Abstract
Background and objective: Different components of the immune system, including innate and adaptive immunity (T effector lymphocytes and T regulatory lymphocytes – TREGs) may be involved in the development of hypertension, vascular injury and inflammation. However, no data are presently available in humans about possible relationships between T-lymphocyte subtypes and microvascular oxidative stress. Our objective was to investigate possible relationships between T-lymphocyte subtypes and systemic and microvascular oxidative stress in a population of normotensive subjects and hypertensive patients.
Patients and methods: In the present study we enrolled 24 normotensive subjects and 12 hypertensive patients undergoing an elective surgical intervention. No sign of local or systemic inflammation was present. All patients underwent a biopsy of subcutaneous fat during surgery. A peripheral blood sample was obtained before surgery for assessment of T lymphocyte subpopulations by flow cytometry and circulating indices of oxidative stress.
Results: A significant direct correlation was observed between Th1 lymphocytes and reactive oxygen species (ROS) production (mainly in microvessels). Additionally, significant inverse correlations were observed between ROS and total TREGs, or TREGs subtypes. Significant correlations were detected between circulating indices of oxidative stress/inflammation and indices of microvascular morphology/Th1 and Th17 lymphocytes. In addition, a significant inverse correlation was detected between TREGs in subcutaneous small vessels and C reactive protein.
Conclusions: Our data suggest that TREG lymphocytes may be protective against microvascular damage, probably because of their anti-oxidant properties, while Th1–Th17 lymphocytes seem to exert an opposite effect, confirming an involvement of adaptive immune system in microvascular damage.
Introduction
In the last decades an important role has been attributed to low grade inflammation in the development of hypertension and cardiovascular disease [Citation1–5]. Indeed, patients with cardiovascular disease show increased plasma concentrations of inflammatory markers [Citation6,Citation7]. Specifically, in hypertensive patients increased plasma levels of inflammatory markers, such as of tumoral necrosis factor α (TNF-α), interleukin (IL)-6, intercellular adhesion molecule-1 (ICAM-1), vascular cells adhesion molecule 1 (VCAM-1), E-selectin, von Willebrand factor (vWF) and C-reactive protein (CRP) have been reported [Citation8–17]. Moreover, inflammatory markers are independently associated with essential hypertension, even after adjustment for other cardiovascular risk factors [Citation18]. The mechanism underlying the relation between inflammation and hypertension involve different components of the immune system, including innate immunity (e.g., macrophages) and adaptive immunity (T effector lymphocytes and T regulatory lymphocytes - TREGs) [Citation19,Citation20] which orchestrate the inflammatory response leading to the development and progression of hypertension, vascular remodeling and target organ damage [Citation21,Citation20,Citation22,Citation23].
T effector cells include T-helper (Th)1 (interferon-γ-producing), Th2 (producing interleukin-4: IL-4), Th 17 (producing IL-17); conversely, TREG lymphocytes express fork-head box protein 3 (FoxP3) transcription factor and produces IL-10 and TGF-β (when concentrations of IL-6 are low) becoming an immunosuppressant cell [Citation20]. In genetic, salt sensitive and angiotensin II-dependent experimental models of hypertension, different populations of T effector (particularly Th1) and TREG lymphocytes seem to be involved with opposite roles in the elevation of blood pressure values [Citation21] as well as in vascular remodeling [Citation20,Citation22]. Moreover, it was suggested that adaptive immunity is enhanced in hypertension as a result of a reduction of TREG cells number and/or function, as observed in experimental models of hypertension such as the Dahl salt-sensitive rat [Citation22].
The possible involvement of Th17 and TREGs in the development and/or maintenance of microvascular structural alterations was recently demonstrated also in humans, in a study addressing specifically possible relationships between microvascular morphology and immunity [Citation24]. An increase in circulating interleukin-17A producing CD4 + T cells and both CD4 + and CD8 + T cells that produce interferon-γ was also observed in hypertensive patients compared with normotensive subjects [Citation25].
Recently, it has been reported that the inflammatory response involving T lymphocytes and the unbalance between effector and suppressor T cells may also be triggered by oxidative stress, with associated blood pressure elevation [Citation20,Citation26]. Also B-lymphocytes may contribute to create a pro-inflammatory milieu in angiotensin II-infused mice [Citation27], even though they are not probably the trigger cell for the development of hypertension [Citation28].
Oxidative stress, which is part of innate immunity responses, has been recognized to plays a critical role in the onset of cardiovascular diseases and hypertension [Citation20,Citation26]. Many hypertensive stimuli, including angiotensin II, aldosterone, cytokines and shear stress, may stimulate NADPH oxidase, uncoupled nitric oxide synthase, and the mitochondria to produce increased reactive oxygen species (ROS) that may trigger inflammatory response [Citation29]. Increased ROS production in hypertension may contribute to vascular damage by promoting vascular smooth muscle cell growth, extracellular matrix deposition, activation of matrix metalloproteinases, inflammation, endothelial dysfunction, and increased vascular tone [Citation30]. In addition, ROS can modulate T-cell function and survival [Citation31]. However, the mechanisms implicating immune response in hypertension are still, in part, elusive [Citation3]. In addition, no data are presently available concerning possible relationships between T lymphocyte subpopulations, and vascular or systemic oxidative stress/inflammation in humans. Recently, non-invasive approaches for the assessment of microvascular structure were proposed and validated [Citation32,Citation33], including the measurement of the wall to lumen ratio of retinal arterioles by scanning laser Doppler flowmetry, or capillaroscopic/videmicroscopic measurement of capillary density. For the previously mentioned reasons, our aim was to evaluate the possible relationship between Th1 or TREG lymphocytes and microvascular or systemic oxidative stress in human beings.
The present study refers to subgroups of patients enrolled in our previously mentioned study [Citation24], but in which additional experiments and further investigations were performed, addressing specifically the issue of possible relationships between immune system and systemic or vascular oxidative stress.
Patients and methods
We enrolled in the study 36 subjects and patients admitted to the Surgical Department of our hospital for indication to standard abdominal surgery, with no signs of systemic inflammation. Indications for surgery were an elective cholecystectomy (15 cases), an incidentaloma of the adrenals with no evidence of hormonal secretion (6 cases), an intervention for inguinal, crural or incisional hernia (15 cases). Twenty-four of them were normotensive and 12 hypertensive, according to the European Society of Hypertension/European Society of Cardiology guidelines 2013 [Citation34]. Ten out of 12 hypertensive patients were on treatment with antihypertensive drugs. Blood pressure was recorded by standard sphygmomanometric approach (three measurements in lying position, 5 min apart) the day before surgical intervention. No wash-out of antihypertensive treatment was possible; therefore blood pressure values recorded were those on-treatment in 10 out of 12 cases. Antihypertensive drugs used included diuretics (3 patients), ACE inhibitors (3 patients), calcium channel blockers (2 patients), angiotensin receptor blockers (6 patients), β-blockers (3 patients) and α1-blockers (1 patient). Other treatments include antiplatelet drugs (21% of patients) or statins (33% of patients). No patients had diabetes mellitus.
The protocol of the study was approved by the ethics committee of our institution (Medical School, University of Brescia), and written informed consent was obtained from each participant. The procedures followed were in accordance with institutional guidelines. The study was performed conform the Declaration of Helsinki.
Oxidative stress in subcutaneous biopsies
We have investigated 11 normotensive subjects and 6 hypertensive patients undergoing an election surgical intervention. All subjects were submitted to a biopsy of subcutaneous fat from the anterior abdominal region. The biopsy of the abdominal subcutaneous fat was taken during the surgical procedure. Surgery was never performed in patients with sign of systemic inflammation.
Superoxide anion production, as index of oxidative stress, was evaluated in the bioptic tissue by the florescent dye dihydroethidium and quantified using an image analyzer, as previously described [Citation35]. Positivity is localized mainly in the microvasculature of the derma and adipose tissue.
Evaluation of retinal arteriolar morphology
All subjects were submitted to a non-invasive evaluation assessment of the retinal arteriolar structure [Citation24,Citation32,Citation33] 3–4 days after surgical intervention. The methods used are reported in the online supplemental material.
Evaluation of skin capillary density
Skin capillary density was evaluated by capillaroscopy before and after venous congestion, as described elsewhere [Citation17,Citation36]. The methods used are reported in the online supplemental material.
Evaluation of circulating CD4 + T lymphocyte phenotype, Cytokine production by CD4 + T cells after in vitro activation and TREG lymphocyte evaluation in small resistance arteries by methylation PCR Analysis
Circulating lymphocytes subpopulations were assessed by flow cytometry, while TREG lymphocytes in the wall of subcutaneous small resistance arteries were evaluated by methylation PCR analysis, as reported in the online supplemental material. TREG lymphocyte content in the wall of subcutaneous small resistance arteries was evaluated in 11 normotensive subjects and 8 hypertensive patients.
The following circulating subpopulations of TREGs were evaluated: TREG RTE: recent thymus emigrant cells; TREG Naïve: no previous contact with antigens; TREG CM; T Central Memory cells; TREG EM: effector memory cell (highly antigen-experienced cells active in adaptive response); TREG TDEM: TDEM Terminal Differentiated Effector Memory: highly antigen-experienced cells that assume pro-apoptotic properties.
Evaluation of systemic oxidative stress/inflammation
In 24 normotensive subjects and 12 hypertensive patients the following circulating indices of oxidative stress/inflammation were evaluated by ELISA/RIA, as previously described [Citation16,Citation17,Citation37].
IL-6, monocyte chemoattractant peptide-1 (MCP-1), IL-18, CRP, TNFα, ICAM-1, VCAM-1, PAI-1, total antioxidant power (TAO), malonyldialdehyde (MDA), lipid peroxidation (LPO).
Statistical analysis
All data are expressed as mean + SD. Differences between groups were evaluated by Student’s unpaired t-test. Distribution of categorical variables between groups was evaluated by χ-square test. Relationships between variables were assessed by calculation of Pearson’s correlation. All parameters were normally distributed. Since this may be considered a hypothesis-generating study, no correction for multiple comparison was performed. A value of p < 0.05 was considered statistically significant. All analyses were carried out with SPSS software (version 13.0, SPSS Inc., Chicago, Illinois, USA).
Results
Demographic characteristics of the population investigated in the study patients have been previously reported [Citation24], and are summarized in .
Table 1. Demographic and clinical characteristics of the population evaluated.
Microvascular structure and circulating T-lymphocytes
Microvascular structural parameters and data about circulating CD4 + lymphocytes in the whole population, as well as in normotensive subjects and in hypertensive patients have been previously reported [Citation24], and are here summarized in the online supplemental material.
Relationships with oxidative stress in subcutaneous biopsies or with circulating indices of inflammation/oxidative stress
ROS production was not different between normotensive subjects and hypertensive patients (4.86 ± 2.89 vs. 3.73 ± 2.76 units per area, respectively, p = NS).
A significant direct correlation was observed between Th1 lymphocytes and ROS production (mainly localized in microvessels) (). Additionally, significant inverse correlations were observed between ROS and total TREGs, RTE and EM TREGs ().
Figure 1. Left: direct correlation between Th1 lymphocytes (%) and reactive oxygen species in the subcutaneous tissue biopsies (ROS) (unit per area). Right: inverse correlation between total TREGs (absolute number) and reactive oxygen species in the subcutaneous tissue biopsies (ROS) (unit per area).
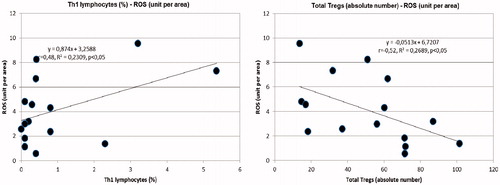
Figure 2. Left: inverse correlation between RTE TREGs (%) and reactive oxygen species in the subcutaneous tissue biopsies (ROS) (unit per area). Right: inverse correlation between RTE TREGs (absolute number) and reactive oxygen species in the subcutaneous tissue biopsies (ROS) (unit per area).
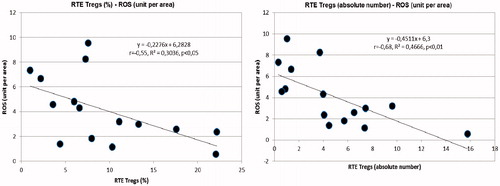
Figure 3. Left: inverse correlation between EM TREGs (absolute number) and reactive oxygen species in the subcutaneous tissue biopsies (ROS) (unit per area). Right: inverse correlation between small resistance arteries TREG (unmethylated FoxP3) and circulating C Reactive Protein.
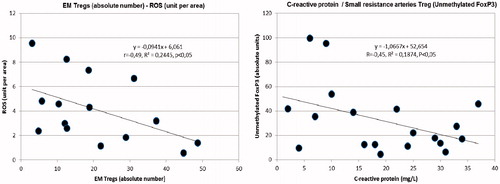
Significant correlations were detected between circulating indices of oxidative stress/inflammation and indices of microvascular morphology, Th1 and Th17 lymphocytes or biohumoral and clinical parameters (). Circulating indices of inflammation/oxidative stress were similar in hypertensive patients and normotensive subjects (data not shown), apart from MCP-1 which was significantly higher in hypertensive patients ().
Figure 4. Increased circulating levels of monocyte chemoattractant peptide-1 (MCP-1) (pg/ml) in hypertensive patients compared with normotensive subjects.
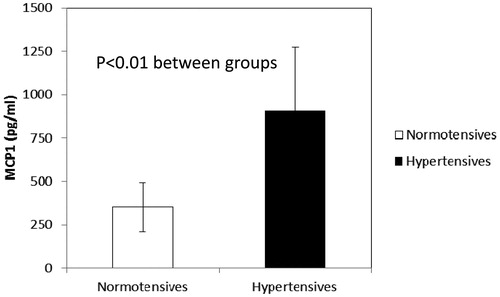
Table 2. Correlations observed with indices of oxidative stress
Relationship between TREG lymphocytes in small resistance arteries and C-reactive protein
TREGs in subcutaneous small resistance arteries were not different between normotensive subjects and hypertensive patients (31.6 ± 27.3 vs. 31.3 ± 28.8 absolute units, respectively, p = NS).
A significant inverse correlation was detected between TREGs in subcutaneous small vessels and CRP (−0.45, r2 = 0.19, p < 0.05) ().
Discussion
In the present study for the first time it has been demonstrated that some lymphocyte subpopulations may be related to indices of microvascular and systemic oxidative stress/inflammation in humans. In hypertension several inflammatory mechanisms, such as adhesion molecules and chemokines expression, cytokine release, immune cell activation and infiltration, and oxidative stress seem to be increased [Citation3] These processes may increase blood pressure values and contribute to vascular remodeling and kidney damage [Citation21]. Recent data suggest that the innate and adaptive immune systems participate to low-grade inflammation and, therefore, may play a role in the development and progression of hypertension and end-organ damage. In particular, the balance between Th effector (especially Th1/interferon γ-producing lymphocytes) and TREGs may affect the degree of vascular inflammation. Indeed, Th1 infiltration is the prominent response found in inflamed vessels of experimental animal models with cardiovascular disease [Citation38], and it may contribute to increased blood pressure by affecting the kidney and vascular remodeling both directly, via the effects of produced cytokines, and through effects on perivascular fat [Citation21,Citation39]. How activation of immune responses is triggered remains presently unknown, but neo-antigens could be generated by elevated blood pressure levels [Citation21].
In our study, a significant direct correlation was observed between Th1 lymphocytes and ROS production (mainly localized in microvessels). ROS may play a critical roles in the cardiovascular system. Oxidative stress has been shown to be linked to a proinflammatory state that includes upregulation of adhesion molecules (VCAM-1 and ICAM-1), through activation of several proinflammatory transcription factors and chemotactic molecules, such as MCP-1, that promote tissue accumulation of inflammatory cells [Citation28]. It has been proposed that the infiltration of immune cells may lead to oxidative stress and/or increased release of angiotensin II contributing to elevated levels of arterial blood pressure and renal damage. Moreover, ROS itself can affect T cell polarization and cytokine secretion [Citation19,Citation28,Citation29].
ROS have been reported to induce sympathetic outflow in the central nervous system; they may also cause vasoconstriction at vascular level and may promote sodium and volume retention in the kidney. All these effects may directly induce an increase in blood pressure levels and amplify inflammatory responses, further promoting an increase in blood pressure values.
In the present study we also observed significant inverse correlations between ROS and total TREGs, RTE and EM TREGs. It has been reported that TREGs are crucial for vascular homeostasis, regulate inflammatory responses by suppressing the functions of reactive T cells and protect from blood pressure elevation [Citation21]. It seems also that TREGs suppress angiotensin II–mediated vascular injury in part through anti-inflammatory actions [Citation23]. These findings strongly support the idea that immune mechanisms modulate angiotensin II–induced blood pressure elevation, vascular oxidative stress, inflammation, and endothelial dysfunction [Citation40–42]. Kassan M et al addressed the issue of possible relationships between TREGs and vascular dysfunction in hypertension, suggesting that TREGs imbalance may be a major factor in vascular endothelial dysfunction, plaque rupture and myocardial infarction [Citation43,Citation44]. Interestingly enough, in the present study a significant inverse correlation was detected between TREGs in subcutaneous small vessels and circulating C-reactive protein.
Available data about possible involvement of effector T lymphocytes in humans are rather limited [Citation24,Citation25,Citation45]. In human hypertension, with aging, effector lymphocytes may become senescent, with consequent increase in surface adhesion molecules and cytolytic enzyme expression, and enhanced cytotoxicity [Citation46]. We have observed an increase of CD4 + CD28-IFN-γ + Th1 circulating lymphocytes in hypertensive patients, compared with normotensive subjects [Citation24]. This population corresponds to senescent lymphocytes, and was previously demonstrated to be increased in some pathological conditions, including patients with aortic aneurisms [Citation47], unstable angina [Citation48] and early atherosclerotic damage [Citation49]. Therefore, T cells might contribute to human hypertension, as suggested by animal studies, and may also play a role in the development/maintenance of microvascular structural alterations [Citation40].
Although in our study [Citation24] no difference was observed between normotensive subjects and hypertensive patients, previous data are in favor of a possible role of Th17 lymphocytes in hypertension and organ damage [Citation3,Citation25,Citation50]. Indeed, IL-17 has been demonstrated to contribute to a vascular inflammatory response and it represents an important mediator in angiotensin II-induced hypertension as well as in other model of experimental hypertension [Citation3,Citation50]. Moreover, several evidences have demonstrated an increase in Th17 cells in patients with acute coronary syndrome and unstable carotid atherosclerotic plaques with a significant functional imbalance of Th17/TREG lymphocytes [Citation51]. Similarly, an increase of the Th17 subtype was observed also in patients with type 2 diabetes mellitus and hypertension [Citation25], suggesting that Th17 subset may particularly take part in the pathogenesis of cardiovascular and metabolic diseases [Citation52].
Limitation of the study
Our study has the limitations inherent to many human studies adopting locally invasive procedures. The number of the subjects and patients enrolled is relatively limited, the population enrolled partially heterogeneous (treated and non-treated patients) and the correlations observed were relatively weak. In particular, our hypertensive patients had well controlled blood pressure values, and therefore no difference in indices of microvascular structural alterations was observed compared with normotensive controls, as previously observed in other studies [Citation53], in which no differences in wall to lumen ratio of retinal arterioles were observed between treated hypertensive and normotensive controls. This may have reduced the possibility to detect closer correlations between the evaluated variables due to the lack of subjects/patients with severely altered vessels.
Glomerular filtration rate (GFR) was slightly different between hypertensives and normotensives, but within normal limits in the majority of our population.
Age represents a factor that may affect vascular structure per se; however, there were no significant correlations between either GFR or age, and microvascular morphological parameters or lymphocytes subpopulations, both in the overall population and in our subgroups. Also previous antihypertensive treatment may have represented a confounding factor. It is not presently know whether antihypertensive treatment might affect TREGs or other lymphocyte subpopulations, while it is well known that some antihypertensive drugs are more effective that others in terms of regression of microvascular structural alterations [Citation32]. In addition, only clinic blood pressure values were available in our population; for practical reasons we could not perform 24 hour non-invasive blood pressure monitoring, which could have provided more meaningful information.
Finally, being a hypothesis-generating study, we did not make corrections for multiple comparisons. This might have exposed to the risk of generating false positive results.
Clinical perspectives
As mentioned, subsets of T lymphocytes have been implicated in the pathogenesis of hypertension and vascular remodeling. This is an area of active research and rapid development in cardiovascular and renal diseases [Citation19]. It was already shown that immunosuppressant drugs such as mycophenolate mofetil may reduce blood pressure values and protect from renal damages Dahl salt-sensitive rats [Citation54], as well as mice infused with angiotensin II [Citation55]. Interestingly enough, in immune-suppressed patients with human immune deficiency virus-associated disease, although cardiovascular disease may occur in part as a result of side effects of antiviral agents used in the treatment of the disease, there is no excess prevalence of hypertension that has been noted [Citation41]. In conclusion, adaptive immune systems may contribute to the pathogenesis of cardiovascular disease and vascular remodeling probably through inflammation and oxidative stress. TREG lymphocytes, which elicit an anti-inflammatory activity, may be crucial for blood pressure elevation and organ damage development, at least in experimental models.
Our data suggest that TREG lymphocytes may be protective against microvascular damage, probably because of their anti-inflammatory and anti-oxidant properties, while Th1–Th17 lymphocytes seem to exert an opposite effect, confirming an involvement of adaptive immune system in microvascular damage. These new data implicating T lymphocytes will eventually allow discovery of new therapeutic targets that may improve outcomes in hypertension and cardiovascular or renal disease in humans [Citation21,Citation20].
Disclosures statement
A declaration of interest statement reporting no conflict has been inserted.
Rizzoni_et_al._supplemental_content.docx
Download MS Word (1 MB)References
- Tracey KJ. Reflex control of immunity. Nat Rev Immunol. 2009; 9:418–428.
- Intengan HD, Schiffrin EL. Vascular remodeling in hypertension: roles of apoptosis, inflammation, and fibrosis. Hypertension. 2001;38:581–587.
- Leibowitz A, Schiffrin EL. Immune mechanisms in hypertension. Curr Hypertens Rep. 2011;13:465–472.
- Harrison DG, Guzik TJ, Lob HE, et al. Inflammation, immunity, and hypertension. Hypertension. 2011;57:132–140.
- Harrison DG, Vinh A, Lob H, et al. Role of the adaptive immune system in hypertension. Curr Opin Pharmacol. 2010;10:203–207.
- Blake GJ1, Ridker PM. Novel clinical markers of vascular wall inflammation. Circ Res. 2001;89:763–771.
- Sesso HD, Buring JE, Rifai N, et al. C-reactive protein and the risk of developing hypertension. JAMA.2003;290:2945–2951.
- Preston RA, Ledford M, Materson BJ, et al. Effects of severe, uncontrolled hypertension on endothelial activation: soluble vascular cell adhesion molecule-1, soluble intercellular adhesion molecule-1 and von Willebrand factor. J Hypertens. 2002;20:871–877.
- Blake GJ, Rifai N, Buring JE, et al. Blood pressure, C-reactive protein, and risk of future cardiovascular events. Circulation. 2003;108:2993–2999.
- Blann AD, Tse W, Maxwell SJ, et al. Increased levels of the soluble adhesion molecule E-selectin in essential hypertension. J Hypertens. 1994;12:925–928.
- Ferri C, Desideri G, Baldoncini R, et al. Early activation of vascular endothelium in nonobese, nondiabetic essential hypertensive patients with multiple metabolic abnormalities. Diabetes.1998;47:660–667.
- DeSouza CA, Dengel DR, Macko RF, et al. Elevated levels of circulating cell adhesion molecules in uncomplicated essential hypertension. Am J Hypertens. 1997;10:1335–1341.
- Buemi M, Allegra A, Aloisi C, et al. Cold pressor test raises serum concentrations of ICAM-1, VCAM-1, and E-selectin in normotensive and hypertensive patients. Hypertension.1997;30:845–847.
- Ferri C, Bellini C, Desideri G, et al. Clustering of endothelial markers of vascular damage in human salt-sensitive hypertension: influence of dietary sodium load and depletion. Hypertension. 1998;32:862–868.
- Verhaar MC, Beutler JJ, Gaillard CA, et al. Progressive vascular damage in hypertension is associated with increased levels of circulating P-selectin. J Hypertens. 1998;16:45–50.
- De Ciuceis C, Rossini C, Tincani A, et al. Effect of antihypertensive treatment with lercanidipine on endothelial progenitor cells and inflammation in patients with mild to moderate essential hypertension. Blood Press.2016;25:337–343.
- De Ciuceis C, Salvetti M, Rossini C, et al. Effect of antihypertensive treatment on microvascular structure, central blood pressure and oxidative stress in patients with mild essential hypertension. J Hypertens. 2014;32:565–574.
- Bautista LE, Vera LM, Arenas IA, et al. Independent association between inflammatory markers (C-reactive protein, interleukin-6, and TNF-alpha) and essential hypertension. J Hum Hypertens. 2005;19:149–154.
- Virdis A, Schiffrin EL. Vascular inflammation: a role in vascular disease in hypertension? Curr Opin Nephrol Hypertens. 2003;12:181–187.
- Schiffrin EL. T lymphocytes: a role in hypertension? Curr Opin Nephrol Hypertens. 2010;19:181–186.
- Schiffrin EL. Immune mechanisms in hypertension and vascular injury. Clin Sci. 2014;126:267–274.
- Viel EC, Lemarié CA, Benkirane K, et al. Immune regulation and vascular inflammation in genetic hypertension. Am J Physiol Heart Circ Physiol. 2010; 298:H938–H9344.
- Barhoumi T, Kasal DA, Li MW, et al. T regulatory lymphocytes prevent angiotensin II-induced hypertension and vascular injury. Hypertension. 2011;57:469–476.
- De Ciuceis C, Rossini C, Airò P, et al. Relationship between different subpopulations of circulating CD4+ T-lymphocytes and microvascular structural alterations in humans. AJHYPE. 2017;30:51–60.
- Itani HA, McMaster WG, Jr., Saleh MA, et al. Activation of human T cells in hypertension: studies of humanized mice and hypertensive humans. Hypertension. 2016;68:123–132.
- Guzik TJ, Hoch NE, Brown KA, et al. Role of the T cell in the genesis of angiotensin II induced hypertension and vascular dysfunction. J Exp Med. 2007;204:2449–2460.
- Chan CT, Sobey CG, Lieu M, et al. Obligatory role for B cells in the development of angiotensin II-Dependent Hypertension. Hypertension. 2015;66:1023–1033.
- Caillon A, Schiffrin EL. Role of inflammation and immunity in hypertension: recent epidemiological, laboratory, and clinical evidence. Curr Hypertens Rep. 2016;18:21–25.
- Harrison DG, Gongora MC. Oxidative stress and hypertension. Med Clin North Am. 2009;93:621–635.
- Touyz RM, Schiffrin EL. Reactive oxygen species in vascular biology: implications in hypertension. Histochem Cell Biol. 2004;122:339–352.
- Jackson SH, Devadas S, Kwon J, et al. T cells express a phagocyte-type NADPH oxidase that is activated after T cell receptor stimulation. Nat Immunol. 2004;5:818–827.
- Virdis A, Savoia C, Grassi G, et al. Evaluation of microvascular structure in humans: a 'state-of-the-art' document of the Working Group on Macrovascular and Microvascular Alterations of the Italian Society of Arterial Hypertension. J Hypertens. 2014;32:2120–2129.
- Rizzoni D, Porteri E, Duse S, et al. Relationship between media-to-lumen ratio of subcutaneous small arteries and wall-to-lumen ratio of retinal arterioles evaluated noninvasively by scanning laser Doppler flowmetry. J Hypertens. 2012;30:1169–1175.
- Mancia G, Fagard R, Narkiewicz K, Task Force Members, et al. 2013 ESH/ESC Guidelines for the management of arterial hypertension: the Task Force for the management of arterial hypertension of the European Society of Hypertension (ESH) and of the European Society of Cardiology (ESC). J Hypertens. 2013;31:1281–1357.
- Rizzoni D, Porteri E, De Ciuceis C, et al. Hypertrophic remodeling of subcutaneous small resistance arteries in patients with Cushing’s syndrome. J Clin Endocrinol Metab. 2009;94:5010–5018.
- De Ciuceis C, Rossini C, Porteri E, et al. Circulating endothelial progenitor cells, microvascular density and fibrosis in obesity before and after bariatric surgery. Blood Press. 2013;22:165–172.
- De Ciuceis C, Porteri E, Rizzoni D, et al. Effects of weight loss on structural and functional alterations of subcutaneous small arteries in obese patients. Hypertension. 2011;58:29–36.
- Taleb S, Tedgui A, Mallat Z. Regulatory T-cell immunity and its relevance to atherosclerosis. J Intern Med. 2008;263:489–499.
- Carnevale D, Lembo D. Immunological aspects of hypertension. High Blood Press Cardiovasc Prev. 2016;23:91–95.
- Schiffrin EL. Immune modulation of resistance artery remodelling. Basic Clin Pharmacol Toxicol. 2012;110:70–72.
- Schiffrin EL. The immune system: role in hypertension. Can J Cardiol. 2013;29:543–548.
- Schiffrin EL. Immune mechanisms in hypertension: how do T-regulatory lymphocytes fit in? J Hypertens. 2013;31:1944–1945.
- Kassan M, Wecker A, Kadowitz P, et al. CD4 + CD25 + Foxp3 regulatory T cells and vascular dysfunction in hypertension. J Hypertens. 2013;31:1939–1943.
- Kassan M, Galan M, Partyka M, et al. Interleukin-10 released by CD4 + CD25+ natural regulatory T cells improves microvascular endothelial function through inhibition of NADPH oxidase activity in hypertensive mice. Arterioscler Thromb Vasc Biol. 2011;11:2534–2542.
- Zhang J, Crowley SD. Role of T lymphocytes in hypertension. Curr Opin Pharmacol. 2015;21:14–19.
- Youn J-C, Yu HT, Lim BJ, et al. Immunosenescent CD8+ T cells and C-X-C chemokine receptor type 3 chemokines are increased in human hypertension. Hypertension. 2013;62:126–133.
- Duftner C, Seiler R, Klein-Weigel P, et al. High prevalence of circulating CD4 + CD28- T-cells in patients with small abdominal aortic aneurysms. Arterioscler Thromb Vasc Biol. 2005;25:1347–1352.
- Liuzzo G, Goronzy JJ, Yang H, et al. Monoclonal T-cell proliferation and plaque instability in acute coronary syndromes. Circulation. 2000;101:2883–2888.
- Gerli R, Schillaci G, Giordano A, et al. CD4 + CD28- T lymphocytes contribute to early atherosclerotic damage in rheumatoid arthritis patients. Circulation. 2004;109:2744–2748.
- Madhur MS, Lob HE, McCann LA, et al. Interleukin 17 promotes angiotensin II-induced hypertension and vascular dysfunction. Hypertension. 2010;55:500–507.
- Cheng X, Yu X, Ding YJ, et al. The Th17/Treg imbalance in patients with acute coronary syndrome. Clin Immunol. 2008;127:89–97.
- Wang Q, Zhai X, Chen X, et al. Dysregulation of circulating CD4 + CXCR5+ T cells in type 2 diabetes mellitus. APMIS. 2015;123:146–151.
- Harazny JM, Ritt M, Baleanu D, et al. Increased wall:lumen ratio of retinal arterioles in male patients with a history of a cerebrovascular event. Hypertension. 2007;50:623–629.
- De Miguel C, Das S, Lund H, et al. T lymphocytes mediate hypertension and kidney damage in Dahl salt-sensitive rats. Am J Physiol Regul Integr Comp Physiol. 2010;298:R1136–R1142.
- Rodríguez-Iturbe B, Pons H, Quiroz Y, et al. Mycophenolate mofetil prevents salt-sensitive hypertension resulting from angiotensin II exposure. Kidney Int. 2001;59:2222–2232.