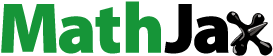
Abstract
Purpose: The aim was to investigate the association between blood pressure (BP), carotid stiffness, carotid-femoral pulse wave velocity (cfPWV) and left ventricular (LV) remodeling in never-treated hypertensive patients.
Material and methods: 178 never-treated hypertensive underwent transthoracic echocardiography, 24-hour ambulatory BP monitoring (ABPM), local carotid stiffness and regional cfPWV assessed using a high-definition echo-tracking ultrasound system and a tonometric transducer, respectively. LV parameters and arterial stiffness were also considered in dippers and non-dippers.
Results: Mean night-time BP best correlated with carotid and LV parameters. Carotid stiffness parameters (β-index, pressure-strain elastic modulus, one-point PWV) correlated with LV mass, relative wall thickness, and E/A ratio while cfPWV correlated only with E/A ratio. In multiple regression analysis, age and mean night-time ABPM had a stroger relation with carotid stiffness than cfPWV. In a second multiple regression analysis, day and night ABPM and carotid stiffness were independently related with LV remodeling and left atrial volume. In non-dippers, local carotid stiffness parameters were significantly higher than in dippers, whereas cfPWV was not significantly different.
Conclusions: Carotid stiffness parameters are independently associated with LV remodeling and have an additive effect to BP and over cfPWV moreover local arterial stiffness is higher in non-dippers.
Introduction
Arterial stiffening is a key feature of atherosclerosis and is considered an independent predictor of cardiovascular morbidity and mortality. In turn, age, hypertension and cardiovascular risk factors are also responsible for arterial stiffness [Citation1–4]. Although carotid-femoral pulse wave velocity (cfPWV) is considered as the gold standard for measuring arterial stiffness and improving cardiovascular risk stratification in hypertensive patients, estimation of local carotid stiffness seems to be promising and easily obtainable from ultrasound-based systems, particularly in young and healthy adults showing an impedance mismatch between a highly compliant ascending aorta and the common carotid artery (CCA). In pathological conditions as well as in the elderly, increased ascending aorta stiffness may match CCA stiffness, supporting the use of the latter as a surrogate measure of the former. In a population based-cohort followed for 7.6 years, van Sloten and colleagues found that carotid and femoral stiffness indices were independently associated with cardiovascular events and all-cause mortality [Citation5]. In a recent study by Bruno et al., cfPWV and carotid stiffness were differentially associated with target organ damage (TOD) in never treated hypertensive patients: regional arterial stiffness was associated with renal involvement, whereas local carotid stiffness was related to cardiac organ damage [Citation6]. The development of TOD may therefore reflect the close relationship between arterial stiffness and cardiovascular events [Citation6]. Given the interaction between the heart and the arterial system, widely known as ventricular–arterial coupling, arterial stiffness should be considered together with cardiac function. In this respect, the 2013 ESH/ESC guidelines for the management of hypertension recommend the assessment of arterial stiffness by PWV as a marker of TOD [Citation7]. Additionally, ambulatory blood pressure monitoring (ABPM) measurements reflect more accurately the actual blood pressure (BP) load and have a stronger correlation with subclinical TOD than office BP measurements [Citation7–9].
Aim was to investigate the independent effect of local carotid stiffness on left ventricular (LV) adaptation beyond of the arterial load in never treated hypertensive patients. In addition we also aimed at investigate the relationship between blood pressure, in particular mean night-time ABPM and arterial stiffness.
Methods
Study population
From 2014 to 2016, a total of 219 newly diagnosed and never-treated hypertensive patients were consecutively enrolled in the study. They were referred to the hypertensive outpatient clinic of the Cardiology Department of the San Antonio Hospital, San Daniele del Friuli, Udine (Italy) for recent diagnosis of high BP and cardiovascular risk stratification.
All subjects underwent physical examination and anthropometric measurements. Data on medical history and dietary habits, including coffee intake, alcohol use, and cigarette smoking, were collected from all participants. Physical activity was assessed using a standardized questionnaire [Citation10].
The diagnosis of essential hypertension was established upon BP readings of ≥140/90 mmHg on three consecutive clinic visits. No patient developed more than grade 2 hypertension, as defined in the ESH/ESC guidelines [Citation11]. Participants were excluded if they had secondary hypertension, diabetes mellitus, nephropathy, cardiovascular disease, or any condition requiring chronic medication [Citation12].
The study was approved by the institution's ethics board and informed consent was obtained from the participants.
Blood pressure measurements
Office brachial BP was measured using an oscillometric semiautomatic sphygmomanometer and heart rate (HR) recordings were taken twice in supine position, in the right arm, 10 min apart in a quiet room. Overall, 190 hypertensive subjects underwent ABPM with the Takeda TM 2420 Model 7 device (A&D Co., Tokyo, Japan) or the ICR Spacelabs 90207 monitor (Spacelabs Inc., Redmond, WA), according to standardized procedures [Citation13]. Both devices have been validated [Citation13,Citation14]. The ABPM was performed during a routine working day, with BP measured every 15 min during daytime (7 a.m.–10 p.m.) and every 20 min during night-time (10 p.m.–7 a.m.). Non-dipping BP was calculated as (daytime systolic BP – night-time systolic BP)/daytime systolic BP ×100. A day–night dip in systolic BP ≥10% was used to categorize patients as normal “dippers”, whereas a day–night dip in systolic BP <10% was used to categorize patients as “non-dippers” [Citation15].
A total of 178 subjects had a valid 24-hour ABPM recording and constituted the present study population.
Regional arterial stiffness
Just before to apply the 24h ABPM, cfPWV was measured using a single high-fidelity tonometric Millar transducer (SphygmoCor, Model SCOR-Px, software version 7.01, AtCor Medical Pty Ltd, Sydney, NSW, Australia). The pulse wave was recorded sequentially at the carotid and femoral arteries by the transducer. The transit time between carotid and femoral pulse waves was calculated using the “foot-to-foot” method via ECG. The surface distance was measured between the recording point at the carotid artery and the sternal notch (distance 1) and between the sternal notch and the recording point at the femoral artery (distance 2).
The distance traveled by the pulse wave (DPW) and cfPWV were calculated by SphygmoCor according to the following formula [Citation16]:
Echocardiography
In all 178 subjects with a valid 24-hour ABPM recording, a comprehensive transthoracic Doppler echocardiographic examinations were performed under continuous ECG recording using commercially available equipment (Alpha 10; Aloka, Tokyo, Japan), in accordance with the EACVI and ASE recommendations [Citation17]. Measurements of LV dimension were done, and LV mass (LVM) and relative wall thickness (RWT) were derived [Citation18]. The LVM was indexed to body surface area (BSA) [Citation17]. Left atrial maximal volume was measured at the point of mitral valve opening using the biplane area–length method and corrected for BSA [Citation17]. LV stroke volume (SV) was calculated as the product of the Doppler velocity-time integral and cross-sectional area at the level of the LV outflow tract, and then the SV index (SV/BSA) was derived. Conventional and pulsed tissue Doppler-derived parameters of LV diastolic function were measured according to the ASE/EACVI recommendations [Citation19]. Each variable was averaged from three to five consecutive cardiac cycles. All parameters were re-estimated offline with an image processing workstation (ComPACS v.10.5.8, MediMatic, Genoa, Italy).
Local carotid artery stiffness parameters
Local carotid stiffness was measured at the end of the echocardiographic exam. The method has been described in detail elsewhere [Citation20]. Briefly, local arterial stiffness was evaluated at the level of the left CCA, at one-point between 1 and 2 cm before its bifurcation using a high-definition echo-tracking ultrasound system (Alpha 10, Aloka, Tokyo, Japan) with a multifrequency 5–13 MHz linear probe. Echo-tracking uses the raw radiofrequency signals with an accuracy of 0.01 mm. The optimal angle between the ultrasound beam and the vessel wall for diameter change measurements by echo-tracking is 90° (left side panel A), but given that blood flow velocity is perpendicular to the beam and cannot be detected, a different independent steerable ultrasound beam was used. (right side panel A) shows a long-axis view of CCA and the ultrasound beam configuration with the independent beam steering function. The solid line shows the ultrasound beam direction for velocity measurements, whereas the dotted line shows the beam direction for measurements of diameter change. Both beams were steered to intersect at the center of the range gate. The ultrasound beam steering angle can be changed from −30° to +30° with 5° angular increments.
Figure 1. (A) Movement of the vessel wall is tracked automatically and displayed on M-Mode. Waveforms changes in vessel diameter (distension wave) in real time (left side) and in the right side solid line shows the ultrasound beam direction for velocity measurements, whereas the dotted line shows the beam direction for measurements of diameter change. Both beams were steered to intersect at the center of the range gate. (B) Graphic: Carotid diameter change (top); blood pressure wave and electrocardiographic tracing (bottom). Representative output of carotid stiffness parameters.
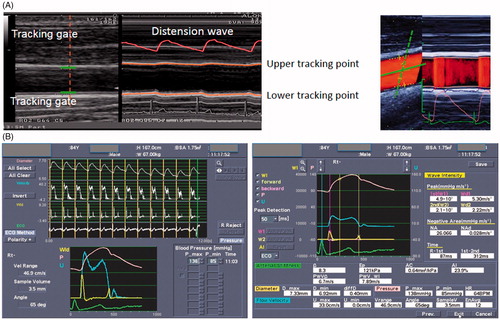
The echo-tracking gates were manually set at the boundaries between the intima and media of the anterior and posterior walls. The rate gate for velocity measurements was automatically positioned at the center of the diameter using echo-tracking gates. Flow velocity was corrected for the angle between the ultrasound beam and the blood flow velocity vector (, panel A). Brachial cuff pressure was measured just before starting ultrasound imaging and was entered into the system for calculation of carotid stiffness indices [Citation21]. At least five consecutive beats were averaged to obtain a representative waveform. The parameters derived from CCA measurements are displayed in , panel B.
The formulas for the calculation of the carotid arterial stiffness were:
Ds and Dd, carotid arterial systolic and diastolic diameter; Ps and Pd, systolic and diastolic brachial pressure (used as a surrogate of carotid systolic and diastolic blood pressure). ρ, blood density (1050 kg/m3).
Inter, intra variability and reputability were previously published (20).
Statistical analysis
Data were expressed as mean ± standard deviation (SD). Simple Pearson correlation was used to examine associations between BP and local stiffness, local and regional stiffness with LV structure and function parameters. The sample was then divided into tertiles according to mean night-time BP as it better correlated with carotid and LV parameters, and ANOVA was used to determine statistical significance between groups. An unpaired t-test was used to compare differences between the two groups of dippers and non-dippers. A stepwise backward multiple linear regression analysis was carried out to weigh the independent effects of age, gender, office HR, physical activity, body mass index (BMI), mean night-time BP, mean day-time BP, mean day–night difference, cfPWV, and local carotid arterial stiffness and compliance parameters on LV structure and function. A second regression analysis was also conducted with carotid stiffness parameters and cfPWV as dependent variables, and age, gender, BMI, physical activity, HR, mean night-time BP, mean day-time BP and mean day–night difference as independent variables. These variables were selected according to their clinical relevance and potential impact on LV structure and function and on arterial stiffness and if they presented a significant value <0.05 in the univariate analysis. In the multivariate linear regression analysis, variable selection was performed using an interactive stepwise backward elimination method. Statistical significance was defined as a p value <.01.
Statistical significance was set at p < .05. All statistical analyses were performed using SYSTAT for Windows release 12.0 (Systat Inc., Chicago, IL, USA).
Results
The general characteristics of the 178 never-treated hypertensive patients with valid 24-hour ABPM (135 men, mean age 50.9 ± 12.3 years) are reported in . The ABPM confirmed high BP values (cut-off values according to the 2013 ESC guidelines: daytime systolic BP ≥135 and/or diastolic BP ≥85 mmHg, night-time systolic BP ≥120 and/or diastolic ≥70 mmHg).
Table 1. General characteristics of the study population (n = 178).
Correlations between different BP measurements (office, ABPM) and one-point carotid stiffness/arterial compliance and cfPWV and BP measurements with LV function and structure are reported in Appendices 1 and respectively. A good correlation was observed between carotid stiffness, cfPWV and BP parameters although it was stronger with AMBP measurements. A good correlation was also found with LV structural variables and AMBP monitoring but not with diastolic function. Correlations between carotid stiffness, cfPWV with LV structure and function are provided in . Arterial stiffness variables correlated with LV parameters but the correlation was stronger for the local stiffness while cfPWV correlated only with E/A ratio (). Mean night-time BP was the parameter that better correlated with the aforementioned target organ indexes, and this association was confirmed when the population was divided into tertiles according to mean night-time BP (). Subjects with higher mean night-time BP were older and had higher LVM, whereas the difference in diastolic function lost statistical significance after adjusting for age, BMI and physical activity. Local carotid arterial stiffness correlated with BP load and, different from local arterial compliance, retained statistical significance also after adjustment for confounders (). The cfPWV increased with BP load increment, though without reaching statistical significance.
Table 2. General characteristics of the hypertensive groups divided in tertiles according to mean night-time BP.
The study population was also divided according to the percent difference in daytime and night-time systolic BP, identifying 18 non-dippers (10.1%) and 124 dippers (91%). The two groups did not differ in age (58.4 ± 18.0 vs 49.6 ± 11.3 years, p = .057), BMI (28.6 ± 4.2 vs 26.7 ± 4.3 kg/m2, p = .09), office diastolic BP (91.2 ± 18.3 vs 90.5 ± 10.9 mmHg, p = .8), HR (71.2 ± 13.1 vs 71.7 ± 14.4 bpm, p = .8), LVM (220.6 ± 77.3 vs 189.5 ± 58.2 g, p = .1), and RWT (38.08 ± 7.2 vs 35.17 ± 5.98, p = .1) but they differed in office systolic BP (165.7 ± 24.6 mmHg vs 151.8 ± 17.4 vs, p = .02) that was higher in non-dippers. ABPM measurements were similar between groups (data show in ) but night-time systolic BP (152.8 ± 23.5 vs 126.2 ± 13.6 mmHg, p < .0001) and night-time diastolic BP (84.6 ± 15.2 vs 75.7 ± 8.9 mmHg, p = .026). Among arterial stiffness parameters, differences were found between groups in local carotid stiffness such as β-index (10.10 ± 4.38 vs 7.50 ± 3.05, p = .024), PWVβ (7.41 ± 1.82 vs 6.43 ± 1.45 m/s, p = .039), pressure-strain elastic modulus (Ep) (164.5 ± 78.05 vs 121.55 ± 67.65 kPa, p = .037) were higher in non-dippers, whereas arterial compliance (AC) (0.59 ± 0.31 vs 0.69 ± 0.25 mm2 kPa, p = .1) was similar among the two groups. Regional cfPWV did not differ between groups (8.71 ± 5.66 vs 7.16 ± 2.74 m/s, p = .3).
A multiple regression analysis was performed to determine the independent effect of age, gender, physical activity, BMI, mean night-time BP, mean day-time BP, mean day–night difference office HR, one-point carotid stiffness parameters and cfPWV on LVM, RWT, transmitral E/A ratio, E/Em ratio and left atrial volume (). Local carotid stiffness parameters, PWVβ and AC and mean day-time BP were independently related to LVM and AC to LA volume. Mean night-time BP was associated to RWT and with age to E/Em. The E/A was mostly related to age and HR. A second multiple regression analysis was conducted to evaluate the independent effect of age, gender, physical activity, BMI, office HR and mean night-time BP mean day-time BP, mean day–night difference on local carotid stiffness variables and cfPWV (). Age, mean night-time BP were constantly related to local carotid arterial stiffness while age was the only variable independently related to cfPWV.
Table 3. Significant independent relation of left ventricular parameters, diastolic function and left atrium with local and regional arterial stiffness, hemodynamic parameters, age and life-style habits.
Table 4. Significant independent relation of local and regional stiffness parameters with hemodynamic parameters, age and life-style habits.
Discussion
The results of the present study show that, in never-treated hypertensive patients where BP load was determined using ABPM, a well known and validated method that provides additional information with respect to office BP measurements and (1) mean night-time BP was found to have a positive correlation not only with LV parameters, as previously described [Citation22], but also with local carotid stiffness indexes. (2) Carotid stiffness was independently related to LVM while it was not the case of cfPWV. (3) The carotid arteries were found to be stiffer in non-dippers than in dippers, while cfPWV and LV structural parameters were similar.
The independent and continuous effect of BP on TOD and its negative impact on the development of cardiovascular events in terms of morbidity and mortality are well recognized [Citation23]. This holds true for all ages and ethnic groups. ABPM correlates more closely than office BP with LV hypertrophy, carotid intima-media thickness, arterial stiffness and other markers of TOD [Citation23], and night-time BP is a stronger predictor than daytime BP [Citation24].
Additional factors other than BP significantly affect the left ventricle, including arterial stiffness. In the 2013 ESC/ESH guidelines [Citation7], a cfPWV cut-off of >10 m/s is reported to be a useful parameter for cardiovascular risk stratification. Arterial stiffening has been recognized as a cardiovascular risk factor associated with increased cardiovascular morbidity and mortality in different clinical scenarios, including coronary artery disease, hypertension, diabetes mellitus, smoking, chronic kidney disease, metabolic syndrome, non-alcoholic fatty liver disease, obstructive sleep apnea, and hyperuricemia [Citation25–30], also in population-based cohorts [Citation2].
The CCA, which is usually considered as part of the central conductance vessels, is another site where stiffness can be easily measured using a high-definition echo-tracking system, with satisfactory inter- and intraobserver reproducibility [Citation20,Citation31]. Available literature data have shown a good correlation between regional and local stiffness indexes [Citation32,Citation33]. However, some authors reported that the correlation between cfPWV and carotid stiffness was weaker as the number of cardiovascular risk factors increased, concluding that regional and local stiffness could provide the same information as the effect of age in normal subjects but not in hypertensive and diabetic patients where the aorta stiffened more than the carotid artery [Citation34,Citation35]. Several studies demonstrated the independent effect of carotid stiffness on the left side of the heart in hypertension. In the CATOD study, which enrolled 314 hypertensive patients, carotid and aortic stiffness were differentially associated with TOD, with cfPWV being related with renal damage and local carotid stiffness with cardiac damage [Citation6]. In addition, both obesity and diabetes had an additive effect on TOD [Citation6]. Gaszner et al. found a significant increase in aortic and local carotid stiffness in patients with documented coronary artery disease [Citation33]. In the study of van Sloten et al. involving a population-based cohort of 579 subjects followed for 7.6 years, carotid and femoral stiffness were independently associated with incident cardiovascular events and all cause-mortality [Citation5]. In 114 patients at risk for heart failure followed for 1 year, Sungh et al. found that CCA measures were significantly higher in patients with LV systolic and diastolic dysfunction, and CCA stiffness indices correlated with LVM index, LV end-diastolic volume index, E/A ratio and E/Em ratio, even after adjustment for confounders, including cfPWV [Citation36]. Moreover, the highest quartile of CCA stiffness parameters and the lowest quartile of arterial compliance were associated with a higher event rate of acute heart failure. No relation between the development of heart failure and cfPWV was found [Citation36].
In the present study, BP load determined by ABPM was directly responsible for LV adaptation, mean day-time for LVM and mean night-time for RWT, which is consistent with previous data from the literature [Citation8–10]. Arterial stiffness was coupled with LV structure, and local carotid stiffness was independently related to LVM and left atrial volume. This stronger correlation with carotid stiffness is likely due to at least two factors: (i) the carotid artery is a short artery with a homogeneous elastic structure unlike the aorta that starts as an elastic artery and ends as a muscular one, and (ii) the ageing process accelerated by high BP is faster at the carotid level than in the aorta and may impose a direct pressure-independent effect on the left ventricle. On the other hand, LV remodeling (RWT) and LV filling pressure (E/Em) were related to night BP load. Our results are in line with previous reports [Citation5,Citation6,Citation33,Citation36,Citation37] where local carotid stiffness is coupled with LV structure and left atrial volume over and above cfPWV and, in the present study, also on top of BP load either as mean day-time or night-time BP as more accurately measured by ABPM.
A blunted reduction in night-time BP as occurs in non-dippers is related to LV hypertrophy and cardiovascular mortality [Citation24,Citation38] as well as to increased arterial stiffness. Cicek et al. compared patients with a non-dipper vs dipper pattern and found that cfPWV measured by SphygmocCor was higher in non-dippers despite the two groups had similar baseline characteristics [Citation39]. In other reports, increased aortic stiffness was documented in patients with metabolic syndrome [Citation40], and increased arterial stiffness as measured by the cardio-ankle vascular index was observed in hypertensive diabetic patients. In our study, the absence of nocturnal dipping was associated with higher carotid stiffness but similar cfPWV and LV adaptation. It may be hypothesized that in never-treated hypertensive patients, with a relatively recent diagnosis of high BP and a low cardiovascular risk burden, local carotid stiffness is the earliest sign of TOD and the carotid artery may be more exposed to atherosclerotic processes [Citation28].
In our study, age was independently and constantly associated with arterial stiffness, whereas mean night-time BP along with HR were a constant, independent variables associated with local carotid stiffness, as previously reported [Citation26]. Also in the early stage of hypertension, HR interferes with arterial stiffness/compliance through a shorter time available for arterial wall recoil, which may result in elastic fiber fracture and pulsating strain cycles. In our opinion, the intrinsic characteristics of the carotid artery (i.e. homogeneity in the elastic properties and higher susceptibility to atherosclerotic burden) combined with the use of the echo-tracking technique that allows more precise measurement of vessel changes during the cardiac cycle than the “foot-to-foot” method via ECG, likely account for the differences observed between the two arterial districts.
Clinical implications
The BP, in particular BP measured by 24-hour ambulatory monitoring and arterial stiffness are cardiovascular risk factors in hypertensive patients. In particular the arterial stiffness allowed improving cardiovascular risk stratification and, in this setting of patients local carotid stiffness was independently related to LVM and in non-dippers it was higher compare to regional or aortic stiffness. Moreover local carotid stiffness can be easily measured by echo-tracking system embedded in a commercially available cardiovascular ultrasound machine that allows to evaluate, during the same examination, two target organs, the carotid stiffness and left ventricular involvement.
Limitations
Several limitations should be acknowledged in this study. First, patients with high BP were aware of their hypertensive status for a maximum of one year but we did not know since how long they were diagnosed with hypertension and consequently the impact of BP duration on vascular stiffness. Second, a limited number of women were included in the study, and the impact of hypertension on TOD in the two genders could not be reasonably explored. Nevertheless, this is illustrative of this never-treated unselected population in which male gender is more prevalent. Third, brachial BP was used for local stiffness parameter calculations instead of central BP that was non available at the time of carotid stiffness measurements (echocardiography + carotid stiffness measurements and BP monitoring + cfPWV were done in different days). Peripheral blood pressure measurements are known to overestimate central blood pressure, especially in young populations but Sugawara et al. [Citation21] demonstrated a good linear relationship between carotid arterial pressure and diameter. And finally we did not have a group of normal subjects as a control group with all these diagnostic tests to compare with the hypertensive group.
Conclusions
To the best of our knowledge this is the first time that local carotid arterial stiffness measured by echo-tracking method provided additive effect over BP measured by 24-h ABPM, considered the most accurate method to evaluate the BP load and was independently associated with LV remodeling, regardless to cfPWV in a group of never-treated hypertensive patients with a low cardiovascular risk burden. Age and mean night-time ambulatory BP were found to be constantly and independently related with arterial stiffness, but the weight of both variables was higher in local carotid stiffness than regional arterial stiffness (cfPWV). Moreover also the non-dipping state was associated with increased local carotid stiffness but not with aortic stiffness. In this clinical setting, it seems that carotid artery is much more sensitive to atherosclerotic process than the aorta and the carotid stiffness, in turn seems to have a greater effect on the LV remodeling.
Disclosure statement
No potential conflict of interest was reported by the authors.
References
- Laurent S, Boutouyrie P, Asmar R, et al. Aortic stiffness is an independent predictor of all-cause and cardiovascular mortality in hypertensive patients. Hypertension. 2001;37:1236–1241.
- Mitchell GF, Hwang SJ, Vasan RS, et al. Arterial stiffness and cardiovascular events: the Framingham Heart Study. Circulation. 2010;121:505–511.
- Chen LY, Leening MJ, Norby FL, et al. Carotid intima-media thickness and arterial stiffness and the risk of atrial fibrillation: the Atherosclerosis Risk in Communities (ARIC) Study, Multi-Ethnic Study of Atherosclerosis (MESA), and the Rotterdam Study. J Am Heart Assoc. 2016;5:e002907.
- Mitchell GF, van Buchem MA, Sigurdsson S, et al. Arterial stiffness, pressure and flow pulsatility and brain structure and function: the Age, Gene/Environment Susceptibility-Reykjavik study. Brain. 2011;134:3398–3407.
- van Sloten TT, Schram MT, van den Hurk K, et al. Local stiffness of the carotid and femoral artery is associated with incident cardiovascular events and all-cause mortality: the Hoorn study. J Am Coll Cardiol. 2014;63:1739–1747.
- Bruno RM, Cartoni G, Stea F, et al. Carotid and aortic stiffness in essential hypertension and their relation with target organ damage: the CATOD study. J Hypertens. 2017;35:310–318.
- Vriz O, Lu H, Visentin P, et al. Gender differences in the relationship between left ventricular size and ambulatory blood pressure in borderline hypertension. The HARVEST Study. Eur Heart J. 1997;18:664–670.
- Andreadis EA, Agaliotis G, Kollias A, et al. Night-time home versus ambulatory blood pressure in determining target organ damage. J Hypertens. 2016;34:438–444.
- Ishikawa J, Hoshide S, Eguchi K, et al. Japan Morning Surge-Home Blood Pressure Study Investigators Group. Night time home blood pressure and the risk of hypertensive target organ damage. Hypertension. 2012;60:921–928.
- Winnicki M, Somers VK, Dorigatti F, et al. Lifestyle, family history and progression of hypertension. J Hypertens. 2006;24:1479–1487.
- Mancia G, Fagard R, Narkiewicz K, et al. 2013 ESH/ESC guidelines for the management of arterial hypertension: the Task Force for the Management of Arterial Hypertension of the European Society of Hypertension (ESH) and of the European Society of Cardiology (ESC). Eur Heart J. 2013;34:2159–2219.
- Vriz O, Bossone E, Bettio M, et al. Carotid artery stiffness and diastolic function in subjects without known cardiovascular disease. J Am Soc Echocardiogr. 2011;24:915–921.
- Palatini P, Penzo M, Canali C, et al. Validation of the accuracy of the A&D TM-2420 model 7 for ambulatory BP monitoring and effect of microphone replacement on its performance. J Ambul Monitor. 1991;4:281–288.
- O'Brien E, Mee F, Atkins N, et al. Accuracy of the Spacelabs 90207 determined by the British Hypertension Society protocol. J Hypertens. 1991;9:573–575.
- Sherwood A, Bower JK, Routledge FS, et al. Nighttime blood pressure dipping in postmenopausal women with coronary heart disease. Am J Hypertens. 2012;25:1077–1082.
- Vriz O, Driussi C, La Carrubba S, et al. Comparison of sequentially measured Aloka echo-tracking one-point pulse wave velocity with SphygmoCor carotid-femoral pulse wave velocity. SAGE Open Med. 2013;1:205031211350756.
- Lang RM, Badano LP, Mor-Avi V, et al. Recommendations for cardiac chamber quantification by echocardiography in adults: an update from the American Society of Echocardiography and the European Association of Cardiovascular Imaging. Eur Heart J Cardiovasc Imaging. 2015;16:233–270.
- Devereux RB, Reichek N. Echocardiographic determination of left ventricular mass in man. Anatomic validation of the method. Circulation. 1977; 55:613–618.
- Nagueh SF, Smiseth OA, Appleton CP, et al. Recommendations for the evaluation of left ventricular diastolic function by echocardiography: an update from the American Society of Echocardiography and the European Association of Cardiovascular Imaging. J Am Soc Echocardiogr. 2016;29:277–314.
- Vriz O, Aboyans V, Minisini R, et al. Reference values of one-point carotid stiffness parameters determined by carotid echo-tracking and brachial pressure in a large population of healthy subjects. Hypertens Res. 2017;40:685–695.
- Sugawara M, Niki K, Furuhata H, et al. Relationship between the pressure and diameter of the carotid artery in humans. Heart Vessels. 2000;15:49–51.
- O’Flynn AM, Dolan E, Curtin RJ, et al. Night-time blood pressure and target organ damage: a comparative analysis of absolute blood pressure and dipping status. J Hypertens. 2015;33:2257–2264.
- Gaborieau V, Delarche N, Gosse P. Ambulatory blood pressure monitoring versus self-measurement of blood pressure at home: correlation with target organ damage. J Hypertens. 2008;26:1919–1927.
- Fagard RH, Celis H, Thijs L, et al. Daytime and night-time blood pressure as predictors of death and cause-specific cardiovascular events in hypertension. Hypertension. 2008;51:55–61.
- Wilkinson I, Cockcroft JR. Cholesterol, lipids and arterial stiffness. Adv Cardiol. 2007;44:261–277.
- Doonan RJ, Hausvater A, Scallan C, et al. The effect of smoking on arterial stiffness. Hypertens Res. 2010;33:398–410.
- Seifalian AM, Filippatos TD, Joshi J, et al. Obesity and arterial compliance alterations. Curr Vasc Pharmacol. 2010;8:155–168.
- Christen AI, Armentano RL, Miranda A, et al. Arterial wall structure and dynamics in type 2 diabetes mellitus: methodological aspects and pathophysiological findings. Curr Diabetes Rev. 2010;6:367–377.
- Phillips CL, Butlin M, Wong KK, et al. Is obstructive sleep apnoea causally related to arterial stiffness? A critical review of the experimental evidence. Sleep Med Rev. 2013;17:7–18.
- Gungor O, Kircelli F, Voroneanu L, et al. Hormones and arterial stiffness in patients with chronic kidney disease. J Atheroscler Thromb. 2013;20:698–707.
- Magda SL, Ciobanu AO, Florescu M, et al. Comparative reproducibility of the non invasive ultrasound methods for the assessment of vascular function. Heart Vessels. 2013;28:143–150.
- Nagai Y, Fleg JL, Kemper MK, et al. Carotid arterial stiffness as a surrogate for aortic stiffness: relationship between carotid artery pressure-strain elastic modulus and aortic pulse wave velocity. Ultrasound Med Biol. 1999;25:181–188.
- Gaszner B, Lenkey Z, Illyes M, et al. Comparison of aortic and carotid arterial stiffness parameters in patients with verified coronary artery disease. Clin Cardiol. 2012;35:26–31.
- Paini A, Boutouyrie P, Calvet D, et al. Carotid and aortic stiffness: determinants of discrepancies. Hypertension. 2006;47:371–376.
- Vermeersch SJ, Rietzschel ER, De Bruyzere ML, et al. Determining carotid artery pressure from scaled diameter waveforms: comparison and validation of calibration techniques in 2026 subjects. Physiol Meas. 2008;29:1267–1280.
- Sung S-H, Liao J-N, Yu W-C, et al. Common carotid artery stiffness is associated with left ventricular structure and function and predicts first hospitalization for acute heart failure. Pulse Karger. 2014;2:18–28.
- Jaroch J, Rzyczkowska B, Bociąga Z, et al. Relationship of carotid arterial functional and structural changes to left atrial volume in untreated hypertension. Acta Cardiol. 2016;71:227–233.
- Cuspidi C, Macca G, Sampieri L, et al. Target organ damage and non-dipping pattern defined by two sessions of ambulatory blood pressure monitoring in recently diagnosed essential hypertensive patients. J Hypertens. 2001;19:1539–1545.
- Cicek Y, Durakoglugil ME, Kocaman SA, et al. Non-dipping pattern in untreated hypertensive patients is related to increased pulse wave velocity independent of raised nocturnal blood pressure. Blood Press. 2013;22:34–38.
- Kuzeytemiz M, Karaagac K, Vatansever F, et al. The effect of non-dipper and dipper blood pressure patterns on aortic elasticity in patients with metabolic syndrome. Clin Exp Hypertens. 2013;35:632–636.
Appendices
Appendix 1. Pearson simple correlation between BPs measurements and carotid and regional stiffness.
Appendix 2. Pearson simple correlation between BPs measurements and left ventricular structure and diastolic function.
Appendix 3. Pearson simple correlation between local and regional stiffness parameters and left ventricular structure and diastolic function.
Appendix 4. Difference in 24 h ambulatory BP parameters between non-dippers and dippers.