Abstract
Purpose: Device-guided slow breathing (DGB) is indicated as nonpharmacological treatment for hypertension. The sympathetic nerve activity (SNA) reduction may be one of the mechanisms involved in blood pressure (BP) decrease. The aim of this study is to evaluate the long-term use of DGB in BP and SNA.
Subjects and methods: Hypertensive patients were randomized to listen music (Control Group–CG) or DGB (aim to reduce respiratory rate to less than 10 breaths/minute during 15 minutes/day for 8 weeks). Before and after intervention ambulatory blood pressure monitoring (ABPM), catecholamines and muscle sympathetic nerve activity (MSNA) by microneurography were performed.
Results: 17 volunteers in the DGB and 15 in the CG completed the study. There was no change in office BP before and after intervention in both groups. There was a reduction in systolic and diastolic BP in the awake period by ABPM only in the CG (131 ± 10/92 ± 9 vs 128 ± 10/88 ± 8mmHg, p < 0.05). In relation to SNA, no difference in catecholamines was observed. In the volunteers who had a microneurography record, there was no change the MSNA (bursts/minute): DGB (17(15–28) vs 19(13–22), p = 0.08) and CG (22(17–23) vs 22(18–24), p = 0.52).
Conclusion: Long-term DGB did not reduce BP, catecholamines levels or MSNA in hypertensive patients.
ClinicalTrials.gov identifier: NCT01390727
Introduction
It is well established that nonpharmacological treatments of hypertension are proven to reduce blood pressure (BP). There are a lot of evidences that lifestyle changes, such as DASH/Mediterranean diets, reduced sodium intake, regular physical exercise, weight control and limitation of alcohol consumption can reduce BP [Citation1]. In addition to these strategies, relaxation techniques [Citation2] as yoga [Citation3] and meditation [Citation4] practice can also reduce BP, but with lower levels of evidences.
Device-guided slow breathing [Citation5–9] has also shown efficacy in BP control. Although there are some controversies [Citation10,Citation11], the American Heart Association [Citation12] (AHA) recommend this techniques as a nonpharmacological therapy of hypertension. However, the physiological mechanisms responsible for the BP reduction are not well defined.
A proposed mechanism is that the modulation of sympathetic nerve activity (SNA) could be affected by the respiratory rate (RR). Reductions in RR at around 6 to 10 breaths per minute by increasing tidal volume would stimulate cardiopulmonary stretching receptors, which in turn would reduce the discharge of sympathetic efferent fibers resulting in a decrease in systemic vascular resistance and consequent BP reduction [Citation13].
Study by our group showed that a single session of 15 minutes of device-guided slow breathing significantly reduced muscle sympathetic nerve activity (MSNA) [Citation14]. We also show in a case report [Citation15] significant reduction in plasma norepinephrine levels (903 to 220 pg/ml) and the MSNA (51 to 22 bursts/minute) in a hypertensive patient after regular device-guided slow breathing by 8 weeks. However, there is in the literature only one study that evaluated the MSNA in hypertensive patients who performed device-guided slow breathing by long-term (15 minutes daily for 8 weeks) and MSNA reduction was not observed [Citation16].
Therefore, the aim of this study is to evaluate the responses of BP and MSNA after device-guided slow breathing by long-term in hypertensive patients.
Subjects and methods
Study subjects
Patients were selected at Nephrology Division, Hypertension Unit, University of São Paulo General Hospital, São Paulo, Brazil. Hypertensive patients with the following inclusions criteria were selected: both genders, ≥18 years-old, with or without pharmacological treatment and mean 24-hour BP by ambulatory blood pressure monitoring (ABPM) above the normal range, systolic blood pressure (SBP) ≥ 130 mmHg and/or diastolic blood pressure (DBP) ≥ 80mmHg [Citation17]. Patients in use of beta-blockers or centrally acting sympatholytic agents, 3 or more antihypertensive drugs, diagnosis of secondary hypertension, chronic respiratory disease, diabetes mellitus, chronic renal disease defined as estimated glomerular filtration rate (eGFR) by the equation MDRD Study <60 ml/min [Citation18], coronary artery disease, congestive heart failure, pregnant women and patients with a body mass index (BMI) > 30 kg/m2 were excluded. The inclusion of patients was made between May 2011 and December 2014.
The study was approved by the Ethics Committee from the University of São Paulo General Hospital (Number: 0107/10). All participants agreed to participate in the study and signed the free and informed consent form.
Study protocol
This is a randomized, open-label clinical trial. The randomization was generated using the internet site http://www.randomization.com. The type of randomization was block size. Before the intervention, all participants performed 24-hour ABPM and an experimental session to evaluate the SNA. In the experimental session the SNA was measured by plasma catecholamine levels dosage and microneurography. After the experimental session, participants were randomly divided into two groups: control group (CG) or device-guided slow breathing (DGB). All participants were assessed again after eight weeks from randomization when ABPM and the experimental session were repeated.
ABPM
The ABPM was performed using Mobil-O-Graph® NG device (IEM, Solberg, Germany), validated according to the British Hypertension Society protocol [Citation19]. An appropriately sized cuff for the arm circumference was installed on the non-dominant arm. Blood pressure was measured every 20 minutes by 24 hours, and awake and sleep periods were determined according to information provided by the patients. ABPM was accepted if it had a minimum of 16 awake and 8 sleep measurements. In addition, a minimum time of 22 registration hours was required [Citation15]. If these criteria were not met, the examination should be repeated.
Office blood pressure measurement
The office blood pressure measurement was performed with DX 2710 monitor [Citation20] (Dixtal Biomedical, Manaus, Brazil) after five minutes of rest, with the participant seated, arm at heart level and an appropriately sized cuff for the arm circumference. Three measurements were performed with an interval of two minutes between them in the presence of an observer. For the determination of office blood pressure, the mean of the last two readings was considered [Citation1].
Muscle sympathetic nerve activity (MSNA) recordings
The MSNA was measured during two experimental session directly into the fascicle of the fibular nerve, through the microneurography technique [Citation21], which allows the recording of the action potentials of the fibers A and C in peripheral nerves.
The technique consists of a percutaneous electrical stimulation (40V to 120V) to map the nerve localization. Then a tungsten microelectrode (30–40 mm long, 0.2 mm diameter and uninsulated tip of 1–5 μm) was impacted on the nerve at the site which better response to percutaneous stimulation and another electrode was introduced as reference approximately 2 cm away. The nerve signal was amplified and digitized by PowerLab 8/30 (ADInstruments, Sydney, Australia) and recorded for 20 minutes (10 minutes baseline, 3 minutes handgrip, 2 minutes occlusion, 5 minutes recovery) in the LabChart 7 Pro software (ADInstruments, Sydney, Australia) at a sampling frequency of 2K/s.
After a stable signal was obtained, MSNA was recorded for 10 minutes and the average result obtained from the recording in this period of time was considered the baseline MSNA. From the tenth minute of the MSNA recording, an isometric physical stimulation with manual dynamometer (Baseline®, New York, USA) was performed for 3 minutes at an intensity equivalent to 30% of maximal voluntary contraction. Immediately after the isometric physical stimulus, the arm circulation was occluded for 2 minutes, with a cuff inflated with a pressure of 200 mmHg.
MSNA was measured by counting the number of bursts/minute at baseline (10 minutes) and during the excitatory maneuvers.
Plasma catecholamines
A blood sample for measurement of plasma catecholamines was collected in begin of each experimental sessions (before and after intervention) from the antecubital vein. To perform this procedure, participants remained at rest, in supine position, for 30 minutes and after this time the blood sample was collected. The plasma catecholamines dosage was made by “High Performance Liquid Chromatography”.
Interventions
During the 8-week intervention period, participants who were randomized for DGB used the REPeRATE® device (InterCure Ltd, Tel-Aviv, Israel) daily for 15 minutes with the aim to reduce RR. On the other hand, the participants who were randomized for the CG were instructed to listen an MP3 player that reproduced serene music (different songs´ selections with 2-hour duration).
During this phase of the study participants were followed each four weeks to check adherence to the proposed interventions. Adherence to the interventions was evaluated using a calendar where they marked the duration of the interventions. In DBG, beyond the calendar notes, the downloading of the following data stored in the device memory was made: number of sessions (n), average time spent (minutes) in the zone breathing therapy (<10 breaths/minute), mean initial and final RR and the average time that the respiratory sensor was able to identify breathing (%).
Outcomes
The primary endpoints were change in SNA measured by microneurography and plasma catecholamines after the intervention period (8 weeks). Secondary outcomes were changes in SBP and DBP.
Statistical analysis
Sample size was calculated considering a 5% significance level, 80% test power, 7 bursts/minute as the standard deviation from MSNA measured by microneurography and expecting that the difference between the groups (DGB versus CG) reach 8 bursts/minute [Citation12]. Respecting these premises, a sample size of 10 patients was determined for each group.
Only results of patients who completed the entire protocol were analyzed (per-protocol analysis). Categorical variables were described as absolute number and relative frequency. For continuous variables those with normal distribution were presented as mean ± standard deviation and those with non-normal distribution as median and 1st and 3rd quartiles. Data from the two groups were compared through analysis of variance with repeated measures. The difference was showed with Welch test paired for each treatment combination and phase. Holm test was used to correct multiple comparisons when necessary. p-values <0.05 were considered significant.
Results
Seventeen participants in the DGB and 15 in the CG finished the study protocol. Ten participants from each group had a MSNA record by microneurography ().
The clinical and demographic characteristics of the participants who completed the protocol are shown in . There was no statistically difference between the groups in most of the variables, except for the antihypertensive therapy: a higher percentage of CG participants were in pharmacological therapy for hypertension.
Table 1. Demographic, clinical and laboratory characteristics: Device-Guided Slow Breathing (DGB) and control group (CG).
Office BP, 24-hour ABPM and heart rate (HR)
shows the results of office BP, 24-hour ABPM and HR before and after intervention. There was no change in office BP before and after intervention in both groups. There was SBP and DBP reduction in awake BP before and after intervention only in the CG (131 ± 10/92 ± 9 vs 128 ± 10/88 ± 8 mmHg, p < 0.05).
Table 2. Office and 24-hours blood pressure before and after the intervention: Device-Guided Slow Breathing (DGB) versus Control Group (CG).
It was also observed a HR increase after intervention in DGB: 24 hours (75 ± 7 vs 78 ± 7 bpm, p < 0.05) and sleep (66 ± 8 vs 70 ± 9 bpm, p < 0.05).
SNA-plasma catecholamines and microneurography
Plasma norepinephrine concentration can be seen in . No differences were observed in norepinephrine concentrations in both groups before and after intervention: GC (221 (170–266) vs 242 (179–337) pg/ml, p = 0.54) and in DGB (227 (193–309) vs 211 (153–269) pg/ml, p = 0.54).
Figure 2. Plasma norepinephrine concentration before and after intervention in the Control Group (CG) and Device-Guided Slow Breathing (DGB).
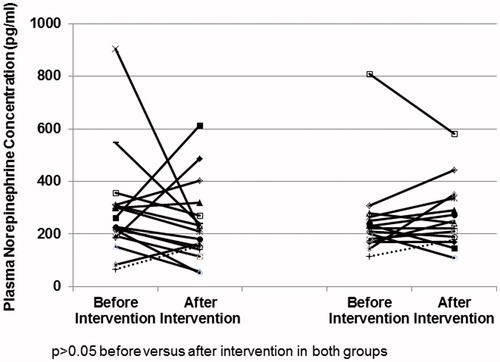
shows the SNA measured by the microneurography. No differences in the MSNA were observed before and after intervention in CG (22 (17–23) vs 22 (18–24) bursts/minute, p = 0.52) and in DGB (17 (15–28) vs 19 (13–22) bursts/minute, p = 0.08).
Figure 3. Muscular Sympathetic Nervous Activity (MSNA) measured by microneurography before and after intervention in the Control Group (CG) and Device-Guided Slow Breathing (DGB).
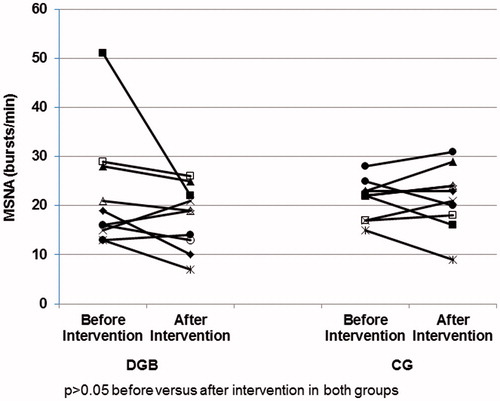
The MSNA changes with the excitatory maneuvers are described in . No attenuation was observed in MSNA increase induced by isometric muscular contraction and blood flow occlusion before and after intervention in both groups.
Table 3. Muscular Sympathetic Nervous Activity (MSNA) measured by microneurography before and after intervention: Device-Guided Slow Breathing (DGB) versus Control Group (CG).
Adherence
Adherence according to the volunteers’ diary was good in both groups: 95% of the sessions were done in the GC and 93% in the DGB. In DBG, the downloading of the data stored in the device memory also shows a good adherence: number of sessions performed (days), 28 ± 5 (1° month) and 28 ± 6 (2° month); average time spent (minutes) in the therapeutic breathing zone (<10 breaths/minute), 13 ± 4 (1° month) and 13 ± 3 (2° month); mean initial RR, 10 ± 3 (1° month) and 9 ± 4 (2° month); mean final RR, 6 ± 2 (1° month) and 6 ± 2 (2° month); and the average time that the respiratory sensor was able to identify breathing (%), 90 ± 6 (1° month) and 91 ± 4 (2° month).
Discussion
The present study demonstrated that the SNA from hypertensive patients measured by plasma catecholamines and microneurography are not affected by long term use DGB (15 minutes daily for 8 weeks). In addition, no BP reduction was observed in the office or ABPM.
Two previous studies [Citation14,Citation16] showed MSNA reduction after an isolated session of DGB in hypertensive patients. However, one of these studies [Citation16] also evaluated the effect of long term (8 weeks) use of DGB on the MSNA and did not show any SNA reduction, thus corroborating the data of the present study.
One possible explanation for these results is that the BP reduction observed in some studies is not mediated by MSNA reduction, that is, other mechanisms would be involved. However, a case report [Citation15] performed by our group shows plasma catecholamines and MSNA reduction after the long-term use of DGB. This particularly patient has an extremely high SNA before intervention (plasma norepinephrine: 903 pg/ml; 51 bursts/minute by microneurography). Although plasma catecholamines are criticized for their accuracy in measuring sympathetic nerve activity in humans [Citation22], this particular clinical case illustrates that the methodology is a useful complementary tool to microneurography.
Thus, to explain the discrepancy of results observed in these different studies, it is possible to suggest the hypothesis that DGB would be effective only in patients with sympathetic hyperactivity. The group of patients included in the present study has clinical characteristics that do not indicate sympathetic hyperactivity: mild hypertensive, without obesity or chronic kidney disease and plasma norepinephrine in the normal range.
Regarding office and 24 hours BP, we did not show BP reduction after long-term (8 weeks) DGB. These findings corroborate the results of an earlier study [Citation23] conducted in a population of non-diabetic hypertensive patients who showed that DGB did not reduce office and 24-hour BP greater than to listen serene music. Two other studies [Citation9,Citation16], one using DGB by 08 weeks and other by 04 weeks, showed significant reductions only in office BP, but not in 24-hour BP. The office BP reduction not reproduced in 24-hour BP could be possibly due to the modulation of the white coat effect [Citation16] by DGB. In the present study, the white coat effect was possibly excluded during the participants' selection, since all the patients included in the study has 24-hour BP elevated by ABPM, independent of the office BP.
Despite DGB is recommended as a nonpharmacological therapy for hypertension by the AHA [Citation10], the effects of this approach on BP are still controversial. Overall, most of the studies published to date have reported favorable effects in relation to office BP in hypertensive patients without other co-morbidities [Citation5,Citation6,Citation7,Citation9,Citation24] and hypertensive patients with DM [Citation8]. However, there are studies that report neutral effects in hypertensive patients with [Citation10,Citation11] and without diabetes [Citation16,Citation23].
The results of one systematic review [Citation25] and two meta-analyzes [Citation26,Citation27] suggest that the DGB is not effective in BP control when used for long term. Therefore, the present study strengthen the results of these reviews. Some authors even suggest that the AHA recommendation would be reviewed [Citation28].
A result that deserves discussion is the BP reduction observed by ABPM in patients randomized to listen music. The BP reduction in control groups in studies with hypertensive patients is not rare [Citation29,Citation30]. The placebo effect could explain this result by itself. Another explanation would be that to listen music daily could have some antihypertensive effect not mediated by SNA reduction. Particularly in the present study, an adherence improvement to prescribed antihypertensive therapy could explain this finding. Although the participants were randomized, a greater percentage of patients taking antihypertensive drugs were present in the CG (75% vs 23%, p < 0.01). Although adherence to drug therapy has not been objectively evaluated, a patient who participates in a clinical protocol may improve adherence to drug therapy and, consequently, had better BP control.
Although DGB is an interesting nonpharmacological therapy for hypertension, it still needs more evidence that demonstrates its real effectiveness. If this method is really able to reduce blood pressure, the mechanisms responsible for this reduction should be more extensively studied and not limited to the role of the sympathetic nervous system.
Disclosure statement
The authors report no conflicts of interest.
Additional information
Funding
References
- Malachias MVB, Souza WKSB, Plavnik FL, et al. 7ª Diretriz Brasileira de Hipertensão Arterial. Arq Bras Cardiol. 2016;107(Suppl.3):1–83.
- Irvine MJ, Johnston DW, Jenner DA, et al. Relaxation and stress management in the treatment of essential hypertension. J Psychosom Res. 1986;30:437–450.
- McCaffrey R, Ruknui P, Hatthakit U, et al. The effects of Yoga on hypertensive persons in Thailand. Holist Nurs Pract. 2005;19:173–180.
- Chesney MA, Black GW, Swan GE, et al. Relaxation training for essential hypertension at the worksite: I. The untreated mild hypertensive. Psychosom Med. 1987;49:250–263.
- Schein MH, Gavish B, Herz M, et al. Treating hypertension with a device that slows and regularises breathing: a randomised, doble-blind controlled study. J Hum Hypertens. 2001;15:271–278.
- Grossman E, Grossman A, Schein MH, et al. Breathing-control lowers blood pressure. J Hum Hypertens. 2001;15:263–269.
- Elliot WJ, Izzo JL, Jr., White WB, et al. Graded blood pressure reduction in hypertensive outpatients associated with use of a device to assist with slow breathing. J Clin Hypertension. 2004;6:553–559.
- Schein MH, Gavish B, Baevsky T, et al. Treating hypertension in type II diabetic patients with device-guided breathing: a randomized controlled trial. J Hum Hypertens. 2009;23:325–331.
- Anderson DE, McNeely JD, Windham BG. Regular slow-breathing exercise effects on blood pressure and breathing patterns at rest. J Hum Hypertens. 2010;24:807–813.
- Logtenberg SJ, Kleefstra N, Houweling ST, et al. Effect of device-guided breathing exercises on blood pressure in hypertensive patients with type 2 diabetes mellitus: a randomized controlled trial. J Hypertens. 2007;25:241–246.
- Landman GW, Drion I, van Hateren KJ, et al. Device-guided breathing as treatment for hypertension in type 2 diabetes mellitus: a randomized, double-blind, sham-controlled trial. JAMA Intern Med. 2013;173:1346–1350.
- Brook RD, Appel LJ, Rubenfire M, American Heart Association Professional Education Committee of the Council for High Blood Pressure Research, Council on Cardiovascular and Stroke Nursing, Council on Epidemiology and Prevention, and Council on Nutrition, Physical Activity, et al. Beyond medications and diet: alternative approaches to lowering blood pressure: a scientific statement from the American Heart Association. Hypertension. 2013;61:1360–1383.
- Goso Y, Asanoi H, Ishise H, et al. Respiratory modulation of muscle sympathetic nerve activity in patients with chronic heart failure. Circulation. 2001;104:418–423.
- Oneda B, Ortega KC, Gusmão JL, et al. Sympathetic nerve activity is decreased during device-guided slow breathing. Hypertens Res. 2010;33:708–712.
- de Barros S, da Silva GV, de Gusmão JL, et al. Reduction of sympathetic nervous activity with device-guided breathing. J Clin Hypertens (Greenwich). 2014;16:614–615.
- Hering D, Kucharska W, Kara T, et al. Effects of acute and long-term slow breathing exercise on muscle sympathetic nerve activity in untreated male patients with hypertension. J Hypertens. 2013;31:739–746.
- V Diretrizes Brasileiras de Monitorização Ambulatorial da Pressão Arterial (MAPA V) e III Diretrizes Brasileiras de Monitorização Residencial da Pressão Arterial (MRPA III). Sociedades Brasileiras de Cardiologia, Hipertensão e Nefrologia. Arq Bras Cardiol. 2011;97(Suppl 3):1–24.
- Levey AS, Stevens LA, Schmid CH, CKD-EPI (Chronic Kidney Disease Epidemiology Collaboration), et al. A new equation to estimate glomerular filtration rate. Ann Intern Med. 2009;150:604–612.
- Jones CR, Taylor K, Chowienczyk P, et al. A validation of the Mobil O Graph (version 12) ambulatory blood pressure monitor. Blood Press Monit. 2000;5:233–238.
- Mano GM, Souza VF, Pierin AM, et al. Assessment of the DIXTAL DX-2710 automated oscillometric device for blood pressure measurement with the validation protocols of the British Hypertension Society (BHS) and the Association for the Advancement of Medical Instrumentation (AAMI). Arq Bras Cardiol. 2002;79:606–610.
- Mark AL. Microneurography: a technique for study of the sympathetic nervous system in human hypertension. J Cardiovasc Pharmacol. 1987;10:S56–S59.
- Grassi G, Mark A, Esler M. The sympathetic nervous system alterations in human hypertension. Circ Res. 2015;116:976–990.
- Altena MR, Kleefstra N, Logtenberg SJ, et al. Effect of device-guided breathing exercises on blood pressure in patients with hypertension: a randomized controlled trial. Blood Press. 2009;18:273–279.
- Meles E, Giannattasio C, Failla M, et al. Nonpharmacologic treatment of hypertension by respiratory exercise in the home setting. Am J Hypertens. 2004;17:370–374.
- van Hateren KJ, Landman GW, Logtenberg SJ, et al. Device-guided breathing exercises for the treatment of hypertension: an overview. World J Cardiol. 2014;6:277–282.
- Mahtani KR, Nunan D, Heneghan CJ. Device-guided breathing exercises in the control of human blood pressure: systematic review and meta-analysis. J Hypertens. 2012;30:852–860.
- Landman GW, van Hateren KJ, van Dijk PR, et al. Efficacy of device-guided breathing for hypertension in blinded, randomized, active-controlled trials: a meta-analysis of individual patient data. JAMA Intern Med. 2014;174:1815–1821.
- van Dijk PR, Landman GW, van Hateren KJ, et al. Call for a re-evaluation of the American Heart Association’s standpoint concerning device-guided slow breathing using the RESPeRATE device. Hypertension 2013;62:e17.
- Beckett NS, Peters R, Fletcher AE, HYVET Study Group, et al. Treatment of hypertension in patients 80 years of age or older. N Engl J Med. 2008;358:1887–1898.
- Bhatt DL, Kandzari DE, O'Neill WW, SYMPLICITY HTN-3 Investigators, et al. A controlled trial of renal denervation for resistant hypertension. N Engl J Med. 2014;370:1393–1401.