Abstract
The Mesoproterozoic Roper Group of the McArthur Basin has excellent petroleum potential, but exploration has been hampered by poor constraints on its post-depositional history that has compromised understanding of the tectonostratigraphic evolution of the basin. The Derim Derim Dolerite occupies an important position in the event chronology of the McArthur Basin, having intruded the Roper Group prior to post-Roper basin inversion, and it is also a major component of Mesoproterozoic intraplate mafic magmatism in northern Australia. Since 1997, the Derim Derim Dolerite has been assigned a magmatic crystallisation age of 1324 ± 4 Ma (all uncertainties are 95% confidence), based on unpublished sensitive high-resolution ion micro probe (SHRIMP) U–Pb analyses on baddeleyite attributed to a dolerite sample from Bureau of Mineral Resources drill-hole Urapunga 5. Herein, we establish that the SHRIMP sample originated from the type locality of the Derim Derim Dolerite in outcrop 90 km northwest of Urapunga 5 and document the 207Pb/206Pb date interpreted from the 1997 dataset. New U–Pb SHRIMP reanalysis of the same grain-mounts yielded a mean 207Pb/206Pb date of 1320.1 ± 5.3 Ma, confirming the 1997 result, and isotope dilution-thermal ionisation mass spectrometry (ID-TIMS) analysis of baddeleyites plucked from the mounts yielded a precise mean 207Pb/206Pb date of 1327.5 ± 0.6 Ma. This date is significantly older than a baddeleyite U–Pb ID-TIMS date of ca 1313 Ma recently reported elsewhere from dolerite in the Beetaloo Sub-basin 200 km to the south, indicating that magmatism attributed to the Derim Derim Dolerite spanned at least 10–15 Ma. Previously documented geochemical variation in Mesoproterozoic intraplate mafic rocks across the Northern Territory (such as the 1325 ± 36 Ma Galiwinku Dolerite in the McArthur Basin, 1316 ± 40 Ma phonolites in the Nimbuwah Domain of the eastern Pine Creek Orogen, and 1295 ± 14 Ma gabbro in the Tomkinson Province) may reflect episodic pulses of magmatism hitherto obscured by the low precision of the available isotopic dates. The timing and geochemistry of Derim Derim-Galiwinku mafic igneous activity is strikingly similar to that of the Yanliao Large Igneous Province (LIP) in the northern North China Craton, and the global paucity of 1330–1300 Ma LIPs suggests that the North Australian Craton and the North China Craton were in relatively close proximity at that time.
We document a previously unpublished U–Pb baddeleyite date of 1324 ± 4 Ma for the Derim Derim Dolerite in the McArthur Basin, obtained via sensitive high-resolution ion micro probe (SHRIMP), and substantiate it with an isotope dilution-thermal ionisation mass spectrometry (ID-TIMS) date of 1327.5 ± 0.6 Ma from the same sample.
Our dates are significantly older than a U–Pb baddeleyite ID-TIMS date of ca 1313 Ma from dolerite in the Beetaloo Sub-basin 200 km to the south, indicating that magmatism attributed to the Derim Derim Dolerite spanned at least 10–15 Ma.
Contemporaneous intraplate mafic magmatism extended hundreds of kilometres across the Northern Territory, spanning the Tomkinson Province, the McArthur Basin, and the Nimbuwah Domain of the eastern Pine Creek Orogen.
The timing and geochemistry of Derim Derim Dolerite magmatism strongly resembles the Yanliao Large Igneous Province (LIP) in the northern North China Craton, which suggests that the North Australian Craton and the North China Craton were in close proximity during the Mesoproterozoic.
KEY POINTS
Introduction
The Mesoproterozoic hydrocarbon-bearing Roper Group within the McArthur Basin of northern Australia () has excellent potential for both unconventional and conventional petroleum (e.g. Abbott et al., Citation2001; Ahmad, et al., Citation2013; Cox et al., Citation2016; Munson, Citation2014, Citation2016; Munson & Revie, Citation2018; Sweet et al., Citation1999; Yang et al., Citation2018). Effective exploration for hydrocarbon resources requires a sound understanding of the tectonostratigraphic evolution of the basin; however, the post-depositional history of the Roper Group is relatively poorly constrained. In this context, the timing of Derim Derim Dolerite emplacement into the McArthur Basin is significant. First, this geographically widespread network of dolerite sills and dykes establishes an important minimum age for deposition of the host Roper Group. Second, bedding-parallel sills of Derim Derim Dolerite are deformed within the host sedimentary successions (Abbott et al., Citation2001; Ahmad et al., Citation2013; Munson, Citation2016), so its magmatic crystallisation age also constitutes a maximum for deformation associated with the ‘Post-Roper Inversion’ event (Rawlings et al., Citation1997).
Figure 1. Geological elements of the northeastern Northern Territory (modified after Munson, Citation2016), showing the distribution of Derim Derim Dolerite exposure, and locations of boreholes (red circles) with intersected dolerite thickness in metres (in square brackets). BMR, Bureau of Mineral Resources; POG, Pacific Oil and Gas Ltd, operators of drill-holes Urapunga 5 and Altree 2, respectively. Bold labels denote sites that have (or have traditionally been attributed) U–Pb datasets from dolerite-hosted baddeleyite. Yellow stars denote Derim Derim Dolerite samples collected in the 1990s by NTGS and AGSO for U–Pb geochronology, including the type locality (Abbott et al., Citation2001). One site is labelled with its GA SampleID; the other four sites (and their SampleIDs) are shown in .
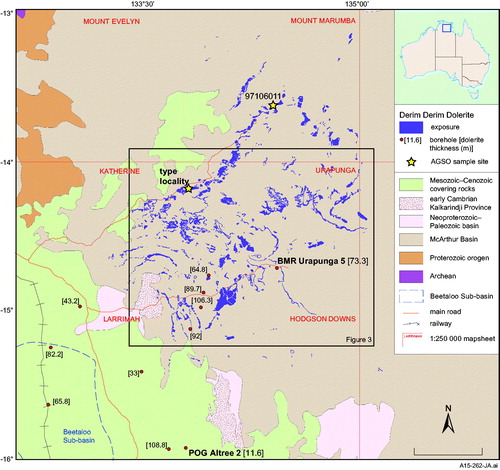
In the mid-1990s, the Australian Geological Survey Organisation (AGSO, now Geoscience Australia [GA]) extracted baddeleyite from a sample of the Derim Derim Dolerite, and U–Pb analyses undertaken via sensitive high-resolution ion micro probe (SHRIMP) in 1997 yielded a weighted mean 207Pb/206Pb date of 1324 ± 4 Ma. Abbott et al. (Citation2001, p. 78–79) attributed the result to ‘J. Claoué-Long, Geoscience Australia, personal communication, 1997’, as part of the stratigraphic definition of the Derim Derim Dolerite. However, this result is clouded in two respects.
First, from the outset, there has been confusion regarding the sampling location of the baddeleyite-bearing dolerite, with the contradictory data inadvertently captured by Abbott et al. (Citation2001). The Derim Derim Dolerite unit description (Abbott et al., Citation2001, p. 47) attributed the SHRIMP date to an outcrop sample from the type locality; however, both the summary of isotopic data (Abbott et al., Citation2001, table 1; p. 6) and the stratigraphic definition of the Derim Derim Dolerite (Abbott et al., Citation2001, p. 78–79) attributed the date to a sill intersected by Bureau of Mineral Resources drill-hole BMR Urapunga 5, 90 km to the southeast of the type locality. Second and more recently, the historic 207Pb/206Pb date of 1324 ± 4 Ma has been challenged by a new isotope dilution-thermal ionisation mass spectrometry (ID-TIMS) baddeleyite U–Pb date of 1312.9 ± 0.7 Ma (Collins et al., Citation2018; Yang et al., Citationin press) from dolerite intersected by Pacific Oil and Gas Ltd drill-hole POG Altree 2, within the Beetaloo Sub-basin of the southern McArthur Basin (). The difference between the two dates is statistically significant, but careful appraisal of the isotopic data and interpretations is required before geological meaning can be attached to the spread of reported ages.
Table 1. Identifiers, locations and metadata for Derim Derim Dolerite samples targeted by NTGS and AGSO for U–Pb geochronology in the 1990s.
In this contribution, first we describe the range of dolerite samples targeted by AGSO and the Northern Territory Geological Survey (NTGS) for U–Pb geochronology in the 1990s and identify the specific sample that yielded baddeleyite for SHRIMP analysis. Second, we document the U–Pb SHRIMP data collected in 1997 and trace the provenance of the interpreted mean 207Pb/206Pb date of 1324 ± 4 Ma. Third, we present new U–Pb SHRIMP data collected from the same grain-mounts (and, in many cases, the same baddeleyite crystals) aimed at confirming the 1997 result, as well as new U–Pb ID-TIMS data on baddeleyite crystals plucked directly from the grain-mounts, collected in order to corroborate the SHRIMP dates and improve the precision of the result. Fourth, we briefly discuss the geological implications of the new results with respect to (1) the Derim Derim Dolerite date from the Beetaloo Sub-basin of the southern McArthur Basin (Collins et al., Citation2018; Yang et al., Citationin press), and (2) other mafic rocks of Mesoproterozoic age and intraplate geochemical affinity across the Northern Territory (e.g. Carson et al., Citation1999; Goldberg, Citation2010; Hollis & Glass, Citation2012; Whelan et al., Citation2016). Fifth, we compare the Derim Derim Dolerite and associated rocks to Mesoproterozoic intraplate mafic magmatism in the Yanliao rift zone of the northern North China Craton (e.g. Zhang et al., Citation2009, Citation2017) as part of the so-called McArthur-Yanliao Gulf (Collins et al., Citation2019), and contrast both with broadly contemporaneous mafic magmatism in the Fraser Complex of the Albany-Fraser Orogen in southwestern Australia (e.g. Glasson et al., Citation2019; Morrissey et al., Citation2017; Smithies et al., Citation2013).
Geology and sampling history of the Derim Derim Dolerite
Geological framework
The Derim Derim Dolerite comprises medium- to coarse-grained dolerite sills and dykes that intruded the Mesoproterozoic Roper Group () at most stratigraphic levels between the Mainoru Formation of the Collara Subgroup and the Bukalorkmi Sandstone of the Maiwok Subgroup (Abbott et al., Citation2001; Munson, Citation2016; see also ), before being deformed with the enclosing stratigraphy during the ‘Post-Roper Inversion’ event (Abbott et al., Citation2001; Ahmad et al., Citation2013; Rawlings et al., Citation1997). The unit therefore occupies an important position in the relative chronology of Mesoproterozoic sedimentation, magmatism and deformation in the McArthur Basin.
Figure 2. Simplified stratigraphic column for the Roper Group, modified after Cox et al. (Citation2016) and Yang et al. (Citation2018) following Munson (Citation2016) and Munson and Revie (Citation2018). Age data sources: 1, Kendall et al. (Citation2009); 2, Collins et al. (Citation2018) and Yang et al. (Citationin press); 3, this study; and 4, Jackson et al. (Citation1999). Derim Derim Dolerite representation is largely schematic but draws on observations by Abbott et al. (Citation2001) and Munson (Citation2016). Labelled sills within Corcoran Formation, Jalboi Formation and Crawford Formation represent those sampled for U–Pb baddeleyite dating by Collins et al. (Citation2018) and Yang et al. (Citationin press), GA SampleID 95640001 (this study), and GA SampleID 97106010 (this study), respectively.
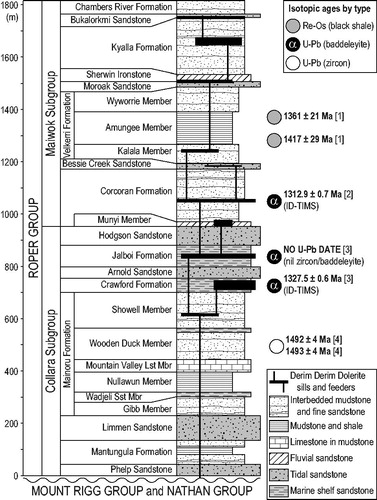
The age of the host Roper Group sedimentary rocks is imperfectly constrained. Most of the Roper Group postdates felsic volcanic rocks of the ca 1492 Ma Mainoru Formation (Jackson et al., Citation1999; see also ), but depositional ages for the sedimentary rocks of the upper Maiwok Subgroup are constrained chiefly by an Rb–Sr date of 1429 ± 31 Ma for carbonate-hosted illite of interpreted diagenetic origin (Kralik, Citation1982), and Re–Os dates of 1361 ± 21 Ma and 1417 ± 29 Ma () for black shales of the Velkerri Formation (Kendall et al., Citation2009). More recently, Yang et al. (Citation2018) dated detrital zircons as young as 1308 ± 41 Ma (n = 1) from the Velkerri Formation, and two samples from the Kyalla Formation yielded dates of 1313 ± 47 Ma (n = 1) and 1317 ± 36 Ma (n = 2), respectively; however, it is unclear whether these dates represent maximum ages for deposition of the host sedimentary rocks, owing to the sparsity of post-1400 Ma dates in the measured spectra. It is possible that some or all of these isolated grains were affected by post-crystallisation isotopic disturbance associated with emplacement of the Derim Derim Dolerite.
Samples targeted for geochronology
McDougall et al. (Citation1965) made the first attempt to date the Derim Derim Dolerite. Pyroxene and plagioclase from ‘somewhat altered’ dolerites intruding the Roper Group yielded a series of K–Ar dates spanning the range 1280–1150 Ma; McDougall et al. (Citation1965) attributed this dispersion to varying degrees of post-crystallisation loss of radiogenic argon, and interpreted a minimum age of ca 1280 Ma for the unit. During the 1990s, NTGS and AGSO undertook second-edition 1:250 000 mapping in the Mount Marumba, Urapunga and Roper River map sheet areas as part of the National Geoscience Mapping Accord (e.g. Abbott et al., Citation2001; Sweet et al., Citation1999). This included new sampling of the Derim Derim Dolerite aimed at improving the accuracy and precision of its magmatic crystallisation age, via U–Pb dating of zircon or baddeleyite.
The first sample of Derim Derim Dolerite targeted by AGSO for U–Pb dating was diamond drillcore from BMR Urapunga 5 (GA SampleID 95640001; , ), which intersected 73.3 m of coarse-grained dolerite spanning the interval 198.1–271.4 m (Sweet & Jackson, Citation1986). In this drill hole, Derim Derim Dolerite intrudes the Jalboi Formation of the Collara Subgroup, which comprises the lower part of the Roper Group (). Sample 95640001, which has hitherto been widely regarded as the source of the SHRIMP 207Pb/206Pb date of 1324 ± 4 Ma obtained in 1997 (e.g. ; Abbott et al., Citation2001, appendix 1), was crushed in the conventional manner for mineral separation targeting zircon but yielded no zircon or baddeleyite. In fact, NTGS and AGSO geologists collected a further four outcrop samples of Derim Derim Dolerite for U–Pb geochronology (SampleIDs 97106009–97106012 inclusive; ; ), one of which yielded the dated baddeleyite population. Sample 97106010 was collected from the type locality (Abbott et al., Citation2001, p. 78–79), 90 km northwest of BMR Urapunga 5 (), where the lower contact of the Derim Derim Dolerite intrudes the Crawford Formation of the Collara Subgroup.
Figure 3. Geological map of the Urapunga region (modified after Munson, Citation2016) showing the distribution of Roper Group exposure at Subgroup-level, and its relationship to the Derim Derim Dolerite. The varying width (across-strike) of each unit primarily reflects dip angle and demonstrate that most Derim Derim Dolerite exposures are sills concordant with (and co-deformed within) the host sedimentary succession (Munson, Citation2016). Yellow stars denote Derim Derim Dolerite samples (labelled with GA SampleID) collected in the 1990s by NTGS and AGSO for U–Pb geochronology.
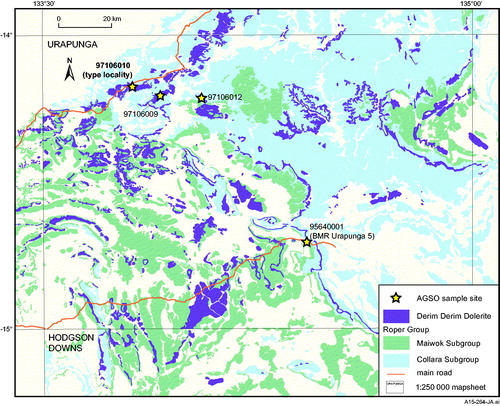
More recently, Collins et al. (Citation2018) and Yang et al. (Citationin press) presented an unpublished ID-TIMS U–Pb baddeleyite age of 1312.9 ± 0.7 Ma (2σ) obtained from dolerite intruding the Corcoran Formation of the lowermost Maiwok Subgroup (which in turn comprises the upper part of the Roper Group), in the Beetaloo Sub-basin, some 200 km south of the Derim Derim Dolerite type locality (). In detail, the age quoted by Collins et al. (Citation2018) is a weighted mean 206Pb/238U date defined by five out of six baddeleyite analyses, and it is supported by a statistically coherent weighted mean 207Pb/206Pb date of 1313.8 ± 1.0 Ma (2σ) defined by all six analyses (Yang et al., Citationin press). The concordance of the 206Pb/238U and 207Pb/206Pb results permits confidence in interpreting the analyses as a cogenetic, isotopically undisturbed baddeleyite population formed during igneous crystallisation of the dolerite.
The apparent difference between the historic ca 1324 Ma SHRIMP date from sample 97106010 and the new ca 1313 Ma ID-TIMS result raises the possibility that dolerites currently attributed to the Derim Derim Dolerite were emplaced over several million years (i.e. 5–15 Ma, based on the 95% confidence intervals of the U–Pb dates), despite the absence of identifiable cross-cutting relationships within the sills and dykes, and their relatively uniform chemistry (Abbott et al., Citation2001; Whelan et al., Citation2016). However, it is necessary to substantiate the original SHRIMP date (via reassessment of the original interpretation, and acquisition of new SHRIMP data on the same grains), and to test the correspondence of SHRIMP and ID-TIMS analytical data, before attaching geological significance to the apparent spread of U–Pb dates.
SHRIMP U–Pb geochronology
Sample preparation and history of analyses
About 400 baddeleyite crystals were separated from sample 97106010, by crushing the sample coarsely and digesting the rock chips in hydrofluoric acid, as described in the Appendix (available, along with Appendix Figures and Appendix Tables, at http://pid.geoscience.gov.au/dataset/ga/127403). About half of the separated grains were set in epoxy mount Z2779, together with several large chips of the reference baddeleyite Phalaborwa (ID-TIMS 207Pb/206Pb date = 2059.60 ± 0.35 Ma; Heaman, Citation2009) and the reference zircon SL13 (ID-TIMS 206Pb/238U date = 572.2 ± 0.4 Ma; Claoué-Long et al., Citation1995). U–Pb isotopic data were acquired using the SHRIMP II instrument in the Research School of Earth Sciences at the Australian National University [ANU], Canberra on 13 July 1997 (, ‘Session 1’), and comprised nine analyses of Phalaborwa and 31 analyses on 29 baddeleyites from 97106010.
Table 2. Summary of SHRIMP session-specific metadata, and parameters measured on reference materials.
The mount was repolished in order to remove the July 1997 probe-pits and facilitate a second analytical session; unfortunately, most of the baddeleyite crystals on Z2779 were mounted with a strong cleavage parallel to the polished surface, and the repolishing process resulted in many grains being plucked from the mount, or otherwise damaged (Appendix Figure A1). As a result, a new grain-mount (Z2895) was prepared using the remaining baddeleyite concentrate from sample 97106010, and the same reference materials as Z2779. Mount Z2895 was also analysed on the ANU SHRIMP II, in a session that commenced on 17 August 1997, and comprised 12 analyses of Phalaborwa and 56 analyses on 55 baddeleyites from 97106010 (, ‘Session 2’). Neither of the 1997 sessions acquired data from the SL13 zircon.
In order to evaluate the repeatability of the 1997 SHRIMP data, both mounts were recovered from the GA archive in March 2019 and prepared for reanalysis. We used potassium iodide solution to remove the legacy layer of gold on the polished surfaces and rephotographed each mount in transmitted and reflected light. Note that the August 1997 probe pits are preserved on the surface of Z2895 (Appendix Figure A2), in contrast to Z2779. The risk of further grain-plucking precluded further repolishing of either mount prior to reanalysis, however desirable it would have been in removing surface Pb accumulated during 20 years in storage, and (on Z2895) legacy probe-pits.
The two mounts were reanalysed during consecutive days (25–27 March 2019) using the SHRIMP II housed at GA, and both sessions incorporated suites of SL13 reference zircon to evaluate the accuracy of mount-specific count-rates at the 204Pb and 204.1 (background) mass-stations. Session 3 () on mount Z2895 comprised 15 analyses of Phalaborwa, 20 analyses of SL13, and 36 analyses of 36 baddeleyites from 97106010. Session 4 () on mount Z2779 comprised 17 analyses of Phalaborwa, 15 analyses of SL13, and 39 analyses of 34 baddeleyites from 97106010.
Data reduction and interpretation
Individual analyses of 97106010 baddeleyites are labelled ‘mount.grain.spot’, with grain-numbering coordinated for consistency across the 1997 and 2019 sessions. Further details of the analytical sessions, calibration protocols, and data-processing procedures and parameters are provided in the Appendix, along with the underlying arrays of analytical data for the reference baddeleyite Phalaborwa (1997 and 2019; Appendix Table A1), the reference zircon SL13 (2019 only; Appendix Table A2), and Derim Derim Dolerite sample 97106010 (1997 and 2019; Appendix Table A3). All statistical data are calculated directly on isotopic ratios, with the end-results converted to dates afterwards, to aid visualisation. The 95% confidence intervals on quoted means are defined as t – sigma (where t is Student’s t) for populations whose mean square of weighted deviates (MSWD; McIntyre et al., Citation1966) is less than or equal to unity, and t – sigma × sqrt (MSWD) when MSWD > 1. In general, populations of isotopic measurements are assumed to be equivalent within their analytical uncertainties when the probability of equivalence (P) is greater than 0.05.
Baddeleyite is a valuable U-bearing phase for dating silica-undersaturated magmatic rocks because: (1) unlike zircon, its physical fragility largely precludes its occurrence as an inherited phase, and (2) baddeleyite is relatively resistant to post-crystallisation loss of radiogenic Pb, and thus commonly preserves an unaltered record of the crystallisation age (Heaman & LeCheminant, Citation1993). However, ion-probe U–Pb dating of baddeleyite presents several difficulties. In addition to the challenges associated with mineral separation, mounting and polishing described above, very slender baddeleyite crystals require the use of a small-diameter primary ion beam, in turn leading to poor counting statistics, especially in crystals poor in U and radiogenic Pb. An additional artefact specific to ion-probe U–Pb dating of baddeleyite is the ‘crystal orientation effect’ (Wingate & Compston, Citation2000), whereby the calibrated 238U/206Pb varies widely from spot to spot, depending on the local orientation of the target crystal lattice, relative to the primary ion beam. This largely precludes meaningful measurement of 238U/206Pb, restricts ion-probe dating to baddeleyites containing enough radiogenic Pb to use orientation-independent 207Pb/206Pb (i.e. typically Mesoproterozoic or older), and compromises evaluation of isotopic concordance, which is a primary test of the reliability of 207Pb/206Pb dates.
SHRIMP U–Pb isotopic data for sample 97106010
Z2779
In July 1997, 31 analyses were collected from 29 baddeleyites (, Appendix Table A3), with two grains (5 and 27) each analysed twice. The crystals yielded predominantly low U contents (19–204 ppm, median 69 ppm), low 232Th/238U (0.02–0.12, median 0.06), and mostly low common 206Pb (median 0.39%). Two analyses are omitted from further consideration: one that featured shorter Pb-isotope counting times than the rest of the dataset, and one with common 206Pb > 4% and a post-1100 Ma 207Pb/206Pb date (Appendix Table A3). Elevated common 206Pb (in a system typified by low values) is a reliable indicator of post-crystallisation disturbance of the U–Pb system in the analysed domain. High common 206Pb commonly reflects ingress of non-radiogenic Pb into the crystal lattice along fractures or cracks, and such conduits can also facilitate leakage of previously ingrown radiogenic Pb. If the leakage event were not geologically recent, it would manifest as an erroneously young 207Pb/206Pb date for the measured domain. The remaining 29 analyses yielded 207Pb/206Pb values that are equivalent within their analytical uncertainties and define a weighted mean date of 1322 ± 9 Ma (MSWD = 1.15, P = 0.27; ).
Figure 4. Tera-Wasserburg concordia diagrams for SHRIMP U–Pb baddeleyite data from sample 97106010, by mount and analytical session. Top row: all data collected from mount Z2779 in (a) 1997 and (b) 2019. Middle row: all data collected from mount Z2895 in (c) 1997 and (d) 2019. Bottom row: pre-1100 Ma 207Pb/206Pb dates for combined mounts from (e) 1997 and (f) 2019. Pale blue fill (Z2779) and pale-yellow fill (Z2895) denote magmatic crystallisation; white fill denotes excluded data. In (e) and (f), excluded data also has grey outlines; heavy blue lines with grey envelopes represent the weighted mean 207Pb/206Pb dates and their 95% confidence intervals for the combined 1997 and 2019 SHRIMP datasets respectively. Heavy red line denotes the ID-TIMS baddeleyite date of 1312.9 ± 0.7 Ma quoted by Collins et al. (Citation2018) and Yang et al. (Citationin press), for comparison.
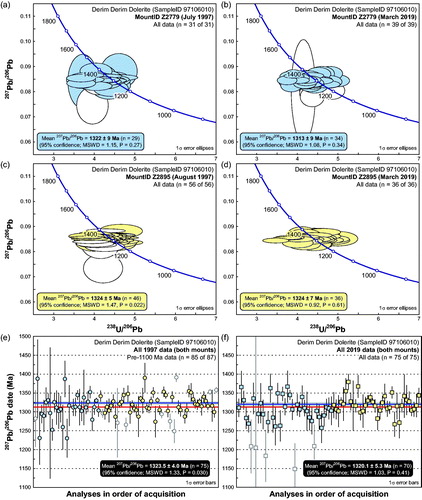
In March 2019, 39 analyses were collected from 34 baddeleyites (, Appendix Table A3), with three grains (3, 26 and 42) each analysed twice, and a fourth (29) analysed three times. Once again, the crystals yielded relatively low U (41–428 ppm, median 139 ppm), 232Th/238U (0.01–0.20, median 0.04), and common 206Pb (median 0.29%). Omitting five analyses characterised by a combination of elevated common 206Pb (>0.70%) and young 207Pb/206Pb dates (post-1210 Ma; Appendix Table A3) that is probably attributable to non-recent U–Pb isotopic disturbance, the remaining 34 analyses define a statistically coherent weighted mean 207Pb/206Pb date of 1313 ± 9 Ma (MSWD = 1.08, P = 0.34; ).
Z2895
In August 1997, 56 analyses were collected from 55 baddeleyites (, Appendix Table A3), with one grain (1) analysed twice. The crystals yielded predominantly low U (22–238 ppm, median 82 ppm), low 232Th/238U (0.02–0.21, median 0.05), and mostly low common 206Pb (median 0.05%). This analytical session was compromised by intermittent electronic instability affecting the SHRIMP II instrument, as recorded by the primary and secondary beam monitor traces. Nine analyses were excluded from further consideration on this basis, along with a tenth which has common 206Pb > 3% and a post-1100 Ma 207Pb/206Pb date (Appendix Table A3), reflecting non-recent U–Pb isotopic disturbance. The remaining 46 analyses define a weighted mean 207Pb/206Pb date of 1324 ± 5 Ma. Although the constituent analyses remain scattered beyond their analytical uncertainties (MSWD = 1.47, P = 0.022; ), a similar degree of excess dispersion is evident in concurrent 207Pb/206Pb analyses of the Phalaborwa reference baddeleyite (MSWD = 3.7, P = 0.000; ). On this basis, we attribute the observed dispersion in measured 207Pb/206Pb to the session-specific electronic instability affecting the instrument, rather than any real geological process.
In March 2019, 36 analyses were collected from 36 baddeleyites (, Appendix Table A3). Once again, the crystals yielded relatively low U (33–391 ppm, median 136 ppm), 232Th/238U (0.02–0.35, median 0.06), and common 206Pb (median 0.09%). This statistically coherent population (Appendix Table A3) defines a weighted mean 207Pb/206Pb date of 1324 ± 7 Ma (MSWD = 0.92, P = 0.61; ).
Combined mounts and sessions
In 1997, a total of 87 analyses were collected from 84 baddeleyites (), 12 of which are excluded from the magmatic population as described above. The two mount-specific weighted mean 207Pb/206Pb dates (1322 ± 9 Ma and 1324 ± 5 Ma) are indistinguishable, and combining the remaining 75 analyses into a single weighted mean yields a 207Pb/206Pb date of 1323.5 ± 4.0 Ma (MSWD = 1.33, P = 0.030). This is the Derim Derim Dolerite age cited by Abbott et al. (Citation2001, p. 78–79) as 1324 ± 4 Ma (J. Claoué-Long, personal communication, 1997)’.
Our March 2019 attempt to replicate the historic SHRIMP dataset employed a relatively small-diameter primary ion beam (18–20 µm, as opposed to the 35–40 µm beam used in 1997; Appendix Figure A2), because improved spatial resolution was needed first to probe scarce remnant crystal fragments in the repolished surface of Z2779, and second to avoid the historic probe-pits preserved in the surface of Z2895. The smaller probe-spot adversely affected ion-counting statistics, and therefore precision at the scale of individual analyses; nevertheless, the 2019 results corroborate those from 1997. The internal agreement of the weighted mean 207Pb/206Pb dates from 1997 (1322 ± 9 Ma on Z2779, 1324 ± 5 Ma on Z2895) is better than those measured in 2019 (1313 ± 9 Ma on Z2779, 1324 ± 7 Ma on Z2895); however the apparent difference between the latter pair is not statistically significant. In 2019, a total of 75 analyses were collected from 70 baddeleyites (), five of which were excluded from the Z2779 magmatic population as described above. Combining the remaining 70 analyses into a single weighted mean yields a statistically coherent 207Pb/206Pb date of 1320.1 ± 5.3 Ma (MSWD = 1.03, P = 0.41), which is indistinguishable from the analogous date of 1323.5 ± 4.0 Ma derived from the combined 1997 datasets. Furthermore all 145 of the analyses attributed to magmatic crystallisation (75 from 1997, 70 from 2019) are equivalent within their analytical uncertainties and yield a statistically coherent weighted mean 207Pb/206Pb date of 1322.3 ± 3.1 Ma (MSWD = 1.19, P = 0.06).
ID-TIMS U–Pb geochronology
Outcrop sample 97106010 at the Derim Derim Dolerite type locality has repeatedly yielded pre-1320 Ma SHRIMP 207Pb/206Pb dates that are distinguishably older than the ID-TIMS 207Pb/206Pb date of 1313.8 ± 1.0 Ma (Yang et al., Citationin press) obtained from dolerite intruding the upper Roper Group in the Beetaloo Sub-basin, 200 km to the south. However, we have not yet assessed the potential for a systematic offset between the measured ages, perhaps as a hitherto-unrecognised artefact of one or both measurement techniques. Furthermore, the existing comparison emphasises the value of high-precision data when seeking to assign geological significance to a discrepancy in measured ages. On that basis, we pursued ID-TIMS dating of baddeleyites from sample 97106010, using crystals plucked directly from our SHRIMP mounts.
Analytical methods
ID-TIMS analyses were conducted in May 2019 at the Isotope Geology Laboratory at Boise State University, USA. Six individual baddeleyite crystals previously analysed via SHRIMP (and labelled in red in Appendix Figures A1 and A2), were manually plucked from mounts Z2779 and Z2895, spiked with the EARTHTIME mixed 202Pb–205Pb–233U–235U tracer solution (ET2535), and each was analysed as a discrete ID-TIMS fraction. Fractions and grain-numbers are cross-referenced in Appendix Table A4, and analytical methods are described in detail in the Appendix.
ID-TIMS U–Pb isotopic data for sample 97106010
Six analyses were collected from six baddeleyites (, Appendix Table A4). Common Pb was very low (0.106–0.144 pg, median 0.109 pg), and 206Pb/204Pb was quite high (5164–12792, median 6478) in view of the low U contents inferred from the SHRIMP analyses. Radiogenic 206Pb as a percentage of total 206Pb spanned the range 99.65–99.86 mol%, with a median of 99.72 mol%.
Figure 5. (a) Conventional (Wetherill) concordia diagram for ID-TIMS U–Pb baddeleyite data from sample 97106010. Heavy black dot-dashed line is the Model 1 discordia regression; grey envelope on concordia curve reflects 235U and 238U decay constant uncertainties of Jaffey et al. (Citation1971), and the upper intercept 95% confidence intervals of 1.1 Ma and 4.0 Ma exclude and include (respectively) those uncertainties. (b) One dimensional 207Pb/206Pb dates in the sequence defined by Appendix Table A4. The 95% confidence interval excludes the decay-constant uncertainties, as the same decay constants are used for all ID-TIMS and SHRIMP datasets being compared.
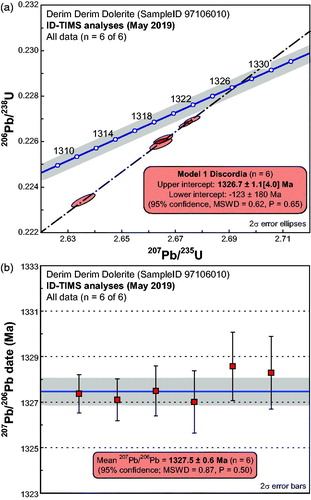
All six analyses are discernibly discordant (0.7–2.4%; , Appendix Table A4), but the population defines a statistically coherent discordia regression with upper and lower concordia intercepts of 1326.7 ± 1.1 Ma and –123 ± 180 Ma, respectively (95% confidence, excluding uncertainties on the 235U and 238U decay constants as measured by Jaffey et al., Citation1971; MSWD = 0.62, P = 0.65). We interpret this array to reflect magmatic crystallisation of a cogenetic baddeleyite suite at ca 1326.7 Ma, with each crystal affected to varying degrees by geologically recent (zero-age) loss of radiogenic Pb. The fact that the lower intercept is indistinguishable from zero means that the magmatic crystallisation age is best estimated using the 207Pb/206Pb measurements (). These too form a statistically coherent population, with their weighted mean (0.085525 ± 0.000026 at 95% confidence, excluding decay constant uncertainties; MSWD = 0.87, P = 0.50) corresponding to a date of 1327.5 ± 0.6 Ma ().
The 207Pb/206Pb ratios and dates in Appendix Table A4 are predicated on present-day 238U/235U = 137.88 (Steiger & Jäger, Citation1977), for direct comparability with the multiple generations of U–Pb SHRIMP data processing. Using the more recently measured terrestrial average 238U/235U (137.818; Hiess et al., Citation2012) would displace the weighted mean 207Pb/206Pb date ca 0.9 Ma younger.
Discussion
Comparing SHRIMP and ID-TIMS results for 97106010
The two technique-specific weighted mean 207Pb/206Pb dates constraining igneous crystallisation of sample 97106010 (1322.3 ± 3.1 Ma defined by 145 of 162 SHRIMP analyses, and 1327.5 ± 0.6 Ma defined by six of six ID-TIMS analyses) are completely distinct at the 95% confidence level. The high-precision ID-TIMS data indicate very minor (0.7–2.4%) loss of radiogenic Pb in geologically recent times, but no other isotopic dispersion is discernible, and the associated weighted mean 207Pb/206Pb date of 1327.5 ± 0.6 Ma is our best estimate of the true magmatic crystallisation age of the Derim Derim Dolerite at its type locality.
The overall tendency of the SHRIMP datasets towards slightly younger 207Pb/206Pb dates could represent analytical artefacts (such as instrumental mass fractionation of Pb isotopes, and/or isobaric interferences resulting in ‘overcounting’ of 204Pb and consequent overcorrection of the measured 207Pb/206Pb for non-radiogenic Pb), or geological dispersion arising principally from varying degrees of ancient leakage of radiogenic Pb. The relative difference between the SHRIMP and ID-TIMS means is less than 0.5%, so analytical artefacts are difficult to rule out; however, instrumental mass fractionation of Pb isotopes is not resolvable at inter-session scale in the sets of concurrent measurements of the Phalaborwa reference baddeleyite (). Three of the four sessions yielded high-precision weighted mean 207Pb/206Pb values (with relative 95% confidence intervals of 0.25–0.30%); the fourth (Z2895 in 1997) yielded a marginally younger value (and a greater degree of internal dispersion), but the concurrently analysed 97106010 baddeleyites yielded the oldest of the four session-specific weighted mean 207Pb/206Pb values, so it is unlikely that the session was affected by instrumental mass fractionation. Isobaric interferences at the 204Pb measurement position are best monitored using a Pb-poor reference material (Black, Citation2005), and the 2019 sessions employed the co-mounted SL13 reference zircon (). The universally low count-rates (of ‘true’ 204Pb and of any putative isobaric interference) means that very low rates of 204Pb-overcounting are difficult to detect, but both mounts yielded 204Pb-corrected 207Pb/206Pb values well within error of the SL13 reference value (albeit with relative 95% confidence intervals of the order of 1.5–2.0%), and the two 204Pb overcount-rates bracket zero (). No analogous measurements were made during the 1997 sessions, but the fact that 204Pb-corrected 207Pb/206Pb values for 97106010 in 1997 tend to be older than those measured in the overcount-free 2019 sessions suggests that the 1997 sessions were similarly unaffected by 204Pb overcounts.
We therefore turn our attention to ancient loss of radiogenic Pb as a potential mechanism for the tendency towards younger 207Pb/206Pb dates in the SHRIMP data relative to ID-TIMS. Although the 145 SHRIMP analyses defining magmatic crystallisation at 1322.3 ± 3.1 Ma are equivalent within their analytical uncertainties (MSWD = 1.19, P = 0.06) across the four analytical sessions, one striking feature of is the prevalence of younger 207Pb/206Pb dates obtained from Z2779 during its 2019 session (). This session was uniquely complicated by the need to analyse an over-polished mount-surface featuring a large number of suboptimal remnant shards of baddeleyite. Some domains within these shards are demonstrably affected by ancient loss of radiogenic Pb, as evidenced by the five analyses (with elevated common 206Pb and post-1210 Ma dates) already culled from the younger end of the measured distribution (), so it is possible that other analyses are affected by minor ‘cryptic’ leakage of radiogenic Pb (i.e. insufficient to disperse the remaining population beyond its [large] experimental uncertainties, but sufficient to bias the weighted mean to a marginally younger value).
This idea can be tested by omitting all 34 of the magmatic analyses collected during the 2019 session on Z2779 and recalculating the combined SHRIMP date based solely on the 111 magmatic analyses collected in the remaining three sessions. The revised calculation yields a statistically coherent weighted mean 207Pb/206Pb date of 1323.5 ± 3.3 Ma (MSWD = 1.19, P = 0.09), which is slightly closer to the ID-TIMS weighted mean 207Pb/206Pb date of 1327.5 ± 0.6 Ma; however, the two 95% confidence intervals still do not overlap. This might indicate that one or more of the remaining three sessions is similarly affected by cryptic ancient loss of radiogenic Pb from some crystal domains.
The history of ancient leakage of radiogenic Pb inferred from the SHRIMP datasets is at odds with the ID-TIMS data (in which recent loss of radiogenic Pb is discernible, but ancient Pb loss is not), but this apparent difference mostly reflects limitations inherent in SIMS U–Pb analysis of baddeleyite, as well as the overall quality of target crystals as a function of the vastly different sizes of the technique-specific analytical populations.
Any assessment of recent loss of radiogenic Pb requires accurate and precise determination of 206Pb/238U for individual analyses so isotopic concordance can be evaluated. As noted above, this is not possible for baddeleyite SHRIMP data, owing to the crystal orientation effect (Wingate & Compston, Citation2000). In our SHRIMP datasets, 2σ uncertainties of individual calibrated 238U/206Pb analyses are of the order of 10–15%, so the 0.7–2.4% discordance indicated by the ID-TIMS data array might well be present in the SHRIMP data as well, but it will not be discernible.
We interpret the apparent prevalence of ancient loss of radiogenic Pb in the SHRIMP datasets as a combination of compromised mount-surfaces (for the 2019 sessions in particular), plus the increased likelihood of analysing non-pristine baddeleyite crystal domains in the course of collecting a total of 162 analyses from a target population numbering only about 400 baddeleyites. In contrast, ID-TIMS dating usually requires only a small number of analyses, so the selection of targets unlikely to be affected by post-crystallisation isotopic disturbance is correspondingly easier.
Mesoproterozoic intraplate magmatism in Northern Australia
All three baddeleyite 207Pb/206Pb datasets from outcrop sample 97106010 (1323.5 ± 4.0 Ma by SHRIMP in 1997, 1320.1 ± 5.3 Ma by SHRIMP in 2019, and 1327.5 ± 0.6 Ma via ID-TIMS) indicate a pre-1320 Ma magmatic crystallisation age for the Derim Derim Dolerite at its type locality in the northwestern McArthur Basin. Our result is distinctly older than the ID-TIMS baddeleyite 207Pb/206Pb date of 1313.8 ± 1.0 Ma (Yang et al., Citationin press) from drill-hole POG Altree 2 (and its corresponding 206Pb/238U date of 1312.9 ± 0.7 Ma; Collins et al., Citation2018) in the Beetaloo Sub-basin 200 km to the south, and indicates a spread of ages for mafic magmatism attributed to the Derim Derim Dolerite.
The age range is regionally significant, as intraplate mafic plutonic rocks of similar geochemistry and broadly similar age extend well beyond the Roper Group, and the McArthur Basin. Whelan et al. (Citation2016) reported a SIMS U–Pb baddeleyite age of 1325 ± 36 Ma (2σ) for the Galiwinku Dolerite (about 170 km northwest of sample 97106010), which is part of a radiating swarm of dykes within the northern margin of the McArthur Basin (Goldberg, Citation2010). These dykes are spatially associated with the peralkaline Maningkorrirr Phonolite and Mudginberri Phonolite in the Nimbuwah Domain of the eastern Pine Creek Orogen (Hollis & Glass, Citation2012; Stuart-Smith & Needham, Citation1984), for which Page et al. (Citation1980) determined an Rb–Sr date of 1316 ± 40 Ma. Similarly, Melville (Citation2010) reported a SHRIMP U–Pb baddeleyite age of 1295 ± 14 Ma from gabbro intruding the Renner Group in the Tomkinson Province southwest of the McArthur Basin, about 220 km south of Altree 2, and over 400 km south of sample 97106010. The samples dated by Melville (Citation2010) and Whelan et al. (Citation2016) are almost 600 km apart.
The relatively low precision of many of these age constraints precludes any detailed assessment of the timing or temporal span of individual pulses of mafic Mesoproterozoic magmatism. Based on the criteria of Bryan and Ernst (Citation2008), the ca 15 Ma separation between the two high-precision ID-TIMS dates from the Derim Derim Dolerite does not preclude the two dated units belonging to the same mafic igneous province. However, the range of emplacement ages offers a partial explanation for some of the regional geochemical variations documented by Whelan et al. (Citation2016), with particular respect to the transition from high- to low-Ti/Y rocks within the Galiwinku Dolerite, Maningkorrirr Phonolite and Mudginberri Phonolite (Whelan et al., Citation2016). Hollis and Glass (Citation2012) interpreted these geochemical variations in terms of different depths of partial melting in the source region, and the new high-precision ID-TIMS dating from the Derim Derim Dolerite raises the possibility that the observed variations in Ti/Y could reflect temporally distinct pulses of magmatism.
Correlation with the Yanliao Large Igneous Province in the North China Craton
Establishing a minimum duration of 10–15 Ma for emplacement of the Derim Derim-Galiwinku mafic igneous province strengthens already striking similarities between the nature and timing of intraplate mafic magmatism in the Northern Territory and that preserved within the predominantly Mesoproterozoic Yanliao rift zone in the northern North China Craton (e.g. Zhang et al., Citation2017). A series of baddeleyite 207Pb/206Pb dates indicate that emplacement of the dolerite sills defining the Yanliao Large Igneous Province commenced at ca 1330 Ma and terminated at ca 1305 Ma, with an apparent peak in magmatic activity at ca 1323 Ma (Wang et al., Citation2014; Zhang et al., Citation2009, Citation2017). Zhang et al. (Citation2017) also emphasised the strong geochemical similarities between the tholeiitic to subalkaline basaltic compositions common to the Yanliao and Derim Derim-Galiwinku igneous provinces, although the Yanliao rocks also encompass more andesitic compositions that are not yet known from Derim Derim-Galiwinku (Whelan et al., Citation2016 and references therein).
Globally, intraplate mafic magmatism in the 1330–1300 Ma range appears to be restricted to the Yanliao and Derim Derim-Galiwinku LIPs (Ernst, Citation2014), which constitutes circumstantial evidence that the North Australian Craton and the North China Craton were at least near neighbours (if not connected) during the Mesoproterozoic (e.g. Collins et al., Citation2019; Zhang et al., Citation2017). Testing of this hypothesis awaits the establishment of mid-Mesoproterozoic paleomagnetic poles for the North Australian Craton, for comparison with existing data from the North China Craton (Xu et al., Citation2014).
Contrast with contemporaneous mafic magmatism in southwestern Australia
The new ID-TIMS dates for the Derim Derim Dolerite establish at least partial contemporaneity between the Derim Derim-Galiwinku igneous province and voluminous mafic plutonism of the Fraser Complex within the Albany-Fraser Orogen of southwestern Australia. Fletcher et al. (Citation1991) determined a whole-rock Sm–Nd age of 1291 ± 21 Ma on Fraser Complex gabbro, and Claoué-Long and Hoatson (Citation2009) grouped the Fraser Complex and Derim Derim Dolerite into the ca 1310 Ma Fraser Event (Mafic Event ME 44 of Thorne et al., Citation2014). Dating magmatic crystallisation of Fraser Complex mafic rocks is complicated by overprinting 1300–1290 Ma high-grade metamorphism (e.g. C. Clark et al., Citation2014; D. J. Clark et al., Citation1999; De Waele & Pisarevsky, Citation2008); however, Glasson et al. (Citation2019) obtained a U–Pb date of 1315 ± 5 Ma from a texturally distinctive zircon–ilmenite association ascribed to igneous crystallisation.
The tectonic setting of Fraser Complex mafic magmatism remains debated (Glasson et al., Citation2019), with interpretations of emplacement into an intracratonic rift (e.g. Smithies et al., Citation2013; Spaggiari et al., Citation2011) competing with hypotheses invoking a back-arc setting (e.g. Clark et al., Citation2014; Kirkland et al., Citation2011; Morrissey et al., Citation2017). The latter emphasises the likelihood of an arc-related origin for temporally related (1330–1280 Ma) calc-alkaline felsic plutonism of the Wankanki Supersuite in the Musgrave Province (e.g. Howard et al., Citation2011; Smithies et al., Citation2010), and, to a lesser extent, the Recherche Supersuite in the southern Albany-Fraser Orogen (e.g. Kirkland et al., Citation2015; Smithies et al., Citation2015), including ca 1300 Ma granites within the Fraser Complex (e.g. Clark et al., Citation1999).
Despite the absence of definitive geochemical and isotopic evidence (Glasson et al., Citation2019; Smithies et al., Citation2013), the tectonic setting of the Fraser Complex appears distinct from that of the Derim Derim Dolerite and its likely correlatives (Melville, Citation2010; Whelan et al., Citation2016). Unlike the Fraser Complex, the Derim Derim-Galiwinku province is notable for its lack of (preserved) coeval felsic magmatic rocks; furthermore, it appears unaffected by post-magmatic regional metamorphism (as evidenced by the preservation of syn-magmatic Rb–Sr dates within Jalboi Formation shales hosting the Derim Derim Dolerite in drill-hole BMR Urapunga 5; Collins et al., Citation2019). Both of these features are consistent with an intraplate origin for the Derim Derim-Galiwinku province. It is therefore likely that Australia-wide mafic magmatism attributed to the ca 1310 Ma Fraser Event (Claoué-Long & Hoatson, Citation2009; Mafic Event ME 44 of Thorne et al., Citation2014) spans a range of tectonic settings in the crust.
Conclusions
For two decades, the magmatic crystallisation age of the regionally significant Derim Derim Dolerite in the McArthur Basin has been constrained by a widely cited (but unpublished) U–Pb SHRIMP baddeleyite date of 1324 ± 4 Ma (J. Claoué-Long, personal communication, 1997 in Abbott et al., Citation2001). Our review of the historic data established that the baddeleyite-bearing sample was collected in outcrop at the type locality of the Derim Derim Dolerite (rather than from drillcore from BMR Urapunga 5, located 90 km to the southeast), and confirmed the weighted mean 207Pb/206Pb date of 1324 ± 4 Ma derived from the U–Pb SHRIMP dataset collected in 1997. Our 2019 SHRIMP reanalysis of the same grain-mounts reproduced this result, yielding a weighted mean 207Pb/206Pb date of 1320.1 ± 5.3 Ma. ID-TIMS U–Pb analyses of baddeleyite crystals plucked from the SHRIMP mounts yielded a high-precision weighted mean 207Pb/206Pb date of 1327.5 ± 0.6 Ma, which is now the best estimate of the magmatic crystallisation age of the Derim Derim Dolerite at its type locality.
This date is significantly older than a baddeleyite U–Pb ID-TIMS date of ca 1313 Ma recently reported from dolerite in the Beetaloo Sub-basin (Collins et al., Citation2018; Yang et al., Citationin press), some 200 km south of the Derim Derim Dolerite type locality, which indicates that magmatism attributed to the Derim Derim Dolerite spanned at least 10–15 Ma. The possibility of episodic emplacement of phonolites and intraplate mafic rocks as far afield as the Nimbuwah Domain of the eastern Pine Creek Orogen (Hollis & Glass, Citation2012; Whelan et al., Citation2016), and the Tomkinson Province (Melville, Citation2010), has hitherto been obscured by the relatively low precision of the available isotopic dates. Hollis and Glass (Citation2012) documented a range of Ti/Y values in mafic rocks of the Nimbuwah Domain, which they interpreted to reflect different depths of partial melting, and it is possible that such variations can be attributed to temporally distinct pulses of magmatism. Zhang et al. (Citation2017) documented a strikingly similar range of baddeleyite 207Pb/206Pb dates (ca 1330 Ma to ca 1305 Ma, with peak activity at ca 1323 Ma) from the Yanliao rift zone of the North China Craton and demonstrated strong geochemical similarities between the Yanliao and Derim Derim-Galiwinku mafic rocks. Even in the absence of supporting paleomagnetic data, these relationships suggest that the North Australian Craton and North China Craton were near-contiguous in the Mesoproterozoic (Zhang et al., Citation2017), and defined the epicontinental ‘McArthur-Yanliao Gulf’ within the supercontinent Nuna/Columbia (Collins et al., Citation2019).
The Derim Derim-Galiwinku and Yanliao igneous provinces are also contemporaneous with voluminous ca 1315–1300 Ma gabbroic rocks of the Fraser Complex in the Albany-Fraser Orogen of southwestern Australia; however, the Fraser Complex is associated with calc-alkaline granitic rocks and was overprinted by regional granulite facies metamorphism. This indicates that coeval mafic magmatic events at continent-scale need not be tectonically linked.
Acknowledgements
The finalisation of this work owes much to many people, over a long period of time. In the mid- to late 1990s, this research was driven by a team of NTGS and AGSO geologists collaborating on second-edition 1:250 000 mapping of the Roper region (under the auspices of the National Geoscience Mapping Accord). Michael Wingate (ex-ANU) advised us on liberating baddeleyite from mafic rocks and supplied Phalaborwa reference material. Chris Foudoulis and Tony Watson (both ex-AGSO/GA) skilfully conducted mineral separations and prepared the SHRIMP mounts for analysis. More recently, Mart Idnurm (ex-AGSO) helped us unravel the extent of Derim Derim Dolerite sampling for U–Pb geochronology, Tim Munson (NTGS) supplied the digital files underpinning and , and Bo Yang (University of Adelaide) shared unpublished details of baddeleyite ID-TIMS U–Pb analyses from drill-hole POG Altree 2. This manuscript was improved by constructive comments and suggestions from Chris Carson and Natalie Kositcin (both GA), and journal reviewers Steven Denyszyn and Jo Whelan. This paper is published with the permission of the Chief Executive Officer, Geoscience Australia. Geoscience Australia eCat ID 127403.
Disclosure statement
No potential conflict of interest was reported by the author(s).
Data availability
The data (Appendix, Appendix Tables, and Appendix Figures) that support the findings of this study are openly available in the Geoscience Australia data repository at http://pid.geoscience.gov.au/dataset/ga/127403.
References
- Abbott, S. T., Sweet, I. P., Plumb, K. A., Young, D. N., Cutovinos, A., Ferenczi, P. A., Brakel, A. T., & Pietsch, B. A. (2001). Roper region: Urapunga and Roper River special, Northern Territory (2nd ed., Sheets SD53-10, SD53-11. 1:250 000 geological map series explanatory notes). Northern Territory Geological Survey. https://geoscience.nt.gov.au/gemis/ntgsjspui/handle/1/81859
- Ahmad, M., Dunster, J. N., & Munson, T. J. (2013). Chapter 15: McArthur Basin. In M. Ahmad & T. J. Munson (Eds.), Geology and mineral resources of the Northern Territory. Special Publication 5. Northern Territory Geological Survey. Retrieved from https://geoscience.nt.gov.au/gemis/ntgsjspui/handle/1/81495
- Black, L. P. (2005). The use of multiple reference samples for the monitoring of ion microprobe performance during zircon 207Pb/206Pb age determinations. Geostandards and Geoanalytical Research, 29(2), 169–182. https://doi.org/https://doi.org/10.1111/j.1751-908X.2005.tb00890.x
- Bryan, S. E., & Ernst, R. E. (2008). Revised definition of Large Igneous Provinces (LIPs). Earth-Science Reviews, 86(1–4), 175–202. https://doi.org/https://doi.org/10.1016/j.earscirev.2007.08.008
- Carson, L. J., Haines, P. W., Brakel, A. T., Pietsch, B. A., & Ferenczi, P. A. (1999). Milingimbi, Northern Territory (2nd ed., Sheet SD53-2. 1:250 000 geological map series explanatory notes). Northern Territory Geological Survey. https://geoscience.nt.gov.au/gemis/ntgsjspui/handle/1/81863
- Claoué-Long, J. C., Compston, W., Roberts, J., & Fanning, C. M. (1995). Two Carboniferous ages: a comparison of SHRIMP zircon dating with conventional zircon ages and 40Ar/39Ar analysis. In W. A. Berggren, D. V. Kent, M.-P. Aubry, & J. Hardenbol (Eds.), Geochronology, Time Scales and Global Stratigraphic Correlation (pp. 3–21, SEPM Special Publication 54). Society for Sedimentary Geology. https://doi.org/https://doi.org/10.2110/pec.95.04.0003
- Claoué-Long, J. C., & Hoatson, D. M. (2009). Guide to using the map of Australian Proterozoic Large Igneous Provinces (Record 2009/44). Geoscience Australia. http://pid.geoscience.gov.au/dataset/ga/70008
- Clark, C., Kirkland, C. L., Spaggiari, C. V., Oorschot, C., Wingate, M. T. D., & Taylor, R. J. (2014). Proterozoic granulite formation driven by mafic magmatism: An example from the Fraser Range Metamorphics, Western Australia. Precambrian Research, 240, 1–21. https://doi.org/https://doi.org/10.1016/j.precamres.2013.07.024
- Clark, D. J., Kinny, P. D., Post, N. J., & Hensen, B. J. (1999). Relationships between magmatism, metamorphism and deformation in the Fraser Complex, Western Australia: constraints from new SHRIMP U–Pb zircon geochronology. Australian Journal of Earth Sciences, 46(6), 923–932. https://doi.org/https://doi.org/10.1046/j.1440-0952.1999.00753.x
- Collins, A., Farkas, J., Glorie, S., Cox, G., Blades, M. L., Yang, B., Nixon, A., Bullen, M., Foden, J. D., Dosseto, A., Payne, J. L., Denyszyn, S., Edgoose, C. J., Close, D. F., Munson, T. J., Menpes, S., Spagnuolo, S., Gustenhuber, J., Sheridan, M., & Close, D. (2018). Orogens to oil: government-industry-academia collaboration to better understand the greater McArthur Basin. In G. C. MacDonald (Ed.), Annual Geoscience Exploration Seminar (AGES) Proceedings, Alice Springs, Northern Territory, 20–21 March 2018 (pp. 49–51). Northern Territory Geological Survey. https://geoscience.nt.gov.au/gemis/ntgsjspui/handle/1/87072
- Collins, A. S., Farkas, J., Glorie, S., Blades, M. L., Cox, G. M., Foden, J. D., Hall, T., Payne, J. L., Yang, B., Nixon, A., Cassidy, E., Subarkah, D., Shannon, A., Higgie, D., Toledo, G., Dosseto, A., Kirscher, U., Edgoose, C., Close, D. F., & Hokin, G. (2019). Life and times of the Proterozoic McArthur–Yanliao Gulf: Update on the ARC–Industry–NTGS Linkage Project. In G. C. MacDonald (Ed.), Annual Geoscience Exploration Seminar (AGES) Proceedings, Alice Springs, Northern Territory, 19–20 March 2019 (pp. 44–48). Northern Territory Geological Survey. https://geoscience.nt.gov.au/gemis/ntgsjspui/handle/1/88374
- Cox, G. M., Jarrett, A., Edwards, D., Crockford, P. W., Halverson, G. P., Collins, A. S., & Li, Z.-X. (2016). Basin redox and primary productivity within the Mesoproterozoic Roper Seaway. Chemical Geology, 440, 101–114. https://doi.org/https://doi.org/10.1016/j.chemgeo.2016.06.025
- De Waele, B., & Pisarevsky, S. A. (2008). Geochronology, paleomagnetism and magnetic fabric of metamorphic rocks in the northeast Fraser Belt, Western Australia. Australian Journal of Earth Sciences, 55(5), 605–621. https://doi.org/https://doi.org/10.1080/08120090801979112
- Ernst, R. E. (2014). Large Igneous Provinces (pp. 653). Cambridge: Cambridge University Press.
- Fletcher, I. R., Myers, J. S., & Ahmat, A. L. (1991). Isotopic evidence on the age and origin of the Fraser Complex, Western Australia: a sample of mid-Proterozoic lower crust. Chemical Geology: Isotope Geoscience Section, 87(3–4), 197–216. https://doi.org/10.1016/0168-9622(91)90021-N
- Glasson, K. J., Johnson, T. E., Kirkland, C. L., Gardiner, N. J., Clark, C., Blereau, E., Hartnady, M. I. H., Spaggiari, C., & Smithies, H. (2019). A window into an ancient backarc? The magmatic and metamorphic history of the Fraser Zone, Western Australia. Precambrian Research, 323, 55–69. https://doi.org/https://doi.org/10.1016/j.precamres.2019.01.011
- Goldberg, A. S. (2010). Dyke swarms as indicators of major extensional events in the 1.9–1.2 Ga Columbia supercontinent. Journal of Geodynamics, 50(3–4), 176–190. https://doi.org/https://doi.org/10.1016/j.jog.2010.01.017
- Heaman, L. M. (2009). The application of U–Pb geochronology to mafic, ultramafic and alkaline rocks: An evaluation of three mineral standards. Chemical Geology, 261(1–2), 43–52. https://doi.org/10.1016/j.chemgeo.2008.10.021
- Heaman, L. M., & LeCheminant, A. N. (1993). Paragenesis and U–Pb systematics of baddeleyite (ZrO2). Chemical Geology, 110(1–3), 95–126. https://doi.org/10.1016/0009-2541(93)90249-I
- Hiess, J., Condon, D. J., McLean, N., & Noble, S. R. (2012). 238U/235U systematics in terrestrial uranium-bearing minerals. Science, 335(6076), 1610–1614. https://doi.org/https://doi.org/10.1126/science.1215507
- Hollis, J. A., & Glass, L. M. (2012). Howship and Oenpelli, Northern Territory (Sheets 5572, 5573. 1:100 000 geological map series explanatory notes). Northern Territory Geological Survey. https://geoscience.nt.gov.au/gemis/ntgsjspui/handle/1/81887
- Howard, H. M., Smithies, R. H., Evins, P. M., Kirkland, C. L., Werner, M., Wingate, M. T. D., & Pirajno, F. (2011). Explanatory Notes for the west Musgrave Province (1:100 000 geological map series explanatory notes). Geological Survey of Western Australia. http://dmpbookshop.eruditetechnologies.com.au/product/explanatory-notes-for-the-west-musgrave-province.do
- Jackson, M. J., Sweet, I. P., Page, R. W., & Bradshaw, B. E. (1999). The South Nicholson and Roper Groups: Evidence for the early Mesoproterozoic Roper Superbasin. In B. E. Bradshaw, & D. L. Scott (Eds.), Integrated basin analysis of the Isa Superbasin using seismic, well-log and geopotential data: an evaluation of the economic potential of the northern Lawn Hill Platform (pp. 36–59, Record 1999/19). Australian Geological Survey Organisation. http://pid.geoscience.gov.au/dataset/ga/30870
- Jaffey, A. H., Flynn, K. F., Glendenin, L. E., Bentley, W. C., & Essling, A. M. (1971). Precision measurements of half-lives and specific activities of 235U and 238U. Physical Review C, 4(5), 1889–1906. https://doi.org/https://doi.org/10.1103/PhysRevC.4.1889
- Kendall, B., Creaser, R. A., Gordon, G. W., & Anbar, A. D. (2009). Re–Os and Mo isotope systematics of black shales from the Middle Proterozoic Velkerri and Wollogorang Formations, McArthur Basin, northern Australia. Geochimica et Cosmochimica Acta, 73(9), 2534–2558. https://doi.org/https://doi.org/10.1016/j.gca.2009.02.013
- Kirkland, C. L., Spaggiari, C. V., Pawley, M. J., Wingate, M. T. D., Smithies, R. H., Howard, H. M., Howard, Tyler, I. M., Belousova, E. A., & Poujol, M. (2011). On the edge: U–Pb, Lu–Hf, and Sm–Nd data suggests reworking of the Yilgarn craton margin during formation of the Albany-Fraser Orogen. Precambrian Research, 187(3–4), 223–247. https://doi.org/https://doi.org/10.1016/j.precamres.2011.03.002
- Kirkland, C. L., Spaggiari, C. V., Smithies, R. H., Wingate, M. T. D., Belousova, E. A., Gréau, Y., Sweetapple, M. T., Watkins, R., Tessalina, S., & Creaser, R. (2015). The affinity of Archean crust on the Yilgarn—Albany–Fraser Orogen boundary: Implications for gold mineralisation in the Tropicana Zone. Precambrian Research, 266, 260–281. https://doi.org/https://doi.org/10.1016/j.precamres.2015.05.023
- Kralik, M. (1982). Rb–Sr age determinations on Precambrian carbonate rocks of the Carpentarian McArthur Basin, Northern Territory, Australia. Precambrian Research, 18(1–2), 157–170. https://doi.org/10.1016/0301-9268(82)90044-4
- McDougall, I., Dunn, P. R., Compston, W., Webb, A. W., Richards, J. R., & Bofinger, V. M. (1965). Isotopic age determinations on Precambrian rocks of the Carpentaria region, Northern Territory, Australia. Journal of the Geological Society of Australia, 12(1), 67–90. https://doi.org/10.1080/00167616508728586
- McIntyre, G. A., Brooks, C., Compston, W., & Turek, A. (1966). The statistical assessment of Rb–Sr isochrons. Journal of Geophysical Research, 71(22), 5459–5468. https://doi.org/https://doi.org/10.1029/JZ071i022p05459
- Melville, P. M. (2010). Geophysics and drilling collaboration final report for drilling program, Lake Woods Project, EL23687, EL24520, EL25631, EL27317, EL27318 (Open File Report CR2010-0226). Northern Territory Geological Survey. https://geoscience.nt.gov.au/gemis/ntgsjspui/handle/1/75769
- Morrissey, L. J., Payne, J. L., Hand, M., Clark, C., Taylor, R., Kirkland, C. L., & Kylander-Clark, A. (2017). Linking the Windmill Islands, east Antarctica and the Albany–Fraser Orogen: Insights from U–Pb zircon geochronology and Hf isotopes. Precambrian Research, 293, 131–149. https://doi.org/https://doi.org/10.1016/j.precamres.2017.03.005
- Munson, T. J. (2014) Petroleum geology and potential of the onshore Northern Territory, 2014 (Report 22). Northern Territory Geological Survey. https://geoscience.nt.gov.au/gemis/ntgsjspui/handle/1/81558
- Munson, T. J. (2016). Sedimentary characterisation of the Wilton package, greater McArthur Basin, Northern Territory (Record 2016-003). Northern Territory Geological Survey. https://geoscience.nt.gov.au/gemis/ntgsjspui/handle/1/83806
- Munson, T. J., & Revie, D. (2018). Stratigraphic subdivision of the Velkerri Formation, Roper Group, McArthur Basin, Northern Territory (Record 2018-006). Northern Territory Geological Survey. https://geoscience.nt.gov.au/gemis/ntgsjspui/handle/1/87322
- Page, R. W., Compston, W., & Needham, R. S. (1980). Geochronology and evolution of the late Archaean basement and Proterozoic rocks in the Alligator Rivers Uranium Field, Northern Territory, Australia. In J. Ferguson, & A. B. Goleby (Eds.), Uranium in the Pine Creek Geosyncline: Proceedings of the International Uranium Symposium on the Pine Creek Geosyncline, Sydney, 4–8 June 1979 (pp. 39–68). International Atomic Energy Agency. https://inis.iaea.org/collection/NCLCollectionStore/Public/12/629/12629252.pdf
- Rawlings, D. J., Haines, P. W., Madigan, T. L. A., Pietsch, B. A., Sweet, I. P., Plumb, K. A., & Krassay, A. A. (1997). Arnhem Bay–Gove, Northern Territory (2nd ed., Sheets SD53-3, 4. 1:250 000 geological map series explanatory notes). Northern Territory Geological Survey. https://geoscience.nt.gov.au/gemis/ntgsjspui/handle/1/81904.
- Smithies, R. H., Howard, H. M., Evins, P., Kirkland, C. L., Kelsey, D. E., Hand, M., Wingate, M. T. D, Collins, A. S., Belousova, E. A., & Allchurch, S. (2010). Geochemistry, geochronology, and petrogenesis of Mesoproterozoic felsic rocks in the west Musgrave Province, Central Australia, and implications for the Mesoproterozoic tectonic evolution of the region (Report 106). Geological Survey of Western Australia. http://dmpbookshop.eruditetechnologies.com.au/product/geochemistry-geochronology-and-petrogenesis-of-mesoproterozoic-felsic-rocks-in-the-west-musgrave-province-central-australia-and-implications-for-the-mesoproterozoic-tectonic-evolution-of-the-region.do
- Smithies, R. H., Spaggiari, C. V., & Kirkland, C. L. (2015). Building the crust of the Albany-Fraser Orogen; constraints from granite geochemistry (Report 150). Geological Survey of Western Australia. http://dmpbookshop.eruditetechnologies.com.au/product/building-the-crust-of-the-albany-fraser-orogen-constraints-from-granite-geochemistry.do
- Smithies, R. H., Spaggiari, C. V., Kirkland, C. L., Howard, H. M., & Maier, W. D. (2013). Petrogenesis of gabbros of the Mesoproterozoic Fraser Zone: constraints on the tectonic evolution of the Albany-Fraser Orogen (Record 2013/5). Geological Survey of Western Australia. http://dmpbookshop.eruditetechnologies.com.au/product/petrogenesis-of-gabbros-of-the-mesoproterozoic-fraser-zone-constraints-on-the-tectonic-evolution-of-the-albany-fraser-orogen.do
- Spaggiari, C. V., Kirkland, C. L., Pawley, M. J., Smithies, R. H., Wingate, M. T. D., Doyle, M. G., Blenkinsop, T. G., Clarke, C., Oorschot, C. W., Fox, L. J., & Savage, J. (2011). The geology of the east Albany-Fraser Orogen—a field guide (Record 2011/23). Geological Survey of Western Australia. http://dmpbookshop.eruditetechnologies.com.au/product/the-geology-of-the-east-albany-fraser-orogen-a-field-guide.do
- Steiger, R. H., & Jäger, E. (1977). Subcommission on geochronology: Convention on the use of decay constants in geo- and cosmochronology. Earth and Planetary Science Letters, 36(3), 359–362. https://doi.org/10.1016/0012-821X(77)90060-7
- Stuart-Smith, P. G., & Needham, R. S. (1984). Late Proterozoic peralkaline intrusives of the Alligator Rivers region, Northern Territory. BMR Journal of Australian Geology and Geophysics, 9, 9–12. Geoscience Australia. http://pid.geoscience.gov.au/dataset/ga/81166
- Sweet, I. P., Brakel, A. T., Rawlings, D. J., Haines, P. W., Plumb, K. A., & Wygralak, A. S. (1999). Mount Marumba, Northern Territory (2nd ed., Sheet SD53-6. 1:250 000 geological map series explanatory notes). Australian Geological Survey Organisation. http://pid.geoscience.gov.au/dataset/ga/11223
- Sweet, I. P., & Jackson, M. J. (1986). BMR stratigraphic drilling in the Roper Group, Northern Territory, 1985 (Record 1986/19). Bureau of Mineral Resources. http://pid.geoscience.gov.au/dataset/ga/14111
- Thorne, J. P., Cooper, M., & Claoué-Long, J. C. (2014). Guide to using the Australian Mafic–Ultramafic Events GIS Dataset: Archean, Proterozoic and Phanerozoic Magmatic Events (Record 2014/39). Geoscience Australia. https://doi.org/https://doi.org/10.11636/Record.2014.039
- Wang, Q.-H., Yang, H., Yang, D.-B., & Xu, W.-L. (2014). Mid-Mesoproterozoic (∼1.32 Ga) diabase swarms from the western Liaoning region in the northern margin of the North China Craton: Baddeleyite Pb–Pb geochronology, geochemistry and implications for the final breakup of the Columbia supercontinent. Precambrian Research, 254, 114–128. https://doi.org/https://doi.org/10.1016/j.precamres.2014.08.005
- Whelan, J. A., Beyer, E. E., Donnellan, N., Bleeker, W., Chamberlin, K. R., Soderlund, U., & Ernst, R. E. (2016). 1.4 billion years of Northern Territory geology: insights from collaborative U–Pb zircon and baddeleyite dating. In G. C. MacDonald (Ed.), Annual Geoscience Exploration Seminar (AGES) Proceedings, Alice Springs, Northern Territory, 15–16 March 2016 (pp. 115–123). Northern Territory Geological Survey. https://geoscience.nt.gov.au/gemis/ntgsjspui/handle/1/82725
- Wingate, M. T. D., & Compston, W. (2000). Crystal orientation effects during ion microprobe U–Pb analysis of baddeleyite. Chemical Geology, 168(1–2), 75–97. https://doi.org/10.1016/S0009-2541(00)00184-4
- Xu, H., Yang, Z., Peng, P., Meert, J. G., & Zhu, R. (2014). Paleo-position of the North China craton within the supercontinent Columbia: Constraints from new paleomagnetic results. Precambrian Research, 255(1), 276–293. https://doi.org/https://doi.org/10.1016/j.precamres.2014.10.004
- Yang, B., Collins, A. S., Cox, G. M., Jarrett, A. J. M., Denyszyn, S., Blades, M. L., Farkaš, J., & Glorie, S. (in press). Using Mesoproterozoic sedimentary geochemistry to reconstruct basin tectonic geography and link organic carbon productivity to nutrient flux from a northern Australian Large Igneous Province. Basin Research, https://doi.org/https://doi.org/10.1111/bre.12450.
- Yang, B., Smith, T. M., Collins, A. S., Munson, T. J., Schoemaker, B., Nicholls, D., Cox, G., Farkas, J., & Glorie, S. (2018). Spatial and temporal variation in detrital zircon age provenance of the hydrocarbon-bearing upper Roper Group, Beetaloo Sub-basin, Northern Territory, Australia. Precambrian Research, 304, 140–155. https://doi.org/https://doi.org/10.1016/j.precamres.2017.10.025
- Zhang, S.-H., Zhao, Y., Li, X.-H., Ernst, R. E., & Yang, Z.-Y. (2017). The 1.33–1.30 Ga Yanliao large igneous province in the North China Craton: Implications for reconstruction of the Nuna (Columbia) supercontinent, and specifically with the North Australian Craton. Earth and Planetary Science Letters, 465, 112–125. https://doi.org/https://doi.org/10.1016/j.epsl.2017.02.034
- Zhang, S.-H., Zhao, Y., Yang, Z.-Y., He, Z.-F., & Wu, H. (2009). The 1.35 Ga diabase sills from the northern North China Craton: Implications for breakup of the Columbia (Nuna) supercontinent. Earth and Planetary Science Letters, 288(3–4), 588–600. https://doi.org/https://doi.org/10.1016/j.epsl.2009.10.023