Abstract
New SHRIMP U – Pb dating of the rocks from the Willyama Supergroup clarifies the geochronology of the lower to middle parts of the sequence and contemporaneous intrusions, and confirms that the prospective upper part of the Broken Hill Group extends into South Australia. In the Rantyga Group that constitutes the Redan geophysical zone in the southeastern part of the Broken Hill Domain, the relative stratigraphic positions of the Redan Gneiss (lowermost) and (newly named) Farmcote Gneiss (uppermost) are confirmed. An age of 1710 ± 4 Ma on a probable metavolcanic gneiss in the Redan Gneiss confirms this formation as the oldest dated unit in the Rantyga Group and in the Broken Hill Inlier. Metasediments in the Farmcote Gneiss contain approximately contemporaneous zircons dated at 1705 ± 5 Ma. The Rantyga Group is considered part of the Willyama Supergroup, rather than Archean or Early Paleoproterozoic basement as was suggested earlier. Previous attempts to date the Thackaringa Group concentrated on albitised metasediments, producing a range of detrital dates, with no definitely contemporaneous zircon. In the present study, three probable metavolcanic rocks from the Cues Formation yielded a consistent age of ca 1700 Ma, slightly younger than the underlying Alma Granite Gneiss (metagranite). The metagranite was intruded under very shallow cover. Three other concordant metagranites were dated. The 1685 ± 5 Ma Wondervale Well Granite Gneiss is indistinguishable in age from the 1683 ± 3 Ma Rasp Ridge Granite Gneiss. However, the Stephens Creek Granite Gneiss appears to be slightly older in that the stratigraphically lower part was dated at 1689 ± 5 Ma, while the upper part was dated at 1686 ± 4 Ma. Previous correlations of the lower and upper parts, with the Alma and Rasp Ridge Granite Gneisses, respectively, are therefore invalid. The 1695 ± 4 Ma Georges Bore Granite Gneiss correlates with neither the Alma Granite Gneiss nor the Rasp Ridge Granite Gneiss. All three granite gneisses dated in this study have intruded the upper Thackaringa Group and/or lower Broken Hill Group, indicating very shallow levels of emplacement, probably <1 km of current stratigraphic thickness. Very shallow emplacement of sill-like granites more or less coincides with deposition of Broken Hill-type mineralisation, and may have influenced ore formation. Samples from two critical parts of the Broken Hill Group were analysed. A sample of suspected Hores Gneiss from near Mingary in South Australia gives an interpreted age of 1684 ± 6 Ma, indistinguishable from Hores Gneiss in the type area north of Broken Hill. An age of 1693 ± 4 Ma on Potosi-type gneiss from the Parnell Formation north of Broken Hill confirms earlier dating from the same location. The new geochronological information shows that the time interval between the oldest stratigraphic unit (Redan Gneiss) and the top of the Broken Hill Group (Hores Gneiss) is only ∼25 million years, leaving little space for major time breaks. More specifically, the time interval between the middle Thackaringa Group (Cues Formation) and the lower Broken Hill Group (Ettlewood Calc-Silicate Member) is only ∼5 million years, suggesting continuous or near-continuous deposition.
Introduction
This study follows on from Page et al. (Citation2005a), and addresses several remaining issues relating to the depositional history of the Willyama Supergroup and age of syn-depositional intrusions into the supergroup.
The Paleoproterozoic Willyama Supergroup comprises the oldest rocks exposed in the Curnamona Province that straddles the New South Wales – South Australian border. Mapping over recent decades has shown that there are significant differences between the northwestern and southeastern exposures of the Willyama Supergroup. The supergroup can be regarded as occupying two relatively different outcrop domains, the Broken Hill Domain mostly in New South Wales, but crossing into South Australia, and the Olary Domain, mostly in South Australia (Laing Citation1996).This paper focuses on the Broken Hill Domain (), which hosts the ∼280 Mt Broken Hill Pb – Zn – Ag orebody. The Broken Hill Inlier and Euriowie Inlier () are the outcropping expression of the Broken Hill Domain.
Figure 1 Geological map of the Broken Hill and Euriowie Inliers, Broken Hill Domain, showing sample locations. Note: sample numbers are abbreviated, e.g. 5816 is sample 200218.5816, 5036 is sample 9818.5036.
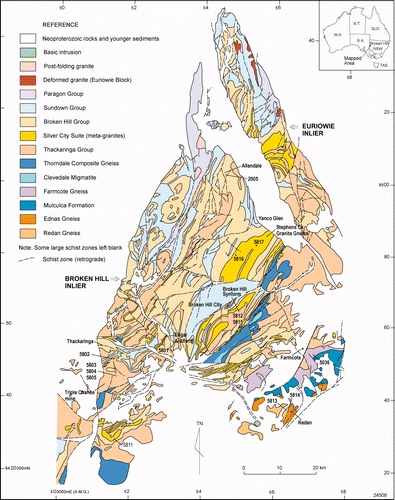
For many years, the age of the metamorphosed and deformed Willyama Supergroup was only known approximately, from Rb – Sr dating (Pidgeon Citation1967). It was not until SHRIMP zircon U – Pb dating was applied that convincing ages of any of the stratigraphic units were determined. Page & Laing (Citation1992) determined the age of the Hores Gneiss that hosts the Broken Hill orebody. Further determinations were made by Nutman & Ehlers (Citation1998a, Citationb) and others, but some of these were challenged on geological grounds by Stevens (Citation1999) and on isotopic grounds by Page et al. (Citation2005a). SHRIMP zircon U – Pb dating by Page et al. (Citation2005a, b) in both the Broken Hill and Olary Domains established an age framework for much of the Willyama Supergroup. In the Broken Hill Domain, several stratigraphic units in the Broken Hill Group and upper Paragon Group () were dated. Key results in terms of understanding depositional ages are shown in .
Figure 2 Stratigraphic column for the Willyama Supergroup in the Broken Hill and Euriowie Inliers. Geochronological data from Page et al. (Citation2005a) and this study.
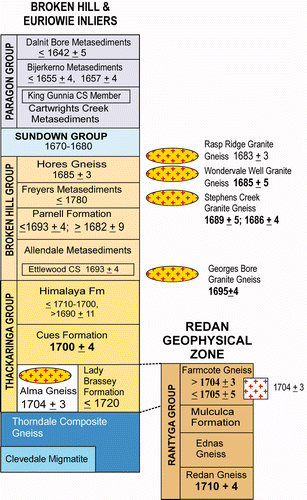
Table 1 Key depositional ages in the Broken Hill Domain.
In addition, Page et al. (Citation2005a) dated the Alma Granite Gneiss (previously the Alma Gneiss at 1704 ± 3 Ma and the Rasp Ridge Granite Gneiss (previously the Rasp Ridge Gneiss: see Appendix 1) at 1683 ± 3 Ma. The Rasp Ridge Granite Gneiss had been regarded as a metavolcanic unit by some previous workers (Willis et al. Citation1983), and as a metagranite by others (Vassallo & Vernon Citation2000). The age obtained is too young for its stratigraphic position at the top of the Thackaringa Group; consequently it is now interpreted as a syn-sedimentary granitic intrusion. By analogy the Alma Granite Gneiss is also interpreted as a granitic intrusion.
After Page et al. (Citation2005a), knowledge gaps remained in the lower Willyama Supergroup and in the Sundown and lower Paragon Groups (). The current study attempted to address these gaps, and to further investigate the granitic gneiss bodies that are concordant or near-concordant with the stratigraphic sequence. The study failed to resolve the gaps in the upper, Sundown – Paragon part of the sequence but was more successful with the lower part and the granitic gneisses.
Three samples of fine-grained graphitic metasandstone from the upper Cartwrights Creek Metasediments (lower Paragon Group) were found to contain no zircons, while a medium-grained weathered metasandstone from the lower part of the formation contained a zircon assemblage which gave no satisfactory results, as it consisted dominantly of extraneous zircon introduced in Tertiary and recent weathering processes. As a result, uncertainty remains concerning the depositional ages of this part of the sequence.
In the lower part of the sequence, significant results were obtained from the Thackaringa Group, Farmcote Gneiss and Redan Gneiss, and from three bodies of granitic gneiss (). In addition, samples from two critical parts of the Broken Hill Group have been analysed.
The new analyses have important bearing on several issues. Prior to this study, there was little constraint on the age of the oldest exposed part of the Willyama Supergroup in the Broken Hill Domain, and hence no control on the duration of depositional breaks that may be present. Several previous researchers have interpreted the Redan geophysical zone in the southeastern part of the Broken Hill Domain as significantly older basement to the Willyama Supergroup. Our analyses from the Redan and Farmcote Gneisses test this interpretation.
The Cues Formation of the Thackaringa Group has some lithological resemblance to the Parnell Formation of the Broken Hill Group, and it has been suggested that the Cues Formation might be a structural repetition of the Parnell Formation. Rocks from both the Cues Formation and the Parnell Formation were analysed in this study.
Several bodies of granitic gneiss have previously been correlated with the Rasp Ridge Gneiss on the basis of their stratigraphic position, and on the interpretation that they and the Rasp Ridge Gneiss are metamorphosed volcanic rocks. The major granitic gneiss body at Stephens Creek had been arbitrarily subdivided into Alma Gneiss and Rasp Ridge Gneiss units. We analysed a sample from the base and a sample from the top of this body.
Analysis of a sample of Potosi-type* gneiss from the Mingary area has implications for interpreting the extent of the Broken Hill Domain and for the prospectivity of that part of South Australia.Footnote
Regional Geology
The Willyama Supergroup is a succession of Paleoproterozoic metasediments and minor metavolcanics, extensively intruded by syn-depositional dolerite or gabbro (now basic gneiss) dykes and sills and granite (now granite gneiss) sills. The sequence was deformed in the ca 1600 Ma Olarian Orogeny, accompanied by syn- to late-tectonic granite intrusion, and in the ca 500 Ma Delamerian Orogeny. Metamorphic grade ranges from greenschist in the northwest to hornblende granulite in the southeast. The effects of retrograde metamorphism are widespread.
In recent years, the outcropping areas of the Willyama Supergroup have been referred to two domains: the Broken Hill Domain and the Olary Domain (Laing Citation1996). Recently, correlations of some units have been established (Page et al. Citation2005b), but significant differences exist in the stratigraphic successions and syn-sedimentary intrusive histories in the two domains. The Broken Hill Domain is characterised by a relative abundance of basic gneiss (amphibolite and basic granulite, interpreted as syn-sedimentary mafic sills and dykes) and the presence of distinctive garnet – biotite quartzo-feldspathic gneiss (Potosi-type gneiss). The Olary Domain contains fewer syn-sedimentary mafic intrusions and no Potosi-type gneiss. Several other notable differences are documented in Page et al. (Citation2005b). It was thought that the boundary between the Broken Hill and Olary Domains was a major fault, the extension of the north-northeast-trending Mundi Mundi Fault. However, this view was disputed by Crooks (Citation2001), who saw the boundary as less well defined, and its nature remains unclear. One of the samples analysed in this study helps to confirm that the Broken Hill Domain extends into South Australia, and to delimit the domain boundary.
Retexturing due to amphibolite- to granulite-grade metamorphism, obscured the origins of various rock types that comprise the Broken Hill Domain, leading to much controversy (see Haydon & McConachy Citation1987). In recent years, the origins have become clearer as a result of field studies, textural studies and SHRIMP zircon U – Pb geochronology. For example, the volcanic origin of the Potosi-type gneiss in the Hores Gneiss stratigraphic unit has been clarified by textural studies in the lowest metamorphic grade area, and recognition of less well-preserved equivalents in higher-grade areas (Stevens & Barron Citation2002). However, there are some rock types and stratigraphic units that only occur in high metamorphic grade areas. The Redan Gneiss, Farmcote Gneiss and Cues Formation are only recognised in granulite-grade areas, and as a result there have been doubts about the protoliths of the metamorphic rock types. It is not clear whether some rocks were supracrustal or intrusive. SHRIMP U – Pb zircon studies help to resolve these questions, but some uncertainty remains.
Prior to the current study, the oldest strata of the Willyama Supergroup, in the Broken Hill Domain, were thought to be either the Thorndale Composite Gneiss/Clevedale Migmatite, or the rocks of the Redan geophysical zone (Redan Sub-Block in ). The former are partially melted metasediments with or without albitic alteration. The latter (termed Rantyga Group by Stevens Citation2006a) are characterised by abundant albite – quartz – magnetite rocks with or without amphibole. Depositional ages for neither were confidently determined, although Nutman & Ehlers (Citation1998a) preferred an Archean age for a sample from the Rantyga Group. The relationship between the Rantyga Group and the remainder of the Broken Hill Inlier was unclear (Stevens & Corbett Citation1993).
The Thackaringa Group overlies the Thorndale Composite Gneiss and underlies the Broken Hill Group. It comprises metasediments, including some thick units of albitised psammitic metasediment, minor quartz – magnetite and quartz – garnet rocks, and minor leucogneiss and Potosi-type gneiss. Sills and dykes of basic gneiss are abundant in many places. Maximum ages established for the Thackaringa Group, from detrital zircon grains in the Himalaya Formation, were ca 1720 Ma. The possibility existed of a depositional or erosional gap of up to 25 million years between the top of the Thackaringa Group and the base of the Broken Hill Group.
The Broken Hill Group consists of siliciclastic metasediments with distinctive Potosi-type gneiss at two levels, and minor calc-silicate rock, garnet-rich banded iron-formation, quartz – gahnite rock and garnet – quartz rock. Sills of basic gneiss are generally present. Geochronology by Page et al. (Citation2005a) established an age span of ca 1693 – 1685 Ma for deposition of the Broken Hill Group.
The Sundown and Paragon Groups mostly comprise siliciclastic metasediments. The metasediments of the Sundown Group are indistinguishable from those in the Broken Hill Group, and it is likely that they represent a continuation of the same depositional system after the brief interruption represented by the 1685 ± 3 Ma Hores Gneiss volcaniclastic mass flows. Sundown Group deposition continued until some time after 1672 Ma. The metasediments of the Paragon Group tend to be graphitic, and the group contains the minor King Gunnia Calc-Silicate Member. Deposition of the Bijerkerno Metasediments commenced at about 1657 – 1655 Ma, but the age range of the Cartwrights Creek Metasediments has not been established; thus the duration of any possible hiatus between the Sundown and Paragon Groups could be from zero to 15 million years. The youngest strata dated in the Paragon Group are the ca 1642 Ma upper Dalnit Bore Metasediments.
There is abundant evidence of a high-grade metamorphic event accompanying deformation between about 1600 Ma and 1590 Ma (Olarian Orogeny). The evidence comes from most, if not all, of the metamorphic zircon and rutile ages, as well as the ages of syn- and late-tectonic granites. Nevertheless, speculation persists that a high-grade deformation or deformations may have occurred between ca 1690 Ma and 1600 Ma (Gibson & Nutman Citation2004). We believe this speculation is groundless in the Broken Hill Domain, and not well substantiated in the Olary Domain (see later discussion). The ca 500 Ma Delamerian Orogeny was accompanied by lower-grade metamorphism, with virtually no new zircon growth.
Analytical Methods
Zircons were separated by normal crushing, gravity and magnetic separation methods, mounted with chips of standard zircon in epoxy, polished to expose grain centres, Au-coated, and analysed using the SHRIMP II instrument in the Research School of Earth Sciences, Australian National University, Canberra and in Curtin University, Perth. The U – Th – Pb analysis of zircon by SHRIMP as used in this study is described by Compston et al. (Citation1984) and Williams & Claesson (Citation1987). Data on standards and unknowns generally were collected in sets of seven scans in analytical sessions of 72 – 96 h. Monitoring of SHRIMP data acquisition by means that include cathodoluminescence imagery and 207Pb/206Pb standardisation ensured the accuracy of sample 207Pb/206Pb measurements. Data reduction to calculate isotopic ratios used counting statistics augmented by uncertainties from the degree of nonlinearity of the ratioed pairs of isotopic species; these were evaluated using software developed by Ireland (in Muir et al. Citation1996). Corrections were made for initial Pb using values from Cumming & Richards (Citation1975) based on measured 204Pb/206Pb.
Results were monitored by 207Pb/206Pb measurement of the TIMS-measured QGNG (quartz – gabbro – norite – gneiss) zircon standard (Mortimer Citation1984; Daly et al. Citation1998), and this zircon was also used as the Pb/U calibration standard (206Pb/238U of 0.3324). Black et al. (Citation2003) confirmed the TIMS 207Pb/206Pb age of the QGNG standard with a weighted mean at 1851.1 ± 0.6 Ma (2σ). Uncertainties in Pb/U include a component of uncertainty of ∼1.5% (1σ) in the calibration of the QGNG standard. Decay constants used are those recommended by the IUGS Subcommission on Geochronology (Steiger & Jäger Citation1977). Errors in calculated ages (not augmented for uncertainty in concordia) are quoted as 95% confidence limits, although analytical errors for individual analyses in data tables and concordia plots are at the 1σ level. The SHRIMP analytical data are given in Appendix 2* in tables identified as Appendix 2.1 to Appendix 2.17 in the text.
Deposition of the Thackaringa Group
The Thackaringa Group immediately underlies the Broken Hill Group (). Its main distinguishing feature is its abundant albitised metasediment. The Lady Brassey Formation at the base and the Himalaya Formation at the top are both defined by the presence of thick, mappable units of albitic metasediment, and the Cues Formation and Alders Tank Formation also contain minor units of albitic metasediment. In contrast with the Olary Domain, where discordant albitisation is common, albitisation in the Broken Hill Domain is concordant to bedding and occurred very soon after sedimentation. Aside from the albitisation, the Himalaya Formation tends to be much more psammitic than the overlying Broken Hill Group.
The Cues Formation comprises psammopelitic to psammitic metasediments with generally abundant bodies of basic gneiss, now interpreted as metadolerite sills. Thin lenses or pods of quartz – feldspar – biotite – garnet gneiss (Potosi-type gneiss) are common, and in some areas there is one or more units of 1 – 3 m-thick leucocratic quartzo-feldspathic gneiss. The leucogneiss units and lenses of Potosi-type gneiss tend to occur close together near the middle of the Cues Formation. Stratiform horizons of Fe-garnet – quartz, quartz – Fe-sulfide, quartz – magnetite rocks are a characteristic of the Cues Formation. The Alders Tank Formation is relatively undistinguished, and it is recommended that it be incorporated into the Cues Formation, and the name discontinued.
The Thackaringa Group, as defined by Stevens et al. (Citation1983) and Willis et al. (Citation1983) also included the Alma Gneiss and Rasp Ridge Gneiss, but data presented in Page et al. (Citation2005a) and here indicate that this is no longer valid.
Previous geochronology of the Thackaringa Group
Until now, there have been no direct ages determined for the Thackaringa Group, due to the apparent lack of suitable lithologies such as felsic volcanic or tuffaceous rocks. The importance of dating the Thackaringa Group is emphasised by recent work that dates what were thought to be stratigraphically comparable rocks between 1720 and 1710 Ma in the Olary Domain (the Curnamona Group: Conor Citation2000; Page et al. Citation2005b). Love (Citation1992) and Donaghy et al. (Citation1997a, Citation1998) attempted to constrain the age of the layered albite – quartz-rich rocks in the Himalaya and Lady Brassey Formations, by SHRIMP U – Pb zircon dating. The albite – quartz-rich rocks were interpreted by Plimer (Citation1977), Willis et al. (Citation1983) and Brown et al. (Citation1983) as altered felsic tuffs, considered amenable targets for zircon geochronology. However, Love (Citation1992), Donaghy et al. (Citation1997a, Citation1998) and Page et al. (Citation2005a) found mostly small zircon grains with detrital characteristics and with a wide range of Paleoproterozoic ages. It has become clear that the albitic rocks originated as detrital sediments without a significant component of contemporaneous volcanic detritus. Hence, these previous studies provide only maximum depositional ages.
Northeast of Allendale (), Love (Citation1992) found the ages of the most confidently identified youngest group of detrital zircon grains in two samples from the Himalaya Formation to be ca 1798 ± 15 and ca 1762 ± 17 Ma. In the Thackaringa area, Donaghy et al. (Citation1997a, Citation1998) found the youngest pre-metamorphic group of zircon grains to be ca 1710 – 1700 Ma. Page et al. (Citation2005a) sampled an albite – quartz rock from the Himalaya Formation in Spring Creek northeast of Allendale, and found several age clusters of zircon grains, the most prominent being ca 3000 – 2980, 2680 – 2650, 1850, 1790 and 1730 Ma. The youngest group, a major peak close to 1730 Ma, is regarded as the maximum age for deposition of the Himalaya Formation.
Current study of the Thackaringa Group (Cues Formation)
Given the lack of a well-defined contemporaneous zircon population and resultant poor age definition from the low-metamorphic grade part of the Himalaya Formation, we targeted higher-grade felsic gneisses in the older Cues Formation. Willis (Citation1982) concluded that some of these felsic gneisses include metavolcaniclastic components. In addition, the Cues Formation contains Potosi-type gneiss lithologies of the type that have been shown to be metavolcaniclastic and of use in dating the Hores Gneiss (Broken Hill Group). Three rock types were sampled from the Cues Formation: amphibolite, leucocratic quartzo-feldspathic gneiss (leucogneiss) and medium-grained quartz – feldspar – biotite – garnet gneiss (Potosi-type gneiss). shows a summary of the new age determinations from the Cues Formation.
Table 2 Summary of U – Pb SHRIMP ages for Cues Formation rock types.
Amphibolite occurs at several stratigraphic levels in the Cues Formation, and tends to be most abundant in the middle of the formation. The amphibolites are generally concordant and most probably represent metamorphosed and deformed sills (Stevens Citation1998).
Sample 200218.5804 is a concordant amphibolite retaining a microdoleritic texture of fine-grained, interlocking feldspar laths, visible in hand specimen. It is one of a number of amphibolite bodies that occur with minor Potosi-type gneiss and leucogneiss in Cues Formation 1 km southeast of Old Coolgardie Tank, near the Triple Chance road. The zircon suite consists mostly of lenticular and ovoid, high-U metamorphic grains, 14 acceptable analyses defining the metamorphic age at 1593 ± 5 Ma (MSWD 1.00). Two Paleoproterozoic xenocrysts have inherited 207Pb/206Pb ages ca 2261 Ma and 1968 Ma (Appendix 2.1*). An incompletely recrystallised grain (405.2) is discordant, with a geologically meaningless 207Pb/206Pb age of 1671 Ma whereas two other analyses are concordant and closer to 1600 Ma. The 17 metamorphic zircons are all concordant, but the data points have an unexpectedly large 207Pb/206Pb age scatter from ca 1620 to 1540 Ma (; Appendix 2.1*). The youngest two (403.1, 415.1) could reflect a hitherto unrecognised metamorphic event (1550 – 1540 Ma), but this is speculative, and the aberrantly young ages for these two high-U grains are more likely due to inadequate Pb/U measurement.
Figure 3 Tera – Wasserberg concordia plot for zircon U – Pb SHRIMP data in Cues Formation basic granulites: (a) 200218.5804; (b) 200218.5815.
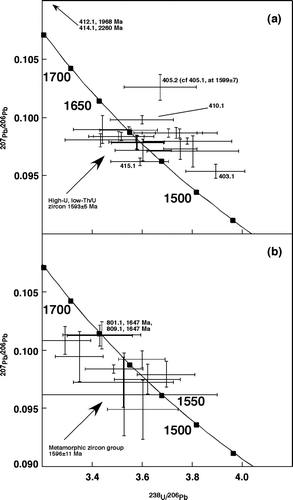
Sample 200218.5815 was from a concordant hornblende granulite body with orthopyroxene poikiloblasts and subtle layering. It is one of a number of prominent basic granulite bodies, defining the closure of the Broken Hill Synform. Cathodoluminescence imagery shows that the population of zircons is incompletely recrystallised, with remnant protolith cores being embayed by both low- and high-U metamorphic zircon phases (). Some analyses incorporate mixed protolith/metamorphic components as exemplified by two conformable data points (801.1, 809.1) at ca 1650 Ma (; Appendix 2.2*). Zircon U contents are variable and 207Pb/206Pb age errors are generally large. Ignoring the older two analyses, the remaining nine data points define a metamorphic age grouping (MSWD 1.06) at 1596 ± 11 Ma ().
Figure 4 Cathodoluminescence image of zircon from hornblende granulite in Cues Formation, Broken Hill Synform, sample 200218.5815. Variably luminescent cores are overgrown and partly replaced by bright (light grey to white), low-U metamorphic rims.
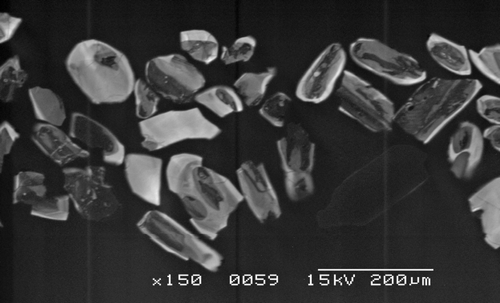
Leucogneiss occurs as up to three close-spaced, prominent, discontinuous layers (each layer generally 1 – 3 m thick) in the middle of the Cues Formation in the granulite-grade Thackaringa—Triple Chance area (Willis Citation1982, Citation1985; Stroud Citation1989a). Although no volcanic textures have been found, these units were interpreted by Willis (Citation1982) as rhyolitic air-fall tuffs, based on their composition and thin, extensive, conformable nature and persistence and their commonly laminated texture. If these units are tuffs, the magmatic zircon population would date this part of the Cues Formation. However, if these units are thin felsic intrusions into the Cues Formation, the zircon results provide a minimum age for the stratigraphy. It transpires (see below) that the age of the leucogneiss is identical to that of a Cues Formation Potosi-type gneiss in which the zircon grains are considered detrital, thus providing Cues Formation with identical maximum and minimum ages. This then suggests that the leucogneiss is indeed volcanic in origin.
Sample 200218.5802 was taken from the most southeasterly (stratigraphically lowest) one of three parallel leucogneiss units, just north of the Indian-Pacific rail line, 2 km northeast of the hills known as Camels Humps. This 3 m-thick body and the other two leucogneiss units dip about 80°SE and are separated from each other by foliated metasedimentary schists. The stratigraphically uppermost body pinches out further north and is not present near where the sample was taken. The leucogneiss body sampled is a fine-grained quartz – feldspar – biotite gneiss with scattered garnet grains to about 2 mm, and abundant very thin, concordant pegmatitic veinlets. Retrograde muscovite can be seen on joint planes. The primary (protolith) zircons in this sample are mantled and corroded by metamorphic zircon recrystallisation features, but there are ample zoned grain interiors of magmatic origin. A cluster of 24 concordant SHRIMP analyses defines a magmatic crystallisation age of 1699 ± 4 Ma (MSWD 0.90) (). Seven of the zoned interiors are inherited xenocrysts with 207Pb/206Pb ages in the range 2585 – 1805 Ma, and a further three analyses (223.1, 223.2, 229.1) are also demonstrably ∼30 million years older than the main population (; Appendix 2.3ast;). Five analyses from the main population are affected by recent Pb loss and are between 7% and 18% discordant.
Figure 5 Tera – Wasserberg concordia plot for zircon U – Pb SHRIMP data in Cues Formation felsic gneisses: (a) 200218.5802 leucogneiss; (b) 200218.5805 leucogneiss; (c) 200218.5803 Potosi-type gneiss; (d) 200218.5812 Potosi-type gneiss.
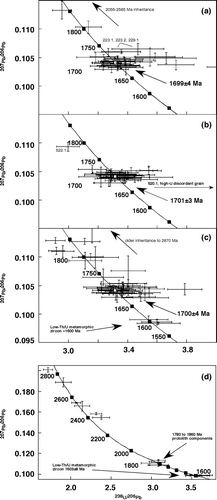
Sample 200218.5805 is a slightly weathered, fine-grained quartz – feldspar – biotite gneiss with very thin, concordant pegmatitic segregations, taken from a narrow leucogneiss body. It occurs 20 m from amphibolite sample 200218.5804, southeast of Old Coolgardie Tank. The zoned magmatic zircon interiors are rimmed by high-U metamorphic zircon, and analysis 520.1 (1630 ppm U) is highly discordant. Only one xenocryst (ca 1790 Ma) was detected in this sample. All 33 analyses of the magmatic population form a coherent grouping at 1701 ± 3 Ma (MSWD 0.99) ().
Potosi-type gneiss in the Cues Formation occurs as lenticular bodies up to ∼20 m thick and ∼100 m long, generally associated with amphibolite. Similar Potosi-type gneiss in the Hores Gneiss was shown by Laing et al. (Citation1984), Laing (Citation1996) and Stevens & Barron (Citation2002) to be volcanic in origin, on the basis of preserved volcanic textures. Although no such textures have yet been documented from Potosi-type gneiss in the Cues Formation, two samples were studied with the aim of at least determining maximum ages for the Cues Formation sequence. (The most likely alternative origin for Potosi-type gneiss is sandstone incorporating some volcanic detritus: see discussion of the Potosi-type gneiss in the Parnell Formation, below.)
Sample 200218.5803, a Potosi-type gneiss from the Old Coolgardie Tank area was a dark, medium- to fine-grained quartz – feldspar – biotite gneiss with scattered garnets to 4 mm. It was taken from a small body associated with leucogneiss and abundant amphibolite, about 180 m west of amphibolite sample 200218.5804. The ovoid morphology of the zircon population is due to metamorphic recrystallisation and zircon overgrowths present in all of the 51 analysed zircons. The bulk of the zircon in this rock forms a unimodal age cluster for 31 data points at 1700 ± 4 Ma (MSWD 0.98). Rigorously, this is a maximum depositional age for the protolith of the Potosi-type gneiss. The few overgrowths thick enough to be analysed (high-U, low Th/U rims 311.1, 337.1, 339.1) have normal metamorphic (Olarian) 207Pb/206Pb ages close to 1600 Ma (; Appendix 2.4*). The inherited zircon components have the same age range as found in the leucogneiss units (13 analyses between 2870 and 1760 Ma).
A second Potosi-type gneiss (200218.5812) was collected from a body 100 m long and 15 m wide, interlayered with basic granulite in the closure of the Broken Hill Synform. This darkish, medium-grained quartz – feldspar – biotite gneiss with abundant garnet porphyroblasts to 20 mm contains no magmatic zircon, and is therefore unlikely to be magmatic or even dominantly volcaniclastic in origin. It is more likely an immature sediment, and its multi-age inherited components have the same broad range (ca 2830 – 1785 Ma: Appendix 2.5*) as found in the previous Potosi-type gneiss and in the leucogneiss units. This suggests that all these lithologies were supracrustal and were exposed to the same provenance input. More than 90% of the zircon suite in this Potosi-type gneiss is recrystallised, and SHRIMP analyses of six such grains are concordant and define a metamorphic age of 1603 ± 8 Ma ().
Depositional and Metamorphic ages of the Redan and Farmcote Gneisses, Redan Geophysical Zone
Redan geophysical zone
The Redan Gneiss was interpreted by Stevens & Corbett (Citation1993) as the lowest formation in the Redan geophysical zone, a zone in the southeastern part of the Broken Hill Inlier exhibiting a strong magnetic response. The uppermost unit in this zone was interpreted by Stevens & Corbett (Citation1993) as an equivalent of the Lady Brassey Formation, the lowermost formation of the Thackaringa Group. Nutman & Ehlers (Citation1998a) loosely referred to part of this as the ‘Farmcote gneiss,’ and Page et al. (Citation2005a ) replaced the term ‘Lady Brassey Formation’ with ‘Farmcote Gneiss,’ but only within the Redan geophysical zone. The Farmcote Gneiss (defined in Appendix 1) is significantly different from the Lady Brassey Formation type section (Stevens et al. Citation1983).
Stevens (Citation2006a) proposed the name Rantyga Group to include the four formations that constitute the Redan geophysical zone: the Redan Gneiss, Ednas Gneiss, Mulculca Formation and Farmcote Gneiss. All four are magnetite-bearing, and all but the Mulculca Formation are albite-rich. The essential characteristics are: (i) the Redan Gneiss contains abundant layered quartz – sodic feldspar – hornblende – magnetite rock and quartz – sodic feldspar – magnetite gneiss; (ii) the Ednas Gneiss contains both of the above rock types, but the hornblende-bearing rock is minor; (iii) the Mulculca Formation contains partially melted metasediments and minor quartz – magnetite rocks; and (iv) the Farmcote Gneiss contains abundant albitised metasediments, leucogneiss and basic gneiss (the last two were probably intrusive). Outcrop of the Ednas Gneiss and Mulculca Formation is very poor.
The stratigraphic relationship between the Rantyga Group and the remainder of the Broken Hill Inlier is unclear. Several authors suggested that the Rantyga Group is ‘basement’ to the Willyama Supergroup. Tucker (Citation1983), from geophysical modelling, suggested that the Rantyga Group dips under the Broken Hill Inlier. Stevens & Corbett (Citation1993) found that the boundary between the Rantyga Group and the remainder of the Broken Hill Inlier is concordant with units on either side, and that the boundary is folded. On this basis, they suggested that the boundary is conformable. Nutman & Ehlers (Citation1998a) reported zircon U – Pb ages from two rock units in the Farmcote Gneiss in the northern part of the Redan geophysical zone: a layered albite-rich rock and a felsic leucogneiss. They found abundant Archean zircon in the layered albite-rich rock, with a few grains as young as 1960 Ma and 1820 Ma. They interpreted this either as a deformed Archean trondhjemite or as a metasediment derived from nearby Archean rocks. Our work shows that this metasediment is not Archean, is not necessarily derived from nearby Archean rocks and is in fact dominated by the effects of Olarian (ca 1600 Ma) metamorphism and zircon recrystallisation (see below). Our new dates from the Redan geophysical zone (Rantyga Group) are summarised in .
Table 3 Summary of new age determinations for Redan and Farmcote Gneisses.
Previous studies of the Redan Gneiss
The Redan Gneiss is typified by two rock types: laminated albite – quartz – hornblende – magnetite rock, and albite – quartz – magnetite rock. In both rock types, the feldspar is generally referred to as albite, but in fact K-feldspar comprises between nil and more than half of the feldspar, and the plagioclase ranges from albite to oligoclase. In places, the hornblende-bearing rock contains abundant epidote and/or minor clinopyroxene, and both rocks contain minor sphene in places.
The first SHRIMP U – Pb zircon work from the Redan Gneiss was done by Love (Citation1992), who found Late Archean to Paleoproterozoic inherited ages (2760 – 2390 Ma), and a range of other detrital ages between 2000 and 1800 Ma, 1700 and 1660 Ma, and a group of overgrowth ages ca 1570 Ma. From a thinly layered quartz – albite – amphibole – magnetite gneiss (his sample GC1), Love (Citation1992) obtained zircon populations with a SHRIMP U – Pb age of 1827 ± 18 Ma interpreted as a maximum age, probably defined by detrital zircon grains, a population of ca 1680 Ma (considered by Love to be unreliable), and 1563 (+20/ – 7) Ma for a zircon metamorphic closure age. Notably, the ca 1563 Ma zircon grains are stubby, euhedral, typical igneous shapes, rather than the normal round metamorphic zircon shapes. From another quartz – albite – hornblende – magnetite gneiss (his sample BH22), the most coherent zircon population had a median age of 1916 Ma, while four metamorphic overgrowths gave a median age of 1576 Ma.
Previous studies of the Farmcote Gneiss
Nutman & Ehlers (Citation1998a) reported zircon U – Pb ages from two rock units in the Farmcote Gneiss: a layered albite-rich rock (95BH-15), which they considered may be a deformed Archean trondhjemite, and an adjacent felsic leucogneiss (95BH-16), which they interpreted as a ca 1650 Ma intrusive trondhjemite (data shown in Nutman & Gibson Citation1998 ).
Page et al. (Citation2005a) analysed sample 9818.5035 from this same felsic leucogneiss outcrop and analysed another leucogneiss sample (9818.5034) within the same body. They concluded that it was a granitic or subvolcanic intrusion and determined crystallisation ages of 1703 ± 3 Ma and 1705 ± 3 Ma, and metamorphic recrystallisation ages in the two samples of 1605 ± 3 Ma and 1602 ± 4 Ma, respectively.
Nutman & Ehlers (Citation1998a p. 692) considered their ‘Archean’ banded gneiss (95BH15) to be ‘… a trondhjemite reworked in several tectonothermal events giving rise to a layered orthogneiss.’ Because they found that most of the zircons in the gneiss were Archean in age, and found no 1800 – 1730 Ma zircons that are ‘normally’ present in most Willyama Supergroup supracrustal rocks, these authors suggested that their trondhjemitic gneisses were part of an Archean basement (2654 ± 16 Ma?) which underwent reworking events at ca 2400 Ma, 2100 Ma and probably later times. Nutman & Ehlers (Citation1998a) also canvassed an alternative interpretation that the felsic gneisses might be Paleoproterozoic metasediments containing inherited Archean zircons, although they argued against this model because (i) they considered the felsic gneiss to be a trondhjemitic orthogneiss, and (ii) the zircons in the gneiss are anomalous in respect to other Broken Hill rocks and ‘… are larger, less rounded and predominantly Archaean in age with … …. none of the 1800 – 1730 Ma ones that are so abundant in all Willyama Supergroup metasedimentary and magmatic rocks’ (Nutman & Ehlers Citation1998a pp. 692 – 693).
Current study of the Redan Gneiss
The thinly layered gneiss at Love's (Citation1992) GC1 site was resampled from the location 2 km west-northwest of Redan homestead (note: not east, as stated in Love Citation1992). Our sample at the GC1 site (sample 200218.5813) is an oligoclase – microcline – quartz – epidote – hornblende – magnetite gneiss with minor sphene and apatite, displaying well-developed bedding lamination, like that shown in Stevens & Corbett (Citation1993 ). Cathodoluminescence imagery () shows that this metasediment sample has a complex provenance among the relict ovoid zircon cores. The complexity is masked in transmitted light images, as the metamorphic overgrowths impart euhedral prismatic and pyramidal faces that might otherwise be interpreted as igneous morphology. Zircons similar to this are as described by Nutman & Ehlers (Citation1998a) for their sample 95BH09 about 0.5 km(?) south of here (= our location 5814) from which they interpreted a magmatic age of 1563 ± 9 Ma.
Figure 6 Zircons from Redan Gneiss calcalbitite sample 200218.5813 under (a) cathodoluminescence and (b) transmitted light (m, metamorphic; p, protolith).
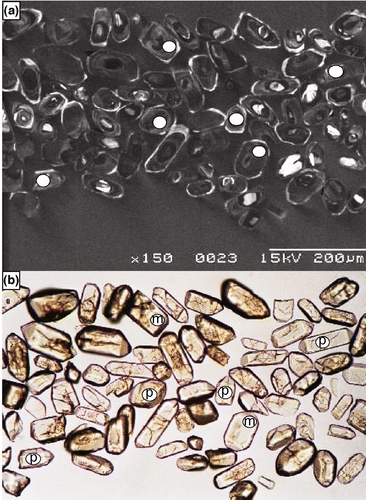
Our SHRIMP zircon U – Pb data (sample 200218.5813) are generally concordant (; Appendix 2.6*) and indicate at least five provenance age clusters: two Late Archean ages, and age groups at ca 1914, 1850 and 1800 Ma. The youngest protolith age population (207Pb/206Pb age 1704 ± 13 Ma) is defined by only two zircon cores (407, 439) that were analysed in duplicate. Euhedral metamorphic overgrowths and recrystallisation features dominate this zircon suite (), and the majority of the SHRIMP data (13 analyses) define a coherent 207Pb/206Pb age population at 1575 ± 4 Ma (MSWD 1.40). Although this is a normal high-U (400 – 1400 ppm) metamorphic zircon suite, its Th/U (0.1 – 0.95) is not measurably different from the general protolith zircon population. Zircon grains 448 and 443 give concordant ages of 1516 ± 10 Ma and 1532 ± 9 Ma, which are not readily explicable unless they are indicative of an as yet unrecognised younger metamorphic event.
Figure 7 Tera – Wasserberg concordia plots for zircon U – Pb SHRIMP data in Redan Gneiss samples: (a) 200218.5813 (= Love Citation1992 GC1 site); (b) 200218.5814 (∼Love Citation1992 BH22 and Nutman & Ehlers Citation1998a 95BH09 site). Ehlers & Nutman (1997) and Nutman & Ehlers (Citation1998a p. 689) indicated a magmatic age of 1563 ± 9 Ma for site 95BH09.
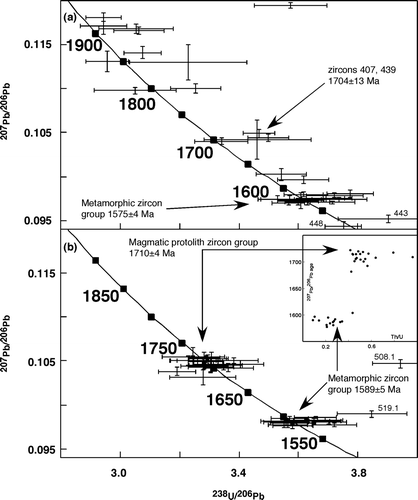
A sample (200218.5814) of poorly layered quartz – albite – K-feldspar – magnetite gneiss with minor sphene and secondary muscovite was taken from near Love's (Citation1992) BH22 sample site, about 1.5 km northwest of Redan homestead. This poorly layered albitic sample contains a less complex zircon population, with essentially two age populations of zircons: protolith zircons with metamorphic zircon rims, and discrete metamorphic zircon grains. The protolith zircons are uniformly zoned and have a homogeneous igneous aspect. All 19 SHRIMP U – Pb analyses within the protolith relics form one age cluster at 1710 ± 4 Ma (MSWD 0.87) including the one analysis (508.1) that is discordant (; Appendix 2.7*). This is interpreted as a reliable maximum depositional age and is in accord with the less well-defined youngest protolith age population (1704 ± 13 Ma) found in the previous sample. The high-U, low-Th/U metamorphic zircon rims and separate metamorphic grains have well-defined prismatic form. Like those in the previous sample (), these too could be morphologically interpreted from transmitted light images as of igneous origin, but cathodoluminescence imagery shows these high-U, low-Th/U zircons are totally recrystallised. The concordant U – Pb dataset from 13 of the 14 zircon areas defines a metamorphic age of 1589 ± 5 Ma. Some 207Pb/206Pb scatter in excess of experimental uncertainty (MSWD 2.11) may be due to unrecognised inheritance from recrystallised protolith zircon. Given this uncertainty, it is doubtful whether the two metamorphic ages (1575 ± 4, 1589 ± 5 Ma) determined from these rocks are geologically distinguishable, and we interpret the combined data as providing evidence for a significant metamorphic event in the Redan area at approximately 1580 Ma.
Current study of the Farmcote Gneiss
The coarse-grained, biotite – muscovite quartzo-feldspathic gneiss (our sample 9818.5036B at Nutman & Ehlers' 1998a site 95BH15) is layered on a 1 – 10 cm scale, with whitish albitic metasediment layers, more biotite-rich layers and thin discontinuous melt layers. The discontinuous felsic pods (melt ± albitic metasediment) were concentrated after a coarse crushing (sample 9818.5036C). A more massive, medium-grained amphibole-bearing grey gneiss (our sample 9818.5036) without any obvious quartzo-feldspathic banding was sampled 2 m east of the quartzo-feldspathic gneiss. A second massive grey gneiss layer (9818.5036A) was sampled a few metres southeast of the quartzo-feldspathic gneiss.
Sample 9818.5036B, Layered Albitic Gneiss
SHRIMP U – Pb zircon data for 53 zircon analyses from sample 9818.5036B are given in Appendix 2.8* and . As this sample is from the same site as 95BH15, it might be expected that the data would closely mimic those of Nutman and Ehlers (Citation1998a p. 690) who pointed out that ‘… most grains consist of only one generation of zircon.’ However, we find no evidence from our data to support Nutman & Ehlers (Citation1998a) supposed tectonothermal events (at 2400, 2100, 1950, 1820 Ma), but instead recognise a younger and far more significant tectonothermal event at Olarian time. Zircon grown in this younger event forms clear high-U selvedges on most of the older grains, has remarkably uniform Th/U around 0.15 ( inset), and from 16 analyses defines a metamorphic age of 1603 ± 3 Ma (MSWD 1.67). Both Late Archean (e.g. 203, 209) and ca 1800 Ma (e.g. 216, 237) grains have such overgrowths. Similar metamorphic ages date the Olarian Orogeny in many other cases studied in the Curnamona Province (Page & Laing Citation1992; Page et al. Citation2005a).
Figure 8 Tera – Wasserberg concordia plots for zircon U – Pb SHRIMP data in Farmcote Gneiss samples: (a) banded gneiss 9818.5036B; (b) felsic segregation phase 9818.5036C.
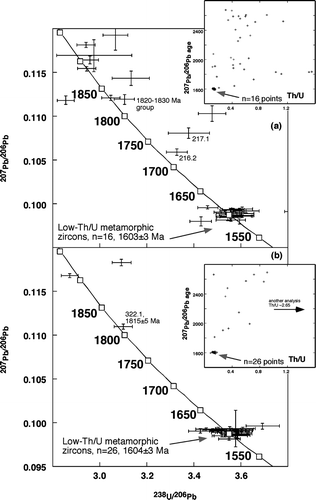
Although the pattern of Late Archean to Paleoproterozoic inherited 207Pb/206Pb ages is broadly similar to that reported by Nutman & Ehlers (Citation1998a), it is not identical, and the differences are significant to the geological outcome. The two oldest grains (209.1, 239.1) are close to 2670 Ma, and there is a range of ages to about 2550 Ma. Nutman & Ehlers (Citation1998a) found this to be the predominant zircon population, from which their Archean protolith basement age of 2654 ± 16 Ma was obtained. Only two of our 53 analyses would fit such an age.
In addition to the various age sub-peaks between 2300 and 1900 Ma, there is another relatively high Th/U age group at ca 1830 – 1820 Ma (grains 216, 236, 237) that represents a detrital component of the metasediment, In contrast, Nutman & Ehlers (Citation1998a) suggested that post-2654 ± 16 Ma zircons may be dating tectonothermal events. We note that none of these postulated events is evident as overgrowths on earlier formed zircon, and it is our view that all the apparent age peaks, from 2600 to ca 1830 – 1820 Ma, are detrital zircon age contributions, the youngest of which can be taken as a maximum age for the metasediment. All of these ages are common provenance inputs in Australian Paleoproterozoic terrains.
Sample 9818.5036C, Felsic Components
Sample 9818.5036C represents in situ melt leucosome ± albitic metasediment layers, and exhibits the same zircon morphologies as sample 5036B, and very similar SHRIMP data (; Appendix 2.9*). However, in the leucosome phase, there is an even more prominent, uniformly low-Th/U (0.15) metamorphic fraction, and 26 analyses define the same metamorphic age, of 1604 ± 3 Ma (MSWD 1.29). It is reasonable to pool all 95 analyses from banded gneiss 5036B and felsic component 5036C (). This provides enough data to recognise six clearer provenance age groupings within the oldest suites at about 2670, 2600 – 2580, 2520 – 2450, 2000, 1920 – 1900 and 1820 Ma.
Figure 9 Relative zircon age probability plots for Farmcote Gneiss samples: (a) 9818.5036; (b) 9818.5036B and 9818.5036C; (c) comparison with sample 95BH15 from Nutman & Ehlers (Citation1998a).
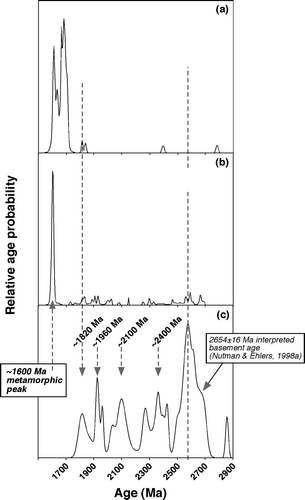
Samples 9818.5036A, 9818.5036, Massive Grey Gneiss
As a means of tightening control on the maximum age of this Farmcote Gneiss sequence, we sampled two massive grey gneiss outcrops (9818.5036A and 9818.5036) within a few metres of the banded gneisses 9818.5036B/C. These massive, medium-grained, equigranular, amphibole- and biotite-bearing gneisses are generally devoid of leucosome. Only monazite was recovered from the first grey gneiss sample (9818.5036A), appearing as subhedral to anhedral grains. The 207Pb/206Pb ages of 1616, 1604 and 1603 Ma (Appendix 2.8*) indicate that the monazite was either formed or recrystallised in the same metamorphic event recorded in the zircon systematics from the banded coarse-grained gneisses.
Zircons from the second massive grey gneiss (9818.5036) are complex, partly recrystallised and resorbed. Many of the grains have residual prismatic and pyramidal faces but are embayed (giving rise to some bulbous- and dumbbell-shaped grains), preferentially altered and recrystallised, and selvedged with high-U metamorphic zircon rims. This partial or complete recrystallisation has affected most of the zircon, but relict primary zircon domains are detectable in both transmitted and cathodoluminescence images. The less-altered, remnant grain centres are sparse, and SHRIMP 207Pb/206Pb ages for these range from Late Archean to Late Paleoproterozoic (2784 to 1705 Ma: ; Appendix 2.10*). The proportion of old grains in this grey gneiss layer is much less than that in samples 5036B/C, and some intermediate age groups are missing (e.g. no ages of ca 2300, 2000 or 1900 Ma). Nutman & Ehlers (Citation1998a) suggested that intermediate age zircons (in their work: 2400, 2100, 1950 and 1820 Ma) were due to separate tectonothermal events. These differences between our data and theirs suggest that these intermediate ages reflect minor provenance ingredients and differences in sediment maturity across stratigraphy ().
Figure 10 Tera – Wasserberg concordia plot for zircon U – Pb SHRIMP data in Farmcote Gneiss sample 9818.5036: 67 zircon data points (circles) between 1700 and 1600 Ma, and (inset) an additional 11 high-Th/U data points (triangles) at 1705 ± 5 Ma.
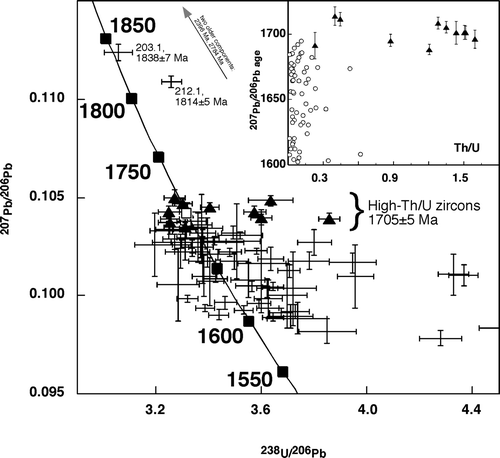
The youngest group of four high-Th/U, probably detrital zircon grains (202, 214, 215, 227), found in the grey gneiss were measured in replicate in order to verify the significance of this group. They are morphologically similar to many other partly resorbed grains and have low to moderately high U but in general have distinctly higher Th/U than most other zircons in the rock ( inset). Like all the other pre-1600 Ma grains, they are rimmed by low-Th/U metamorphic zircon.
Nine analyses within error (MSWD 1.06) give a pooled 207Pb/206Pb age of 1705 ± 5 Ma. We regard this as a viable primary crystallisation age for the source of the high-Th/U zircon group, and it is thus a maximum depositional age for the sedimentary precursor of the grey gneiss. Only seven of the above nine analyses are concordant, but discordance is attributed to recent Pb loss. Two analyses in grain 202 were rejected: in 202.1, the primary ion beam crossed a rim boundary, in discordant analysis 202.3, the ion beam crossed a crack.
Partial Metamorphic Re-Setting in the Massive Grey Gneiss
The high-U, low-Th/U recrystallised and partly recrystallised grains and grain rims from the grey gneiss (9818.5036) have a 100 million year smear in 207Pb/206Pb age between 1700 and 1600 Ma (). The spread of the 63 data points gives a meaningless average age of ca 1650 Ma with considerable internal scatter. If 12 discordant points are rejected, the remaining 51 analyses (>95% concordance) still give a geologically meaningless age of ca 1650 Ma, which corresponds to a probability minimum (age trough). These low-Th/U zircons have morphological vestiges of their primary origins, but are variably isotopically reset, and the data are distributed in a concordant to sub-concordant field between ca 1700 Ma (possibly their primary age) and ca 1600 Ma (approximate metamorphic age) with irresolvable sub-peaks at about 1685, 1665, 1635 and 1610 Ma (). These are fortuitous age blends due to incomplete resetting and are of no geological significance (e.g. grain 28 replicate analyses) This link between the extent of age resetting and Th/U is evident in the whole dataset of analyses between 1700 and 1600 Ma ( inset). These intermediate ages are artefacts of an original age or ages selectively reset by U-rich metamorphic fluids in the ca 1600 Ma event. The 1600 Ma age towards which the low-Th/U grains trend is the age of metamorphism as defined by SHRIMP ages of zircon rims in the adjacent banded gneiss, and also in the metamorphic overgrowths in the nearby 1700 Ma leucogneiss (granitoid) (Page et al. Citation2005a).
Age Range of Granitic Gneisses in the Broken Hill Inlier
Page et al. (Citation2005a) dated the Alma Granite Gneiss from near Broken Hill Airport at 1704 ± 3 Ma, and the Rasp Ridge Granite Gneiss from the Hanging Wall Synform (Broken Hill City) at 1683 ± 3 Ma. These granite gneiss units are interpreted as granites intruded as concordant sheets during Willyama sedimentation. In the present study, other granite gneisses previously correlated with the Alma and Rasp Ridge Granite Gneisses have been dated. Summary results are given in .
Table 4 Summary of new age determinations for granitic gneisses.
Stephens Creek Granite Gneiss
The major granite gneiss body () on the Stephens Creek 1:25 000 sheet (Stroud Citation1989b) was previously subdivided into a stratigraphically lower Alma Gneiss section and a stratigraphically higher Rasp Ridge Gneiss section, separated by a discontinuous screen of metasediments. The body forms the core of a major anticline of probable F2 age and is estimated to be 2 km thick. The granite gneiss body is zoned from the anticlinal core outwards. The innermost zone is characterised by the presence of ‘essential sillimanite aggregates’ (Stroud Citation1989b) (i.e. sillimanite aggregates are a constant feature); the next zone contains variable amounts of K-feldspar megacrysts or augen; the outer zone contains few megacrysts and little or no sillimanite, and is in contact with Broken Hill Group rocks.
Figure 11 Simplified interpretive diagram of lithological zonation in the Stephens Creek Granite Gneiss (data from Stroud Citation1989b), showing sample locations. Metasediment included in the granite gneiss is part of the Thackaringa Group; granite gneiss is enclosed by the Broken Hill Group.
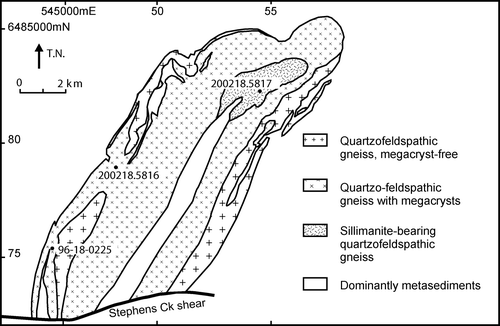
Nutman & Gibson (Citation1998, ) illustrated SHRIMP zircon U – Pb data from a sample of the Stephens Creek Granite Gneiss. Neither a location nor an age was given, but the data points cluster around ca 1690 Ma.
Our sample 200218.5817 was taken from the stratigraphically lowest zone, the sillimanite-bearing quartzo-feldspathic gneiss in the core of the fold. The sample consists of quartz – feldspar domains ∼20 mm × 5 mm, wrapped by biotite laminae, giving it an augen texture. Sericitised sillimanite is present in nearby outcrops, but was not obvious in the sample. The major schistosity dips at 85° to 129° true. Pegmatitic veins comprise about 5 – 10% of the rock in the vicinity of the sample. The subhedral zircon population owes its generally ovoid shapes to the high-U zircon recrystallisation features that form unevenly thick rims on most grains. These resemble the normal ca 1600 Ma metamorphic rims observed elsewhere and were generally avoided for this study. The zoned zircon grain interiors include a few analyses whose 207Pb/206Pb ages indicate inheritance between 2684 and 2604 Ma as well as a few inherited grains at 1907 – 1843 Ma (; Appendix 2.11*). All 29 of the analysed magmatic zircons define a single concordant age population at 1689 ± 5 Ma (MSWD 1.13). Deletion of one somewhat discordant analysis (430.1) does not alter the result, which can be interpreted as the magmatic age for this stratigraphically lower part of the Stephens Creek Granite Gneiss.
Figure 12 Tera – Wasserberg concordia plot for zircon U – Pb SHRIMP data in the Silver City Suite of granitic gneiss samples: (a) 200218.5801 Wondervale Well Granite Gneiss; (b) 200218.5811 Georges Bore Granite Gneiss; (c) 200218.5816 Stephens Creek Granite Gneiss; (d) 200218.5817 Stephens Creek Granite Gneiss.
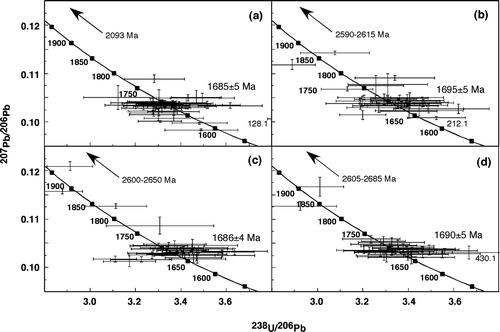
Sample 200218.5816 was from close to the western margin of the body, near its stratigraphic top. The sample was a coarse- to medium-grained quartz – feldspar – biotite (±garnet) gneiss with evenly distributed, small feldspar augen. Some zircon grains retain their magmatic prismatic form, and others have ovoid shapes influenced by strongly recrystallised high-U zircon edges. The pattern of the SHRIMP data is similar to the previous sample, with several inherited 2650 – 2600 Ma grains and younger inheritance between 1970 and 1780 Ma (; Appendix 2.12*). A single concordant age population at 1686 ± 4 Ma (MSWD 1.40, 25 analyses) is interpreted as the best magmatic age for this upper part of the Stephens Creek Granite Gneiss. Three of the youngest analyses that are aberrantly reversely discordant were omitted.
Wondervale Well Granite Gneiss
Sample 200218.5801 from near Wondervale Well is from a body of granite gneiss forming the core of the Edgar Antiform, an anticlinal closure between two areas of Broken Hill Group about 15 km southwest of Broken Hill (Brown Citation1984). It consists of coarse- to medium-grained quartz – feldspar – biotite gneiss, with some zones containing poikiloblastic garnets. The northeastern part of this body has an outer (stratigraphically upper) zone of leucocratic quartz – feldspar – biotite gneiss. Part of the body is rotated into the major Pine Creek Retrograde Schist Zone. Brown (Citation1978) and Slack et al. (Citation1993) described small (20 – 50 mm), round, pebble-like aggregates of quartz, tourmaline and minor alkali feldspar in the leucocratic gneiss phase. These resemble miarolitic cavity fillings, typically found in very shallow intrusions. Brown (Citation1984) correlated this body with the Rasp Ridge Granite Gneiss.
The sample analysed was a quartz – feldspar – biotite gneiss with abundant garnet porphyroblasts and minor pegmatitic veins. The gneissosity is weakly developed, and the pegmatitic veins are folded. The squat, prismatic to ovoid-shaped zircon grains have thick high-U selvedges. Data from 30 zoned magmatic zircon cores form a concordant cluster and a pooled age of 1685 ± 5 Ma (MSWD 1.10). The age is identical to that of the Hores Gneiss and within error of the Rasp Ridge Granite Gneiss measured near Broken Hill (Page et al. Citation2005a). Low-Th/U grain selvedges targeted in SHRIMP analyses 110.1, 121.1, 128.1 and 132.2 (Appendix 2.13*) have apparent 207Pb/206Pb ages between 1637 and 1593 Ma reflecting various degrees of recrystallisation in the Olarian metamorphic event (). Another grain of this age (106.1) is peculiarly young, given its normal magmatic Th/U and does not fit either a metamorphic or magmatic interpretation.
Georges Bore Granite Gneiss
Sample 200218.5811 is from an extensive body (or bodies) of granite gneiss about 7 km northwest of Oakdale homestead. This body was mapped by Stroud (Citation1986) as a coarse- to very coarse-grained quartz – feldspar – biotite gneiss with abundant K-feldspar megacrysts, and lesser fine- to medium-grained gneiss lacking megacrysts. It occupies part of a complex fold structure and is flanked in part by the Himalaya Formation and in part by the Broken Hill Group. The sample is a quartz – feldspar – biotite gneiss with K-feldspar augen to 30 mm long. The zircon population in this sample has a heterogeneous morphology of mostly slender to squat prismatic types and minor tabular grains. Both zircon types are zoned internally and rimmed by high-U zircon overgrowth or unevenly thick recrystallisation features. The concordant grouping of 27 analyses that define the magmatic population give an age of 1695 ± 4 Ma (MSWD 1.16). Some inherited xenocrysts have ages between 2615 and 2590 Ma and between 1870 and 1745 Ma (; Appendix 2.14*). An aberrantly young outlier (212.1) in the main magmatic zircon suite was rejected, as the SHRIMP ion beam may have overlapped onto part of a metamorphic rim.
Ages of Broken Hill Group Rock Units
In the current project, two samples from the Broken Hill Group were dated, one a correlative of Hores Gneiss in the South Australian part of the Broken Hill Domain, the other a quartzo-feldspathic gneiss from the Parnell Formation near the Allendale mine north of Broken Hill.
Sample 200218.5874 is a medium- to coarse-grained quartz – feldspar – biotite – garnet gneiss from southeast of Mingary, South Australia (). The garnet gneisses, interpreted as Hores Gneiss equivalent, occupy the core of an F3 fold formed during the Olarian Orogeny. Shearing in the limbs of the fold has brought the gneiss into contact with upper Thackaringa Group psammites. The SHRIMP U – Pb zircon age of 1684 ± 6 Ma based on 15 grains (Appendix 2.15*) confirms the correlation with Hores Gneiss and the presence of upper Broken Hill Group in this part of South Australia.
Figure 13 Geology of the Mingary area, South Australia, showing Hores Gneiss sample locality in relation to the interpreted Broken Hill Domain—Olary Domain boundary.
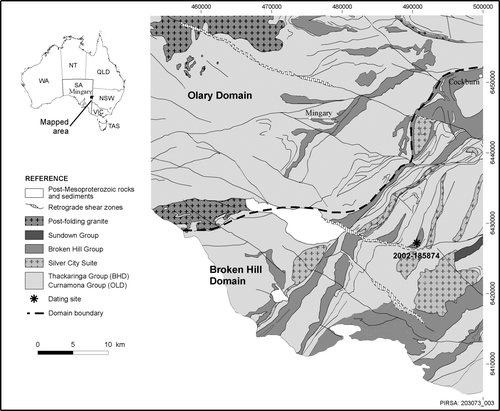
The zircon grains are dominantly rounded and ovoid, with a clear metamorphic imprint. Cathodoluminescence imagery reveals that more than 90% of the zircon grains are recrystallised. Relict protolith zircon (areas free of recrystallisation) remains in some grains, but in all cases this is overgrown by high-U metamorphic zircon rims or recrystallised areas. Fifteen acceptable analyses of zoned cores give an age of 1684 ± 6 Ma (MSWD 0.83), interpreted as a maximum age for the protolith. The youngest (421.1) and next youngest (410.1, with anomalously high U of 989 ppm) zoned cores were rejected. This age is indistinguishable from that of the Hores Gneiss in the upper Broken Hill Group (Page et al. Citation2005a). Older inheritance or detrital ages at ca 1850 and ca 1770 Ma, and older Late Archean and Paleoproterozoic ages, closely resemble the inheritance age pattern found in the Hores Gneiss, further supporting the correlation ().
Figure 14 (a) Tera – Wasserberg concordia plot for zircon U – Pb SHRIMP data in East Mingary Potosi-type gneiss sample 200218.5874; (b, c) age probability comparison of (b) East Mingary Potosi-type gneiss with (c) Hores Gneiss at Yanco Glen, Broken Hill (8618.2001. 106 data points: Page & Laing Citation1992; Page et al. Citation2005a).
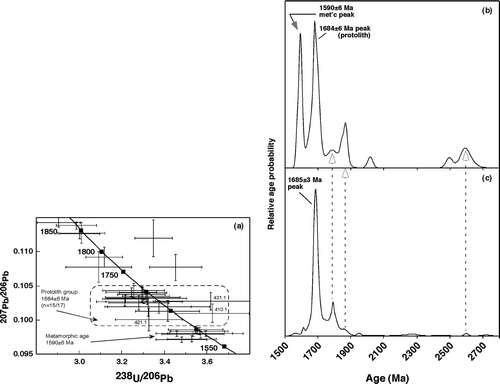
The metamorphic zircon analyses (Appendix 2.15*) are characterised by high-U, low-Th/U and all 10 data points form a single 207Pb/206Pb age distribution. One point (434.1) is discordant, and the remaining nine give the age of this high-grade metamorphic event at 1590 ± 6 Ma (MSWD 1.23). A suite of colourless to pale-green, round to subangular monazite grains was separated, and four 207Pb/206Pb measurements clearly indicate a metamorphic origin with ages between 1606 and 1574 Ma (Appendix 2.16*).
Sample 200218.2005 was taken from the Parnell Formation southeast of the Allendale mine, from the same outcrop as sample 2005 analysed by Page & Laing (Citation1992). The rock unit is a quartz – feldspar – biotite – garnet gneiss (Potosi-type gneiss). The sample was collected to evaluate the previous result in the light of advances in technology. The new SHRIMP data (; Appendix 2.17*) show that the majority of zircon grains in the sampled part of this outcrop are inherited/detrital in origin, including a number of Archean zircons ranging from 3580 to 2520 Ma, and the usual range of Paleoproterozoic ages found elsewhere in the Willyama Supergroup between 2480 and 1750 Ma (). The youngest group of 14 zircon grains gave a 207Pb/206Pb age of 1693 ± 4 Ma (MSWD 1.71). This result is unchanged when one discordant analysis (547.1) and another aberrantly young analysis (545.1 has a high error and the lowest U in the suite) are discarded, and the weighted mean 207Pb/206Pb age of the 12 data points is 1693 ± 4 Ma (MSWD 1.60). This age is identical to the 1693 ± 5 Ma determined by Page & Laing (Citation1992) and is a maximum depositional age.
Figure 15 (a) Tera – Wasserberg concordia plot for zircon U – Pb SHRIMP data in Parnell Formation gneiss 200218.2005; (b, c) age probability comparison of (b) Parnell Formation gneiss 200218.2005 with (c) Parnell Formation sample 8618.2005 (Page & Laing Citation1992).
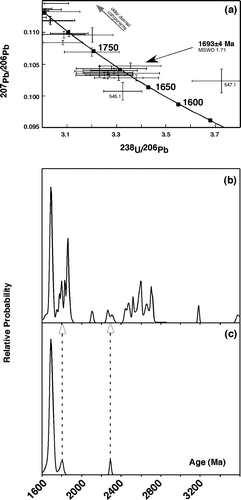
Discussion
Cues Formation
The ca 1700 Ma ages of the two leucogneiss samples (1699 ± 4, 1701 ± 3 Ma) and Potosi-type gneiss 2002 185803 (1700 ± 4 Ma) from the Cues Formation are identical. Given that the two rock types occur very close together in the middle of Cues Formation, we argue that this is a stratigraphic age for the Cues Formation and identifies an igneous event or closely spaced events older than the 1693 ± 4 Ma Ettlewood Calc-Silicate Member of the lower Broken Hill Group (see Page et al. Citation2005a). A more conservative interpretation might take the leucogneiss ages as a minimum age for the stratigraphy (if the leucogneiss was intrusive) and the Potosi-type gneiss age as a maximum age for the stratigraphy (if the Potosi-type gneiss was sedimentary). However, as these ages are virtually coincident, this interpretation also demands that the Cues Formation stratigraphic age is close to 1700 Ma. This clearly demonstrates that the Cues Formation is older than the Broken Hill Group, and not a structural repetition of the Parnell Formation as once proposed (Donaghy et al. Citation1997a, Citationb).
Our tentative conclusion is that the leucogneisses and the Potosi-type gneiss were volcanic or volcaniclastic in origin, and therefore deposition of the middle part of the Cues Formation took place at 1700 ± 4 Ma.
Relationship of Cues Formation to the Alma Granite Gneiss
In the stratigraphic scheme of Stevens et al. (Citation1983) and Willis et al. (Citation1983), the Alma Granite Gneiss is stratigraphically equivalent to the Lady Brassey Formation, the lowest unit of the Thackaringa Group. Both are overlain by the Cues Formation (including the now-discontinued Alders Tank Formation) and then the Himalaya Formation. The megacryst-rich Alma Granite Gneiss was dated by Page et al. (Citation2005a) at 1704 ± 3 Ma and interpreted as a deformed sill-like granite. The 1700 ± 4 Ma age of the leucogneisses and Potosi-type gneiss in the Cues Formation is within error of the 1704 ± 3 Ma age of the underlying Alma Granite Gneiss. If the age of the Cues Formation units represents the age of sedimentation, as we tentatively conclude, then the Alma Granite Gneiss (metagranite) was emplaced under very little cover.
Redan Gneiss and Farmcote Gneiss (Rantyga Group)
The nature of the layering in the thinly layered quartz – sodic plagioclase – hornblende – magnetite (±K-feldspar, epidote) rocks (such as sample 200218.5813) characteristic of the Redan Gneiss suggests a sedimentary origin. Stevens & Corbett (Citation1993 p. 328) considered that the rocks ‘… most likely comprised detritus derived directly from intermediate volcanic rocks.’ However, the pattern of zircon ages implies the rocks were detrital sediments with a range of provenance ages, rather than contemporaneous volcanics. The rocks were more likely to have been siliciclastic sediments with an evaporitic component represented by albitisation and by Ca – Mg carbonate-rich layers, now hornblende – epidote-rich layers. The rocks are very similar in the nature of their layering and in composition, to layered albitic metasediments of the Ethiudna Subgroup, at lower metamorphic grade in the Olary Domain. Some of those metasediments contain rhombs of diagenetic dolomite (or actinolite pseudomorphs), suggesting an evaporitic environment (Cook Citation1992; Cook & Ashley Citation1992). No rhombs have been found in the Redan Gneiss, but the analogy with Ethiudna Subgroup rocks is considered sufficiently strong to interpret an evaporitic component in the thinly layered rocks in the Redan Gneiss.
The poorly layered quartz – albite – K-feldspar – magnetite gneiss provides fewer clues to its origin. The vague layering does not resemble bedding in a sedimentary rock. The grainsize is not coarse, and no relict granitic or volcanic textures have been found. The clustering of zircon protolith ages at 1710 ± 4 Ma, with no detected older zircon, suggests that the rock was igneous and probably a volcanic rock of that age. If the 1710 ± 4 Ma date represents the depositional age of the rock, it is consistent with the interpretation of Stevens & Corbett (Citation1993) that the sequence from Redan Gneiss through Ednas Gneiss and the Mulculca Formation to the 1704 ± 3 Ma Farmcote Gneiss is upward younging and suggests a relatively short depositional time span for the Rantyga Group. The quartz – albite – K-feldspar – magnetite gneiss is tentatively interpreted as an altered (albitised) and metamorphosed volcanic rock.
Archean rocks?
Nutman & Ehlers (Citation1998a) suggested that the Farmcote Gneiss was either Archean or derived from nearby Archean rocks. Our evidence that the banded quartzo-feldspathic gneiss and the grey gneiss are part of a Paleoproterozoic metasedimentary sequence hinges on the interpretation of the younger populations of zircon in these rocks. We find that the youngest is a metamorphic zircon suite, with all the usual characteristics: particularly that they form high-U, low Th/U selvedges on older grains of all ages. The few monazite analyses support this conclusion. This 1600 Ma metamorphic age was not recognised in the Farmcote Gneiss data presented by Nutman & Ehlers (Citation1998a). A few grains of 1820 – 1800 Ma are found in all the dated gneisses from this sequence. Nutman & Ehlers (Citation1998a) considered that their 1820 Ma zircons were the product of the last of four tectonothermal events. Our data do not support this. Not only do the 1820 Ma zircons have no metamorphic features, but they do not rim older zircon, and are rimmed by typical high-U metamorphic zircon that is 1600 Ma. More importantly, the grey gneiss contains a 1705 ± 5 Ma zircon suite interpreted as detrital, thus determining a maximum depositional age. A minimum depositional age of 1703 ± 3 Ma and 1705 ± 3 Ma is defined by the age of an adjacent felsic leucogneiss (deformed granite) (Page et al. Citation2005a). As the maximum and minimum ages of deposition of the Farmcote Gneiss are inseparable, we conclude that this is a good stratigraphic age for this formation.
The new data and constraints outlined above negate Nutman & Ehlers' (Citation1998a) hypothesis that the Farmcote Gneiss is a piece of Archean crustal basement to the Willyama Supergroup. They also negate the conclusion that the gneisses are dominated by Archean zircons and therefore if not actually Archean crust, then ‘… derived largely from nearby Archaean and earliest Palaeoproterozoic crust’ (Nutman & Ehlers Citation1998a p. 693) The Sm – Nd data acquired by the late S-S. Sun (pers. comm. 1999) demonstrate that the Farmcote Gneiss is normal Paleoproterozoic crust with a contribution of detrital Archean crust similar in all respects to that found in many Paleoproterozoic terrains of the world.
Age range of granite gneisses
Earlier geochronological work Page et al. (Citation2005a) identified two periods of granitic intrusion during deposition of the Willyama Supergroup, in the Broken Hill Domain: (i) Alma Granite Gneiss, 1704 ± 3 Ma; and (ii) Rasp Ridge Granite Gneiss, 1683 ± 3 Ma.
The new data show a ∼12 million year spread of granitic intrusion between 1695 Ma and 1683 Ma, in addition to the older Alma Granite Gneiss, as follows: (i) Alma Granite Gneiss, 1704 ± 3 Ma; (ii) Georges Bore Granite Gneiss, 1695 ± 4 Ma; (iii) Stephens Creek Granite Gneiss (lower part), 1689 ± 5 Ma; (iv) Stephens Creek Granite Gneiss (upper part), 1686 ± 4 Ma; (v) Wondervale Well Granite Gneiss, 1685 ± 5 Ma; and (vi) Rasp Ridge Granite Gneiss (Broken Hill City), 1683 ± 3 Ma.
The ages of the Wondervale Well and Stephens Creek Granite Gneisses are within error of that of the Rasp Ridge Granite Gneiss in Broken Hill City, but the Georges Bore Granite Gneiss is significantly older. It could be argued that there were three discrete ages of intrusion, corresponding to the Alma, Georges Bore and Rasp Ridge Granite Gneisses. However, given the continuous overlap of dates between the Georges Bore and Rasp Ridge Granite Gneisses, we consider it preferable to treat this range as a continuum.
The geological contexts of the three newly dated granitic gneisses do not conflict with the geochronological evidence. The Stephens Creek Granite Gneiss was previously subdivided into a stratigraphically lower Alma Gneiss section and a stratigraphically higher Rasp Ridge Gneiss section, separated by a discontinuous screen of metasediments named the Cues Formation (Stroud Citation1989b; Willis et al. Citation1989). The dates from the stratigraphically upper (1686 ± 4 Ma) and lower (1689 ± 5 Ma) parts of the body are within error of each other and within error of Page et al.'s (Citation2005a) date for the Rasp Ridge Granite Gneiss. The lower section of the Stephens Creek Granite Gneiss is too young to correlate with the Alma Granite Gneiss. The discontinuous screen of metasediments separating the lower and upper parts, although named Cues Formation, does not have distinctive features of any particular stratigraphic unit.
The Stephens Creek Granite Gneiss immediately underlies (stratigraphically) typical Parnell Formation (Broken Hill Group) lithologies including banded iron-formation (Mn-garnet – magnetite – quartz – apatite rock). The age range and stratigraphic position of the granite gneiss suggest that this granite was intruded as a concordant sill at a very shallow depth. At the time of emplacement, the cover consisted of part or all of the Broken Hill Group. The data also permit the body to have been a thick volcanic pile. However, constraints on the Rasp Ridge Granite Gneiss in Broken Hill City, dated by Page et al. (Citation2005a), indicate it was intrusive, and by analogy the Stephens Creek Granite Gneiss is also considered intrusive. A ∼2 km-thick granite intruding with its upper surface <1 km below the seafloor might be expected to have some influence on seafloor topography and sedimentation. Yet, no great impact is apparent. The banded iron-formation and quartz – gahnite rocks just above the top of the granite body may be indications of hot-spring activity driven by the shallow magma.
The Wondervale Well Granitic Gneiss is stratigraphically overlain by the Purnamoota Subgroup of the Broken Hill Group. It is dated at 1685 ± 5 Ma, identical to Hores Gneiss, which lies at the top of the Purnamoota Subgroup. This interpreted granite intrusion, when emplaced, was covered solely by the Broken Hill Group, a thickness of <1 km. Interpretation of the round, pebble-like aggregates of quartz, tourmaline and minor alkali feldspar in the leucocratic gneiss phase, described by Brown (Citation1978) and Slack et al. (Citation1993), as miarolitic cavity fillings, supports a shallow intrusive origin for this granite gneiss.
The Georges Bore Granite Gneiss is slightly older than the Rasp Ridge Granite Gneiss, and the Wondervale Well and probably Stephens Creek Granite Gneisses. It also appears to have been emplaced slightly lower in the stratigraphic pile, being overlain by part of the Himalaya Formation and the Broken Hill Group. The 1695 ± 4 Ma age of the granite gneiss is within error of the 1693 ± 3 Ma Ettlewood Calc-Silicate Member (lower Broken Hill Group). It is concluded that the Georges Bore Granite Gneiss was a granitic sill emplaced under shallow cover of the upper Thackaringa and lower Broken Hill Groups.
Revised nomenclature for Broken Hill granitic gneisses
Willis et al. (Citation1983) subdivided Broken Hill granitic gneisses into two stratigraphic units: the Rasp Ridge Gneiss and the Alma Gneiss, based on approximate stratigraphic positions, and influenced by the interpretation that these rocks were metavolcanic. Vassallo & Vernon (Citation2000) provided textural evidence that these gneisses were granite sills, and Page et al. (Citation2005a) provided supporting geochronological evidence. Geochronology in this paper indicates that there is a significant variation in ages of these sills. Consequently, the granitic gneisses are more appropriately dealt with in terms of granite nomenclature. Details are provided in Appendix 1, but the essential scheme is as follows (largely based on concepts by White et al. Citation2001).
Each physically separate granitic gneiss body is given a geographic name, followed by ‘Granite Gneiss’: e.g. the Rasp Ridge Granite Gneiss, Stephens Creek Granite Gneiss, Alma Granite Gneiss. All of the granite gneisses ranging in age from the Alma Granite Gneiss to the Rasp Ridge Granite Gneiss have similar field and chemical characteristics (as far is as known at present) and therefore are grouped as the Silver City Suite. Felsic volcanic rocks within this age range include the Potosi-type gneiss in the Cues Formation and Hores Gneiss, and the leucogneiss dated from the Cues Formation. The Potosi-type gneiss in the Parnell Formation may be included if it is a direct volcanic product, but excluded if it is shown to be a derived sediment, as discussed below. The above volcanic rocks are grouped as the Barrier Suite. In accordance with standard stratigraphic practice, the volcanic rocks also remain part of the Cues Formation and Hores Gneiss (and possibly Parnell Formation). Both the Silver City Suite and the Barrier Suite are part of the Potosi Supersuite, which also includes volcanic and intrusive rocks in the Olary Domain of South Australia.
Constraints on the ages of basic gneisses
This section deals with basic gneisses (amphibolites and basic granulites) that were metamorphosed during the Olarian Orogeny. Post-Olarian dykes affected only by the Delamerian Orogeny (ca 500 Ma) are not considered.
Basic gneiss bodies are abundant within the granitic gneisses. Brown et al. (Citation1983) interpreted the granitic gneisses (Rasp Ridge and Alma Granite Gneisses) as volcanic units and part of the Thackaringa Group. This permitted the basic gneisses in these granitic gneisses to be interpreted as basaltic volcanics and/or sills/dykes, as old as the Thackaringa Group. More recently, the granitic gneisses have been interpreted as granite sills (see discussions above). The basic gneisses show no evidence of being inclusions in the granites and are interpreted as intrusions post-dating the granites. Largely as a result of an unsuccessful 30 year search for pillow structures or other distinctively volcanic textures, and the confinement of the basic gneisses to the middle and lower parts of the sequence, Stevens (Citation1998) interpreted all of the Willyama Supergroup basic gneisses as syn-sedimentary doleritic sills and dykes. However, it was not known whether the basic gneisses all intruded during Broken Hill Group time (and slightly later), or whether intrusion below the Broken Hill Group also took place at earlier times.
All of the dated granitic gneisses are intruded by basic gneiss bodies, providing maximum ages for those basic gneisses. All of the granite gneisses except the Alma Granite Gneiss were emplaced during the time span of deposition of the Broken Hill Group. Therefore, the basic gneisses that intrude them were emplaced during or after Broken Hill Group time. Given that there are no known basic gneisses intruding the Sundown or Paragon Groups, it is likely that all those intruding the granite gneisses, and those intruding the Broken Hill Group, were emplaced during Broken Hill Group time or earliest Sundown Group time. The only published, relatively precise SHRIMP zircon U – Pb date from a basic gneiss is the 1683 ± 5 Ma age from Round Hill (Cues Formation: Nutman & Ehlers Citation1998b). Other published dates (Gibson & Nutman Citation2004) include discordant data and are therefore less reliable.
Relationship of granitic gneisses to Pb – Zn mineralisation
Stevens (Citation2003) suggested that the granite sill now represented as the Rasp Ridge Granite Gneiss may have played a role in the origin of the Broken Hill orebody, perhaps as a heat source lithifying the overlying seal of clayey sediment, thus permitting fracturing and escape of the ore fluid. Heat from the cooling granite may also have perturbed the flow pattern of hydrothermal cells.
From Pb isotope evidence, Parr et al. (Citation2005) concluded that deposition of Broken Hill-type Pb – Zn – Ag mineralisation in the Broken Hill Inlier took place over a period of about 16 million years. If the oldest mineralisation in the Broken Hill orebody is assumed to be the same age as the host Hores Gneiss (1685 ± 3 Ma), then the earliest known Broken Hill-type mineralisation formed at about 1695 Ma (the Pinnacles Pb Lodes). The time span of mineralisation is about the same as the span of granitic intrusion from the Georges Bore Granite Gneiss to the Rasp Ridge Granite Gneiss. The proximity of granitic gneiss may be an important factor in mineral exploration. The time span of mineralisation may also be similar to that of the basic gneisses. They, or related magma chambers at depth, may have supplied much of the heat for hydrothermal circulation cells. The proximity of basic gneisses is a less likely exploration discriminant, because of their ubiquitous distribution.
Broken Hill Group
The current study confirmed a maximum age of 1693 ± 4 Ma for the Potosi-type gneiss in the Parnell Formation. The fact that the great majority of zircon grains in the rock are detrital in origin, with ages ranging from 3580 to 1750 Ma, could cast doubt on whether the 1693 ± 4 Ma grains were derived from volcanism contemporaneous with deposition of the Parnell Formation. However, in the previous sample from this same outcrop, analysed by Page & Laing (Citation1992), almost all of the zircon grains were from the 1693 ± 5 Ma population; they were unabraded and interpreted as contemporaneous volcanic grains. This remarkable variation in zircon populations within a single outcrop is difficult to explain. The zircon assemblage in the Page & Laing (Citation1992) sample is typical of a volcanic rock, while that in our sample is typical of a sediment.
Geological evidence relevant to this problem comes from outcrop and thin-section examination of textures in Potosi-type gneisses in the Parnell Formation and in Hores Gneiss. Stevens & Barron (Citation2002) documented bimodal grainsize textures in Potosi-type gneiss from Hores Gneiss in the Mt Gipps, Rise and Shine, Southern Cross and North mine areas. The bimodal textures are defined by large relict quartz and feldspar phenocrysts in a finer matrix (). The textures are readily seen in thin-sections of samples from the Mt Gipps and Rise and Shine areas, but in higher metamorphic grade areas such as Southern Cross and North mine, they are only identified in thin-section where calc-silicate alteration has preserved fine matrix grainsize. In outcrop, the quartz phenocryst textures are apparent from the Mt Gipps area to parts of the Southern Cross area. In contrast, Potosi-type gneiss from the Parnell Formation does not show bimodal grainsize either in outcrop or in thin-section. Thin-sections from the lowest metamorphic grade area (lowest sillimanite grade) southwest of Mt Gipps homestead show a more or less equigranular texture, resembling that of a sandstone (). The probably detrital quartz and feldspar grains are angular to subrounded, and moderately sorted, with most grains 0.2 – 0.7 mm (medium to coarse sand). Quartz grains preserving phenocryst shapes are relatively rare ().
Figure 16 Comparison of textures of felsic gneisses from the Parnell Formation and Hores Gneiss, from within 400 m of each other, lowest sillimanite metamorphic grade, southwest of Mt Gipps homestead. (a, b) Felsic gneiss from Parnell Formation, field of view 10 mm across: (a) plane-polarised light, showing sandstone-like texture, (b) crossed polars. (c) Parnell Formation sample showing rare, angular quartz grain resembling quartz phenocryst from Hores Gneiss (crossed polars). Field of view 3 mm across. (d) Porphyritic texture in Hores Gneiss, showing quartz phenocrysts and one altered feldspar phenocryst in a fine-grained matrix (crossed polars). Field of view 10 mm across. (a – c) are from GR 550730 6498470 (1966 geoid), and (d) is from GR 551040 6498600 (1966 geoid), all from Zone 54, on the Yanco Glen 1:25 000 map sheet.
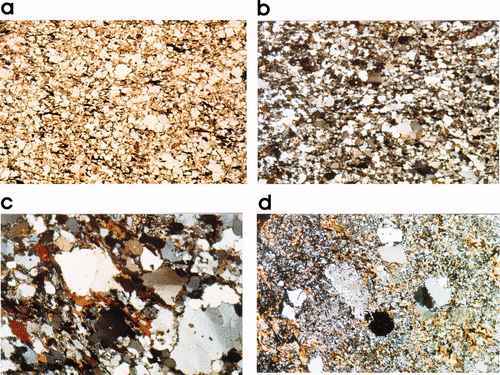
Contrasting zircon populations from a single outcrop may suggest alternating volcanic and non-volcanic detritus, but there is no corresponding change in the major detrital components (e.g. quartz and feldspar). An alternative explanation is that the Potosi-type gneiss was a volcaniclastic sediment incorporating zircon grains winnowed from earlier sediments.
New data on ages of metamorphism and deformation in the Broken Hill Domain
In several samples, zircons with metamorphic character (low Th/U ratio, generally occurring as anhedral and unzoned grains or overgrowths) were analysed. The results, summarised in , are consistent with a high-grade metamorphic event at 1600 – 1590 Ma, as previously determined by Page & Laing (Citation1992) and Page et al. (Citation2005a). No metamorphic zircon older than ca 1600 Ma was detected. However, zircon grains from sample 2002 185813 from the Redan Gneiss gave a significantly younger age of 1574 ± 4 Ma. Love (Citation1992) also determined anomalously young ages of 1563 (+20/ – 7) Ma for zircon grains from the Redan Gneiss. A possible explanation is that these zircons grew from fluids after peak metamorphism.
Table 5 U – Pb ages of zircon grains considered to be metamorphic in origin.
No metamorphic zircon was dated from the interval 1700 – 1655 Ma during which Gibson & Nutman (Citation2004) and Gibson et al. (Citation2004) proposed a high-grade metamorphic extensional event. Hence, this proposal is not supported (see also Conor et al. Citation2005; Stevens Citation2006b).
Conclusions
The current study confirms that most, if not all, of the rocks exposed in the Broken Hill Domain are part of a relatively continuous Willyama Supergroup sequence. The Thackaringa Group pre-dates the Broken Hill Group without a major time break. The Redan geophysical zone, which contains the Redan and Farmcote Gneisses (Rantyga Group), is not an Archean or Early Paleoproterozoic basement, but is only slightly older than the Thackaringa Group. The only portion of the Willyama Supergroup in the Broken Hill Domain now not constrained by geochronology is the Clevedale Migmatite and Thorndale Composite Gneiss, mapped as the lowest stratigraphic units in the main part of the domain. These units appear to be conformably overlain by the Thackaringa Group, inferring that they are approximate age equivalents of the Rantyga Group.
A history of granitic magmatism and felsic volcanism extends from ca 1710 to ca 1683 Ma. Acid volcanism was associated with deposition of the Redan Gneiss, Cues Formation, Ettlewood Calc-Silicate Member and Hores Gneiss. The Parnell Formation may not contain any direct volcanic deposits, but may be a volcanic sandstone derived from reworking of slightly older volcanic products. Sill-like granitic intrusions were emplaced at ca 1704 Ma (Alma Granite Gneiss and leucogneiss intruding the Farmcote Gneiss) and over the time interval from ca 1695 to ca 1683 Ma (Georges Bore, Stephens Creek, Wondervale Well and Rasp Ridge Granite Gneisses). All of the granitic bodies were emplaced less than 15 million years after deposition of their host rocks: the Alma Granite Gneiss and the leucogneiss intrusion into the Farmcote Gneiss are virtually the same age as their host metasediments. All of these granitic sills were very high-level intrusions, emplaced within a kilometre of the surface.
Parr et al. (Citation2005) showed that Broken Hill-type mineralisation in the Broken Hill Domain was formed over a ∼14 million year period, commencing at about 1695 Ma. This corresponds closely with the range of intrusion ages of the Georges Bore, Rasp Ridge, Stephens Creek, Wondervale Well and Rasp Ridge Granite Gneisses. Stevens (Citation2003) suggested that the Rasp Ridge Granite Gneiss may have played a role in the origin of the Broken Hill orebody, either in supplying heat to a convection cell or in focusing the hydrothermal fluids. It is possible that the shallow granitic intrusions were an essential component of the hydrothermal system(s) that formed all of the Broken Hill-type deposits.
We confirm the presence of Hores Gneiss in the Mingary area of South Australia, supporting mapping by Crooks & Hill (Citation2002) who recognised the prospective upper part of the Broken Hill Group in the Mutooroo – Mingary area.
The earliest high-grade metamorphism detected in this study was at ca 1600 – 1590 Ma, the age of the Olarian Orogeny, reinforcing the results of previous studies (Page & Laing Citation1992; Page et al. Citation2005a, Citationb). An unusual phenomenon confirmed in this study is the existence of euhedral metamorphic zircon grains. Normally metamorphic zircon is anhedral, and euhedral zircons are igneous. However, in the Redan Gneiss, the youngest zircon forms euhedral overgrowths on a range of older zircons and also forms separate euhedral grains. The euhedral zircon exhibits high U content and low Th/U ratios, and is unzoned. An apparently younger metamorphic age of 1575 ± 4 Ma was calculated for one sample of Redan Gneiss, inconsistent with the other sample, which gave an Olarian metamorphic age of 1589 ± 5 Ma. A possible explanation is that these zircons grew from fluids after peak metamorphism. This would be consistent with the euhedral shapes of these zircon grains, unexpected for strictly metamorphic zircon grains.
Acknowledgements
This work was undertaken as part of the joint Broken Hill Exploration Initiative (BHEI) between Geoscience Australia, New South Wales Department of Primary Industries, and Primary Industries and Resources South Australia. RWP acknowledges an extended Visiting Fellowship in the Research School of Earth Sciences, Australian National University, and is especially grateful to John Gamble for a Visiting Fellowship in 2005 at the Geology Department, University College, Cork, Ireland. We thank Chris Foudoulis, Tas Armstrong, and Steve Ridgeway of Geoscience Australia for their committed technical expertise throughout the course of this work. We thank Colin Conor for helpful comments on an early version of the manuscript, and Wolfgang Preiss and an anonymous reviewer for very useful comments. This paper is published with permission of the Deputy Director General, New South Wales Primary Industries Mineral Resources and with permission of the Executive Director, Primary Industries and Resources South Australia.
Additional information
Notes on contributors
A. Crooks‡
‡Present address: Uranium Equities Limited, Level 6 West, 50 Grenfell Street, Adelaide SA 5000, Australia.Notes
Appendix 2[indicated by an asterisk (*) in the text and listed at the end of the paper] is a Supplementary Paper; copies may be obtained from the Geological Society of Australia's website (www.gsa.org.au) or from the National Library of Australia's Pandora archive (http://nla.gov.au/nla.arc-25194).
Potosi-type gneiss is a quartz – feldspar – biotite – garnet gneiss, generally with medium to fine grainsize and evenly distributed garnets, interpreted as a metavolcanic rock.
References
- Barron , L. M. 1981 . The petrology and geology of the Stephens Creek granite gneiss body . Geological Survey of New South Wales Report , GS1981/040 (unpubl.)
- Black , L. P. , Kamo , S. L. , Williams , I. S. , Mundil , R. , Davis , D. W. , Korsch , R. J. and Foudoulis , C. 2003 . The application of SHRIMP to Phanerozoic geochronology: a critical appraisal of four zircon standards . Chemical Geology , 200 : 171 – 188 .
- Bradley , G. M. and Brown , R. E. 1988 . Rockwell 1:25 000 Geological Sheet 7233-IV-N , Sydney : Geological Survey of New South Wales .
- Brown , R. E. 1978 . The geology of the Pinnacles 1:25 000 Sheet area, Broken Hill, New South Wales . Geological Survey of New South Wales Report , GS1978/057 (unpubl.)
- Brown , R. E. 1984 . Pinnacles 1:25 000 Geological Sheet, 7133-I-N , Sydney : Geological Survey of New South Wales .
- Brown , R. E. , Stevens , B. P. J. , Willis , I. L. , Stroud , W. J. , Bradley , G. M. and Barnes , R. G. 1983 . “ 3. Quartzo-feldspathic rocks ” . In Rocks of the Broken Hill Block: their Classification, Nature, Stratigraphic Distribution and Origin Edited by: Stevens , B. P. J. and Stroud , W. J. 127 – 226 . Records of the Geological Survey of New South Wales 21(1)
- Compston , W. , Williams , I. S. and Meyer , C. 1984 . U – Pb geochronology of zircons from Lunar breccia 73217 using a sensitive high mass-resolution ion microprobe . Journal of Geophysical Research , 89 : B525 – B534 .
- Conor , C. H. H. 2000 . Definition of major sedimentary and igneous units of the Olary Domain, Curnamona Province . MESA Journal , 19 : 51 – 56 .
- Conor , C. H. H. , Preiss , W. V. , Page , R. W. , Stevens , B. P. J. , Plimer , I. R. and Ashley , P. M. 2005 . Discussion on ‘Detachment faulting and bimodal magmatism in the Palaeoproterozoic Willyama Supergroup, south-central Australia: keys to recognition of a multiply deformed Precambrian metamorphic core complex.’ . Journal of the Geological Society of London , 162 : 409 – 416 .
- Cook , N. D. J. 1992 . “ Geology of metamorphosed Proterozoic playa lake deposits, Olary Block, South Australia ” . Armidale : University of New England . PhD thesis (unpubl.)
- Cook , N. D. J. and Ashley , P. M. 1992 . Meta-evaporite sequence, exhalative chemical sediments and associated rocks in the Proterozoic Willyama Supergroup, South Australia: implications for metallogenesis . Precambrian Research , 56 : 211 – 226 .
- Corbett , G. J. and Willis , I. L. 1989 . Redan 1:25 000 Geological Sheet 7233-IV-S , Sydney : Geological Survey of New South Wales .
- Crooks , A. F. 2001 . Olary – Broken Hill domain boundary—Mingary 1:100 000 map area, Curnamona province . MESA Journal , 20 : 44 – 45 .
- Crooks , A. F. and Hill , P. W. 2002 . Mutooroo North Geological Map, Digital Geological Maps of South Australia 1:25 000 Series , Adelaide : Geological Survey of South Australia .
- Cumming , G. L. and Richards , J. R. 1975 . Ore lead isotopes in a continuously changing earth . Earth and Planetary Science Letters , 28 : 155 – 171 .
- Daly , S. J. , Fanning , C. M. and Fairclough , M. C. 1998 . Tectonic evolution and exploration potential of the Gawler Craton, South Australia . AGSO Journal of Australian Geology & Geophysics , 17 : 145 – 168 .
- Donaghy , A. G. , Hall , M. and Gibson , G. Structure and geochronology of the Thackaringa region: implications for stratigraphic interpretation . Broken Hill Exploration Initiative Conference Abstracts . Edited by: Denham , D. pp. 30 – 33 . Australian Geological Survey Organisation Record 1997/49
- Donaghy , A. G. , Hall , M. and Gibson , G. 1997b . Structure high grade metamorphism and stratigraphy at Thackaringa, Broken Hill, NSW . Geological Society of Australia Abstracts , 46 : 21
- Donaghy , A. G. , Hall , M. , Gibson , G. and Nutman , A. 1998 . The age of deposition and first deformation of the Palaeoproterozoic Willyama Supergroup, Broken Hill, Australia . Geological Society of Australia Abstracts , 49 : 118
- Ehlers , K. and Nutman , A. P. Thermochronological evolution of the Willyama Complex . Abstracts, Geodynamics and Ore Deposits Conference . Edited by: Price , G. pp. 26 – 28 . Ballarat : Australian Geodynamics Cooperative Research Centre .
- Gibson , G. M. and Nutman , A. P. 2004 . Detachment faulting and bimodal magmatism in the Palaeoproterozoic Willyama Supergroup, south-central Australia: keys to recognition of a multiply deformed Precambrian metamorphic core complex . Journal of the Geological Society of London , 161 : 55 – 66 .
- Gibson , G. M. , Peljo , M. and Chamberlain , T. 2004 . Evidence and timing of crustal extension versus shortening in the early tectonothermal evolution of a Proterozoic continental rift sequence at Broken Hill, Australia . Tectonics , 23 : TC5012 doi:10.1029/2003TC001552
- Haydon , R. C. and McConachy , G. W. The stratigraphic setting of Pb – Zn – Ag mineralization at Broken Hill . Economic Geology , 82 826 – 856 .
- Laing , W. P. 1996 . “ Volcanic related origin of the Broken Hill Pb + Zn + Ag deposit, Australia ” . In New Developments in Broken Hill Type Deposits , Edited by: Pongratz , J. and Davidson , G. J. 53 – 56 . CODES Special Publication 1 .
- Laing , W. P. , Sun , S.-S. and Nesbitt , R. W. 1984 . Acid volcanic precursor to Potosi Gneiss at Broken Hill and its implications for ore genesis . Geological Society of Australia Abstracts , 12 : 318 – 321 .
- Laing , W. P. , Marjoribanks , R. W. and Rutland , R. W. R. 1978 . Structure of the Broken Hill mine area and its significance for the genesis of the orebodies . Economic Geology , 73 : 1112 – 1136 .
- Love , S. 1992 . “ Possible ages and origins for some rocks of the mid-Proterozoic Willyama Supergroup New South Wales ” . Canberra : Australian National University . BSc (Hons) thesis (unpubl.)
- Mortimer , G. E. 1984 . “ Early to Middle Proterozoic granitoids, basaltic dikes, and associated layered rocks of S.E. Eyre Peninsula, South Australia ” . Adelaide : University of Adelaide . PhD thesis (unpubl.)
- Muir , R. J. , Ireland , T. R. , Weaver , S. D. and Bradshaw , J. D. 1996 . Ion microprobe dating of Palaeozoic granitoids: Devonian magmatism in New Zealand and correlations with Australia and Antarctica . Chemical Geology , 127 : 191 – 210 .
- Nutman , A. P. and Ehlers , K. 1998a . Archaean crust near Broken Hill? . Australian Journal of Earth Sciences , 45 : 687 – 694 .
- Nutman , A. P. and Ehlers , K. 1998b . Evidence for multiple Palaeoproterozoic thermal events and magmatism adjacent to the Broken Hill Pb – Zn – Ag orebody, Australia . Precambrian Research , 90 : 203 – 238 .
- Nutman , A. P. and Gibson , G. M. 1998 . “ Zircon ages from metasediments, granites, and mafic intrusions: reappraisal of the Willyama Supergroup ” . In Broken Hill Exploration Initiative Conference, October 1998 Edited by: Gibson , G. M. 86 – 88 . Australian Geological Survey Organisation Record 1998/25
- Page , R. W. , Conor , C. H. H. , Stevens , B. P. J. , Gibson , G. M. , Preiss , W. V. and Southgate , P. N. 2005b . Correlation of Olary and Broken Hill Domains, Curnamona Province: possible relationship to Mount Isa and other north Australian Pb – Zn – Ag-bearing successions . Economic Geology , 100 : 663 – 676 .
- Page , R. W. and Laing , W. P. 1992 . Felsic metavolcanic rocks related to the Broken Hill Pb – Zn – Ag orebody, Australia: geology, depositional age, and timing of high-grade metamorphism . Economic Geology , 87 : 2138 – 2168 .
- Page , R. W. , Stevens , B. P. J. and Gibson , G. M. 2005a . Geochronology of the sequence hosting the Broken Hill Pb – Zn – Ag orebody, Australia . Economic Geology , 100 : 633 – 661 .
- Parr , J. M. , Stevens , B. P. J. , Carr , G. R. and Page , R. W. 2005 . Subseafloor origin for Broken Hill Pb – Zn – Ag mineralization, New South Wales, Australia . Geology , 32 : 589 – 592 .
- Pidgeon , R. T. 1967 . A rubidium – strontium geochronological study of the Willyama Complex, Broken Hill, Australia . Journal of Petrology , 8 : 283 – 324 .
- Plimer , I. R. 1977 . The origin of the albite-rich rocks enclosing the cobaltian pyrite deposits at Thackaringa, N.S.W., Australia . Mineralium Deposita , 12 : 175 – 187 .
- Slack , J. F. , Palmer , M. R. , Stevens , B. P. J. and Barnes , R. G. 1993 . Origin and significance of tourmaline-rich rocks in the Broken Hill district, Australia . Economic Geology , 88 : 505 – 541 .
- Steiger , R. H. and Jäger , E. 1977 . Subcommission on geochronology: convention on the use of decay constants in geo- and cosmochronology . Earth and Planetary Science Letters , 36 : 359 – 362 .
- Stevens , B. P. J. The origins of Broken Hill rock types and their relevance to mineralisation . Broken Hill Exploration Initiative Conference, October 1998 . Edited by: Gibson , G. M. pp. 109 – 114 . Australian Geological Survey Organisation Record 1998/25
- Stevens , B. P. J. 1999 . Evidence for multiple Palaeoproterozoic thermal events and magmatism adjacent to the Broken Hill Pb – Zn – Ag orebody, Australia—Discussion . Precambrian Research , 98 : 1 – 5 .
- Stevens , B. P. J. Understanding the Broken Hill Pb – Zn fluid system . Broken Hill Exploration Initiative Conference May 2000 . Edited by: Peljo , M. pp. 166 – 169 . Australian Geological Survey Organisation Record 2000/10
- Stevens , B. P. J. Advances in understanding Broken Hill geology . Broken Hill Exploration Initiative Conference September 2006 . Edited by: Korsch , R. J. and Barnes , R. G. pp. 166 – 175 . Geoscience Australia Record 2006/21
- Stevens , B. P. J. 2006b . Comment on ‘Evidence and timing of crustal extension versus shortening in the early tectonothermal evolution of a Proterozoic continental rift sequence at Broken Hill, Australia’ by G. M. Gibson, M. Peljo & T. Chamberlain 2004 . Tectonics , 25 : TC4009 doi:10.1029/2005TC001853
- Stevens , B. P. J. and Barron , L. M. 2002 . Volcanic textures in the Palaeoproterozoic Hores Gneiss, Broken Hill, Australia . Geological Survey of New South Wales Quarterly Notes , 113 : 1 – 22 .
- Stevens , B. P. J. and Corbett , G. J. 1993 . The Redan geophysical zone: part of the Willyama Supergroup? Broken Hill, Australia . Australian Journal of Earth Sciences , 40 : 319 – 338 .
- Stevens , B. P. J. , Willis , I. L. , Brown , R. E. and Stroud , W. J. 1983 . The Early Proterozoic Willyama Supergroup: definitions of stratigraphic units from the Broken Hill Block, New South Wales . Geological Survey of New South Wales Record , 21 : 407 – 442 .
- Stroud , W. J. 1978a . Geology of the Stephens Creek 1:25 000 sheet Broken Hill, New South Wales . Geological Survey of New South Wales Report , GS1978/060 (unpubl.)
- Stroud , W. J. 1978b . Geology of the Oakdale 1:25 000 sheet area, Broken Hill, New South Wales . Geological Survey of New South Wales Report , GS1978/053 (unpubl.)
- Stroud , W. J. 1986 . Oakdale 1:25 000 Geological Sheet, 7133-III-N , Sydney : Geological Survey of New South Wales .
- Stroud , W. J. 1989a . Triple Chance 1:25 000 Geological Sheet, 7133-IV-S , Sydney : Geological Survey of New South Wales .
- Stroud , W. J. 1989b . Stephens Creek 1:25 000 Geological Sheet, 7234-III-N , Sydney : Geological Survey of New South Wales .
- Tucker , D. H. The characteristics and interpretation of regional magnetic and gravity fields in the Broken Hill district . Broken Hill Conference July 1983 . pp. 81 – 114 . Australasian Institute of Mining and Metallurgy Conference Series 12
- Vassallo , J. J. and Vernon , R. H. 2000 . Origin of megacrystic felsic gneisses at Broken Hill . Australian Journal of Earth Sciences , 47 : 733 – 748 .
- White , A. J. R. , Allen , C. M. , Beams , S. D. , Carr , P. F. , Champion , D. C. , Chappell , B. W. , Wyborn , D. and Wyborn , L. A. I. 2001 . Granite suites and supersuites in eastern Australia . Australian Journal of Earth Sciences , 48 : 515 – 530 .
- Williams , I. S. and Claesson , S. 1987 . Isotopic evidence for the Precambrian provenance of Caledonian metamorphism of the high grade paragneisses from the Seve Nappes, Scandinavian Caledonides: II. Ion microprobe zircon U – Th – Pb . Contributions to Mineralogy and Petrology , 97 : 205 – 217 .
- Willis , I. L. 1982 . Description and interpretation of a useful leucogneiss stratigraphic marker in the Willyama Complex, Broken Hill Block, N.S.W. . Journal and Proceedings of the Royal Society of New South Wales , 115 : 21 – 32 .
- Willis , I. L. 1985 . Thackaringa 1:25 000 Geological Sheet,7133-IV-N , Sydney : Geological Survey of New South Wales .
- Willis , I. L. 1988 . Kinalung 1:25 000 Geological Sheet , Sydney : Geological Survey of New South Wales .
- Willis , I. L. , Brown , R. E. , Stroud , W. J. and Stevens , B. P. J. 1983 . The Early Proterozoic Willyama Supergroup: stratigraphic subdivisions and interpretations of high- to low-grade metamorphic rocks in the Broken Hill Block, New South Wales . Journal of the Geological Society of Australia , 30 : 195 – 224 .
- Willis , I. L. , Stevens , B. P. J. , Barnes , R. G. , Bradley , G. M. , Brown , R. E. and Stroud , W. J. 1989 . Broken Hill Stratigraphy Map 1:100 000 , Sydney : Geological Survey of New South Wales .
- Wyborn , L. A. I. , Budd , A. R. and Bastrakova , I. V. 1997 . The metallogenic potential of Australian Proterozoic granites. GIS Data Package , Canberra : Australian Geological Survey Organisation .
Appendix 1: Definitions of stratigraphic units
Farmcote Gneiss (new name)
The name Farmcote Gneiss is applied to an area of rocks that were previously interpreted as part of the Lady Brassey Formation. The change has been made because neither the association of lithologies nor the magnetic character is typical of Lady Brassey Formation, and because the stratigraphic position relative to the Thackaringa Group is uncertain.
Derivation of name Farmcote homestead is situated very close to the main outcrops.
Previous work Shown as Lady Brassey Formation by Bradley & Brown (Citation1988), Willis (Citation1988), Corbett & Willis (Citation1989), Willis et al. (Citation1989) and Stevens & Corbett (Citation1993). Nutman & Ehlers (Citation1998a) referred to all or part of the Farmcote Gneiss informally as ‘Farmcote gneiss,’ while Page et al. (Citation2005a , ) used the formal term ‘Farmcote Gneiss’ but did not define it.
Distribution The Farmcote Gneiss is the northwesternmost and uppermost stratigraphic unit in the Redan geophysical zone of Stevens & Corbett (Citation1993). It extends from 10 km east of Farmcote homestead, westwards to the Mulculca Fault, and also crops out southwest of Huonville homestead.
Type section No exposed section fulfils the ideal criteria for a type section. The type section nominated between GRs 570250E 6454800N and 569500E 6450000N (Zone 54 AGD1966) (southeast of Farmcote homestead) exhibits the upper contact with the Cues Formation, and shows all of the component rock types. However, structural complications could mean that the southern half of the section is a partial repetition of the northern half. A reference section is nominated south of Huonville homestead, between GRs 553600E 6447100N and 556850E 6445850N, showing good outcrop of the upper half of the Farmcote Gneiss.
Description The component rock types were described by Stevens & Corbett (Citation1993) as Association A: leucocratic albite – quartz-rich metasediments (Pl symbol on published maps), leucocratic quartz – K-feldspar – plagioclase (±biotite, magnetite) gneiss (leucogneiss Lf), amphibolite/basic granulite (although it is believed that granulite-facies metamorphic grade was attained, most of the basic gneisses have retrogressed to amphibolite). The amphibolite/basic granulite bodies were probably dolerite sills/dykes (Stevens Citation1998) intruded before or during Broken Hill Group time. They are therefore not part of this stratigraphic unit, although sills tend to cluster at certain levels of the stratigraphy. Nutman & Ehlers (Citation1998a) noted that one of the leucogneiss bodies is discordant to layering in the enclosing albitic rocks (altered metasediments) and interpreted it as a deformed leucogranite. However, low-angle discordance between massive and layered rock units in a highly deformed terrain could result from rheological differences. Page et al. (Citation2005a) and this paper showed that the age of the leucogneiss and the host metasediments are within error, permitting the leucogneiss to have been volcanic in origin, although more likely a granitic or subvolcanic intrusion. Hence, it is uncertain whether the leucogneisses should be considered part of the stratigraphic unit.
Thickness It is difficult to estimate the true thickness of the Farmcote Gneiss; outcrop width varies from about 1 to 5 km. True thickness may be of the order of 1 – 2 km.
Relationships Relationships to apparently underlying and overlying units are unclear, largely as a result of the scarcity of outcrop. The Farmcote Gneiss forms the uppermost part of the very magnetic Redan geophysical zone, apparently overlain by the Cues Formation of the Thackaringa Group. It is unclear whether the upper boundary is a stratigraphic boundary, or a folded fault, but the age of 1705 ± 5 Ma is consistent with the Farmcote Gneiss correlating with the lower Thackaringa Group. Stevens (Citation2006a) introduced the name Rantyga (pronounced ran-teega) Group to include the four formations comprising the Redan geophysical zone, from base to top: Redan Gneiss, Ednas Gneiss, Mulculca Formation, Farmcote Gneiss. There is no outcrop of the lower contact of the Farmcote Gneiss with the Mulculca Formation, but on a regional scale the two are conformable.
Age Maximum 1705 ± 5 Ma (this paper), minimum 1703 ± 3 Ma, 1705 ± 3 Ma (Page et al. Citation2005a). SHRIMP U – Pb zircon dating.
Silver City Suite (new name)
Willis et al. (Citation1983) observed that a number of granitic gneiss bodies approximately occupy two stratigraphic positions and interpreted them as metamorphosed acid volcanic rocks, part of the Thackaringa Group. More recent evidence, especially geochronology, has shown that these gneisses are metamorphosed and deformed granites (see text). Therefore, they are excluded from the Thackaringa Group. The Silver City Suite is erected to accommodate these metagranites.
Derivation of name After the Silver City Highway which passes close to, or over several of the metagranites.
Constituent units Alma Granite Gneiss, Georges Bore Granite Gneiss, Stephens Creek Granite Gneiss, Wondervale Well Granite Gneiss, Rasp Ridge Granite Gneiss and other unnamed granite gneiss bodies.
Previous work Described as Rasp Ridge Gneiss and Alma Gneiss by Stevens et al. (Citation1983) and Willis et al. (Citation1983). Individual bodies described elsewhere (see below). Characteristics of the metagranites were described by Brown et al. (Citation1983). Wyborn et al. (Citation1997) discussed the geochemistry as part of their ‘Potosi Supersuite.’
Distribution Mainly concentrated in a belt extending northeastwards from near Oakdale homestead, through Broken Hill to Stephens Creek and in the Euriowie Inlier near Paringa homestead. Other metagranite bodies include those north of Thackaringa, south of Silverton, at Mundi Mundi Creek and in the eastern part of the Darling Range.
Type body The large Stephens Creek Granite Gneiss body is nominated as the type metagranite body.
Description The Silver City Suite of metagranites are quartz – feldspar – biotite gneisses with or without garnet, sillimanite and/or retrograde muscovite. The gneisses include evenly medium- to fine-grained varieties and megacrystic varieties. Leucocratic variants occur in some bodies. Partial melting during the ca 1600 Ma Olarian Orogeny produced granitic to pegmatitic leucosome veins and patches, constituting up to 20% of the volume of the granite gneisses.
Wyborn et al. (Citation1997) classed the “Potosi Supersuite', including the proposed Silver City Suite, as S-type, restite-rich and showing no evidence of fractionation.
Dimensions The granite gneiss bodies tend to be elongate parallel to the enclosing stratigraphy, except where they occupy fold closures. The largest body is the Stephens Creek Granite Gneiss, a mass 18 km × 5 km occupying a fold hinge, while some of the smaller bodies are 50 – 300 m across and a few kilometres in length.
Relationships All of the granite gneiss bodies of the Silver City Suite are intrusive into the Thackaringa Group and/or into lower Broken Hill Group. The Alma Granite Gneiss intrudes at the lowest stratigraphic level, into the Alders Tank Formation or the lowest part of the Cues Formation. The Rasp Ridge Granite Gneiss and the Wondervale Well Granite Gneiss were emplaced at the top of the Thackaringa Group. Zircon U – Pb dating (see text) indicates that granite emplacement took place no more than ∼10 million years after deposition of the host sedimentary rocks.
Age Ranges from 1704 ± 3 Ma to 1683 ± 3 Ma. SHRIMP U – Pb zircon dating: Page et al. Citation2005a and this paper.
Rasp Ridge Granite Gneiss (modified from Stevens et al. Citation1983)
After Laing et al. (Citation1978) correlated the previously named ‘upper’ and ‘lower’ granite gneisses, Stevens et al. (Citation1983) defined the Rasp Ridge Gneiss to include both of these and many other granite gneiss bodies throughout the Broken Hill district. The name is here modified to Rasp Ridge Granite Gneiss and confined to the bodies on either side of the Broken Hill Line of Lode, correlated across the Broken Hill Antiform by Laing et al. (Citation1978). Some of the other bodies previously classed as Rasp Ridge Gneiss are not of the same age (see earlier discussion).
One mass crops out discontinuously from near Kelly's Creek, passing west of White Leeds mine to the closure of the Hanging Wall Synform at Block 10 Hill in Broken Hill City. The other main mass crops out in the hinge of the Broken Hill Synform, extending along the western limb through Rasp Ridge and south of Broken Hill City. In the eastern limb, it terminates abruptly.
Wondervale Well Granite Gneiss (new name)
Derivation of name Wondervale Well lies 5 km west-northwest of the North Pinnacle, and ∼2 km northwest of the granite gneiss body.
Previous work The Wondervale Well Granite Gneiss was mapped and described by Brown (Citation1978, 1984) and Brown et al. (Citation1983).
Distribution The body extends in a northeasterly direction for about 5 km, crossing the Indian-Pacific rail line 3 km west of the North Pinnacle. At its northeastern tip, it enters the Pine Creek Schist Zone, where it is attenuated and dragged northwest for 2 km.
Type section A type section is nominated from GR 529230E 6456700N to GR 5294750E 6456270N (Zone 54 AGD 1966).
Description The body occupies the core of the Edgar Anticline. It is zoned, with complex inner zones of biotite-rich and biotite – garnet-rich granite gneiss, enclosed in a partial outer zone of leucocratic biotite-poor gneiss. Brown (Citation1978) and Slack et al. (Citation1993) described aggregates of quartz – tourmaline and minor alkali feldspar.
Dimensions The main part of the body crops out over a length of 5 km and width of ∼500 m.
Relationships Rocks of the Purnamoota Subgroup (probably the Parnell Formation) occur adjacent; the Wondervale Well Granite Gneiss intruded at or just above the base of the Broken Hill Group.
Age 1685 ± 5 Ma. SHRIMP U – Pb zircon dating: this paper.
Stephens Creek Granite Gneiss (new name)
Derivation of name Stephens Creek flows (very occasionally) across the southern, poorly outcropping part of this granite gneiss.
Previous work The body was mapped and described by Stroud (Citation1978a, Citation1989b) and Barron (Citation1981). Willis et al. (Citation1983) correlated the stratigraphically upper part with the Rasp Ridge Gneiss, and the much larger lower part with the Alma Gneiss. Geochronology (see text) indicates that the correlation with the Alma Gneiss is invalid, and that the upper and lower sections are likely to be the same age. Consequently, it is now regarded as a single (meta-) granite intrusion.
Distribution and dimensions Occupies the core of the Stephens Creek Fold, 18 km long and 5 km across the folded width. Thickness before folding was probably about 2 km.
Type section Between GR 529230E 6456700N and GR 529475E 6456270N (Zone 54 AGD 1966). This is a double thickness type section across the width of the Stephens Creek Fold (Stroud Citation1989b). The grid references above are both on the stratigraphic top of the Stephens Creek Gneiss. The mid-point of the line is close to the probable base of the body.
Description The Stephens Creek Granite Gneiss comprises three main varieties of granite gneiss (Stroud Citation1978a, Citation1989b) (simplified in ), zoned as follows: (i) the lowest zone consists of medium- to coarse-grained quartz – feldspar – biotite gneiss with sillimanite aggregates; (ii) the middle zone consists of medium- to coarse-grained gneiss with abundant K-feldspar megacrysts; and (iii) the relatively thin upper zone consists of medium- to coarse-grained quartz – feldspar – biotite gneiss with few megacrysts.
Amphibolite/basic granulite sills and dykes are abundant in the upper half of the Stephens Creek Granite Gneiss.
Relationships On both limbs of the Stephens Creek Fold, the Stephens Creek Granite Gneiss is stratigraphically overlain by Broken Hill Group, specifically the Allendale Metasediments or the Parnell Formation. In the core of the fold, there is an area of psammitic to psammopelitic metasediments lacking any diagnostic features for stratigraphic classification. Some of these metasediments contain disseminated magnetite.
Age Ranges from 1689 ± 5 to 1686 ± 4 Ma. SHRIMP U – Pb zircon dating, this paper.
Georges Bore Granite Gneiss (new name)
Derivation of name Georges Bore is about 2 km northeast of the geochronology sample site from this body.
Previous work Mapped and described by Stroud (Citation1978b, Citation1986).
Distribution and dimensions A large, irregular body or bodies extending about 17 km in northeast strike length, and up to 4 km outcrop width. Located between Oakdale and Montana homesteads (both currently unoccupied), and enclosing the hill known as the Sentinel.
Type section The irregular shape of the body makes it difficult to specify a representative type section. The section from GR 512900E 6430750N to GR 515300E 6427850N (Zone 54 AGD 1966) is chosen because it exhibits most of the lithological variation in the gneiss, incorporates areas of intervening metasediments, and passes through the geochronology sample site.
Description Consists of apparently irregular areas of megacryst-rich and megacryst-poor quartz – feldspar – biotite gneiss, and minor garnet-bearing gneiss (Stroud Citation1986).
Relationships Intrusive into Thackaringa Group composite gneiss and albitised metasediments, and into Broken Hill Group metasediments.
Age 1695 ± 4 Ma. SHRIMP U – Pb zircon dating, this paper.
Supplementary Paper
APPENDIX 2: SHRIMP ANALYTICAL DATA
1 SHRIMP U – Pb zircon data for a basic granulite from the Cues Formation: sample 200218.5804.
2 SHRIMP U – Pb zircon data for a basic granulite from the Cues Formation: sample 200218.5815.
3 SHRIMP U – Pb zircon data for a leucogneiss of the Cues Formation: sample 200218.5802.
4 SHRIMP U – Pb zircon data for a felsic Potosi-type gneiss lithology in the Cues Formation, Thackaringa Group: sample 200218.5803.
5 SHRIMP U – Pb zircon data for a felsic Potosi-type gneiss lithology in the Cues Formation, Thackaringa Group: sample 200218.5812.
6 SHRIMP U – Pb zircon data for a layered quartzo-feldspathic gneiss in the Redan Gneiss: sample 200218.5813.
7 SHRIMP U – Pb zircon data for a poorly layered quartzo-feldspathic gneiss in the Redan Gneiss: sample 200218.5814.
8 SHRIMP U – Pb zircon data for a banded quartzo-feldspathic gneiss in the Farmcote Gneiss, Rantyga Group: sample 9818.5036B (analyses 201 – 247); and Pb – Pb monazite data for sample 9818.5036A (analyses 102 – 104).
9 SHRIMP U – Pb zircon data for felsic material from layered albitic gneiss in the Farmcote Gneiss, Rantyga Group: sample 9818.5036C.
10 SHRIMP U – Pb zircon data for a massive dark grey gneiss in the Farmcote Gneiss, Rantyga Group: sample 9818.5036.
11 SHRIMP U – Pb zircon data for Stephens Creek Granite Gneiss, Silver City Suite: sample 200218.5817.
12 SHRIMP U – Pb zircon data for Stephens Creek Granite Gneiss, Silver City Suite: sample 200218.5816.
13 SHRIMP U – Pb zircon data for Wondervale Well Granite Gneiss, Silver City Suite: sample 200218.5801.
14 SHRIMP U – Pb zircon data for Georges Bore Granite Gneiss, Silver City Suite: sample 200218.5811.
15 SHRIMP U – Pb zircon data for a high-grade Potosi-type felsic gneiss in the East Mingary region: sample 200218.5874.
16 SHRIMP Pb – Pb monazite data for a high-grade Potosi-type felsic gneiss in the East Mingary region: sample 200218.5874.
17SHRIMP U – Pb zircon data for a quartzo-feldspathic gneiss (Potosi-type gneiss) from the Parnell Formation, Broken Hill Group: sample 200218.2005 [GR 545838 6502485 (1966 geoid) Brewery Well 1:25 000 sheet, Zone 54].
1. *In all tables denotes the percentage of common 206Pb in the total measured 206Pb.
2. Grid references for all samples except sample 200218.2005 (table 17) are given in – .