Abstract
Objective: In a randomised controlled trial, we investigated the blood pressure (BP) lowering effect of continuous positive airway pressure (CPAP) in patients with moderate-severe obstructive sleep apnoea syndrome (OSAS, an apnoea-hypopnoea index, AHI of 15 or higher) and nocturnal hypertension (night-time systolic/diastolic BP ≥120/70 mmHg).
Methods: Sixty patients were randomly assigned to CPAP or sham CPAP, while maintaining their antihypertensive treatment. Ambulatory BP monitoring was performed at baseline (first run-in visit) and the end of follow-up. Clinic and home BP were measured at baseline and each of the monthly follow-up visits.
Results: Of the 60 patients, 47 completed the 3-month study. CPAP (n = 26), compared with sham CPAP (n = 21), slightly and non-significantly reduced 24-h systolic/diastolic BP by −2.8/−2.5 mmHg (p ≥ 0.27), with a slightly greater between-group difference in the daytime (−4.0/−2.8 mmHg, p ≥ 0.29) than night-time (−0.2/−1.5 mmHg, p ≥ 0.50). The CPAP treatment did not significantly influence clinic or home BP during follow-up (p ≥ 0.27). Nonetheless, simple and partial correlation analyses showed that the ambulatory BP lowering effect was dependent on the daytime pulse rate at baseline (r ≥ 0.47, p ≤ 0.01). In patients with a daytime pulse rate greater than 85 beats/min, the mean changes in daytime systolic BP were significantly greater in the CPAP (n = 10) than sham CPAP group (n = 11), with a between-group mean difference of −10.1 mmHg (p = 0.048).
Conclusions: The CPAP treatment did not show significant ambulatory BP lowering effect in patients with moderate-severe OSAS and nocturnal hypertension. However, it may be effective in lowering daytime BP in patients with a faster pulse rate.
Introduction
Most of the current hypertension guidelines consider obstructive sleep apnoea syndrome (OSAS) as a common secondary cause of hypertension [Citation1,Citation2]. Continuous positive airway pressure (CPAP) is a proven effective treatment for ameliorating symptoms of OSAS, such as daytime sleepiness [Citation3]. However, in keeping with the negative results of the recent sleep trials for cardiovascular prevention [Citation4,Citation5], CPAP only had moderate blood pressure lowering effect in patients with OSAS and with or without hypertension [Citation6–8]. One of the speculative explanations is that by treating apnoea, hypopnoea, and hypoxaemia, CPAP is probably only effective in lowering blood pressure in a subgroup of patients, for instance, those with OSAS as a cause, instead of, a sole comorbidity of hypertension.
We and others previously found that nocturnal hypoxaemia, as assessed by oxygen desaturation index, was more closely associated with night-time than daytime blood pressure [Citation9,Citation10]. We therefore hypothesised that CPAP might be more efficacious in lowering blood pressure in patients with nocturnal hypertension, and designed a randomised controlled trial to test the feasibility of treating nocturnal hypertension with CPAP. In the present analysis of this single-center feasibility study, we investigated the ambulatory and home blood pressure lowering effect of CPAP in patients with moderate and severe OSAS and nocturnal hypertension and aimed to identify possible subgroups of hypertensive patients who would be sufficiently responsive to the CPAP treatment.
Methods
Study design
The study was a randomised, prospective, single-blind, parallel group trial conducted from November 2014 to October 2016 in Ruijin Hospital in Shanghai, China. The study complied with the International Conference on Harmonisation Guidelines for Good Clinical Practice local regulations and the ethical principles of the Declaration of Helsinki. The study protocol was approved by the Ethics Committee of Ruijin Hospital, Shanghai Jiaotong University School of Medicine, Shanghai, China.
If at a screening clinic visit, a patient had moderate-severe OSAS diagnosed by full polysomnography and gave written informed consent, he or she was invited for the first run-in clinic visit (baseline) for clinical examinations and determination of eligibility. To exclude those patients who would be unwilling or unlikely to adhere to CPAP therapy, clinically eligible patients entered a 1-week sham CPAP (fixed pressure of 4 cm H2O) tolerance period as we did in a previous trial with a slightly modified protocol [Citation4,Citation11]. Those tolerant to the CPAP treatment (defined as the treatment time with CPAP higher than 2 h/night and 3 nights/week) were randomly assigned to the CPAP treatment or sham CPAP control group, and blinded to their treatment. We titrated CPAP using auto-CPAP (REMstar Auto A-Flex 557 P, Philips Respironics). The fixed pressure was maintained throughout the study in those patients assigned to the CPAP treatment group. Patients assigned to the sham CPAP control received the fixed pressure of 4 cm H2O. We assessed compliance with CPAP (both CPAP and sham CPAP) using the internal clock of the device. The device in the study (REMstar Auto A-Flex 557 P) was supplied free of charge for the whole study period by Philips Respironics. All patients were followed-up at 1, 2 and 3 months of treatment for efficacy and safety evaluations. Unless clinically indicated, patients were instructed at baseline to the end of follow-up not to start antihypertensive drug treatment if previously untreated, and not to change the use of antihypertensive medication if previously treated.
Study population
Potential eligible patients were men and women in the age range from 18 to 75 years and with moderate or severe OSAS (apnoea-hypopnoea index, AHI ≥ 15 events/h) and nocturnal hypertension (night-time systolic blood pressure ≥120 mmHg or diastolic blood pressure ≥70 mmHg). To be eligible for inclusion, a patient had to be tolerant to the CPAP treatment, defined as the treatment time with CPAP higher than 2 h/night and 3 nights/week.
Exclusion criteria included secondary hypertension, severe hypertension (clinic systolic blood pressure ≥180 mmHg or diastolic blood pressure ≥110 mmHg), previous or current use of CPAP or other treatments for OSAS, any contraindication to CPAP, insomnia, and other severe sleep disorders, unable to give informed consent due to cognitive impairment, having a plan of pregnancy or surgery, and incompliance to CPAP.
Efficacy and safety evaluations
The primary efficacy variable was the change from baseline in 24-h ambulatory blood pressure at the end of the 3 months’ treatment. Secondary efficacy variables included systolic and diastolic blood pressure changes from baseline in the daytime and night-time ambulatory blood pressure at the end of the 3 months’ treatment and clinic and home blood pressure at each of the clinic follow-up visits.
Safety evaluations included collection of information on all adverse events and serious adverse events.
Sleep evaluations
Full overnight polysomnography (Philips Respironics, Alice 6) was performed in a sleep laboratory according to the international recommendations [Citation12]. Sleep stages, arousal, oxygen saturation, apnoeas, and hypopnoea events were scored according to the standard criteria [Citation13]. We defined an apnoea event as a > 90% reduction of airflow of at least 10 s and a hypopnoea event as any discernible reduction in airflow (around 50%) for at least 10 s, along with a drop in oxygen saturation of more than 3% (oxygen desaturation event), or an electroencephalographic arousal, or both. AHI was calculated by dividing the total number of apnoea and hypopnoea events by total sleep time in hours. Oxygen desaturation index (ODI) was calculated by dividing the total number of oxygen desaturation events by total sleep time in hours. Obstructive and central apnoeas were distinguished according to the respiratory effort channels (presence or absence of a thoracoabdominal movement during the breathing cessation). A polysomnography recording was considered valid if the total sleep time was longer than 180 min.
Blood pressure measurements
Clinic blood pressure was measured three times consecutively at baseline and at each of the follow-up visits using a validated automated oscillometric blood pressure monitor (Omron HEM-7011-C1, Omron Healthcare, Kyoto, Japan), after the patients had rested for at least 5 min in the sitting position. A cuff with an appropriately sized bladder was used. These three blood pressure readings were averaged for analysis.
Ambulatory blood pressure monitoring was performed at baseline and after 3 months of treatment. We programmed validated oscillometric SpaceLabs 90217 monitors (SpaceLabs, Redmond, Washington) to obtain ambulatory blood pressure readings at 20-min intervals from 06:00 to 22:00 and at 30-min intervals from 22:00 to 06:00. Patients were instructed to complete a diary card, and recorded the sleep and wake up time for the definition of daytime and night-time. A recording was considered valid and included in the analysis, if it included ≥70% of the programmed readings and had at least 20 and 7 blood pressure readings in the daytime and night-time, respectively [Citation14]. Within individual subjects, we weighted the means of the ambulatory blood pressure level by the time interval between successive readings. Ambulatory pulse rate was derived from the same ambulatory blood pressure recording.
Home blood pressure monitoring was performed at baseline and at each of the follow-up visits using the Omron HEM-7080-C1 monitor. At the study entry, patients were instructed for the procedure of home blood pressure measurement. The patients were advised to measure their blood pressure at home three times consecutively every morning and evening, respectively, for seven consecutive days after the first run-in visit and before every clinic visit during follow-up, after at least 5 min of rest in the sitting position. A pause of 30–60 s was allowed between two successive measurements. The patients were also instructed to place an appropriately sized cuff directly on their non-dominant arm and keep the position of the cuff at the level of the heart. Morning blood pressure was measured before breakfast and drug intake (if treated) and within 1 h from waking up, and evening blood pressure at least 2 h after dinner, after shower or bath and drug intake (if treated), and before going to bed. The blood pressure and pulse rate readings in the morning and evening sessions were averaged separately and together for analysis.
Statistical analysis
Sample size estimation of our study was based on the assumptions of a between-group mean 24-h systolic blood pressure difference of ≥10 mmHg in favour of the CPAP treatment, an α level of 5%, and a power of 80%. The number of patients per group was estimated to be 28. Assuming a drop-out rate of 10%, approximately 30 patients per group would be required.
For database management and statistical analysis, we used SAS software (version 9.2, SAS Institute, Cary, NC, USA). Means and proportions were compared by the Student’s t-test and the χ2 test, respectively. The changes from baseline in blood pressure and pulse rate were computed by subtracting the values at the first run-in clinic visit from those at each of the follow-up visits. Analysis of covariance was performed to compute the least square mean changes from baseline for the CPAP treatment or sham CPAP control group and the between-group mean difference (95% confidence interval) with the baseline value as a covariate and treatment as a factor. Simple and partial correlation analyses were performed for the changes in 24-h systolic/diastolic blood pressure in relation to sex and age, body mass index, AHI, ODI, pulse rate and blood pressure at baseline by treatment group. All tests were two-sided and a p value < 0.05 was considered to be statistically significant.
Results
Characteristics of the randomized patients
Of the 260 screened patients with moderate to severe OSAS, 120 patients were potentially eligible for inclusion and entered the 1-week CPAP tolerance period. Of these 120 patients, 60 (50%) patients were tolerant to the CPAP treatment and randomised to the CPAP (n = 30) and sham CPAP control groups (n = 30). The baseline characteristics were comparable between the CPAP and sham CPAP control groups ( and ).
Table 1. Baseline characteristics of the participants who completed study.
Table 2. Clinic, ambulatory and home blood pressure and pulse rate at baseline.
Of the randomised patients, 26 (86.7%) in the CPAP group and 21 (70%) in the sham CPAP control group completed the study (p = 0.21, ). During follow-up, the use of CPAP and sham CPAP was on average 4.9 and 4.1 h/night, respectively, and at least 4 h/night in 18 patients (69.2%) and 10 patients (47.6%), respectively. The mean (±SD) CPAP pressure used in the CPAP group was 11.6 (±2.7) cm H2O. Adverse events were reported in 7 (23.3%, rhinobyon 2, eye discomfort 1, premature heartbeat 1, psychological stress 1, retinal detachment 1, and tinnitus 1, respectively) and 5 (16.7%, chest stress 1, common cold 1, gout 1, pneumonia 1, and sinusitis 1, respectively) in the CPAP and sham CPAP groups, respectively (p = 0.52). Of these 7 and 5 patients with adverse events, 1 and 2, respectively, withdrew from the trial (). No serious adverse event was reported in either group.
Treatment effect on blood pressure
The CPAP treatment, compared with the sham CPAP control, slightly and non-significantly reduced 24-h ambulatory systolic and diastolic blood pressure, with a between-group mean difference (95% confidence interval) of −2.8 mmHg (−10.0 to 4.4, p = 0.44) and −2.5 mm Hg (−7.0 to 2.0, p = 0.27), respectively (). The treatment effect was not significant (p ≥ 0.29) either for daytime or night-time ambulatory blood pressure, despite that the blood pressure reductions were slightly greater for daytime than for night-time ( and ).
Figure 2. Changes in daytime ambulatory blood pressure at 12 weeks of treatment from baseline (first run-in clinic visit) by treatment group. The bars represent median and interquartile range. Negative values indicate a decrease from baseline.
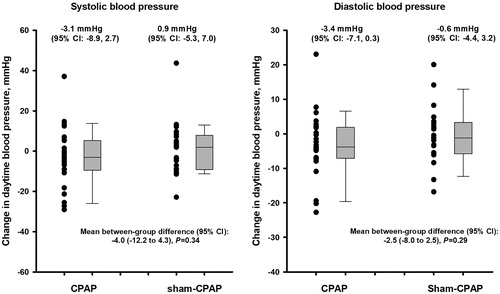
Table 3. Mean change in ambulatory blood pressure at 3 months of CPAP treatment from baseline.
The CPAP treatment did not significantly influence clinic or home blood pressure at the end of the 3 months’ treatment (p ≥ 0.27, ). Nonetheless, CPAP, but not the sham CPAP, significantly (p ≤ 0.03) reduced home systolic/diastolic blood pressure in the morning at 1 and 2 months of follow-up from baseline by a mean change (±standard error) of 3.6 ± 0.9/2.8 ± 0.7 mmHg and 3.1 ± 1.4/3.2 ± 1.1 mmHg, respectively, despite that the between-group difference did not reach statistical significance (p = 0.05–0.80, ).
Figure 3. Clinic and home blood pressure at baseline and at 1, 2 and 3 months of follow-up in the CPAP (dot with solid line) and sham CPAP groups (circle with dashed line). Symbols indicate mean values of blood pressure. *p < 0.05 vs baseline.
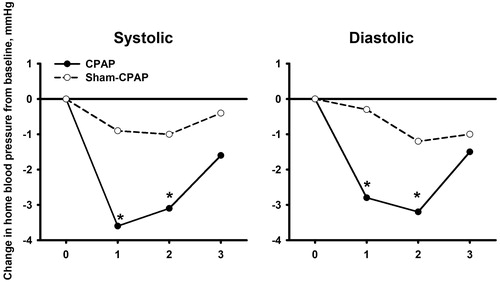
In analyses restricted to those treated with the CPAP (n = 18) or sham CPAP (n = 10) for at least 4 h/night, the between-group mean differences in systolic/diastolic blood pressure were −0.3/−1.8, −0.9/−1.8, and 1.4/−1.6 mm Hg, respectively, for the 24-h, daytime and night-time ambulatory measurement at 3 months of follow-up (p ≥ 0.36) and −2.3 to 1.2/−3.6 to −1.6 mm Hg, respectively, for the home measurement at 1, 2 and 3 months of follow-up (p ≥ 0.26).
Determinants of changes in daytime ambulatory blood pressure
In both simple and partial (for sex and age) correlation analyses, the changes in daytime systolic (r ≥ 0.47, p ≤ 0.01) and diastolic blood pressure (r ≥ 0.37, p ≤ 0.07) in the CPAP treatment group were dependent on daytime blood pressure and daytime pulse rate at baseline (). Indeed, the mean changes in daytime systolic blood pressure were significantly greater in patients with a daytime pulse rate of ≥85 beats/min (above the 84.6 beats/min of mean daytime pulse rate in the CPAP treatment group, n = 10) than those with a daytime pulse rate of <85 beats/min (n = 16), with a mean difference of −13.6 mm Hg (95% confidence interval −24.4 to −2.8 mm Hg, p = 0.016, ). Similar trends were observed for daytime diastolic blood pressure, with a corresponding mean difference of −6.4 mm Hg (95% confidence interval −13.8 to +0.9 mm Hg, p = 0.08).
Figure 4. Change in daytime systolic blood pressure in relation to daytime pulse rate (PR) at baseline in the CPAP (dot with solid line) and sham CPAP groups (circle with dashed line).
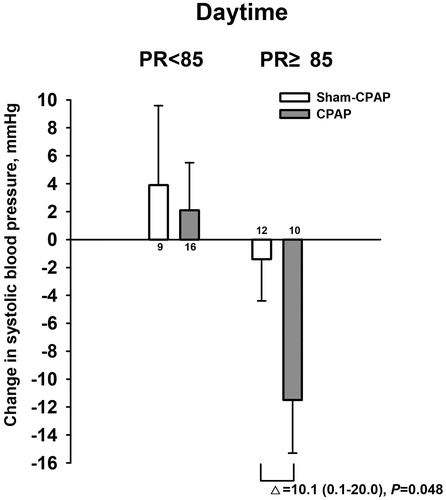
Table 4. Correlates of the changes in daytime systolic and diastolic blood pressure.
Discussion
Our study in patients with moderate-to-severe OSAS and nocturnal hypertension showed similar ambulatory blood pressure lowering effect of CPAP as those previous studies in patients with clinic hypertension, being in a size of 2–3 mmHg after 3 months of treatment. In addition, our study showed that CPAP had a greater daytime ambulatory blood pressure lowering effect in patients with a faster pulse rate.
Our study did not show significant effect on the primary efficacy variable, 24-h ambulatory systolic blood pressure, because the observed effect was far below the expected 10 mm Hg. In fact, the observed effect was virtually similar to the blood pressure reductions in previous studies in patients not selected by night-time ambulatory blood pressure [Citation6–8,Citation14]. Our study with the present sample size therefore had insufficient power to show a significant blood pressure lowering effect. The use of 24-h instead of night-time blood pressure as the primary efficacy variable probably did not account for the negative results of our study, because the observed between-group difference was greater for daytime than for night-time ambulatory blood pressure. Nocturnal hypertension is closely related to OSAS [Citation10], but is probably not a good predictor of treatment effect of CPAP.
Our study showed in post-hoc analysis that the blood pressure lowering effect was dependent on pulse rate at baseline. In those with a faster heart rate, CPAP significantly reduced ambulatory blood pressure. This observation is physiologically possible, because fast heart rate is a rough measure of sympathetic activation. In a recent 6-month CPAP treatment study in patients with OSAS (n = 60), Castro-Grattoni et al reported that night-time heart rate significantly predicted ambulatory blood pressure response during CPAP treatment [Citation15]. In addition, we found in a large number of subjects that the association between oxygen desaturation and high ambulatory blood pressure was mediated by fast heart rate [Citation10]. Pulse rate therefore might have to be included as an inclusion criterion for the recruitment of patients in future blood pressure lowering trials with CPAP in OSAS. However, in our study this observation on ambulatory daytime recording was not replicated on home morning blood pressure, although the latter was also slightly reduced by CPAP. The mechanism for this discrepancy remains under investigation. A possible explanation could be that daytime ambulatory recording improved accuracy of measurement for both blood pressure and pulse rate by covering a much longer daytime period and hence the power of the study in the detection of possible treatment effect. Nonetheless, if our observation would be confirmed in future randomised controlled trials, CPAP may become an effective treatment for hypertension in patients with OSAS and faster heart rate. Indeed, a heart rate of 80 beats/min or faster was recently recommended by the European hypertension guidelines as a risk factor for risk stratification in the management of hypertension [Citation2].
Our study specifically investigated ambulatory blood pressure lowering effect of CPAP in patients with OSAS and nocturnal hypertension. However, our study did not show preferential blood pressure lowering effect at night. This finding may be a chance one because of small sample size. Nonetheless, similar results were observed in a study of 40 patients with resistant hypertension [Citation16]. In this previous randomised controlled trial, medical therapy plus CPAP, compared with medical therapy only, reduced daytime awake and night-time asleep systolic/diastolic blood pressure by 3.4/2.4 mmHg and −1.2/−1.0 mmHg, respectively. The results of our research and this previous study suggest the complexity in the pathogenesis of night-time blood pressure elevation beyond OSAS.
Our observation on the more prominent home blood pressure lowering during earlier than later follow-up is in keeping with the results of a previous study [Citation13]. In this particular study, 340 patients with OSAS were randomised to 3-months’ treatment of CPAP or sham CPAP. CPAP reduced blood pressure greater in the early (6 weeks) than in the late (12 weeks) months of follow-up. Several reasons may explain why blood pressure lowering effect diminishes with time of follow-up. First, with the early blood pressure reductions, treated patients may stop or reduce their antihypertensive medication. Second, patient compliance with CPAP may also decrease with time of follow-up in some of the randomised patients. Finally, it is also possible that the treatment effect is reduced by counter-regulatory mechanisms.
We also observed a greater home blood pressure lowering effect of CPAP in the morning than evening. Previous studies did show a close relationship between OSAS and blood pressure in the morning [Citation17,Citation18]. A possible underlying mechanism might be that CPAP, by treating sleep apnoea during sleeping hours, may reduce sympathetic activity and hence the morning blood rise. Future studies in patients with OSAS may have to consider morning blood pressure or even morning blood pressure surge as an outcome measure.
Our study should be interpreted within the context of its strengths and limitations. Our study investigated ambulatory, clinic and home blood pressure monitoring. With comprehensive blood pressure measurements, our study showed interesting results on daytime ambulatory blood pressure and home morning blood pressure during early follow-up. However, our study was a single-center feasibility trial, and our sample size estimation had been overoptimistic because of too high expectation of blood pressure lowering effect of CPAP in nocturnal hypertensive patients. In addition, a higher proportion of patients discontinued CPAP or sham CPAP treatment, and the use of the randomised treatment was relatively short. These limitations further reduced power of our study to show any between-group difference. Finally, we chose sham CPAP as control, because it is probably the most suitable placebo [Citation19] and has been commonly used in previous CPAP trials [Citation5]. However, sham CPAP may cause discomfort and therefore influence sleep quality and quantity [Citation19]. Probably because the effect size was rather small [Citation19], we did not observe any significant rise in blood pressure from baseline to the sham CPAP treatment period.
In conclusion, our feasibility trial did not show a greater or preferential blood pressure lowering effect with CPAP in patients with nocturnal hypertension and moderate-severe OSAS. However, our study showed that CPAP might have a greater blood pressure lowering effect in the morning and those with a faster pulse rate. These findings should be investigated in future randomised controlled trials with CPAP therapy in patients with OSAS and hypertension.
Acknowledgement
The authors gratefully acknowledge the participation of all patients and expert technical help of Yi-Ni Zhou (The Shanghai Institute of Hypertension, China).
Disclosure statement
Dr Wang reports having received lecture and consulting fees from Bayer, Daiichi-Sankyo, Novartis, Omron, Pfizer, and Servier. Drs Shen and Yin are employees of Philips Research. The other authors declare no conflict of interest.
Additional information
Funding
References
- Whelton PK, Carey RM, Aronow WS, et al. 2017 ACC/AHA/AAPA/ABC/ACPM/AGS/APhA/ASH/ASPC/NMA/PCNA guideline for the prevention, detection, evaluation, and management of high blood pressure in adults: executive summary: a report of the American College of Cardiology/American Heart Association Task Force on Clinical Practice Guidelines. Hypertension. 2018;71:1269–1324.
- Williams B, Mancia G, Spiering W, et al. 2018 ESC/ESH guidelines for the management of arterial hypertension. Eur Heart J. 2018;39(33):3021–3104.
- Denker MG, Cohen DL. Use of continuous positive airway pressure for sleep apnea in the treatment of hypertension. Curr Opin Nephrol Hypertens. 2014;23(5):462–467.
- McEvoy RD, Antic NA, Heeley E, et al. CPAP for prevention of cardiovascular events in obstructive sleep apnea. N Engl J Med. 2016;375(10):919–931.
- Yu J, Zhou Z, McEvoy RD, et al. Association of positive airway pressure with cardiovascular events and death in adults with sleep apnea: a systematic review and meta-analysis. JAMA. 2017;318(2):156–166.
- Montesi SB, Edwards BA, Malhotra A, et al. The effect of continuous positive airway pressure treatment on blood pressure: a systematic review and meta-analysis of randomized controlled trials. J Clin Sleep Med. 2012;8(5):587–596.
- Schein AS, Kerkhoff AC, Coronel CC, et al. Continuous positive airway pressure reduces blood pressure in patients with obstructive sleep apnea: a systematic review and meta-analysis with 1000 patients. J Hypertens. 2014;32(9):1762–1773.
- Hu X, Fan J, Chen S, et al. The role of continuous positive airway pressure in blood pressure control for patients with obstructive sleep apnea and hypertension: a meta-analysis of randomized controlled trials. J Clin Hypertens. 2015;17(3):215–222.
- Tkacova R, McNicholas WT, Javorsky M, et al. Nocturnal intermittent hypoxia predicts prevalent hypertension in the European Sleep Apnoea Database cohort study. Eur Respir J. 2014;44(4):931–941.
- Chen Q, Cheng YB, Liu CY, et al. Ambulatory blood pressure in relation to oxygen desaturation index as simultaneously assessed by nighttime finger pulse oximetry at home. J Clin Hypertens. 2018;20(4):648–655.
- McEvoy RD, Anderson CS, Antic NA, et al. The sleep apnea cardiovascular endpoints (SAVE) trial: rationale and start-up phase. J Thorac Dis. 2010;2(3):138–143.
- Qaseem A, Dallas P, Owens DK, et al. Diagnosis of obstructive sleep apnea in adults: a clinical practice guideline from the American College of Physicians. Ann Intern Med. 2014;161(3):210–220.
- Duran-Cantolla J, Aizpuru F, Montserrat JM, et al. Continuous positive airway pressure as treatment for systemic hypertension in people with obstructive sleep apnoea: randomised controlled trial. BMJ. 2010;341:c5991.
- Parati G, Stergiou G, O’Brien E, et al. European Society of Hypertension practice guidelines for ambulatory blood pressure monitoring. J Hypertens. 2014;32(7):1359–1366.
- Castro-Grattoni AL, Torres G, Martinez-Alonso M, et al. Blood pressure response to CPAP treatment in subjects with obstructive sleep apnoea: the predictive value of 24-h ambulatory blood pressure monitoring. Eur Respir J. 2017;50(4):1700651.
- Pedrosa RP, Drager LF, de Paula LKG, et al. Effects of OSA treatment on BP in patients with resistant hypertension: a randomized trial. Chest. 2013;144(5):1487–1494.
- Cho JS, Ihm SH, Kim CJ, et al. Obstructive sleep apnea using Watch-Pat 200 is independently associated with an increase in morning blood pressure surge in never-treated hypertensive patients. J Clin Hypertens. 2015;17(9):675–681.
- Hoffstein V, Mateika J. Evening-to-morning blood pressure variations in snoring patients with and without obstructive sleep apnea. Chest. 1992;101(2):379–384.
- Rodway GW, Weaver TE, Mancini C, et al. Evaluation of sham-CPAP as a placebo in CPAP intervention studies. Sleep. 2010;33(2):260–266.