation2007b); and (4) indirect development with two pelagic larval stages – a lecithotrophic trochophore and a long-lived planktotrophic pelagosphera (1–7 months) (Aspidosiphon parvulus – Rice Citation1976, Citation1981; Aspidosiphon fisheri – Rice Citation1975; Golfingia pellucida (=Nephasoma pellucidum) – Rice Citation1976, 1981; Phascolosoma agassizii – Rice Citation1967; Maiorova and Adrianov Citation2007b, Citation2008; Phascolosoma perlucens – Rice Citation1975; Phascolosoma varians (=Phascolosoma nigrescens) – Rice Citation1975; Antillesoma antillarum – Rice Citation1975; Sipunculus nudus – Hatschek Citation1883; Apionsoma misakianum – Rice Citation1978; Jaeckle and Rice Citation2002; Siphonosoma cumanense – Rice Citation1988).
The embryonic development of sipunculans has been studied most completely for Sipunculus nudus (Hatschek Citation1883), Phascolosoma gouldi (=Phascolopsis gouldii) (Gerould Citation1907), and Phascolosoma vulgaris (=Golfingia vulgaris) (Gerould Citation1907).
Some species of sipunculans (A. misakianum, T. nigra, T. pyroides, P. agassizii, P. perlucens, P. varians (=P. nigrescens) were successfully cultured in the laboratory, allowing a detailed description of the larval morphology of these species (Rice Citation1975, Citation1976, Citation1981, Citation1988; Maiorova and Adrianov Citation2007a, Citation2007b; Citation2008).
Individual stages of development have been described, in a varying degree of detail, for several sipunculan species: Golfingia elongate (Åkesson Citation1961), G. pugettensis (=T. nigra) (Rice Citation1967), Golfingia misakiana (=Apionsoma misakiana) (Rice Citation1978), G. pellucida (=N. pellucidum) (Rice Citation1976, Citation1981), P. agassizii (Rice Citation1967, Citation1973), P. varians (=P. nigrescens) (Rice Citation1975), T. pyroides (Rice Citation1967), T. alutacea (Rice Citation1976), S. cumanense (Rice Citation1988), A. parvulus (Rice Citation1981).
The entire cycle of development from fertilized eggs to juvenile stages and adult forms has been fairly completely illustrated for only two species: G. misakiana (=A. misakianum – Cutler Citation1994) and S. cumanense (Rice Citation1978, Citation1981, Citation1988).
The first and, so far, the only attempt to synthesize information on the time and conditions during the breeding season and the specific features of embryogenesis and larval development in the different species of sipunculans is a work of Rice (Citation1967) performed on laboratory maintained specimens of three East-Pacific (British Columbia) species (P. agassizii, Golfingia puggetensis (=T. nigra), and T. pyroides).
Interestingly, these species having an amphi-Pacific distribution and are the most common sipunculans in the West Pacific, including the Sea of Japan; however, due to the substantial differences in their habitat conditions, they have a large number of specific features of reproductive biology, compared to their North-American counterparts (Adrianov and Maiorova Citation2010).
Rice (Citation1967) was the first to present data on the embryonic and larval development stages of P. agassizii cultured in the laboratory. These data illustrated with light micrographs make it possible to follow the main stages of development of this sipunculan. Complete data on the anatomy and histogenesis of the laboratory cultured larval stages of P. agassizii from British Columbia were provided in a detailed work of Rice in 1973; however, again the illustrations here were light microscopy photographs of histological sections of the presented specimens, which, in the absence of total images and summarizing schemes, do not allow a complete representation of the morphogenesis of all developmental stages in this species. Furthermore, all available descriptions of the larvae of P. agassizii are based on specimens obtained in the laboratory, while descriptions of the larvae from wild populations of this species are completely lacking.
The aim of this study was to illustrate and describe, in detail, the stages of embryogenesis and larval development of P. agassizii from the Sea of Japan and to compare the specific features of development of this species in the West and East Pacific, as well as to compare the morphological features of laboratory cultured and wild larvae.
Materials and methods
Specimens of P. agassizii (Keferstein 1867) were collected from April through November during 2003–2008 in Vostok Bay from mussel drusen (Crenomytilus grayanus) at a depth of 1–10 m. The worms were maintained in containers with filtered seawater and the adults were later placed in aquaria with running seawater at a temperature of 16–18°C.
Planktonic larval stages were collected with a Juday net in Vostok Bay and fixed in 10% formalin.
At the end of August, the adults were replaced in 1-L containers (10–20 individuals in each), where the sipunculans began to spawn after several hours at a water temperature of 20–22°C. The sex products were transferred to another container to obtain embryos. The embryos were placed in Petri dishes with filtered seawater and maintained at 18–20°C. Observations of all stages of embryonic development were made. Seven days after commencement of development, P. agassizii reached the stage of planktonic pelagosphera, and the larvae were fed a mixture of unicellular algae Isochrisis galbana and Nannochloris maculata.
To obtain stages with a fully extended introvert, individuals were relaxed by gradual addition of 7.5% MgCl2 in seawater, and their state was monitored under a binocular microscope. The relaxed specimens were fixed in 10% formalin or 2.5% glutaraldehyde. For scanning electron microscopy, material was washed in distilled water and dehydrated via an alcohol and acetone series. After critical point drying, the samples were mounted on metal stubs and sputter-coated with gold. Material was examined with a LEO-430 scanning electron microscope at the Electron Microscopy Center (Institute of Marine Biology, Far East Branch of the Russian Academy of Sciences).
Results
In the Far Eastern seas of Russia, P. agassizii was recorded at the coast of Sakhalin and in the Russian sector of the Sea of Japan from the Tatar Strait to Possjet Bay. It is the most common species of sipunculans in the Sea of Japan. Phascolosoma agassizii reaches the highest densities of up to 450 specimens m−2 in the drusen of a large mussel C. grayanus. Specimens of this species are also encountered within the clumps of Modiolus kurilensis, Mytilus trossulus, and Crassostrea gigas, in the growths of sea grasses Zostera marina and Phillospadex iwatensis, in patches of the substratum between large stones and boulders, within the rhizoids of macrophytic algae, in silty and silty-sandy substrata at depths of 0.5–10 m; however, their density in these biotopes is usually lower than in large drusen of Crenomytilus. Unlike two other common species from the Sea of Japan, T. nigra and T. pyroides, which are seston feeders, P. agassizii can apparently combine seston feeding and detritus feeding. Their tentacular apparatus consists merely of a dorsal arc of relatively short tentacles, and the circular ciliary fold around the mouth opening used for collection of detritus is devoid of any appendages. The trunk region of the mature worms varied from 5 to 40 mm, and the fully extended introvert of these worms is 1.3–1.5 times the length of the trunk. The spawning of P. agassizii in Peter the Great Bay occurs during August–September.
The eggs of P. agassizii are ellipsoidal in shape and measure 100 × 70 µm, regularly rounded in cross-section along the short axis (, , , and ). Mature eggs are pigmented orange-pinkish. At the poles of the ellipsoid, the egg envelope has noticeable depressions, and the depression at the animal pole of the egg is more marked than at the vegetal pole ( and ). The egg envelope is about 3 µm thick and bears a conspicuous transverse striation of microvillar channels.
Figure 1. Embryonic stages and larvae of P. agassizii. A: 20 min; B: 40 min; C: 1 h 40 min; D: 2 h 10 min; E: 2 h 40 min; F: 3 h 10 min; G: 4 h 10 min; H: 6 h 30 min; I: 8 h; J: 24 h, lateral view; K: 48 h, frontal view; L: 48 h, lateral view. Numerals indicate serial numbers of blastomeres. AO, apical organ; AR, archenteron; BP, blastopore; DD, descendant of 2d blastomere; EB, entodermal blastomeres; EN, entoderm; MB, mesoblast; MD, mesoderm; MO, mouth; PH, pharynx; PR, prototroch; RA, rudiment of apical organ.
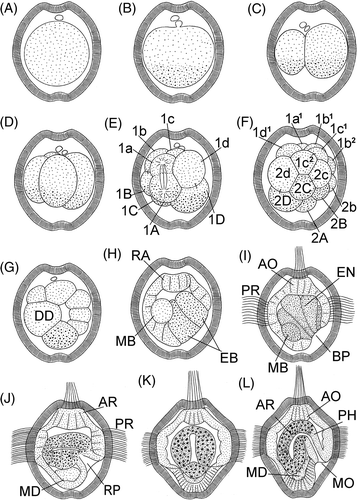
Figure 2. Larvae of P. agassizii. A: 68 h, frontal view; B: 80 h, frontal view; C: 80 h, lateral view. Arrowheads indicate mouth opening. AO, apical organ; CM, coelomic mesoderm; CO, coelom; EY, eyespot; GU, thin gut; PH, pharynx; PR, prototroch; RB, rudiment of buccal organ; RH, rudiment of hindgut; ST, stomach.
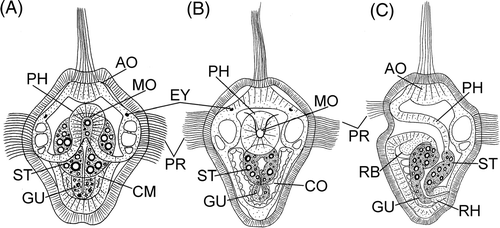
Figure 3. Embryonic stages and larvae of P. agassizii, light micrographs. A: 40 min; B: 1 h 20 min; C: 2 h 20 min; D: 3 h; E: 5 h; F: 7 h; G: 9 h; H: 12 h; I: 50 h; J: 60 h; K: 1 month, lateral view. Bars: 20 µ. AN, anus; AP, tuft of apical cilia; EY, eyespot; GU, thin gut; LI, lip; MO, mouth; MT, metatroch; PR, prototroch; TE, retracted terminal organ.
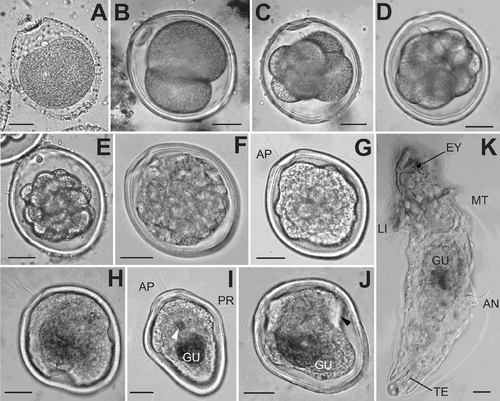
Figure 4. Embryonic stages and larvae of P. agassizii. SEM micrographs. A–G – stages rared in the laboratory, H – larvae from plankton. A–B, eggs; C–E, trochophores; F–H, pelagospheras. A: 2 h, lateral view; B: 2 h, view from vegetal pole; C: 9 h; D: 70 H; E: 80 h; F: 10 days; G: 15 days; H: 1 month. Bars: A–C 10 µ, D–G 20 µ, H 60 µ. White arrowhead indicates sensory groove. AD, apical depression; AN, anus; AP, tuft of apical cilia; DL, dorso-lateral ciliary lobes; LI, lip; MO, mouth; MT, metatroch; PR, prototroch; TP, trunk papillae; VD, vegetal depression.
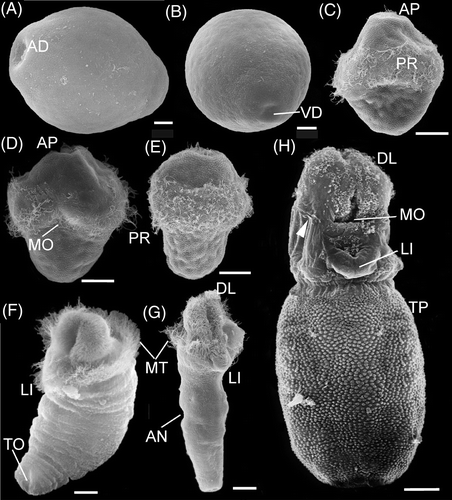
Twenty minutes after fertilization, separation of the first polar body occurs, and the second body separates 40 min after fertilization (, ). The two polar bodies appear at one of narrow poles of the egg, which subsequently becomes the anterior pole of the larva. After separation of polar bodies, an increased concentration of gray-greenish yolk granules is clearly visible at the opposite, (vegetal), pole of the egg. The eggs undergo a typical spiral unequal division ( and ). The first division occurs 1 h 40 min after fertilization, and the two first blastomeres differ in size: the CD blastomere is markedly greater than AD ( and ). An increased concentration of yolk is also observed at the vegetal pole of both blastomeres. The second cleavage division occurs 30 min after completion of the first (). All four blastomeres are located in the same plane, and the D blastomere is markedly larger than the other cells. The third cleavage division is not quite synchronous. The D blastomere begins to divide earlier than other cells of the embryo, the vegetative cell 1D resulting from unequal division is markedly larger than the animal cell 1d ( and ). Separation of the first quartet of micromeres occurs in a dexiotropic direction. The other blastomeres divide almost uniformly: the cells of the first micromere quartet are only slightly smaller than cells of the first quartet of macromeres.
The fourth cleavage division occurs in a neotropic direction, the cells 2d and 2D in the 16-celled embryo are larger than the other blastomeres of the embryo (). The 2A–2D blastomeres also contain larger amounts of yolk than other embryo's cells. We were unable to follow the genealogy of blastomeres at later stages.
Four hours after fertilization, the organization of the embryo is that of a few-celled blastula (). A large cell poor in yolk granules is clearly visible in the wall of the blastula, which is presumably a descendant of 2d blastomere of the 16-celled stage.
Six hours after fertilization gastrulation begins (). Large entodermal cells rich in yolk move inside the embryo. The sinking (epiboly) of the entodermal cells occurs not precisely at the vegetal pole but at the ventral side of the embryo. Early during gastrulation, a large cell poor in yolk, probably 4d blastomere (mesoblast), is observed in the embryo. At the apical pole, the embryo develops an anlage of an apical plate.
Eight hours after fertilization, the embryo begins to swim in the water column (). At this stage, the entodermal cells that have sunken into the interior entirely displace the blastocoel. The blastopore represents a ventral depression in the vegetal half of the embryo.
The main locomotory organ of an early larva is a wide equatorial ciliary band perforating the striated envelope ( and ). A tuft of cilia on the apical plate perforates the egg envelope at the animal pole (, , and ).
The entodermal cells that have moved inside the embryo form a gut, in which a distinct cavity appears 20–24 h after fertilization (). At this stage, the entodermal cells are well-distinguished by the larger, greenish yolk granules. After 22–24 h, the embryo begins to form a primordium of a pharynx shaped as a funnel that opens at the ventral side under the ciliary band. A cluster of transparent mesodermal cells is at the embryo's vegetal pole.
After 48 h, the embryo actively swims in the water column (). The gut is a sac-like organ of cells containing bright-green granules and communicates with the external medium via a tubular pharynx. A mouth opens at the ventral side under the prototroch. The mesoderm consists of two paired bands of transparent cells in ventral to the gut in the embryo's vegetal half.
At the stage about 50 h, eyes appear in a larva (). After 64–68 h, the gut becomes differentiated into a broad stomach and a midgut (). The mesodermal cells form two coelomic sacs to the left and right of the gut in the vegetal part of the embryo and large vacuoles are visible in the cells of the ciliary band ().
During the subsequent 20 h, the mouth opening is gradually shifted forward, into the wide ciliary band (). The posterior part of the larva gradually elongates, with the result that the mouth opens at the anterior part of the larva and the ciliary band becomes adjacent to the mouth opening on the sides and at the anterior.
Eighty hours after fertilization, the gut has differentiated into four parts, a pharynx, a stomach, a small gut, and a hindgut (). The pharynx and the hindgut consist of a transparent tissue and are ectodermal formations. The stomach and the small gut are emerald green and contain large granules refracting the light. Cells of the ciliary band contain large vacuoles. The larva is coated by an egg envelope, which attaches to the epidermis and matches the shape of the larva's body. As a matter of fact, it can be said that the egg envelope transforms to a larval cuticle.
After 90–96 h, the larva becomes vermiform and is capable of contraction as a result of the presence of two pairs of retractor muscles (). At this stage, the larva has a thorough digestive tract. The ciliary band covers the anterior part of larva in the dorsal region and on the sides and adjoins the mouth on the right and on the left. A ring of long cilia, the metatroch, is differentiated behind the mouth to become the main locomotory organ of the larva. Very large vacuoles are visible in the cells underlying the ring of the prototroch. The epidermis under the apical ciliary tuft thickens, probably forming an anlage of a cerebral ganglion. A depression of the epidermis with ciliary tuft is formed under the mouth – the glandular-sensory organ. Rudiments of glands of the lower lip are visible in optical sections of the larva. A rudimentary protrusible tongue, or the buccal organ, is already distinguishable in the anterior digestive tract. A dorsal anus is located at the border of the posterior third of the trunk from the metatroch to the posterior body end. At the posterior end, the larva begins to develop a terminal, or caudal, organ. It is a papilla with large gland cells lying at its base.
Figure 5. Pelagospheras of P. agassizii. A: 96 h, lateral view; B: 96 h, frontal view; C: 120 h, lateral view; D: 180 h, frontal view; E: 180 h, lateral view; F: 1 month, lateral view. AO, apical organ; AN, anus; AS, apical sensilla; BO, buccal organ; BR, brain; CC, circular coelomic canal; CE, coelomocyte; CL, coelomic canals in dorsa-lateral lobes; DL, dorso-lateral ciliary lobes; DR, dorsal retractor; EY, eyespot; GU, thin gut; HG, hindgut; LG, lip gland; LI, lip; ME, mesenterium; MO, mouth; MT, metatroch; NE, nephridium; PH, pharynx; PO, sensory-glandular pore; PR, prototroch; PC, pharyngeal connectives; RN, ventral nerve cord; RP, rudiment of prototroch; RT, retractor of terminal organ; SP, sensory papilla; ST, stomach; TO, terminal organ; VR, ventral retractor.
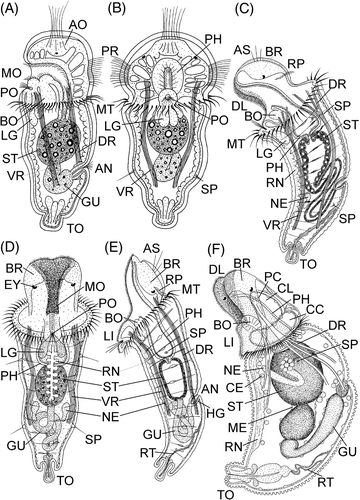
The apical tuft of cilia disappears between 96 and 110 h. The larva at the age of 120 h loses the well-marked prototroch, which is apparently accompanied by the degeneration of vacuolated ectodermal cells (). The epidermis of the anterior end of the larva in the area of the former prototroch is thinned and devoid of the cuticle. The cuticle (a remnant of the egg envelope) is retained on the head lobe and on the trunk behind the metatroch. Remnants of the ciliary band in the form of two ciliary lobes remain on the right and left of the mouth opening. Another remnant of the ciliary belt of early larva is a transverse ciliary band at the dorsal side of the head lobe, which is a remnant of the prototroch. Three to four rigid ciliated sensilla project over the thickening of the epidermis – the anlage of the cerebral ganglion – and pigmented eyes are clearly visible in it. The head region becomes distinctly two-lobed as a result of the formation of dorso-lateral lobes, which subsequently give rise to anlages of primary tentacles. The ventral surface of these lobes is covered by cilia, which are a continuation of the near-mouth ciliary band. The head lobe is already capable of being completely retracted into the trunk region with the aid of well-developed dorsal and ventral retractor muscles. A glandular-sensory pore is visible on the lower lip of the larva and large gland cells lie at its base. At this stage, the larva has formed the protrusible tongue (buccal organ) in ventral to the ectodermal pharynx. The small gut following a spacious stomach markedly elongates, compared to the preceding stage, and assumes a characteristic loop-like form. The larva continues to grow by enlargement of the trunk region posterior to the dorsal anal opening. At the ventral side of the body, anlages of a pair of nephridia are formed at the level of the anal opening. The anlage of a ventral nerve cord is clearly visible at the ventral side of the body. Individual circular muscle fibers are clearly visible in the lumen of the trunk wall, and their contraction ensures the drawing-out of the withdrawable head lobe. Two pairs of lateral sensory papillae with sensilla are clearly visible on the trunk region. The terminal organ is already capable of being withdrawn into the trunk as a result of the formation of a specific retractor attached to the body wall at the dorsal side behind the anal opening.
After 180 h, the lower lip distinctly protrudes under the mouth opening of the larva, and the small gut elongates to become thrown into three conspicuous loops ().
At day 10 the pelagospheras actively swimming in the laboratory aquarium periodically settle on the fully formed lower lip and creep over the substratum (). Also, the larvae continue attaching themselves periodically, by the terminal organ to the aquarium bottom or to the surface water film. The elongation of the body occurs by enlargement of the region behind the dorsal anal opening, which is thus located almost in the middle of the trunk region in a 15-day-old larva (). In the laboratory, 1-month-old larvae spend most of time in the attached state. Being attached by the glandular terminal organ to the aquarium bottom, they characteristically bend the body and actively feed on microalgae from the substratum surface.
Cultured late pelagospheras of P. agassizii and specimens from plankton have a similar structure (, , and ). Late pelagospheras (about 1-month old) sampled from plankton have the head region with two conspicuous lobes; they have developed the lower lip, the protrusible tongue (buccal organ) and all parts of the digestive tract, the dorsal and ventral retractor muscles, and the ventral nerve cord; at this stage, the withdrawable terminal organ and its retractor are well-marked ( and ). Free-swimming cells, coelomocytes, can be clearly seen in the spacious coelom of the trunk of semi-transparent planktonic larvae (). In the tentacular coelom, the larvae have developed the circular coelomic canal and coelomic canals extending into the dorso-lateral lobes of the head, which after settlement will give rise to a pair of primary tentacles. In pelagospheras of P. agassizii, characteristic narrow sensory grooves are well seen laterally to the mouth (), which are also always present in adult worms, being situated laterally to the dorsal nuchal organ because of the overgrowth of the circumoral field and forming the tentacular arc in meiobenthic juvenile worms. However, some differences in the fine structure of wild and cultured stages of the same age can be identified. Unlike laboratory larvae of the same age, which were regularly fed microalgae, planktonic larval stages had a relatively short small gut without characteristic loops ().
In the laboratory, the development of settled pelagospheras, although feeding abundantly, stopped when a characteristic papillar micro-relief of the cuticle had not been formed and the dorsal anal opening still remained in the middle of the trunk region of the larva (). At the age of more than 1 month, larvae developing in nature have the trunk region completely covered by rounded cuticular papillae (). Unlike laboratory stages, the dorsal anus in these larvae is already located at the border of the first third of the trunk length and their retractor muscles are attached to the body wall not in the posterior trunk region but at the level of the anal opening. In nature, such larvae begin to settle on the substratum and transform to vermiform meiobenthic stages.
During this study, we failed to obtain meiobenthic stages from pelagospheras that settled after 1 month of development in the laboratory. The cultured pelagospheras lived for several months after settlement on the bottom of the aquaria but subsequently died, never proceeding to metamorphosis to benthic postlarval stages.
Discussion
Embryonic and larval development of P. agassizii from the Sea of Japan is similar in type to that of representatives of this species from the San Juan Archipelago, which was also examined under laboratory conditions (Rice Citation1967, 1973). The existing differences in the structure and duration of embryonic and larval development stages can be attributable to the specific features of reproductive biology in these isolated populations and to the marked differences in the temperature regime during cultivation in the laboratory and in natural habitats.
On the East-Pacific coast at the San Juan Archipelago, P. agassizii spawns from mid-June to early September (Rice Citation1967) and the embryonic and larval development of this species occurs at lower temperatures (about 12°C) than in the Sea of Japan (about 20°C). The eggs in specimens from the East-Pacific population, though having a characteristic ellipsoidal shape, bear a conspicuous depression only at the animal pole, and are almost 1.5 times larger than those in the Sea of Japan population. In addition, the eggs in the East-Pacific population are markedly compressed on the sides, i.e., they are markedly wider in the frontal than in the sagittal plane (140 × 110 × 91 µm) (Rice Citation1967).
Interestingly, specimens of P. agassizii from the Sea of Japan have one of the smallest values of egg dimensions previously reported not only for species with lecithotrophic trochophore and pelagic pelagosphera, but for the phylum Sipuncula as a whole (Hatschek Citation1883; Gerould Citation1907; Åkesson Citation1958, Citation1961; Rice Citation1967, Citation1970, Citation1973, Citation1975; Adrianov et al. Citation2008).
Among the representatives of the genus Phascolosoma, of which all have ellipsoidal eggs, the smallest egg dimensions were reported for circumtropical P. perlucens (112 × 91 × 86 µm) and P. nigrescens (=P. varians) (104 × 90 µm), and in the former species, the eggs are somewhat compressed in the sagittal plane, as is the case for East-Pacific P. agassizii (Rice Citation1975). Like P. agassizii from the Sea of Japan, the eggs of P. perlucens are characterized by the presence of a terminal depression not only at the animal but also at the vegetal pole. It should be noted that among the phascolosomatids, A. misakianum and A. antillarum also have ellipsoidally shaped eggs (Rice Citation1970, Citation1978).
All main stages of the embryonic and postembryonic development in the P. agassizii population from the Sea of Japan take markedly shorter periods of time than in the cold-water East-Pacific population of this species. Thus, in P. agassizii from San Juan the first and second polar bodies appear 1.5–4 h after fertilization, the first cleavage division occurs 1 h after the second polar body appears, and the second egg division occurs 1 h after that. In specimens from the Sea of Japan, the first cleavage division occurs 1 h and 40 min after fertilization, and the second division already begins 30 min after the first division is completed. In East-Pacific specimens, the few-celled blastula, having a prototroch and an apical tuft, begins to swim near the aquarium bottom 16 h after fertilization, and gastrulation by epiboly occurs between 24 and 36 h of development (Rice Citation1967). In specimens from the Sea of Japan, epiboly takes place as early as 6 h after fertilization.
In East-Pacific specimens, the metamorphosis of trochophore into pelagosphera, which is accompanied by the marked elongation of the body (up to 250 µm), the complete transformation of the egg envelope into the larval cuticle, the breaking of the mouth and anal openings, and the change to feeding on microalgae with the use of a buccal organ protrusible from the mouth cavity, only occurs at day 9–10 (Rice Citation1967, 1973), while in specimens from the Sea of Japan, the above changes have taken place at day 4–5. After 180 h (day 8), cultured pelagosphera larvae of P. agassizii from the Sea of Japan were fully formed. In contrast to pelagospheras from the Sea of Japan having two pairs of long sensory setae, late pelagospheras of P. agassizii from San Juan have three pairs of such setae.
It is interesting to note that tropical species that possess planktotrophic larval stages and spawn under even warmer conditions than P. agassizii from the Sea of Japan exhibit even higher rates of postembryonic and larval development. For example, in laboratory cultured (25°C) Caribbean P. perlucens having very similar eggs to those of P. agassizii from the Sea of Japan, the first division occurs as early as 1 h after fertilization and within 2 h the embryo has reached the 8-celled stage (Rice Citation1975). Eight hours after fertilization, the larva with a ciliary prototroch and an apical ciliary tuft is actively swimming, and after 36 h a lecithotrophic trochophore is fully developed, which at day 3 transforms into a planktotrophic pelagosphera (Rice Citation1975). At day 10, like P. agassizii from the Sea of Japan, pelagosphera of P. perlucens is already capable of settling on its withdrawable terminal organ on the aquarium bottom, where it actively feeds using a buccal organ and can move over the substratum. The structure of late pelagosphera of P. perlucens well-illustrated by Rice (Rice Citation1975, fig. 16) is very similar to that of P. agassizii from the Sea of Japan.
Despite the above similarities in the egg structure and development of P. agassizii from the Sea of Japan and tropical P. perlucens, the adults of these species well differ from each other, thus excluding possible errors in identification of material from the Sea of Japan and the East Pacific (Cutler Citation1994; Morozov and Adrianov Citation2002). Thus, in contrast to P. agassizii, adult specimens of P. perlucens have a relatively short introvert not exceeding the trunk length and their posterior trunk region is covered by large conical papillae directed backward (Cutler Citation1994). The other tropical species, P. nigrescens, differs from P. agassizii in the unique structure of locomotory hooks and the presence of pigmented bands on the introvert. Furthermore, P. perlucens and P. nigrescens have not been recorded in the Sea of Japan and their geographical ranges in the West Pacific extend no farther north as southern Japan (Cutler et al. Citation1984; Cutler Citation1994).
We note that similar differences in the egg dimensions and specific features of development have been reported for other amphi-Pacific species that have isolated West- and East-Pacific populations. For example, in a West-Pacific species T. nigra spawning in Peter the Great Bay (Sea of Japan) from late July to late August at 20–24°C, the eggs are 120 µm in diameter and young pelagosphera is formed at day 4 (Adrianov and Maiorova Citation2010). In contrast, specimens of East-Pacific T. nigra spawning at the San Juan Archipelago from October to January at 8–9°C have an egg diameter of 160 µm and the metamorphosis of trochophore into lecithotrophic pelagosphera occurs only at day 8 (Rice Citation1967). Amphi-Pacific T. pyroides exhibits even greater differences in development. Thus, in Peter the Great Bay (Sea of Japan), representatives of T. pyroides having eggs of 120 µm in diameter spawn during July–October at a temperature range from 15°C to 24°C and pass through the stage of lecithotrophic pelagosphera (Adrianov et al. Citation2008). As opposed to these observations, the East-Pacific counterparts of T. pyroides spawning at the shores of British Columbia from March to August at a temperature of 10–13°C have very large eggs of 190 µm in diameter and exhibit direct development without planktonic larval stages (Rice Citation1967).
To all appearances, the above differences in development rates and the structure of individual developmental stages in the amphi-Pacific species of sipunculans are linked with the substantially different temperature regimes under which spawning occurs in the West- and East-Pacific populations. We remind that in Peter the Great Bay (Sea of Japan), specimens of P. agassizii spawn from August through September at a temperature of 18–22°C, while in waters of the San Juan Archipelago in the East Pacific the spawning of this species is more extended and occurs from mid-June to early September at 10–13°C.
Acknowledgements
The authors gratefully acknowledge the support of the Russian Foundation of Fundamental research (grants 08-04-01001-a, 11-04-98546-r), the Far East Branch of the Russian Academy of Sciences (grants 09-III-A-190, 09-I-P23-01, 09-I-P16-04, and 10-III-В-06-089), and the Ministry of Education and Science of Russian Federation (grant 11.G34.31.0010) during the course of this study.
References
- Adrianov , AV and Maiorova , AS . 2010 . Reproduction and development of common species of peanut worms (Sipuncula) from the Sea of Japan . Russian Journal of Marine Biology , 36 ( 1 ) (in press)
- Adrianov , AV , Maiorova , AS and Malakhov , VV . 2008 . Embryonic and larval development of the peanut worm Themiste pyroides (Sipuncula: Sipunculoidea) from the Sea of Japan . Journal of Invertebrate Reproduction and Development , 52 ( 3 ) : 143 – 151 .
- Åkesson , B . 1958 . A study of the nervous system of the Sipunculoideae with some remarks on the development of the two species Phascolion strombi Montagu and Golfingia minuta Keferstein . Undersokningar over Oresund , 38 : 1 – 249 .
- Åkesson , B . 1961 . The development of Golfingia elongate Keferstein (Sipunculidae), with some remarks on the development of the neurosecretory cells in sipunculids . Arkiv for Zoologi , 13 : 511 – 531 .
- Cutler , EB . 1994 . The Sipuncula: their systematics, biology, and evolution , New York : Cornell University Press .
- Cutler , EB , Cutler , NJ and Nishikawa , T . 1984 . The Sipuncula of Japan: their systematics and distribution . Publications of the Seto Marine Biological Laboratory , 29 ( 4–6 ) : 249 – 322 .
- Gerould , JH . 1907 . Studies on the development of the Sipunculidae, II. The development of Phascolosoma . Zoologische Jahrbuecher Abteilung fuer Anatomie und Ontogenie der Tiere , 23 : 77 – 162 .
- Hatschek , B . 1883 . Ueber Entwicklung von Sipunculus nudus. Arbeiten aus den Zoologischen . Instituten der Universitdt Wien und der Zoologischen Station in Triest , 5 : 61 – 140 .
- Jaeckle , W and Rice , ME . 2002 . “ Phylum Sipuncula ” . In Atlas of marine invertebrate larvae , Edited by: Young , CM , Sewell , MA and Rice , ME . 375 – 396 . New York : Academic Press .
- Maiorova , AS and Adrianov , AV . 2007a . Meiofaunal stages in the development of sipunculans Thysanocardia nigra and Themiste pyroides from the sea of Japan (Sipuncula: Sipunculidea) . Proceedings of THIRIMCO, 13th International Meiofauna Conference . 2007a , Recife. Brasil, p. 58
- Maiorova , AS and Adrianov , AV . 2007b . The development of two common species of peanut worms (Sipuncula) from the Sea of Japan . Proceedings of 11th ICIRD . 2007b , Panama. pp. 31 – 32 .
- Maiorova , AS and Adrianov , AV . 2008 . Morphology of larval and juvenile stages of peanut worms, Phascolosoma agassizii and Themiste pyroides (Sipuncula) . Journal of Morphology , 269 ( 12 ) : 1486
- Morozov , TB and Adrianov , AV . 2002 . Sipunculan fauna of Vostok bay, the Sea of Japan, Russian . Journal of Marine Biology , 28 : 405 – 410 .
- Rice , ME . 1967 . A comparative study of the development of Phascolosoma agassizii, Golfingia pugettensis, and Themiste pyroides with a discussion of developmental patterns in the Sipuncula . Ophelia , 4 : 143 – 171 .
- Rice , ME . 1970 . Asexual reproduction and development in a sipunculan worm . Science , 167 : 1618 – 1620 .
- Rice , ME . 1973 . Morphology, behavior and histogenesis of the pelagosphera larva of Phascolosoma agassizii (Sipuncula) . Smithsonian Contributions to Zoology , 32 : 1 – 51 .
- Rice , ME . 1975 . “ Sipuncula ” . In Reproduction of marine invertebrates , Edited by: Giese , AC and Pearse , JS . Vol. 2 , 67 – 127 . New York : Academic Press .
- Rice , ME . 1976 . Larval development and metamorphosis in Sipuncula . American Zoologist , 16 : 563 – 571 .
- Rice , ME . 1978 . “ Morphological and behavioral changes at metamorphosis in the Sipuncula ” . In Settlement and metamorphosis of marine invertebrate larvae , Edited by: Chia , F-S and Rice , ME . 83 – 102 . Amsterdam : Elsevier/North-Holland Biomedical Press .
- Rice , ME . 1981 . Larvae adrift: patterns and problems in life histories of sipunculans . American Zoologist , 21 : 605 – 619 .
- Rice , ME . 1988 . Observations on the development and metamorphosis of Siphonosoma cumanense with comparative remarks on Sipunculus nudus (Sipuncula, Sipunculidae) . Bulletin of Marine Science , 42 : 1 – 15 .
- Williams , JA . 1972 . Development of a rock burrowing sipunculid inhabiting stony coral . American Zoologist , 12 : 723