Abstract
Background: Oxidative stress and increased cardiovascular reactivity are associated with endothelial dysfunction and cardiovascular disease development. These factors along with early vascular compromise are more pronounced in black populations. We aimed to compare cardiovascular reactivity and investigate associations thereof with oxidative stress in two bi-ethnic cohorts (younger: 25.0 ± 3.19yrs; older: 44.7 ± 9.61yrs).
Methods: Cardiovascular reactivity using the color-word conflict test was measured with the Finometer device. Oxidative stress markers included superoxide dismutase (SOD), γ-glutamyl transferase (γ-GT) and reactive oxygen species (ROS).
Results: Black groups displayed greater cardiovascular responses to stress than white groups. In younger white participants, diastolic blood pressure (DBP) (β = 0.31; p = 0.001) and mean arterial blood pressure (MAP) (β = 0.28; p = 0.002) associated with ROS. In older black participants, DBP (β = 0.23; p = 0.009), MAP (β = 0.18; p = 0.033), stroke volume (β = −0.20; p = 0.023) and arterial compliance (β = −0.25; p = 0.005) associated with γ-GT. In older white participants, systolic blood pressure (β = −0.20; p = 0.006) and MAP (β = −0.19; p = 0.009) associated with SOD.
Conclusions: In the older black group, cardiovascular reactivity associated with markers of glutathione metabolism, suggesting a possible compensatory up-regulation thereof in order to correct their heightened responses to stress. Independent of age, findings in the white groups support a regulatory role of ROS to maintain vascular tone during stress.
Trial registration: ClinicalTrials.gov identifier: NCT03292094.
Background
Cardiovascular disease is a growing concern in the developing world [Citation1,Citation2], with South Africa displaying an alarming increase in the incidence of hypertension, especially amongst urban black populations [Citation3–5]. One of the key role players in the development of hypertension is oxidative stress, which occurs due to either an increased production of reactive oxygen species (ROS), or a decrease in ROS scavenging by the antioxidant system [Citation6]. Physiologically, ROS play an important role in vascular biology and cell signaling as it is involved in control of vascular tone and endothelial function, cell growth, differentiation and apoptosis [Citation6–9]. However, oxidative stress aggravates endothelial dysfunction, vascular remodeling and inflammation which may result in elevated blood pressure, peripheral resistance, arterial stiffness and atherosclerosis [Citation6,Citation7,Citation9], all of which worsen with aging [Citation10,Citation11]. Acute stress has also been associated with both endothelial dysfunction as well as oxidative stress [Citation12,Citation13], and a heightened cardiovascular response to stress is a risk factor for the development of cardiovascular disease, including hypertension [Citation14–16]. Upon acute stress, black populations have shown increased cardiovascular reactivity when compared to white populations [Citation17–19]. Black South Africans are also more prone to early vascular changes [Citation20], and previous results linked increased blood pressure to oxidative stress [Citation21,Citation22]. However, it has not been previously investigated whether oxidative stress plays a role in a heightened cardiovascular response in black and white South Africans, and whether this link is age dependent. Therefore we aimed to compare oxidative stress and cardiovascular reactivity profiles between two age-stratified South African cohorts, and to determine whether relationships exist between cardiovascular reactivity and oxidative stress markers.
Methods
Study populations
This study is embedded in the baseline phases of the African Prospective study on the Early Detection and Identification of Cardiovascular disease and Hypertension (African-PREDICT) study (mean age: 25.0 years) and the Sympathetic activity and Ambulatory Blood Pressure in Africans (SABPA) study (mean age: 44.7 years).
After an initial screening phase (evaluating blood pressure, cholesterol, glucose levels, anthropometry, HIV status and a general health questionnaire) apparently healthy normotensive or pre-hypertensive (systolic blood pressure (SBP) <140 and diastolic blood pressure (DBP) <90mmHg) black and white men and women between 20 and 30 years of age were invited to participate in the African-PREDICT study [Citation23]. Participants who are not permanent residents of Potchefstroom or surrounding areas (or plan regular trips to the area) and who are unable to read or understand English were excluded from the study. Participants with elevated glucose levels >5.6 mmol/L (or glycated haemoglobin ≥6.5%), who were HIV infected and those with ear temperature >37.5 °C were also excluded. Further exclusion criteria included previous diagnoses of (or took medication for) type 1 or 2 diabetes mellitus, liver disease, cancer, tuberculosis, renal or cardiovascular diseases, who recently had surgery or trauma and who was pregnant or breastfeeding. This study included the first 387 participants with complete antioxidant enzyme activity data divided into black (N = 191) and white groups (N = 196).
Detail on the SABPA study was published elsewhere [Citation24]. In summary, participants were between 20 and 65 years of age, and participants who were pregnant, lactating, exhibited ear temperature >37 °C, used alpha and beta blockers, confirmed psychotropic substance abuse and those who donated blood or were vaccinated 3 months prior to their participation were excluded. We included 409 participants of the SABPA study divided into black (N = 200) and white (N = 209) groups.
Both studies complied with the Declaration of Helsinki regarding investigations among human participants, and were further approved by the Health Research Ethics Committee of the North-West University. The African-Predict study was registered as a clinical trial as required (ClinicalTrials.gov Identifier: NCT03292094). All participants were informed of each study in their home language, including stressor test protocols, and written informed consent was obtained before commencement.
Questionnaires
Participants completed a general health questionnaire to obtain demographic data and information on alcohol usage.
Anthropometric and physical activity measurements
All anthropometric measurements were performed using standardized methods [Citation25]. These measurements included body height measured with a stadiometer (SECA 213 SECA, Hamburg, Germany and Invicta, IP 1465, Invicta, London, UK), body weight measured with an electronic scale (SECA 813, SECA, Hamburg, Germany and Precision Health Scale, A & D Company, Tokyo, Japan) and waist circumference measured with a metal tape (Lufkin Steel Anthropometric Tape, W606PM, Lufkin, Apex, MD, USA and Holtain unstretchable flexible 7mm wide metal tape, Crosswell, Wales). Body mass index (BMI) was calculated as kg/m2 and body surface area was calculated as m2 according to the Mosteller formula [Citation26]. Total energy expenditure (TEE) was monitored over 7 days in the African-PREDICT study (ActiHeart physical activity monitor, CamNtech Ltd., England, UK) and over 24 hours in the SABPA study (Actical® activity monitor, Mini Mitter Co., Inc., Bend, OR; Montreal, Quebec, Canada).
Cardiovascular reactivity
Cardiovascular measurements were made non-invasively using the validated Finometer device (FMS, Finapres Medical Systems, Amsterdam, Netherlands). The Finometer device provides a beat-to-beat arterial blood pressure waveform after adjusting for hydrostatic height from the heart while computing additional cardiac parameters by way of the Modelflow method [Citation27]. The finger cuff of the Finometer was fitted on the left middle-finger, and after a 10 minute resting period, a 5 minute continuous measurement of resting variables was recorded.
During the recording, after 2 minutes, a return-to-flow systolic calibration was performed to provide an individual subject-level adjustment of the finger arterial pressure with the brachial artery pressure, which allows for the highest precision in measurements. Resting measurements included SBP, DBP, mean arterial pressure (MAP), stroke volume (SV), total peripheral resistance (TPR) and Windkessel arterial compliance (Cwk). Thereafter the color-word conflict test was applied for 1 minute during which the cardiovascular variables were measured. The mean of the last minute of the resting values, and the last 20 seconds during exposure were used for the calculations. Cardiovascular reactivity was calculated as the percentage change from resting values [Citation28].
Biochemical measurements
Fasting blood samples were obtained from each participant by a registered nurse from the antebrachial vein. Serum and plasma samples were prepared using standardized procedures, aliquoted into cryovials and stored in bio-freezers at −80 °C until analysis to maintain efficient long-term stability of antioxidant enzymes [Citation29]. Antioxidant enzyme activities measured included glutathione reductase (GR), glutathione peroxidase (GPx), superoxide dismutase (SOD) and γ-glutamyl transferase (γ-GT). Additional oxidative stress markers included total glutathione (tGSH) and reactive oxygen species (ROS) (measured as serum peroxides and reported in units, where 1 mg H2O2/L equates to 1 unit)[Citation30]. In addition, fluoride plasma glucose and serum total cholesterol, triglycerides, cotinine, interleukin-6 (IL-6) and cortisol levels were determined. All apparatus used are summarized in Table S1.
Statistical analyses
Statistica version 13.2 (Dell, TX, USA) was used to perform the statistical analyses of this study. Normal distribution of data was assessed and the central tendency and spread for normally distributed variables were expressed as arithmetic mean and standard deviation. Skewed variables were logarithmically transformed (triglycerides, IL-6, total cholesterol, SOD and γ-GT in the African-PREDICT study and glucose, triglycerides, IL-6, GR, SOD and γ-GT in the SABPA study) and expressed as the geometric mean and the 5th and 95th percentile intervals.
Interactions of race and sex were tested on the relationships between main cardiovascular reactivity variables and oxidative stress markers using multiple regression analyses. Continuous variables were compared using independent T-tests, while proportions were compared using Chi-square tests. Single regression analyses were performed to evaluate unadjusted associations between cardiovascular variables and oxidative stress related variables. Thereafter, partial correlations were performed to evaluate the associations between variables while adjusting for age, sex and BMI. Multiple regression analyses were performed to evaluate the independent associations between cardiovascular reactivity variables and oxidative stress markers. Covariates entered into the models included the relative oxidative stress marker, age, sex, BMI, TEE, self-reported alcohol use, cotinine, glucose, IL-6, triglycerides and cortisol. Sensitivity analyses were performed by repeating the multiple regression analyses after including statin usage and anti-hypertension medication usage into the models.
In post hoc analyses the achieved power (1−β) was determined for multiple regression models with 11 covariates, with a group size of N = 191 for black (1−β = 0.97) and N = 196 for white African-PREDICT (1−β = 0.97), and N = 200 for black (1−β = 0.97) and N = 209 for white SABPA (1−β = 0.98) (G*power v3.1.9.2) [Citation31].
Results
Characteristics of the study population
No interactions of sex on the relationships between cardiovascular reactivity and oxidative stress markers were found. Meanwhile, interactions of race were found on the association of Cwk with SOD (β = 0.285; p = 0.026) in the African-PREDICT study, and on the associations of SBP with SOD (β = −0.477; p = 0.018), MAP with SOD (β = −0.578; p = 0.004) and TPR with tGSH (β = 0.635; p = 0.015) in the SABPA study. Stratification was done accordingly to compare black and white groups.
Black participants of the African-PREDICT study were younger (24.4 ± 3.39; p < 0.001) with a lower BMI (24.5 ± 5.41; p = 0.013) than their white counterparts, while in the SABPA study the black participants displayed a higher BMI (30.1 ± 7.00; p < 0.001) than the white group ().
Table 1. Characteristics of black and white groups.
Oxidative stress markers including ROS, tGSH, GPx and γ-GT revealed similar profiles in both studies with black participants having higher ROS (African-PREDICT: 197 ± 70.9; p = 0.033; SABPA: 192 ± 63.0; p < 0.001), tGSH (African-PREDICT: 1231 ± 295; p < 0.001; SABPA: 895 ± 189; p < 0.001) and γ-GT (African-PREDICT: 25.4 (10.6; 82.8); p < 0.001; SABPA: 47.4 (20.1; 184; p < 0.001) with lower GPx activity (African-PREDICT: 18.5 ± 1.76; p < 0.010; SABPA: 33.3 ± 14.0; p = 0.010) than their white counterparts. Although no differences were found in the African-PREDICT study, GR activity was higher in the black participants of the SABPA study (7.06 (2.55; 16.8); p < 0.001).
Cardiovascular reactivity
In the younger cohort, black participants displayed higher DBP, MAP and TPR but lower SV and Cwk at rest and after acute stress when compared to the younger white participants (). Similarly, black participants of the older cohort also displayed higher DBP and MAP with lower Cwk at rest and after acute stress than the white participants. Additionally, this group also displayed higher SBP at rest and after acute stress along with a higher TPR than their white counterparts after acute stress only.
Figure 1. Rest and change in cardiovascular variables after the color-word conflict test showing significant differences between black and white groups of the African-PREDICT and SABPA studies.
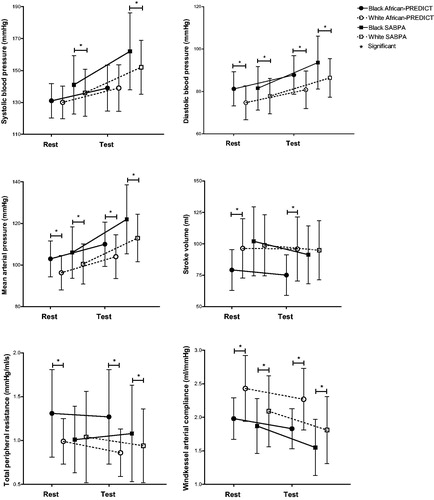
In the younger black participants, a larger decrease in SV (p < 0.001) and smaller decrease in TPR (<0.001) were noted in comparison to the younger white participants (). In the older black group, cardiovascular reactivity after applying the stressor revealed greater increases in DBP (p = 0.002), and MAP (p = 0.031) than their white counterparts. When comparing change in TPR the older black group experienced an increase in TRP whereas the white group experienced a decrease in TPR, and these responses differed significantly between the two groups (p = 0.014). The older black group also showed a significantly larger decrease in Cwk (p = 0.001) and SV (p < 0.001) when compared to their white counterparts.
Regression analyses
In single and partial regression analyses (Table S2), we found an association of change in TPR with SOD in the young black group, however, this lost significance after full adjustments were made (R2 = −0.004; β = −0.12; p = 0.145). In the younger white group, changes in DBP (R2 = 0.05; β = 0.31; p = 0.001) and MAP (R2 = 0.10; β = 0.28; p = 0.002) associated positively with ROS, while change in Cwk associated positively with SOD activity (R2 = 0.09; β = 0.18; p = 0.023) ().
Table 2. Summary of multiple regression analyses of cardiovascular reactivity with markers of oxidative stress in participants of the African-PREDICT and SABPA studies.
In the older black group, single, partial (Table S3) and multiple regression analyses () indicated an increase in DBP (R2 = 0.03; β = 0.23; p = 0.009) and MAP (R2 = 0.06; β = 0.18; p = 0.033) to be positively associated with γ-GT levels. Meanwhile, a decrease in SV (R2 = 0.06; β = −0.20; p = 0.023) and Cwk (R2 = 0.07; β = −0.25; p = 0.005) was negatively associated with γ-GT levels. In the same group, an increase in TPR was borderline negatively associated with tGSH (R2 = 0.04; β = −0.15; p = 0.062). The borderline association of decreased SV with GPx in partial regression analyses (p = 0.054) became significant after multiple regression analyses (R2 = 0.06; β = 0.16; p = 0.032) in the older black group. In the older white group, increased SBP (R2 = 0.04; β = −0.20; p = 0.006), increased MAP (R2 = 0.01; β = −0.19; p = 0.009) were inversely associated with SOD. In the same group a decrease in SV was positively associated with tGSH (R2 = 0.02; β = 0.20; p = 0.009) and negatively associated with SOD (R2 = 0.0004; β = −0.14; p = 0.052). All other associations lost significance after full adjustments.
Sensitivity analyses
The African-PREDICT study excluded participants using statins and anti-hypertension medication. After adding these variables as covariates into multiple regression analyses in the SABPA study, only the borderline negative association of TPR with tGSH in the black group became significant after including anti-hypertension medication into the model (R2 = 0.05; β = −0.17; p = 0.039).
Multiple regression analyses of SV with oxidative stress markers in the older group were repeated after substituting stroke volume with stroke volume index (SVI) (calculated as stroke volume/body surface area) as the main dependent variable in the model. Similar results were obtained in sensitivity analyses showing a significant negative association of SVI with γ-GT (R2 = 0.06; β = −0.20; p = 0.023) in black groups, and a positive association of SVI with tGSH (R2 = 0.02; β = 0.20; p = 0.009) in white groups. The negative association of SVI with SOD (R2 = 0.0004; β = −0.14; p = 0.052) was borderline significant in the older white group after full adjustments.
Discussion
We found age-related disparities in the link between cardiovascular reactivity and markers of oxidative stress in black populations. In the young black group TPR decreased less and SV decreased more, but we found no link between cardiovascular reactivity and markers of oxidative stress. In the older black group with a heightened cardiovascular response to stress, increased TPR and decreased SV and Cwk was associated with markers of glutathione metabolism. In contrast, our findings in the white groups indicated that cardiovascular reactivity was associated with ROS and important determinants of ROS availability, namely SOD and tGSH, independent of age.
Our findings in the older black group confirm results from a previous study by our research team, in which older black men displayed increased TPR reactivity to acute stress [Citation18]. Increased TPR reactivity may enhance the afterload and subsequently decrease SV, which related to end-organ damage in this cohort [Citation18]. Similar findings were noted in the younger black adults described in the present study, where TPR decreased less and SV decreased more when compared to the white adults during stress. This is in agreement with previous findings in another young black group (29.9 ± 2.4 years) where it was suggested that increased TPR may play a role in the pathogenesis of hypertension in blacks [Citation19]. Despite evidence of an altered cardiovascular response in the young black group, we were unable to link this to any oxidative stress markers investigated in this study. This finding may suggest that factors other than ROS, such as enhanced arterial stiffness and premature vascular aging, may play a more prominent role in cardiovascular reactivity in young black South Africans [Citation20,Citation32].
In the older black group, on the other hand, increased TPR and decreased SV and Cwk were associated with markers of glutathione metabolism. Glutathione, a powerful antioxidant, exists in either reduced (GSH) or oxidized (GSSG) form [Citation33]. The synthesis of glutathione is dependent on the intracellular availability of its amino acid constituents. While γ-GT was originally considered a marker for alcohol abuse and liver dysfunction, the enzyme acts as an oxidative stress marker since it is involved in the breakdown of extracellular glutathione to increase the availability of amino acids for intracellular glutathione synthesis [Citation34]. In this regard γ-GT has a dual function since it is also able to produce ROS directly [Citation35–38]. The link between heightened cardiovascular reactivity and markers of glutathione metabolism may be explained by two possible mechanisms; firstly that acute stress may induce increased shear stress which may promote oxidative stress [Citation12,Citation39], and secondly that oxidative stress may leads to a deficiency in nitric oxide, thus further diminishing endothelial function [Citation6,Citation7,Citation40]. During conditions of oxidative stress, glutathione may be consumed at a higher rate, leading to possible increased expression of γ-GT in an effort to increase intracellular glutathione levels [Citation36]. The elevated γ-GT in this group may then also perhaps be explained as compensatory in an effort to stabilize the redox balance, which is further strengthened by the presence of higher tGSH in this group.
In contrast, the findings in the white cohorts indicated that cardiovascular reactivity was associated with ROS and important determinants of ROS availability, namely SOD and tGSH, independent of age. This was also indicated in an experimental study in which a role for ROS in the regulation of blood pressure during and after acute stress was confirmed. Here it was suggested that a lack of ROS is implicated in a greater MAP reactivity and a longer recovery period from acute stress [Citation41]. This finding supports the important role of physiologically regulated ROS signaling in the maintenance of vascular tone during stress, while suggesting a possible role for an adequate antioxidant system, especially SOD activity, in the prevention of a heightened cardiovascular response to stress in white participants regardless of age.
This study has to be interpreted within the context of its strengths and limitations. Both our study populations included participants from the Potchefstroom area, and may not represent the South African population as a whole. This being a cross-sectional study, causality cannot be inferred, and although our results were consistent after multiple adjustments, we cannot exclude confounding of unknown factors. Measurements of ROS were made using an indirect biomarker and we cannot discern the sources of ROS generation in this study. Participants were not screened or excluded based on previous exposure to the color-word conflict test, which may be considered for future studies in this field. However, to the best of our knowledge, this is the first study investigating the associations of oxidative stress with cardiovascular reactivity in 2 age-stratified cohorts of black and white South Africans. Both studies were well executed under controlled conditions in a fully-equipped research facility. This study offers further insight into the role of redox signaling in cardiovascular responses to acute stress.
In conclusion, age-related differences were found in the link between cardiovascular reactivity and markers of oxidative stress in the black cohorts. Heightened cardiovascular reactivity during the color-word conflict test associated with markers of glutathione metabolism in older black participants, suggesting a possible compensatory up-regulation of this system in order to correct their unfavourable cardiovascular responses to stress. Findings in the white groups support a possible regulatory role of ROS in the maintenance of vascular tone during stress, independent of age.
Table_S3_Regressions_SABPA..doc
Download MS Word (58 KB)Table_S2_Regressions_African-PREDICT..doc
Download MS Word (52.5 KB)Table_S1_Apparatus_used_during_the_studies..doc
Download MS Word (50.5 KB)Acknowledgements
The authors are grateful towards all individuals participating voluntarily in the study. The dedication of the support and research staff as well as students at the Hypertension Research and Training Clinic at the North-West University are also duly acknowledged.
Disclosure statement
The authors have no conflicts of interest to disclose. Any opinions expressed and conclusions arrived at, are those of the authors alone.
Additional information
Funding
References
- Addo J, Smeeth L, Leon DA. Hypertension in sub-saharan africa: a systematic review. Hypertension. 2007;50:1012–1018.
- Ogah OS, Rayner BL. Recent advances in hypertension in sub-saharan africa. Heart. 2013;99:1390–1397.
- Schutte AE, Schutte R, Huisman HW, et al. Are behavioural risk factors to be blamed for the conversion from optimal blood pressure to hypertensive status in black south africans? A 5-year prospective study. Int J Epidemiol. 2012;41:1114–1123.
- Opie LH, Seedat YK. Hypertension in sub-saharan african populations. Circulation 2005;112:3562–3568.
- Kandala N, Tigbe W, Manda SO, et al. Geographic variation of hypertension in sub-saharan africa: a case study of South Africa. Am J Hypertens. 2013;26:382–391.
- Montezano AC, Dulak-Lis M, Tsiropoulou S, et al. Oxidative stress and human hypertension: vascular mechanisms, biomarkers, and novel therapies. Can J Cardiol. 2015;31:631–641.
- Touyz RM, Briones AM. Reactive oxygen species and vascular biology: implications in human hypertension. Hypertens Res. 2011;34:5–14.
- Touyz R, Schiffrin E. Reactive oxygen species in vascular biology: implications in hypertension. Histochem Cell Biol. 2004;122:339–352.
- Briones AM, Touyz RM. Oxidative stress and hypertension: current concepts. Curr Hypertens Rep. 2010;12:135–142.
- Higashi Y, Kihara Y, Noma K. Endothelial dysfunction and hypertension in aging. Hypertens Res. 2012;35:1039–1047.
- Wang JC, Bennett M. Aging and atherosclerosis: mechanisms, functional consequences, and potential therapeutics for cellular senescence. Circ Res. 2012;111:245–259.
- Huang CJ, Webb HE, Zourdos MC, et al. Cardiovascular reactivity, stress, and physical activity. Front Physiol 2013;4:314.
- Ghiadoni L, Donald AE, Cropley M, et al. Mental stress induces transient endothelial dysfunction in humans. Circulation 2000;102:2473–2478.
- Treiber FA, Kamarck T, Schneiderman N, et al. Cardiovascular reactivity and development of preclinical and clinical disease states. Psychosom Med. 2003;65:46–62.
- Chida Y, Steptoe A. Greater cardiovascular responses to laboratory mental stress are associated with poor subsequent cardiovascular risk status: a meta-analysis of prospective evidence. Hypertension 2010;55:1026–1032.
- Fonkoue IT, Carter JR. Sympathetic neural reactivity to mental stress in humans: test-retest reproducibility. Am J Physiol Regul Integr Comp Physiol. 2015;309:R1380–R1386.
- Reimann M, Hamer M, Schlaich M, et al. Autonomic responses to stress in black versus caucasian africans: the SABPA study. Psychophysiology 2012;49:454–461.
- Huisman HW, Schutte AE, Schutte R, et al. Exploring the link between cardiovascular reactivity and end-organ damage in african and caucasian men: the SABPA study. Am J Hypertens. 2013;26:68–75.
- Stein CM, Lang CC, Singh I, et al. Increased vascular adrenergic vasoconstriction and decreased vasodilation in blacks. additive mechanisms leading to enhanced vascular reactivity. Hypertension 2000;36:945–951.
- Schutte AE, Huisman HW, Schutte R, et al. Arterial stiffness profiles: investigating various sections of the arterial tree of african and caucasian people. Clin Exp Hypertens. 2011;33:511–517.
- Kruger R, Schutte R, Huisman H, et al. Associations between reactive oxygen species, blood pressure and arterial stiffness in black South Africans: the SABPA study. J Hum Hypertens. 2012;26:91–97.
- Van Zyl C, Huisman HW, Mels CM. Antioxidant enzyme activity is associated with blood pressure and carotid intima media thickness in black men and women: the SABPA study. Atherosclerosis 2016;248:91–96.
- Schutte AE, Gona PN, Delles C, et al. The African prospective study on the early detection and identification of cardiovascular disease and hypertension (African-PREDICT): design, recruitment and initial examination. Eur J Prev Cardiol. 2019;26:458–470.
- Malan L, Hamer M, Frasure-Smith N, et al. Cohort profile: sympathetic activity and Ambulatory Blood Pressure in Africans (SABPA) prospective cohort study. Int J Epidemiol. 2015;44:1814–1822.
- Marfell-Jones MJ, Stewart A, de Ridder J. International standards for anthropometric assessment. 2012. Wellington (New Zealand): International Society for the Advancement of Kinanthropometry.
- Verbraecken J, Van de Heyning P, De Backer W, et al. Body surface area in normal-weight, overweight, and obese adults. A comparison study. Metab Clin Exp. 2006;55:515–524.
- Imholz BP, Wieling W, van Montfrans GA, et al. Fifteen years experience with finger arterial pressure monitoring: assessment of the technology. Cardiovasc Res. 1998;38:605–616.
- Kamarck TW, Lovallo WR. Cardiovascular reactivity to psychological challenge: conceptual and measurement considerations. Psychosom Med. 2003;65:9–21.
- Abiaka C, Al-Awadi F, Olusi S. Effect of prolonged storage on the activities of superoxide dismutase, glutathione reductase, and glutathione peroxidase. Clin Chem. 2000;46:560–576.
- Hayashi I, Morishita Y, Imai K, et al. High-throughput spectrophotometric assay of reactive oxygen species in serum. Mutat Res Genet Toxicol Environ Mutagen. 2007;631:55–61.
- Faul F, Erdfelder E, Lang A, et al. G* power 3: a flexible statistical power analysis program for the social, behavioral, and biomedical sciences. Behav Res Methods. 2007;39:175–191.
- Matthews KA, Katholi CR, McCreath H, et al. Blood pressure reactivity to psychological stress predicts hypertension in the CARDIA study. Circulation 2004;110:74–78.
- Townsend DM, Tew KD, Tapiero H. The importance of glutathione in human disease. Biomed Pharmacother. 2003;57:145–155.
- Mason JE, Starke RD, Van Kirk JE. Gamma-glutamyl transferase: a novel cardiovascular risk biomarker. Prev Cardiol. 2010;13:36–41.
- Lim J, Yang J, Chun B, et al. Is serum γ-glutamyltransferase inversely associated with serum antioxidants as a marker of oxidative stress? Free Radic Biol Med. 2004;37:1018–1023.
- Grundy SM. Gamma-glutamyl transferase: another biomarker for metabolic syndrome and cardiovascular risk. ATVB 2007;27:4–7.
- Lee DS, Evans JC, Robins SJ, et al. Gamma glutamyl transferase and metabolic syndrome, cardiovascular disease, and mortality risk: the framingham heart study. Arterioscler Thromb Vasc Biol. 2007;27:127–133.
- Lee D, Blomhoff R, Jacobs DR. Review is serum gamma glutamyltransferase a marker of oxidative stress? Free Radic Res. 2004;38:535–539.
- Griendling KK, FitzGerald GA. Oxidative stress and cardiovascular injury: part I: basic mechanisms and in vivo monitoring of ROS. Circulation 2003;108:1912–1916.
- Bagi Z, Cseko C, Toth E, et al. Oxidative stress-induced dysregulation of arteriolar wall shear stress and blood pressure in hyperhomocysteinemia is prevented by chronic vitamin C treatment. Am J Physiol Heart Circ Physiol. 2003;285:H2277–H2283.
- Bernátová I, Balis P, Goga R, et al. Lack of reactive oxygen species deteriorates blood pressure regulation in acute stress. Physiol Res. 2016;65:S381.