Abstract
Purpose: Baroreflex emerges as a therapeutic target of hypertension. We investigated blood pressure (BP) lowering effect of the combined passive head-up and -down movement with device-guided slow breathing in untreated mild hypertension or high-normal BP.
Methods: In a randomized, cross-over trial, untreated subjects with an ambulatory systolic/diastolic BP of 125–140/80–90 mmHg and a clinic BP of 130–150/80–90 mmHg were randomized to intervention treatment with head movement and slow breathing or sham control, and then crossed over. Both treatments consisted of 1-week preparation, 2-week treatment, and 1-week recovery. During the 2-week treatment, subjects were treated for a session of 20 min/day. BP, pulse rate and respiration were measured before and after each treatment session. Ambulatory BP monitoring was performed at baseline and the end of the 2-week treatments’ period, and home BP monitoring in the morning and evening for the whole 8-week follow-up period.
Results: 14 subjects completed the study. The intervention treatment, compared to control, reduced respiration rate by −2.1 breaths/min (95% CI −2.9 to −1.2, p = .0001), but not clinic BP and pulse rate (p ≥ .67). The intervention treatment, compared to control, significantly reduced nighttime systolic/diastolic blood pressure by −5.63/−3.82 mm Hg (p ≤ .01) but not 24-h or daytime ambulatory blood pressure (p ≥ .69). Home BP decreased with the intervention treatment, but the between-treatment difference was not statistically significant (p ≥ .27).
Conclusions: The combined head movement with slow breathing did not influence 24-h BP, but reduced nighttime BP in untreated mild hypertension or high-normal BP.
Introduction
Baroreflex sensitivity plays a crucial role in blood pressure regulation [Citation1]. In the presence of impaired baroreflex sensitivity, the level and variability of blood pressure [Citation2,Citation3] and the prevalence of orthostatic hypotension increase in adults [Citation4,Citation5] as well as children and adolescents [Citation6]. Baroreflex is recently emerging as a therapeutic target of hypertension. Baroreflex activation by either slow breathing [Citation7,Citation8] or direct stimulation of carotid sinus [Citation9] may decrease sympathetic activity and blood pressure [Citation10]. In several randomized controlled trials, slow breathing for 10–15 minutes per day reduced systolic/diastolic blood pressure by 7–15/4–10 mm Hg [Citation11–13]. In a scientific statement of the American Heart Association, the device-guided slow breathing was recommended as a non-pharmacological treatment for high blood pressure [Citation14].
With the development of implanted devices, direct stimulation of carotid sinus is possible, and has been demonstrated effective in lowering blood pressure in patients with resistant hypertension [Citation15]. However, such device, because of its invasive nature, is difficult to be applied widely in the management of hypertension. Passive head-up and -down movement or slow breathing is probably more feasible for possible baroreflex activation and blood pressure lowering [Citation16,Citation17]. In the present randomized cross-over study, we investigated the chronic blood pressure lowering effect of the combined passive head-up and -down movement with device-guided slowing breathing in subjects with untreated mild hypertension or high-normal blood pressure.
Methods
General study design
The present study was designed as a single-center, randomized, cross-over trial to test blood pressure lowering efficacy of the combined passive head-up and -down movement with device-guided slow breathing in subjects with untreated mild hypertension or high-normal blood pressure. Eligible participants were randomized to receive intervention treatment and then sham control treatment, or vice versa (). Each randomized treatment period consisted sequentially of a preceding one-week of preparation, two weeks of intervention or sham control treatment and a succeeding one-week of recovery.
Ambulatory blood pressure monitoring was performed at baseline for the determination of eligibility and at the end of the intervention and sham control treatment periods for the evaluation of therapeutic effect. Clinic blood pressure was measured at screening and before and after each session of the intervention or sham control treatment inside the hospital. Pulse rate and respiration were measured in parallel with the clinic blood pressure measurement. Home blood pressure monitoring was performed in the morning and evening during the preparation, treatment and recovery periods of the intervention and sham control treatments.
The Ethics Committee of Ruijin Hospital, Shanghai Jiaotong University School of Medicine, Shanghai, approved the study protocol. All participants gave written informed consent.
Study population
The study subjects were recruited in a specialized hypertension outpatient clinic in Ruijin Hospital, Shanghai Jiaotong University School of Medicine, Shanghai, China, from September 2016 to May 2017. Men and women in the age range from 35 to 70 years were considered for inclusion if at a screening visit they did not take any antihypertensive medication for at least two weeks and had a 24-h ambulatory blood pressure in the range from 125 mm Hg to 140 mm Hg systolic or from 80 mm Hg to 90 mm Hg diastolic and a clinic blood pressure in the range from 130 to 150 mm Hg systolic or from 80 to 90 mm Hg diastolic. Subjects were excluded if they had a body mass index of ≥35kg/m2 or an otolithic disease (e.g. vertigo or Meniere’s disease) or had known symptomatic carotid artery stenosis, renal failure or thyroid disease.
Randomized treatment
During the two-week intervention or sham control treatment period, the study subjects received 20-min treatment daily in the afternoon while lying down in a bed in a quiet room inside the hospital. Intervention treatment consisted of passive head-up and -down movement with an inflation and deflation pillow and device-guided slow breathing with a headphone. For the sham control treatment, the study subjects similarly lay down with a non-moving pillow and a sound proof headphone.
Intervention treatment was administered with a multisensory stimulation system (a prototype manufactured by Philips Research, Eindhoven, The Netherlands) that consists of two belt-type respiration-movement sensors (Alice PDx, Philips) mounted on the chest and upper abdomen, an inflatable head pillow, a cough assist (CA-E70, Philips) and a set of headphones. A computer program runs the whole system to adjust the breathing guidance rate to less than 10 breaths per minute, and synchronously to drive the cough assist for inflation and deflation of the pillow and to play rhythmic wave sounds on the headphone. The period of one inflation-deflation cycle of the pillow is exactly matching the sound of waves. A trained investigator measured the resting respiration rate of the subject in the supine position before and after the treatment period and ran the program to control the frequency of sounds in the headphone to guide the subjects to slow their breathing rate.
Efficacy and safety evaluations
The primary efficacy variable was the change in 24-h ambulatory systolic blood pressure from baseline to the end of two-week treatment. Secondary efficacy variables included changes in 24-h ambulatory diastolic blood pressure and heart rate and daytime and nighttime ambulatory systolic and diastolic blood pressure and heart rate from baseline to the end of the two-week treatment, home systolic and diastolic blood pressure and heart rate from the preparation to the treatment and recovery periods, and clinic systolic and diastolic blood pressure, heart rate and respiration from before to after each session of treatment inside the hospital.
Safety evaluations included adverse events and serious adverse events, including any clinically significant abnormalities on physical examination. Information about symptoms, severity, relation to the study intervention and outcome were documented for all adverse events.
Measurements of ambulatory, home and clinic blood pressure and pulse rate and respiration
Ambulatory blood pressure monitors (SpaceLabs 90207, SpaceLabs Inc) were programmed to obtain blood pressure and pulse rate readings every 20 minutes in the daytime (06:00–22:00) and 30 minutes at night (22:00–06:00). A recording was considered valid if it had 20 readings in the daytime and 10 readings at night, based on the requirement to have at least 70% of measurements being obtained at least every 30 min, or more frequently, throughout the entire 24-h period [Citation18]. In the analysis, we defined daytime and nighttime according to the ambulatory blood pressure monitoring diary.
Clinic and home blood pressure and pulse rate were measured with a validated automated electronic blood pressure monitor (HEM-7081, Omron Healthcare, Kyoto, Japan). On each occasion, blood pressure and pulse rate were measured three times consecutively with a 1 minute interval, after the subject had rested in the seated or supine position for at least 5 minutes.
Respiration was counted with the belt-type respiration-movement sensors mounted on the chest and abdomen at the time of clinic blood pressure measurement.
Sample size estimation and statistical analysis
Sample size was estimated on the basis of an α level of 0.05 and 80% of power. We hypothesized a decrease of 4 mm Hg ambulatory systolic blood pressure at the end of treatment. The sample size was estimated to be 12. To provide better confidence, we finally decided to recruit 14 subjects with complete follow-up.
The SAS software (version 9.2, SAS Institute, Cary, N.C., USA) was used for database management and statistical analysis. Analysis of covariance was performed to compute the least square mean change from baseline with standard error and the between-treatment difference with 95% confidence intervals (CI) with the baseline value as covariate and treatment as a factor. The Fisher exact test was used to compute the risk ratio with 95% CI.
Results
Characteristics of the study subjects
Of the 18 randomized subjects, 4 withdrew from the trial before cross-over, leaving 14 completed the trial and included in the final analysis. None of the randomized subjects had adverse or serious adverse event during the trial. The 14 subjects included 6 men and 8 women, and had a mean age of 51.9 ± 10.5 years, a mean body mass index of 24.7 ± 3.2 kg/m2, and a mean clinic systolic/diastolic blood pressure of 136.4/81.0 mm Hg (). The 24-h, daytime and nighttime ambulatory systolic/diastolic blood pressures at entry were 129.9/84.8 mm Hg, 134.1/88.2 mm Hg and 121.5/78.0 mm Hg, respectively.
Table 1. Characteristics of the study subjects at baseline (n = 14).
Acute effect of treatment on respiration and clinic blood pressure and pulse rate
The intervention treatment significantly slowed respiration in comparison with the sham control treatment, with a between-treatment mean difference of −2.1 breaths/min (95% CI, −2.9 to −1.2; p = .0001). The intervention treatment did not significantly influence clinic blood pressure and pulse rate in comparison with the sham control treatment (P ≥ 0.67, ; ).
Figure 2. Mean values of pulse rate and respiration before and after the intervention (dot with solid line) or sham control treatment session (circle with dashed line) in the clinic. Vertical lines denote standard deviation. P values are given for the within-treatment before and after treatment comparison and for the between-treatment comparison of the changes from before to after treatment.
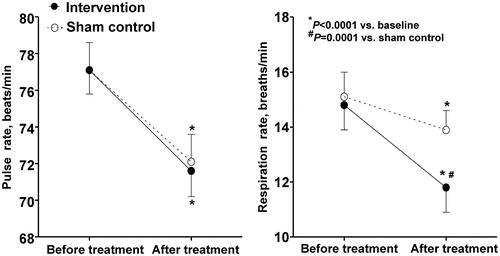
Table 2. Mean level and difference for respiration, clinic and ambulatory blood pressure between treatments.
Treatment effect on ambulatory blood pressure and pulse rate
No significant between-treatment difference was observed on 24-h systolic blood pressure, the primary efficacy variable, nor 24-h diastolic or daytime systolic and diastolic blood pressure (p ≥ .69). However, the intervention treatment significantly reduced nighttime systolic and diastolic blood pressure, in comparison with the sham control treatment, with a between-treatment mean difference of −5.63 mm Hg (95% CI, −8.53 to −2.73) and −3.82 mm Hg (−6.72 to −0.93), respectively (). Accordingly, the intervention treatment, compared with the sham control, significantly increased the nocturnal systolic blood pressure fall and decreased the nigh-to-day systolic blood pressure ratio, with a between-treatment mean difference of 5.03 mm Hg (95% CI, 0.98–9.08 mm Hg; p = .02) and −4.0% (95% CI, −6.7% to −1.2%; p = .009), respectively (; ). Pulse rate was not influenced either in the daytime or at night.
Follow-up of home blood pressure and pulse rate
The mean between-treatment difference in morning and evening home blood pressure did not reach statistical significance at the end of the two-week treatment and one week post-treatment recovery periods in a size from 0.22 to 1.67 mm Hg (p ≥ .27, ). Pulse rate was significantly and similarly increased by a mean of 2.26 beats/min (p = .03) and 2.67 beats/min (p = .008) on the intervention compared with sham control treatment in the morning and evening during the 2-week treatment period. The corresponding differences in the recovery period were not significant (p ≥ .26, ).
Table 3. Mean level and difference of morning and evening home blood pressure and pulse rate during the 2-week treatment and 1-week post-treatment recovery periods between treatments.
Further analysis showed that home systolic/diastolic blood pressure changed from the preparation period to the treatment and post-treatment recovery periods by a maximum decrease of −1.5/−2.0 mm Hg on the intervention treatment and a maximum increase of 1.3/1.1 mm Hg on the sham control treatment (p ≥ .06 for the between-treatment comparisons, ).
Figure 4. Least square mean change from the preparation period in the average of morning and evening home blood pressures during the treatment and recovery periods on the intervention (dot with solid line) and sham control treatments (circle with dashed line). Vertical lines denote standard error. The smallest P value is given for the between-treatment comparison alongside the symbol of the follow-up time point.
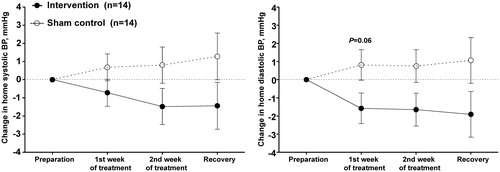
Disscussion
Our study demonstrated that the combined head-up and -down movement with device-guided slow breathing significantly reduced respiration rate acutely by 2 breaths/min and nighttime ambulatory blood pressure chronically by approximately 6 mm Hg systolic and 4 mm Hg diastolic. Home blood pressure showed a trend of decrease in a size up to 2–3 mm Hg systolic and diastolic. If confirmed in a multicenter study and on a large scale, this size of treatment effect may have clinical implications for the management of hypertension.
Our study is the first that has investigated short-term chronic effect of head-up and -down movement on ambulatory and home blood pressure, although the effect of the head-up or head-down position on various pathophysiological conditions has been studied previously [Citation19–22]. The results of these studies suggest that passive head-up or head-down may have modulating effect on sympathetic or parasympathetic activity. However, our study did not include a group of head movement only, nor involve direct or indirect measures of sympathetic or parasympathetic activity, except the rough measure, pulse rate. We could not attribute the observed blood pressure lowering efficacy solely to the passive head-up and -down movement.
The observed significant slowing in respiration may be partly attributable to the effect of the device-guided slow breathing therapy. However, only 6 of the 14 subjects on intervention treatment reached the target of <10 breaths/min of breathing as defined in guidelines [Citation14]. Nonetheless, the observed changes in respiration may contribute to the blood pressure changes during follow-up. Several previous slow breathing studies involved ambulatory [Citation23–28] or home [Citation11,Citation13,Citation23,Citation29] blood pressure monitoring either at entry for the selection of subjects or during follow-up for the therapeutic monitoring. On average, respiration was consistently reduced by 4.3 [Citation29] to 6.9 breaths/min [Citation27]. However, blood pressure was reduced in some [Citation11,Citation13,Citation23–25,Citation29] but not in other studies [Citation26–28]. On the two extremes, 24-h ambulatory and home blood pressures were reduced by 7.2/2.3 and 5.8/3.0 mm Hg, respectively, in an 8-week trial [Citation23], but ambulatory blood pressure was not influenced by slow breathing therapy in several other randomized controlled trials [Citation26–28]. Critics raised the issue of manufacturers’ involvement in early trials that reported positive results [Citation30,Citation31]. However, several other factors may also play a part, such as the adherence to treatment [Citation13], duration of treatment [Citation32], and so on.
Why the reduction in nighttime ambulatory blood pressure is most prominent remains unexplained. One explanation could be that the treatment in the present study was applied in the evening, and hence had a greater effect at night than in the daytime. However, we did not observe any acute effect on clinic blood pressure, whereas the home blood pressure lowering effect remained during the post-treatment recovery period. An alternative explanation therefore could be that the sympatho-vagal imbalance in our study subjects with early blood pressure elevation is more prominent at night than in the daytime. In this case, a sympatho-vagal modulating therapy may be more efficacious on nighttime than daytime blood pressure.
The results of our randomized cross-over study, though interesting, have to be interpreted within the context of their limitations. First, our study was a small-size single-center trial. One physician performed clinical examinations in collaboration with a technician who operated the technical procedure of treatment. To consolidate the methodology, our efficacy evaluations relied on ambulatory and home blood pressure monitoring. Second, as mentioned above, our study did not include groups of monotherapy with the head movement or slow breathing only. We therefore cannot differentiate treatment effects between the two interventions. Third, a 2.6 mm Hg of difference in 24-h systolic blood pressure with an 8 mm Hg of standard deviation was observed at the end of treatment. According to these observations and similar statistical assumptions as the sample size estimation of our study, the statistical power substantially reduced to 36%.
Perspectives
Treatment with the passive head movement and slow breathing devices is feasible and may be efficacious in lowering blood pressure. Before these prototypes are considered for further development, multicenter, randomized, parallel-group comparison trials should be conducted on various clinical conditions. Resistant hypertension can be a target of research. It is possible that in patients with resistant hypertension, blood pressure reductions would be even more significant. If more evidence is available, the up and down moving inflatable pillow with or without the slow breathing device can be developed into an effective and useful home healthcare device for the long-term management of hypertension.
Compliance with ethical standards
The study protocol was approved by the Ethics Committee of Ruijin Hospital, Shanghai Jiaotong University School of Medicine, Shanghai, China.
Conflicts of interest and source of funding
Philips Research China (Shanghai, China) provided funding for this study.
Dr. Ji-Guang Wang was financially supported by grants from the National Natural Science Foundation of China [grants 81170245 and 91639203], Beijing and the Shanghai Commission of Health and Family Planning (a special grant for “leading academics”), Shanghai, China, and reported receiving lecture and consulting fees from Daiichi-Sankyo, Novartis, Omron, Pfizer, Sanofi and Servier. For the other authors, there are no conflicts of interest.
Acknowledgement
The authors gratefully acknowledge the voluntary contribution of the study subjects.
References
- Ketch T, Biaggioni I, Robertson R, et al. Four faces of baroreflex failure: hypertensive crisis, volatile hypertension, orthostatic tachycardia, and malignant vagotonia. Circulation 2002;105:2518–2523.
- Honzikova N, Zavodna E. Baroreflex sensitivity in children and adolescents: physiology, hypertension, obesity, diabetes mellitus. Physiol Res. 2016;65:879–889.
- Floyd CN, Adeel MY, Wolff CB, et al. First-in-man treatment of severe blood pressure variability with baroreflex activation therapy. Int J Cardiol. 2016;220:577–579.
- Tonkin AL, Wing LM, Morris MJ, et al. Afferent baroreflex dysfunction and age-related orthostatic hypotension. Clin Sci. 1991;81:531–538.
- Blaho A, Sutovsky S, Valkovic P, et al. Decreased baroreflex sensitivity in Parkinson's disease is associated with orthostatic hypotension. J Neurol Sci. 2017;377:207–211.
- Fitzgibbon LK, Coverdale NS, Phillips AA, et al. The association between baroreflex sensitivity and blood pressure in children. Appl Physiol Nutr Metab. 2012;37:301–307.
- Bernardi L, Porta C, Spicuzza L, et al. Slow breathing increases arterial baroreflex sensitivity in patients with chronic heart failure. Circulation. 2002;105:143–145.
- Oneda B, Ortega KC, Gusmao JL, et al. Sympathetic nerve activity is decreased during device-guided slow breathing. Hypertens Res. 2010;33:708–712.
- Papademetriou V, Doumas M, Faselis C, et al. Carotid baroreceptor stimulation for the treatment of resistant hypertension. Int J Hypertens. 2011;2011:1.
- Kougias P, Weakley SM, Yao Q, et al. Arterial baroreceptors in the management of systemic hypertension. Med Sci Monit. 2010;16:A1–A8.
- Grossman E, Grossman A, Schein MH, et al. Breathing-control lowers blood pressure. J Hum Hypertens. 2001;15:263–269.
- Schein MH, Gavish B, Herz M, et al. Treating hypertension with a device that slows and regularises breathing: a randomised, double-blind controlled study. J Hum Hypertens. 2001;15:271–278.
- Elliot WJ, Izzo JJ, White WB, et al. Graded blood pressure reduction in hypertensive outpatients associated with use of a device to assist with slow breathing. J Clin Hypertens (Greenwich). 2004;6:553–559, 560–561.
- Brook RD, Appel LJ, Rubenfire M, et al. Beyond medications and diet: alternative approaches to lowering blood pressure: a scientific statement from the American Heart Association. Hypertension. 2013;61:1360–1383.
- Bilgutay AM, Lillehei CW. Surgical treatment of hypertension with reference to baropacing. Am J Cardiol. 1966;17:663–667.
- Bjurstedt H, Rosenhamer G, Tyden G. Cardiovascular responses to changes in carotid sinus transmural pressure in man. Acta Physiol Scand. 1975;94:497–505.
- Mancia G, Ferrari A, Gregorini L, et al. Circulatory reflexes from carotid and extracarotid baroreceptor areas in man. Circ Res. 1977;41:309–315.
- O'Brien E, Asmar R, Beilin L, et al. European Society of Hypertension recommendations for conventional, ambulatory and home blood pressure measurement. J Hypertens. 2003;21:821–848.
- Vardan S, Hill NE, Mehrotra KG, et al. Baroreflex function during 45-degree passive head-up tilt before and after long-term thiazide therapy in the elderly with systolic systemic hypertension. Am J Cardiol. 1994;73:253–257.
- Yokoi Y, Aoki K. Relationship between blood pressure and heart-rate variability during graded head-up tilt. Acta Physiol Scand. 1999;165:155–161.
- Chandler MP, Mathias CJ. Haemodynamic responses during head-up tilt and tilt reversal in two groups with chronic autonomic failure: pure autonomic failure and multiple system atrophy. J Neurol. 2002;249:542–548.
- Tikkakoski AJ, Tahvanainen AM, Leskinen MH, et al. Hemodynamic alterations in hypertensive patients at rest and during passive head-up tilt. J Hypertens. 2013;31:906–915.
- Rosenthal T, Alter A, Peleg E, et al. Device-guided breathing exercises reduce blood pressure: ambulatory and home measurements. Am J Hypertens. 2001;14:74–76.
- Howorka K, Pumprla J, Tamm J, et al. Effects of guided breathing on blood pressure and heart rate variability in hypertensive diabetic patients. Auton Neurosci. 2013;179:131–137.
- Modesti PA, Ferrari A, Bazzini C, et al. Psychological predictors of the antihypertensive effects of music-guided slow breathing. J Hypertens. 2010;28:1097–1103.
- Anderson DE, McNeely JD, Windham BG. Regular slow-breathing exercise effects on blood pressure and breathing patterns at rest. J Hum Hypertens. 2010;24:807–813.
- Hering D, Kucharska W, Kara T, et al. Effects of acute and long-term slow breathing exercise on muscle sympathetic nerve activity in untreated male patients with hypertension. J Hypertens. 2013;31:739–746.
- de Barros S, da Silva GV, de Gusmão JL, et al. Effects of long term device-guided slow breathing on sympathetic nervous activity in hypertensive patients: a randomized open-label clinical trial. Blood Press. 2017;26:359–365.
- Meles E, Giannattasio C, Failla M, et al. Nonpharmacologic treatment of hypertension by respiratory exercise in the home setting. Am J Hypertens. 2004;17:370–374.
- Mahtani KR, Nunan D, Heneghan CJ. Device-guided breathing exercises in the control of human blood pressure: systematic review and meta-analysis. J Hypertens. 2012;30:852–860.
- Landman GW, van Hateren KJ, van Dijk PR, et al. Efficacy of device-guided breathing for hypertension in blinded, randomized, active-controlled trials: a meta-analysis of individual patient data. JAMA Intern Med. 2014;174:1815–1821.
- Modesti PA, Ferrari A, Bazzini C, et al. Time sequence of autonomic changes induced by daily slow-breathing sessions. Clin Auton Res. 2015;25:95–104.