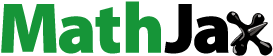
Abstract
The Fraser Zone of the Albany-Fraser Orogen of Western Australia contains an extensive suite of mafic and mafic–ultramafic intrusions. This includes the Nova-Bollinger intrusions (ca 1300 Ma), which host economic Ni-sulfide mineralisation, a prime example of magmatic sulfide liquids emplaced into the deep crust under ambient granulite facies conditions. Sulfur, Sr and Sm–Nd isotopes, and trace elements have been used to evaluate the role of crustal contamination of the parental magmas to Nova-Bollinger and other sulfide-bearing mafic–ultramafic bodies within the Fraser gabbro suite. All ore types have δ34S values between +1.6 and +8.0‰ VCDT with a positively skewed distribution and a median value of +2.3‰ VCDT, anomalous relative to uncontaminated mantle values near 0‰ VCDT. No significant Δ33S anomalism was observed. The initial 87Sr/86Sr and 147Sm/143Nd ratios of most of the Nova-Bollinger igneous rocks have an age of formation of 1300 Ma, which is similar to those of CHUR1300Ma and record limited contamination by lower continental crustal material. The country rocks of the Snowys Dam Formation, locally enriched in iron and sulfur, are likely candidates as crustal contaminants. These metasedimentary sulfides show similar ranges in values for both δ34S and Δ33S to the ores, but their Sr isotopic range is greater than observed in the host intrusions at Nova-Bollinger and in most of the other sampled intrusions. Bollinger globular sulfides with ɛSr values of 63.1 and 144.5 and ɛNd values of −5.7 and −1.8 are suggestive of localised crustal contamination from adjacent wall rock marbles, but otherwise high ɛSr country rocks do not appear to have been dominant contaminants. Bulk assimilation of the country rocks, as modelled in both silicate and sulfide portions of the system, of up to 30% contamination of a primitive mantle melt by siliceous country gneisses, would have been sufficient to produce the degree of crustal contamination recorded in the Nova-Bollinger ores, and more regionally within the Fraser gabbros. Beyond indications that S was crustally derived, it is not possible to identify a specific source, but Snowys Dam sediments are the most likely. There is no compelling evidence for Archean S in the ores, unsurprisingly given the mid-Proterozoic age. It is likely that much of the contamination of the magma and generation of sulfide liquid took place during lateral flow through linked sill complexes within the deeply buried Snowys Dam metasediments, prior to the emplacement of the magmas to form the orebodies.
Nova-Bollinger Ni–Cu–Co sulfide ores have δ34S values between +1.6 and +8.0‰ VCDT, with a median value of +2.3‰ VCDT, anomalous relative to uncontaminated mantle values but similar to the range in the country rock metasediments. Values are consistent with equilibration of sulfide xenomelts at R factors of 200–700.
There is no detectable mass-independent fractionation of δ33S in the Nova-Bollinger ores.
The initial 87Sr/86Sr and 147Sm/143Nd ratios of most of the Nova-Bollinger igneous rocks at the age of formation of 1300 Ma are similar to those of CHUR1300 Ma and record contamination of a primitive mantle melt by up to 30% lower continental crustal material.
Unusual globular ores at Bollinger have anomalous ɛSr indicating localised contamination from adjacent wall rock marble; however, this contamination is not pervasive through the orebody. It is likely that much of the contamination of the magma and generation of sulfide liquid took place during lateral flow through linked sill complexes within the deeply buried Snowys Dam metasediments, prior to the emplacement of the magmas to form the orebodies.
KEY POINTS
Introduction
The Nova-Bollinger Ni-sulfide ore deposit, 160 km east-northeast of Norseman, WA (), is an example of magmatic sulfide mineralisation in a small ultramafic–mafic intrusion within an orogenic belt. This is a class of deposits that has become prominent in the literature on magmatic sulfide ores in recent years and also accounts for a high proportion of new discoveries that have been made over the past two decades. Other examples of the class include the Kalatongke deposit in China (Gao & Zhou, Citation2013; Kang et al., Citation2020; Li et al., Citation2012; Mao et al., Citation2022; Qian et al., Citation2009), numerous deposits in the Tianshan region of the Central Asian Orogenic Belt in China (Lu et al., Citation2019; Mao et al., Citation2019), Xiarihamu in Tibet (Li et al., Citation2015; Song et al., Citation2016), Limoeiro in Brazil (Mota-e-Silva et al., Citation2013) and Savannah (formerly Sally Malay) in northern Australia (Le Vaillant et al., Citation2020). The Nova-Bollinger deposit is unusual in that it was emplaced into granulite facies rocks at lower crustal depths, representing a prime example of this association. Along with the Savannah deposits, it is probably the most deeply emplaced of any magmatic sulfide deposit known worldwide. Its discovery in an otherwise entirely greenfields terrane (Bennett et al., Citation2014) opened up an entirely new search space within the 1500 km long Albany-Fraser Orogen and reinvigorated the Australian nickel exploration effort.
Figure 1. Regional geology of Albany-Fraser Belt, outlining the location of the Fraser Zone, modified from Kirkland et al. (Citation2015), showing location of Nova-Bollinger and other Ni–Cu sulfide occurrences and deposits.
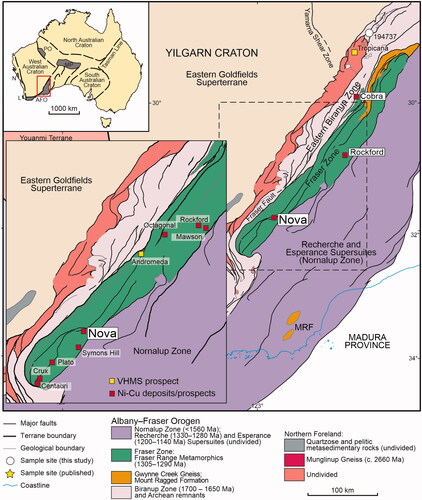
Numerous other magmatic sulfide Ni–Cu–Co deposits and prospects have been identified since this discovery, including Silver Knight, Mawson, Octagonal and Plato (), but to date the Nova-Bollinger discovery remains the largest and also the only operating mine. Understanding the potential for economic deposits elsewhere within the orogen remains an important research goal.
A key unsolved problem at Nova-Bollinger, as in many other intrusion-hosted Ni–Cu sulfide deposits, is the timing of sulfide liquid generation and the origin of the sulfur. While there is a general consensus that externally derived crustal S is an essential component of this deposit style (Ripley & Li, Citation2013), not all deposits show a clear isotopic signal for crustal S, and debate continues as to the possibility of S being endogenously derived from the mantle source of the carrier magmas (Seat et al., Citation2009).
The purpose of this communication is to present new S, Sr and Sm–Nd isotopic data from the Nova-Bollinger deposit and its host rocks, in conjunction with trace-element data previously reported by Taranovic et al. (Citation2022) and sulfide tenor data reported by Barnes et al. (Citation2022a), to constrain the source and degree of potential crustal contamination and the role of contamination in ore genesis. Data are compared with new and previously published data on other mafic and ultramafic rocks within the Fraser Zone. Establishing the source of S at Nova would provide key insights into ore formation processes and prospectivity within the Albany-Fraser Orogen, and more generally into genetic processes in magmatic systems at the base of the continental crust.
Geological setting
The Fraser Zone is a 425 × 50 km linear component of the eastern arm of the Paleo to Meso-Proterozoic Albany-Fraser Orogen (Maier et al., Citation2016; Spaggiari et al., Citation2015) (), clearly delineated by its strong gravity and magnetic signature. It has received close attention over the last decade due to the discovery of the Nova-Bollinger Ni–Cu–Co sulfide ore deposit, associated with a suite of mafic–ultramafic intrusions belonging to the granite-dominated 1330–1280 Ma Recherche Supersuite, the magmatic component of Stage 1 of the Albany-Fraser Orogeny () (Smithies et al., Citation2013; Spaggiari et al., Citation2015). Within the Fraser Zone, a voluminous suite of mafic–ultramafic intrusions was emplaced into sedimentary rocks of the Snowys Dam Formation, deposited within the uppermost part of the Arid Basin after 1332 ± 21 Ma (Spaggiari et al., Citation2015). On a regional scale, the Snowys Dam Formation contains a variety of metamorphosed pelitic and psammitic sediments, marls, limestones, iron formations and probable volcaniclastic rocks developed in a marginal basin with clastic material derived from the Biranup and Nornalup zones in addition to some more distal exotic components (Spaggiari et al., Citation2015).
The mafic–ultramafic intrusions, known informally as the Fraser gabbros (Smithies et al., Citation2013), range in age from 1305 to 1290 Ma (Fletcher et al., Citation1991; Glasson et al., Citation2019; Smithies et al., Citation2013), including the Nova intrusions at 1304 ± 22 Ma (Morrison et al., Citation2022; L. Thomas, unpublished thesis). The Fraser gabbros as a whole are regarded as an extensive regional sheeted sill complex (Spaggiari et al., Citation2011). They range from completely retextured mafic granulites to a suite of igneous intrusions (including Nova, Plato and others located on ) mostly retaining primary igneous cumulate textures and having parent magmas with MgO estimated at around 10% (at Nova specifically). Deformation and granulite-facies metamorphism was synchronous with magmatism, with conditions estimated at 7–9 kb and 810–860 °C (Clark et al., Citation2014; Glasson et al., Citation2019; Torres-Rodriguez et al., Citation2021) commencing at 1304 ± 7 Ma. Sulfide ore textures and other lines of evidence strongly indicate that the igneous-textured intrusions were emplaced synchronously with the metamorphic peak at depths greater than 20 km in the crust (Barnes et al., Citation2020a; Taranovic et al., Citation2022). The emplacement age of the mafic granulite gneisses within the Snowys Dam sequence remains imprecisely known, but they are clearly intruded by the ca 1300 Ma igneous-textured intrusions at Nova. Smithies et al. (Citation2013) identified two main types of gabbro: a ‘hybrid’ and a ‘main’ gabbro based on field observations and geochemistry. Hybrid gabbros were found to have higher modal proportions of quartz and feldspars, and appear to have interacted with assimilated felsic material, whilst main gabbros did not have this interaction. The gabbro melt was most likely achieved by <10% melting of a spinel lherzolite (Smithies et al., Citation2013).
The Fraser Zone has been interpreted as an up-thrust ‘hot zone’, formed by repeated intrusion of mantle-derived magmas into middle to deep-crustal country rocks (Smithies et al., Citation2013; Spaggiari et al., Citation2015). The tectonic setting that this occurred in has been interpreted as either a backarc, potentially with a subduction polarity change (Glasson et al., Citation2019; Kirkland et al., Citation2011a; Spaggiari et al., Citation2015), or due to deep crustal delamination against a cratonic backstop following island arc–continent collision (Sippl et al., Citation2017; Spaggiari et al., Citation2018).
The Snowys Dam metasedimentary rocks form the upper component of the Arid Basin, located at the margin of the Yilgarn Craton and Biranup Zone, separated by the Nornalup Zone representing an ocean–continent transition (Spaggiari et al., Citation2015). The formation is composed of pelitic and semipelitic paragneiss with minor calc-silicate layers (metamorphosed marls) and marbles interlayered with mafic amphibolite or granulite (Smithies et al., Citation2013). Local iron and sulfur enrichments may have been a source of sulfur for the formation of magmatic Ni-sulfide ore deposits in the Frazer Zone (Walker et al., Citation2019).
Nova-Bollinger intrusive complex
The Nova-Bollinger igneous system contains multiple intrusive bodies, of which two have been intensively drilled and studied (Taranovic et al., Citation2022): the Upper Intrusion, consisting of interlayered mafic and ultramafic cumulate rocks; and the Lower Intrusion, comprising mafic and ultramafic cumulate rocks hosting the bulk of sulfide mineralisation (). Taranovic et al. (Citation2022) found that both intrusions were derived via multiple magma pulses of liquid–olivine–sulfide slurries with variable amounts of orthopyroxene, emplaced into the deep crust at pressures of around 0.7 GPa during the peak of regional metamorphism. Most of the sulfide ores are hosted in the Lower Intrusion ().
Figure 2. (a) Simplified Nova-Bollinger east–west schematic section, looking north. Modified from (Parker et al., Citation2017). (b) Interpretative east–west long section constructed using drill-hole logging data with isotopic analyses sample locations. Sulfur isotopic analysis sample locations are shown with yellow stars, Sr and Sm–Nd isotopic analysis sample locations are shown with white stars.
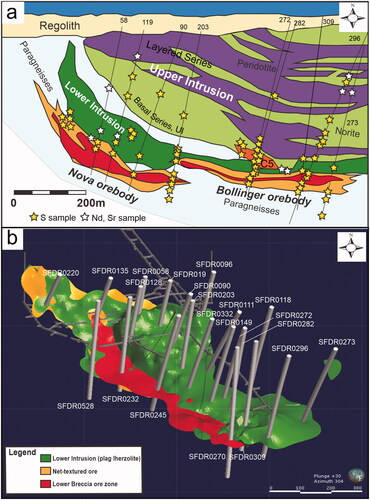
The Upper Intrusion () is a bowl-shaped, internally layered body extending about 2.4 km N–S and about 1.2 km E–W and up to 450 m in thickness, divided into an upper Layered Series and a lower Basal Series. The Layered Series comprises feldspathic lherzolite layers of variable thickness from about 20 cm to 100 m interlayered at sharp phase appearance contacts with norite to gabbronorite rocks. Olivine within the lherzolites shows a distinct signal of sulfide-related olivine depletion (Barnes et al., Citation2022b). The lower Basal Series of the Upper Intrusion is a homogenous to vari-textured unit up to 100 m thick of predominantly orthocumulate norite, olivine orthopyroxenite and feldspathic lherzolite. Taranovic et al. (Citation2022) interpreted this unit to be a ‘basal reversal’ (Latypov, Citation2015) component of the Upper Intrusion.
The Lower Intrusion (), up to about 100 m thick, 300–500 m wide and at least 1500 m long, is a chonolith with a lens-shaped cross-section. Main lithologies are orthocumulate feldspathic lherzolite to poikilitic melagabbro. Abundant orthopyroxene–spinel–hornblende symplectites found at olivine–plagioclase contacts as reaction coronas are ubiquitous in both intrusions (Torres-Rodriguez et al., Citation2021). Olivine clinopyroxenite with globular disseminated sulfides are a less abundant lithology found in the disseminated to net-textured ores in the Lower Intrusion within the Bollinger orebody (Barnes et al., Citation2020a), spatially associated with assimilation of carbonate-rich country rocks and the presence of miarolitic cavities containing calc-silicate mineral assemblages. Taranovic et al. (Citation2022) concluded that the intrusions developed initially as a bifurcating sill, via multiple magma pulses of liquid–olivine–sulfide slurries with variable amounts of orthopyroxene. The lower arm developed into the ore-bearing Lower Intrusion chonolith and the upper inflated into the layered Upper Intrusion.
Sulfide mineralisation
Almost all of the sulfide ores are hosted in the Lower Intrusion (), the geometric form of which is similar to that of other small-intrusion hosted ‘conduit’-style deposits derived from mafic magmas (Ripley & Li, Citation2011) such as those at Savannah (Le Vaillant et al., Citation2020), Tamarack (Taranovic et al., Citation2015, Citation2016), Eagle (Ding et al., Citation2010, Citation2012), Jinchuan (Chai & Naldrett, Citation1992; Li & Ripley, Citation2011; Song et al., Citation2012) and Voisey’s Bay (Lightfoot et al., Citation2012; Li & Naldrett, Citation1999; Naldrett et al., Citation1996; Saumur & Cruden, Citation2015). The mineralisation is divided into the Nova, to the east, and Bollinger, to the west, orebodies (), with a combined pre-mining resource estimate of 13.1 Mt at about 2 wt% Ni, 0.8 wt% Cu and 0.1 wt% Co based on resource figures quoted to the Australian Stock Exchange by IGO Ltd. in 2019.
The Upper Intrusion contains greatly subordinate amounts of sulfides compared to the Lower Intrusion. The largest accumulation of disseminated sulfides is found within the C5 zone (), stratigraphically above the Bollinger ore body within the lower marginal zone of the Upper Intrusion at the point where it is in direct contact with the Lower Intrusion.
The Lower Intrusion predominately hosts net-textured and interstitial disseminated ores in the terminology of Barnes et al. (Citation2017). Locally at Bollinger it contains globular disseminated ores. Massive sulfide veins, sulfide matrix ore breccias and basal massive sulfide segregations are also found in both the Nova and Bollinger ore bodies (), invariably associated with the Lower Intrusion. Much of this sulfide-rich ore is present as semi-massive vein arrays, breccias and infiltration zones extending up to 50 m from the intrusion into the footwall gneisses (Barnes et al., Citation2020a). In some cases, these veins are found to be in close spatial association with felsic pegmatitic rocks sharing space and interacting with them (Barnes et al., Citation2020a), as shown in . Massive sulfides are also localised in boudin necks and hinge zones of recumbent folds. These tectonically mobilised sulfides are found within the intrusion and in the country rocks, on a scale of centimetres to metres. All the massive sulfides, including the mobilised veins, are characterised by well-developed pentlandite–chalcopyrite–pyrrhotite loop textures () attributable to prolonged post-emplacement cooling under granulite facies conditions within the sulfide melting range (Barnes et al., Citation2020b). Sulfide emplacement is interpreted to be a continuum from syn-emplacement sulfide melt migration at an extensive melting-infiltration front (Barnes et al., Citation2018) to tectonic mobilisation during syn- and post-emplacement deformation.
Figure 3. Sulfide ores—underground face pictures (left) and detail (right). (a) Nova breccia—massive ore, variably disaggregated clasts of mafic granulite in sulfide matrix; leu, Si-rich leucosome derived by partial melting of adjacent country rock; (b) same, detail of massive ore showing Po–Pn–Ccp loop texture (Barnes et al., Citation2021), typical of all sulfide-rich ores at Nova-Bollinger. (c) Bollinger, ‘Leopard’ net textures ores, olivine-sulfide cumulate—primary magmatic layering defines by flattened clumps of sulfide-free olivine. (d, e) Photomicrographs of net-textured ore containing magnetite crystallised from sulfide liquid. (f, g) Globular sulfide ore from Bollinger—flattening of globules defines igneous layering. (g) is a phase map showing sulfide globule within a sub-spherical aggregate of diopside + dolomite representing a former CO2-rich fluid bubble (Barnes et al., Citation2021).
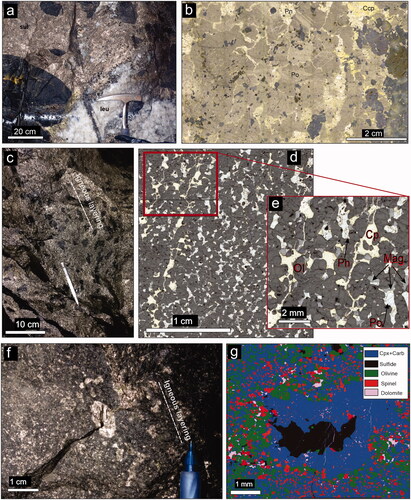
Disseminated sulfides form blebs interstitial to olivine and pyroxene grains ranging in size from 0.2 to 3 mm in size. With increasing abundance these pass into net-textured ores () defined by a network of sulfides encasing slightly rounded euhedral olivine and more rarely pyroxene. These ores also form ‘leopard texture’ () with sulfide-free silicate patches on a scale of a few millimetres to centimetres, comprising medium-grained olivine clumps with interstitial clinopyroxene and hornblende–spinel symplectite after plagioclase.
Globular disseminated ores are rare, being predominantly found within the C5 mineralisation and in leopard-textured ores at Bollinger only (). Sub-spherical sulfide blebs on a scale of about 1 cm are found within unusually fine-grained, spinel-rich leopard textured ore. Bollinger globular sulfides are hosted in a clinopyroxenite, a rare lithology within the system. The globules here occur in close association with carbonate- and calc-silicate filled miarolitic cavities in the margin of the Lower Intrusion in contact with marble. Barnes et al. (Citation2020a) interpreted these features to represent segregation vesicles derived by infilling of CO2 vapour bubbles.
Nova and Bollinger Ni–Cu tenors (metal contents in 100% sulfide) fall across the typical range of deposits hosted by mafic–ultramafic intrusions with mafic parent magmas (Barnes et al., Citation2022a). Sulfide tenors (metal contents in 100% sulfide) show a consistent change from Bollinger to Nova but are indistinguishable between the eastern and western portions of the Nova orebody and show no systematic variation with distance from the country rock contact. At Nova, 90% of samples fall between 5 and 8 wt% Ni and between 0.5 and 10 wt% Cu, with modes at 7 wt% Ni and 2.5 wt% Cu, compared with 4–7 wt% Ni and 0.5–8 wt% Cu at Bollinger with modes at 5.5 wt% Ni and 2 wt% Cu. Their PGE tenors, as exemplified by Pd, fall at the lower end of the mafic range, making Nova-Bollinger among the lowest-PGE magmatic sulfide ores known. Relative to potential mantle sources, Nova-Bollinger ores as a whole are depleted in Pd over Cu by a factor between 10 and 100. The average Ni/Cu ratios are somewhat high compared with many deposits formed from mafic magmas, a feature that is attributed to open-system equilibration of sulfides with flowing olivine–sulfide slurries, the olivine contributing additional Ni to the overall mass balance (Barnes et al., Citation2013; Citation2022a).
Country rocks
The immediate host rocks to the Nova-Bollinger ore deposit are the Snowys Dam Formation garnet-rich pelitic and semipelitic gneisses, calc-silicate rocks, marbles, and mafic plagioclase–orthopyroxene–clinopyroxene granulites (). Iron-rich and S-rich metasedimentary rocks are present within the Snowys Dam sequence within a few kilometres of the deposit but are not prominent in the immediate host rocks to the intrusions based on the drilling to date.
Figure 4. Metasedimentary country-rock gneiss photomicrographs and XFM elemental maps of Sr–Sm–Nd samples: (a) a contact between marble and garnet–pyroxene footwall gneiss, (b) enlarged area from (a), (c) an XFM elemental map of (a), (d) plagioclase–quartz–garnet footwall gneiss, (e) an enlarged area from (d) showing garnets with silicate inclusions and fine-grained pyroxene, and (f) a micro XRF elemental map of (d). Abbreviations: Cal, calcite; Plag, plagioclase; Qtz, quartz; Grt, garnet; Px, pyroxene.
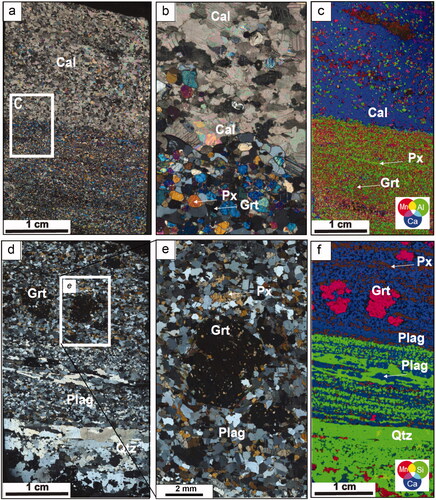
The pelitic gneiss is composed of K-feldspar (up to 40 modal%), quartz (10–25%), perthite (up to 20%), garnet (about 5–10%), pyroxenes (up to 10%), biotite (a few %) and Fe–Ti oxides, sillimanite and plagioclase as accessory phases. The iron and S-rich variety contains thin laminae of alternating magnetite, pyrrhotite and quartz, commonly with garnet and minor pyroxene. Feldspar, quartz, perthite and pyroxenes are medium-grained anhedral to subhedral grains. Poikiloblastic garnets are typically coarse-grained with inclusion trails of quartz, biotite, or Fe-oxides. Accessory phases are found dispersed throughout the rock or in small acicular/tabular aggregates.
Within the package of the Snowys Dam Formation immediately below the Nova-Bollinger ore deposit, the semipelitic to psammitic gneiss is interlayered with mafic amphibolite and marble—metamorphosed limestone or marl. These calc-silicate layers are predominately composed of fine-grained calcite and dolomite in variable proportions (). One sample of this material was analysed for Sm–Nd and Sr isotopes in this study and is referred to as the ‘Marble Gneiss’.
Previous isotopic studies
Maier et al. (Citation2016) provided a limited number of Sr, Nd and S isotopic analyses on disseminated sulfide-bearing cumulates from the Layered Series of the Upper Intrusion. They found the Upper Intrusion rocks to have εSr (38–52) and variable δ34S (+2 to +4‰ VCDT over seven samples) compared with the regional metagabbro (εSr 17–32, δ34S around 0‰ VCDT). Three samples from the Nova Upper Intrusion had εNd from −1.3 to +0.4.
Fletcher et al. (Citation1991) examined the Sr-, Nd- and Pb isotopic composition of a small set of Fraser gabbro samples (not including Nova) from the south end of the zone, concluding that these rocks were the result of remelting of a mafic underplate and intraplate at ca 1300 Ma. The mafic magmas were thought to be derived from a mildly contaminated ca 1900 Ma depleted mantle source with ca 600 Ma of crustal residence.
Walker et al. (Citation2019) collected multiple S isotope data on the Octagonal and Plato prospects and two unlocated samples from Nova-Bollinger. Seven samples from Octagonal gave δ34S values between 3.3 and 5.4‰ VCDT. Plato δ34S ranged from 0.7 to 1.5‰ VCDT over three samples., and the two Nova samples gave 2.3 and 2.4‰ VCDT. No detectable Δ33S anomalies were found in any of these samples. Walker et al. (Citation2022) reported δ34S values between +4.5 and +6.8‰ VCDT and Δ33S values between −0.25 and +0.04 over four samples from the Andromeda Zn–Cu–Ag–Au volcanogenic massive sulfide prospect 75 km NNE of the Nova Mine (). The data sets of Maier et al. (Citation2016) and Walker et al. (Citation2019, Citation2022) have been incorporated into this study.
Morrison et al. (Citation2022) reported a thermochronology study using U–Pb in apatite from two samples the Nova Lower Intrusion and two samples of mafic country rocks. Apatite U–Pb data indicate an initial 15 °C/my cooling rate to 1284 ± 12 Ma from peak metamorphic conditions of ∼850 °C at 1304 ± 22 Ma. After 1284 Ma the cooling rate slowed to 1.2 °C/my until at least 1225 ± 17 Ma.
More broadly, the chronology and tectonic evolution of the Kepa Kurl Booya Complex, comprising the Biranup, Fraser and Nornalup zones (), have been investigated using U–Pb–Lu–Hf isotopic data from zircons in the orthogneisses and metasediments (Kirkland et al., Citation2011a; Citation2011b; Smithies et al., Citation2013). These studies concluded that the complex is primarily the result of reworking of pre-existing Archean crust with the injection of large volumes of juvenile mantle-derived mafic melt.
Sampling and analytical methods
S isotopes
For S isotopic analysis, 83 samples from drill holes NBU0456, SFRD—0058, 0090, 0096, 0118, 0119, 0135, 0203, 0267, 0272, 0296, 0309, and 0332 along with seven underground samples were analysed (). These represent a variety of ore types mainly associated with the orebodies and the Lower Intrusions and include some disseminated sulfide samples from the Basal Series of the Upper Intrusion. The Sr, Sm–Nd isotopic compositions of 10 samples from drill holes SFRD—0058, 0272 and 0296 along with two underground samples were obtained (). Owing to the low volume of disseminated sulfides and bulk sulfide analysis type, the Layered Series of the Upper Intrusion was not analysed in this study, but we incorporate previous results from Maier et al. (Citation2016). Full data are presented in supplementary Table S1.
Sulfur isotopic analysis was performed at University of Queensland Stable Isotope Geochemistry Laboratory. An Elemental Vario Isotope Cube Elemental Analyzer coupled to a PrecisION isotope ratio mass spectrometer (EA-IRMS) was used to simultaneously determine δ34S and δ33S through focusing on SO (masses 48, 49, 50) with the SO2 fragment ion produced in the source. This is an updated method following on from Baublys et al. (Citation2004). Calibration was performed via three-point normalisation using international silver sulfide standards IAEA–S1, S2 and S3 with S-MIF laboratory pyrite standard WD-11 analysed as an unknown. Values of δ34SVCDT for S1, S2 and S3 were −0.30, 22.67 and −32.3 or −32.55‰, respectively (Ding et al., Citation2001) while δ33S values for S1, S2 and S3 were −0.05, 11.48 and −16.65‰, respectively (Baublys et al., Citation2004). Sample reproducibility determined by multiple analyses of standards is ±0.2‰; instrumental measurement uncertainty is less than ±0.05‰ for δ34S. Sample reproducibility for δ33S was ±0.3‰.
The δ33S and δ34S values were used to calculate Δ33S (EquationEqn. 1(1)
(1) ), which represents the deviation from the mass-dependent fractionation line and therefore allows for detecting anomalous 33S signatures.
(1)
(1)
Full data for S isotope analyses collected in this study are archived online.
Sr and Sm–Nd isotopic analyses
Isotopic Sr, Sm and Nd data from 10 samples from the Upper Intrusion, Nova Lower and Bollinger Lower Intrusion, Middle (Norite-Gabbronorite) Intrusion, footwall Garnet Gneiss and Marble Gneiss were analysed at University of Adelaide Mawson Analytical Services (MAS). Approximately 70–200 mg sample powders were digested with an optimised amount of (147Sm + 150Nd) mixed spike. Digestions were carried out in Savillex 15 mL PFA Teflon vials. Initial digestion was performed in 2 mL 7 M HNO3 and 4 mL 28 M HF on hotplate at 140 °C for at least 48 hours with an addition of 1 mL 15 M HNO3 before taken to final dryness. Samples were re-digested in 2 mL 7 M HNO3 and 4 mL 28 M HF on a hotplate at 140 °C for at least another 48 hours. Evaporation to almost dryness on hotplate at 140 °C was followed by an addition of 1 mL 15 M HNO3 before taken to final dryness. Then, the sample was re-dissolved in 6 mL 6 M HCl for conversion to chlorides.
Strontium and Sm–Nd separation was performed via column chromatography: 2 mL AG 50 W X8 200–400 mesh resin in Polyprep columns for Sr and REE (Sm–Nd) extractions, followed by Sm–Nd separation using 1 mL Eichrom Ln resin SPS in quartz glass columns; Sr was separated via 200 µL 50–100 um Eichrom Sr resin in Biospin microcolumns.
Isotopic abundance ratio measurements were carried out on an ISOTOPX Phoenix Thermal Ionisation Mass Spectrometer. Multidynamic Sr measurement with at least five blocks of 20 cycles (100 ratios) of measurements were taken. Normalisation to 86Sr/88Sr = 0.1194 was performed using exponential mass fractionation correction. Multidynamic Nd isotopic measurement with at least five blocks of 20 cycles (100 ratios) of measurements were taken. Normalisation to 146Nd/144Nd = 0.7219, using exponential mass fractionation correction was performed. Nd concentrations were corrected for 100 pg blank. Static Sm isotopic measurement with at least five blocks of 20 cycles (100 ratios) of measurements taken. Sm concentrations corrected for 50 pg blank. Reagents used were AR-grade hydrochloric acid, hydrofluoric acid and nitric acids distilled in Savillex DST-1000 PFA stills, and ultrapure deionised water (18.2 MΩ·cm) produced using Millipore Direct-Q 3 system. The 2σ uncertainty on εNd is estimated at ±0.7 based on a combination of the error bars on 143Nd/144Nd (±0.000003) and 147Sm/144Nd (± relative 2%). The ratio 87Rb/87Sr was not measured directly, so the age correction for 87Sr/86Sr was made using the whole-rock Rb and Sr concentrations and the common relative isotope abundances. A ±20% relative error in this value is the largest source of uncertainty and propagates into an uncertainty in εSr of ±0.8. If this uncertainty is increased to an implausibly high relative 50%, this uncertainty increases to ±2.0, or 0.00015 on age-corrected 87Sr/86Sr. These uncertainties are very small compared with the spread of analyses between samples. Age corrections used decay constant values of 6.54 × 10−12 for 147Sm decay and 1.42 × 10−11 for 87Rb. Full data are presented in .
Table 1. Nd and Sr isotopic data for Bollinger globular sulfide and metasedimentary wall rocks at Nova-Bollinger.
A further group of three samples from Nova-Bollinger and six samples from other intrusions in the Fraser Zone were analysed for Sr and Nd isotopes at the University of Melbourne, following methods described in Yaxley et al. (Citation2013) and Maas et al. (Citation2015). At Melbourne, sample powders were spiked with 149Sm–150Nd and 85Rb–84Sr tracers, and dissolved at high pressure, followed by elemental separation using standard element extraction (Eichrom resins) and cation-exchange techniques. Small splits of these unspiked digests were used for determination of Rb, Sr, U, Th and Pb concentrations by high-precision quadrupole Inductively Coupled Plasma Mass Spectrometry (ICP-MS) (Kamber et al., Citation2003). Blank corrections were negligible in all cases. Isotopic analyses were carried out on a Nu Plasma multi-collector ICP-MS coupled to a CETAC Aridus desolvating system. Raw ratios were internally normalised to 88Sr/86Sr = 8.37521 and 146Nd/145Nd = 2.0719425 (equivalent to 146Nd/144Nd = 0.7219), using the exponential law as part of an online iterative spike-stripping/internal normalisation procedure, and final 87Sr/86Sr and 143Nd/144Nd ratios are reported relative to SRM987 = 0.710230 and La Jolla Nd = 0.511860, respectively. External precision (reproducibility, 2r) is ±0.000040 (Sr) and ±0.000020 (Nd). Full data are presented in .
Trace-element analyses
Trace-element data in this publication are mainly derived from published or online sources. We refer the reader to Taranovic et al. (Citation2022) for Nova-Bollinger data, and the GSWA online WACHEM database (http://geochem.dmp.wa.gov.au/geochem/) (Smithies et al., Citation2013) for regional Fraser Gabbro and Snowys Dam data for details of methods and quality control.
Major- and trace-element data on Crux, Crusader, Plato and other regional Fraser Zone intrusions were measured at Bureau Veritas in Perth on 142 samples from across the Fraser Zone (Bathgate, Citation2019). All samples were cast using a 66:34 flux with a 4% Li nitrate to form a glass bead. X-ray fluorescence (XRF) analysis was then conducted to determine major oxides, Al2O3, CaO, Fe2O3, K2O, MgO, MnO, Na2O, P2O5, SiO2, SO3 and TiO2. Laser ablation inductively coupled plasma mass spectrometry (ICP-MS) was conducted to analyse the trace elements (Ag, As, Ba, Be, Bi, Cd, Ce, Co, Cr, Cs, Cu, Dy, Er, Eu, Ga, Gd, Ge, Hf, Ho, In, La, Lu, Mn, Mo, Nb, Nd, Ni, Pb, Pr, Rb, Re, Sb, Sc, Sm, Sn, Sr, Ta, Tb, Te, Th, Ti, Tl, Tm, U, V, W, Y, Yb, Zn, Zr). Data are available online.
Analytical results
S isotopes
Histograms of δ34S (in ‰ VCDT) are shown in , subdivided by orebody () or host intrusion () and coloured by ore type. Massive and semi-massive ores of vein and breccia type are grouped together. The entire set of ore samples shows a range from −0.6 to +8.0‰, with a median of +2.3, mean of +2.7, and 25th, 75th and 90th percentiles of +2.1, +2.8 and +3.7, respectively.
Figure 5. δ34S‰ (VCDT) for all units at Nova-Bollinger: (a) all ore samples; (b) all sulfides from Bollinger ore body; (c) all samples from the Nova orebody including the Panhandle; (d) sulfides from the Basal Series of the Upper Intrusion, including the C5 unit; (e) the C5 unit only; (f) metasedimentary sulfides from Snowys Dam Formation; and (g) sulfides from the Centauri, Crux, Nova-Bollinger, Plato, Octagonal and Symon’s Hill prospects. Octagonal, Plato data from Walker et al. (Citation2019).
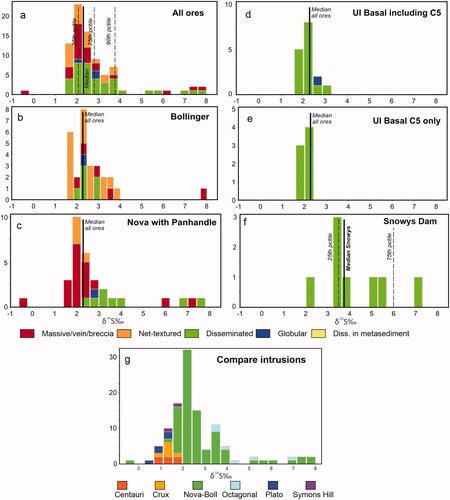
Bollinger disseminated sulfides range from +2.1 to +2.9‰; a single globular sulfide sample has a δ34S value of +2.2‰; net-textured sulfides range from +1.5 to +3.8‰; massive sulfides δ34S value ranges from +2.1 to +3.6‰ with one value of +8.0‰ (). Disseminated sulfides from the C5 mineralisation have δ34S values from +1.6 to +2.4‰. Nova disseminated sulfides () show an even wider range from +2.2 to +7.8‰; a single globular sulfide sample has a δ34S value of +2.8‰; net-textured sulfides show a narrow range from +2.0 to +2.2‰; massive sulfide δ34S values range from +2.5 to +7.3‰, a similar high value to the Lower Intrusion of +8.0‰; sulfide veins and mixed veins with massive sulfides range from +1.7 to +2.9‰. Disseminated sulfides dispersed throughout the lower marginal zone of the Upper Intrusion are characterised by a wider range of δ34S values from +1.8 to +3.3‰ (); a single blebby sulfide sample has a δ34S value of +2.8‰. Snowys Dam metasedimentary rock sulfide δ34S values range from +2.0 to +7.3‰, with a median of +3.8 and quartiles at +3.6 and +6.0 (), more enriched in 34S than the average over the whole deposit but having a similar range to the Nova deposit alone.
Disseminated sulfides from the C5 zone and Bollinger have similar ranges of δ34S values, while Nova disseminated sulfides have a range of values up to +7.8‰ comparable with that in the Nova massive ores although with a higher mean and median. Fewer samples of Nova net-textured sulfides than from Bollinger have been analysed; however, their values fall within the range of values for Bollinger net-textured sulfides. Sulfides from Nova show a positively skewed population of δ34S values (). Bollinger has one single sample in the high tail of the Nova range.
Nova δ34S values are compared with five other Fraser gabbro intrusions, Centauri, Crux, Octagonal, Plato and Symons Hill, (incorporating published data from Walker et al., Citation2019) in . Bearing in mind that only a very small number of samples were analysed from each of these bodies, Octagonal shows the widest spread of values from +3.5 to +7‰, while the others all fall within a restricted mantle-like range from +0.5 to +2‰.
Forty Nova-Bollinger samples have been analysed for δ33S in addition to 18 samples from Centauri, Crux, Plato and Symons Hill originally reported by Bathgate (Citation2019) and a further. Data are plotted in and combined with data from Walker et al. (Citation2019) for Octagonal and Plato in . All the Nova-Bollinger values of Δ33S lie within the sample precision and accuracy of the analytical measurements of ±0.3 δ33S (2σ) except for one net-textured sample from Bollinger, one net-textured sample from Nova both of which have Δ33S of −0.4‰. One sample from Symon’s Hill and three samples from Crux plot slightly outside this range, between −0.5 and −0.7‰, suggesting a slight tendency to presence of anomalous MIF S towards the south of the Fraser Zone. Excluding these small anomalies, no detectable mass anomalous fractionation of 33S has been recorded within the precision of the method. Two samples of the metasedimentary rocks of the Snowys Dam Formation are also non-anomalous in δ33S.
Figure 6. δ34S and δ33S values. (a, b) Nova-Bollinger only. (c) Nova-Bollinger compared with data from Centauri, Crux, Plato, Octagonal and Symon’s Hill prospects, and with the Andromeda VMS deposit (Walker et al., Citation2022). Octagonal, Plato data from Walker et al. (Citation2019). Arrows indicate 1σ error bars. Precision of δ33S data is ±0.3. ‘W et al N-B unspec’—Walker et al. (Citation2019) data points on two unlocated samples from Nova-Bollinger.
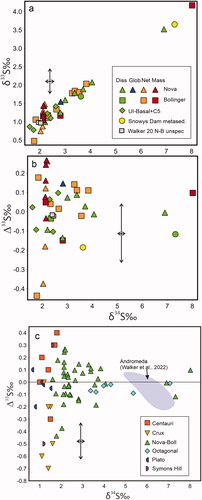
Sr and Nd isotopic systems
The 87Sr/86Sr ratios of Nova-Bollinger igneous rocks at the age of formation of 1300 Ma fall between 0.7058 and 0.7144, with the two highest values being the Bollinger globular sulfide samples. Excluding these samples, the age-corrected initial ratio range is tightly clustered between 0.7058 and 0.7110. By comparison, this ratio for CHUR1300 Ma is 0.7040 and for the Depleted Mantle1300 Ma is 0.7026 (Jacobsen & Wasserburg, Citation1978). Overall, all samples analysed show radiogenic Sr signatures with ɛSr values corrected to 1300 Ma from the Upper Intrusion layered peridotites from 19.1 to 46.4, Upper Intrusion lower marginal zone of 27 and 30, Lower Intrusion from 22.7 to 47.5, Bollinger globular sulfides of 63.4 and 148.0, country rocks Marble Gneiss of 210.0, and Garnet Gneiss of 232.1 (; ).
Figure 7. Nd and Sr data. Data points from this study, from Nova-Bollinger (8 samples, plus 2 from Snowys Dam metasediments), and one sample each from Centauri, Crux, Cobra, Plato, Rockford and Symon’s Hill (originally reported by Bathgate, Citation2019). Boxes show range of data for three samples of Upper Intrusion Layered Series (Nova UI M16) and nine samples of Fraser Zone ‘main gabbro’ (Fraser Main Gabbro M16) from Maier et al. (Citation2016) (M16). Analytical precision bars fall within the sizes of the symbols. Dashed green outline encloses all samples from the Nova-Bollinger intrusions excluding globular sulfide ores. PM1300 = primitive mantle composition at 1300 Ma.
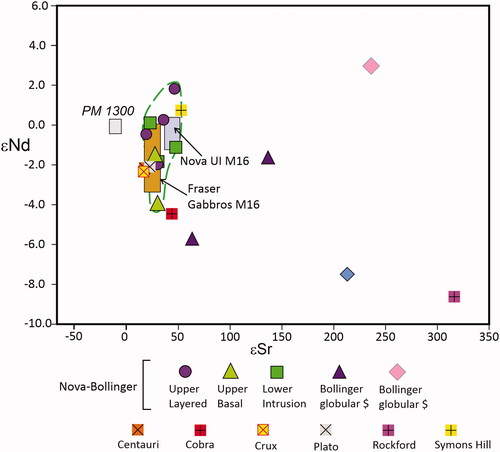
The 143Nd/144Nd ratios of Nova-Bollinger igneous rocks at the age of formation of 1300 Ma fall between 0.51067 and 0.511055. For a comparison, the same ratio for CHUR1300 Ma is 0. 5110 and for the Depleted Mantle1300 Ma is 0. 5113 (Jacobsen & Wasserburg, Citation1978). Upper Intrusion samples have a limited range of εNd values between +1.8 and −3.9. Lower Intrusion samples range from −5.7 to +0.3, with the most extreme low value being one of the Bollinger globular ore samples at −5.7 (; ). Overall, with the exception of the Bollinger globular ore samples, the Lower and Upper intrusions both show similar restricted ranges of εNd and εSr.
and also show Nd and Sr isotope data on one sample each from several of the other mafic–ultramafic intrusions in the Fraser Zone (see for locations). Excluding Rockford to the north, the various mafic and ultramafic intrusions have ɛNd values that range from +0.7 to −4.5 and ɛSr from 17.5 to 52.7, strongly overlapping with the main cluster of Nova-Bollinger data. Considerably more contaminated values are observed at Rockford, with ɛNd −8.6 and ɛSr 316.3, the most radiogenic Sr observed in any sample in the study. Of the other intrusions, Cobra has the most contaminated signal with ɛNd −4.4 and ɛSr 43.8 indicating a regional correlation of isotopic values with the most negative ɛNd samples (i.e. Cobra and Rockford) being recorded in the northern parts of the Fraser Zone (), while intrusions with less negative ɛNd are in the south.
Discussion
Isotopic compositions and crustal contamination
Results from the three isotopic systems reported here are most readily interpreted as the result of generation of sulfide liquid owing to assimilation of sulfidic country rocks. The purpose of this section is to provide some constraints on potential sources, extent and mechanism of contamination.
S isotopes
Metasedimentary Snowys Dam country rocks have δ34S values similar to the overall range of values recorded for the Lower Intrusion sulfides ( and ), suggesting that the Snowys Dam Formation is likely to have been the source of the S. This hypothesis can be tested using isotopic mixing models.
Figure 8. Model for S isotope variation as a function of mass ratio R of pure sulfide component added to a magma with an initial δ34S value of 0 assuming 500 ppm mantle-derived S in the original magma. Upper curve for δ34S 8, lower curves δ34S 4 in putative Snowys Dam contaminant based on approximate maximum and median values, respectively. Grey curve shows equivalent calculation for δ34S 8, 250 ppm in starting magma. Box-whisker plots show values for Snowys Dam sediments and Nova and Bollinger ores—box is interquartile (25th to 75th percentile), whiskers extend to ±1.5 × Q3-Q1 from box. Points are outliers beyond this range. Approximate R values are estimates for Nova and Bollinger ores based on median Ni and Pd tenors from Barnes et al. (Citation2021).
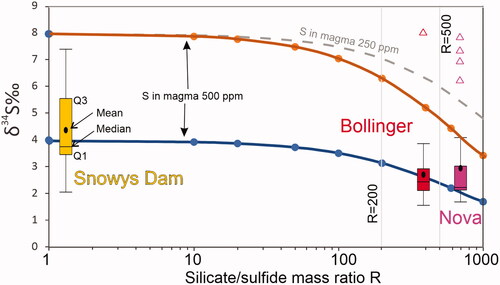
Mixing-contamination lines for δ34S can be calculated in a variety of ways, but the most commonly used in magmatic sulfide systems are (1) simple batch equilibration (Lesher & Burnham, Citation2001) for mixing of a magma containing mantle-derived S with a crustally derived sulfide xenomelt, and (2) progressive upgrading of sulfide melt by progressive reaction with uncontaminated silicate magma in a dynamic conduit system (Naldrett et al., Citation1996a; Ripley & Li, Citation2003). In both models the final isotopic composition of the resulting sulfide liquid is a weighted mixture of that in the original silicate melt before contamination, and that of the contaminant, whereby the smaller the proportion of sulfide added to a given mass of magma (higher R factor), the more the isotopic composition of the sulfide liquid resembles that of the starting magma. Numerical analysis of the two types of model by Mungall et al. (Citation2020) has shown that their consequences are indistinguishable from one another where the integrated mass ratio of sulfide to silicate at the end of the process is the same. In other words, both processes can be adequately approximated by a simple standard isotopic mixing calculation, as is presented in .
shows results of a simple mixing model for incorporating crustal S into a mantle-derived magma assumed to have an initial δ34S value of zero, corresponding to typical values for mafic intrusive rocks where no contamination is indicated (Ripley et al., Citation1999; Seat et al., Citation2009). Ranges of measured δ34S values for combined ore types at Nova-Bollinger are represented as box and whisker plots, showing that 50% of the data in both fall between +2 and +3.2‰, with outliers extending to +8‰. These ranges are plotted at R values corresponding to estimates made by Barnes et al. (Citation2022a) based primarily on Ni and Pd tenors of the ores. Model curves are shown on the assumptions of initial δ34S in the uncontaminated carrier magma of zero, 500 ppm S in the magma, and an assimilated sulfide component with δ34S of 4 and 8‰ (median and upper limit of Snowys Dam data) and 38 wt% S in the sulfide. The choice of S content for the starting magma is a conservatively low number based on typical values for basaltic magmas with around 8–10 wt% MgO (Li & Ripley, Citation2009). The sensitivity of the modelling to the assumption of initial S content in the magma is shown by the grey dashed line in , calculated for 250 ppm S—at R of 1000: this results in a model value about 1‰ higher. Results of this modelling show that most of the range of isotopic compositions of the Nova and Bollinger ores can be matched with a contaminant having δ34S towards the lower end of the Snowys Dam range at R values consistent with the Ni and PGE tenors of the massive ores (Barnes et al., Citation2022a). However, the small number of 34S enriched samples require a contaminant with δ34S around 10‰ or more. Furthermore, the wide range in outlying values suggests a degree of heterogeneity and disequilibrium, such that the sulfide melt in the orebodies was not well homogenised.
The mixing model suggests that the ores at Nova, formed at estimated R factors of around 600–800, should have had more mantle-like S isotopic signatures than Bollinger, whereas there is no significant difference between the two. The likely explanation is that magmas in both intrusions derived their S from a source or sources with a range in δ34S values greater than the expected difference owing to R factors.
No mass anomalous sulfur fractionation was observed either at Nova-Bollinger ores nor in the Snowys Dam metasedimentary rocks (), nor in the other magmatic sulfide occurrences in the Fraser Zone apart from three outlying samples from Crux and a single sample from Symons Hill, which fall by just over the analytical uncertainty outside the mantle range. Walker et al. (Citation2022) reported a small but statistically significant negative Δ33S from three out of four samples of the Andromeda VMS deposit; these values fall within the range observed in the Nova samples. Potentially, the crustal contaminant had the same non-anomalous Δ33S signature, or the more dynamic nature of the Nova-Bollinger system (high R factor) was responsible for obliterating an originally mass anomalous Δ33S signature. The latter explanation can be tested by modelling of Δ33S using the same method as that for the model in : if the initial sediment contaminant had an anomalous Δ33S of +1, the resulting magmatic sulfide ends with a Δ33S of +0.6‰ at an R value of 500, again assuming 500 ppm S in the initial silicate magma. This would be detectable given the analytical uncertainty of ±0.3‰ Δ33S. Hence, high R factor is unlikely to be the explanation; it is more likely that the contaminant was essentially indistinguishable from mantle and there is no evidence for a mass-independent S anomaly in the S source rocks, as noted previously by Walker et al. (Citation2019) for Octagonal, Nova and Plato. This implies that while the Albany-Fraser Orogen in general has a strong signature of reworked Archean basement (Kirkland et al., Citation2011a; Spaggiari et al., Citation2015), there was no detectable recycling of Archean S preserved in the mafic magmatism. This is not surprising: much of the S incorporated into the continental margin sediments of the ca 1340–1320 Ma Snowys Dam sequence is likely to incorporate an unknowable component of juvenile non-MIF fractionated S accumulated in the hydrosphere over more than a billion years since the end of the Archean. We agree with Walker et al. (Citation2019) that the effects of sedimentary processes in the hydrosphere would have diluted any traces of MIF-anomalous Archean S beyond detectability.
Sr and Nd isotopic systems
Considering the Sm/Nd isotopic system, the Nova intrusion rocks record a range in εNd from −5.7 to +1.8, with all samples except one from the Bollinger globular ores falling in a narrow range of ɛSr from 10 to 50 (). Except for the Bollinger globular sulfides, all the Nova-Bollinger rocks fall within half an ε unit of the range of the previously measured values for the Upper Intrusion and the metagabbro from the Fraser Zone as reported by Maier et al. (Citation2016) (). The range of about 8 εNd units and 120 in εSr units in the intrusions requires a role for crustal contamination at least on a local scale. The two Snowys Dam country rock samples analysed here both show high ɛSr in excess of 200 (). The Marble Gneiss and the Garnet Gneiss span the range of εNd seen in the intrusion.
shows some theoretical isotopic mixing models for Nd and Sr in an attempt to identify potential contaminants in the immediate country rocks. Mixing lines are shown for hypothetical Nova parent magmas, having 100 ppm Sr and 10 ppm Nd, with the two analysed Snowys Dam samples, the Marble Gneiss and the Garnet Gneiss () and for a hypothetical lower crust contaminant (). In the absence of a reliable estimate for the parent Nova magma, we took two hypothetical isotopic starting compositions, one corresponding to a ‘MORB’ depleted mantle (DM), and one based on a chondritic primitive mantle (PM), both at 1300 Ma (see for assumptions). Given the large uncertainties in the assumptions, the Bollinger globular ores are consistent with contamination of a DM-like parent magma with around 20% Marble Gneiss contaminant, or a PM-like parent with a component of both Marble Gneiss and Garnet Gneiss. This is consistent with the presence of unusually calcic assemblages including primary magmatic carbonate associated with the sulfides in these rocks, and the proximity of these globular ores to the Marble Gneiss unit. This interpretation contrasts with that of Blanks et al. (Citation2020) for a number of alkalic systems, where the evidence suggests that a CO2-rich volatile phase associated with magmatic sulfides was mantle-derived.
Figure 9. Isotopic mixing between εNd and 87Sr/86Sr (1300) simulating contamination of magma with primitive mantle and depleted mantle isotopic composition (a) by two different units corresponding to the Marble Gneiss and Garnet Gneiss of the Snowys Dam Formation, and (b) by a purely hypothetical lower crust-like composition; Nova shown data in relation to the compositional range of upper and lower continental crust, and contaminated continental flood basalts, after Goldstein et al. (Citation1984).
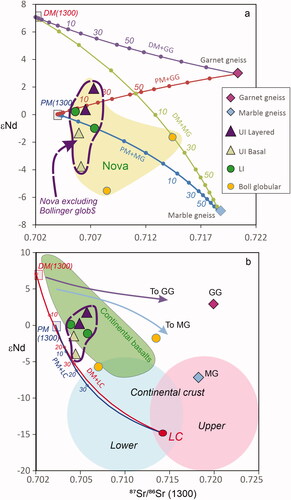
Table 2. Average trace-element contents of Nova rock types and starting assumptions for isotope and trace-element mixing models (all isotope ratios corrected to 1300 Ma).
However, the mixing trends to the Garnet Gneiss are orthogonal to the data array in the other intrusion samples (); if this sample were representative of the immediate wall rocks, the implication would be that the isotopic systematics of the Nova intrusions reflects contamination pre-emplacement rather than in situ, with the exception of local contamination to produce the Bollinger globular ores, unique to that small portion of the orebody (Barnes et al., Citation2020a). The intrusion array excluding the globular ores is best modelled as approximately 10–30% contamination of an original slightly depleted magma (between DM and PM but closer to PM) with some kind of ‘average’ lower continental crust, resembling in the overall trend the typical field seen for continental flood basalts (). A minor component of local wall rock contamination could have served to elevate the radiogenic Sr content above this hypothetical mixing line. A much larger data set would be needed on the diverse Snowys Dam assemblage on a regional scale to determine if this lower crustal material were derived from the Snowys Dam suite, or some deeper crustal basement source, but the absence of an Archean mass-independent S isotope signal favours a Snowys Dam source.
Trace-element constraints
Incompatible trace-element ratios are also potential indicators of contamination (Smithies et al., Citation2013). shows a compilation of trace-element data from this study (mainly from the regional igneous-textured intrusions) with Nova-Bollinger data from Taranovic et al. (Citation2022) and all available data on mafic rocks in the Fraser Zone from Smithies et al. (Citation2013) and from the Geological Survey of WA online geochemical database at (http://geochem.dmp.wa.gov.au/geochem/), amounting to around 130 analyses. (Samples with <0.5 ppm Nb are excluded from the data set to exclude mesocumulate rocks where element ratios are subject to large uncertainties.) A further 135 analyses of Snowys Dam metasediments from the GSWA database are used to define a data cloud for potential Snowys Dam contaminants. Plots are shown in for two incompatible trace-element ratios, Th/Yb vs Nb/Yb and Zr/Y vs Nb/Yb, commonly used as contamination indices, and also widely used as discriminant ratios for tectonic setting of mafic magmas (Pearce, Citation2014). Data for the intrusions are filtered to exclude samples with Th <0.2 ppm and Nb <0.5 ppm, to exclude large uncertainties in the ratio for samples with concentrations near the detection limit, and to minimise variability in the ratios owing to the presence of minor amounts of Yb and Y in cumulus phases (see supplementary material in Barnes et al., Citation2021, for a discussion of this effect).
Figure 10. Plot of Th/Yb and Zr/Y vs Nb/Yb in Nova Upper and Lower intrusion rocks, other mafic rocks from the Nova mine area (‘Nova Other’), combined with data from the Centauri, Cobra, Crux, Plato and Rockford intrusions (this study) and data from other Fraser Gabbro mafic rocks within the Fraser Zone from the Geological Survey of WA online database (‘Regional FG’). Data compared with hypothetical (dashed) mixing lines between a possible starting basaltic magma composition for the Nova intrusions (‘sb’) and (1) a Snowys Dam Si-rich metasediment (SDM) based on the highest data density over 135 samples; (2) average middle continental crust; and (3) average composition of the Munglinup Gneiss, believed to be representative of reworked Archean basement (see text and for details and data sources). Coloured field is kernel data density over the entire Snowys Dam data set for samples with >60% SiO2. Symbols on model curves are at 5% intervals of mass percentage of contaminant in the mixture. Data for the intrusions are filtered to exclude samples with Th <0.2 ppm and Nb < 1 ppm, to exclude large uncertainties in the ratio for samples with concentrations near the detection limit. ‘Mantle array’ field is modified from Pearce (Citation2014) based on combined data clouds from MORB, ocean plateau and ocean island basalts from the compilation of Barnes et al. (Citation2021). nMORB and PM refer to idealised mantle compositions from Pearce (Citation2014).
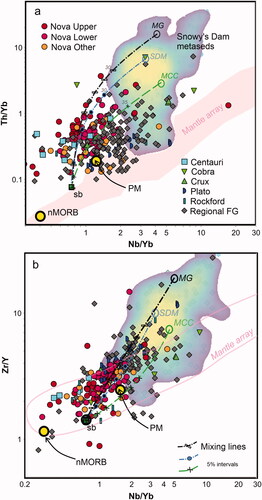
The data form a diffuse cloud on Th/Yb vs Nb/Yb, with a distinct displacement between Nova-Bollinger and the regional Fraser gabbros, which tend to have a higher Nb/Yb and overlap with the ‘mantle array’ field as originally defined by Pearce (Citation2008, Citation2014) on the Th–Yb–Nb plot. Most of the Nova-Bollinger data plot above the mantle array, with a few samples extending back to the very low Nb/Yb and relatively high Th//Yb considered by Pearce (Citation2008, Citation2014) to be diagnostic of arc magmas. Centauri data overlap with Nova-Bollinger; Plato, Crux and Rockford overlap closely with the regional Fraser gabbro field, and Cobra shows a wide scatter.
Data are compared with three hypothetical mixing lines between a possible starting magma composition for the Nova intrusions and two model contaminant compositions: Snowys Dam Si-rich metasediment based on the peak data density over the samples analysed; an estimate of average middle continental crust from Rudnick and Gao (Citation2003); and an average composition of Munglinup Gneiss (Smithies et al., 2013; Smithies 2022 pers comm.). The Munglinup Gneiss is a component of the Northern Foreland (), representing extensively reworked Archean basement that underplates the Fraser Zone. Smithies et al. (2013) suggested on the basis of trace-element modelling that the Fraser gabbros hybridised with the melts that produced this unit. Starting assumptions for the isotopic mixing models are listed in . Starting values for a hypothetical Nova starting magma are based on plots of these elements against MgO for orthocumulates from the two intrusions, with values adjusted to match the ratios in the most ‘primitive’ composition observed, a cumulate from the Upper Intrusion Layered Series; this composition has trace-element ratios falling about halfway between ‘primitive mantle’ and typical ‘n-MORB’ source mantle (Pearce, Citation2014).
Both model curves show a reasonable match to the overall trend of the Nova intrusion data and are consistent with the hypothesis that around 90% of the observed variability in ratios of these elements can be explained by up to 25% contamination of a relatively primitive parent magma with either potential contaminant. The range to high Th/Yb within the Nova-Bollinger samples tends to favour contamination with the relatively more Th-enriched Snowys Dam component. Clearly this a non-unique solution given the wide variability of gneiss compositions and lack of constraints on the actual parent magma (or magmas), but it demonstrates that variable country rock contamination of a single plausible parent magma is probably enough to explain the spread in the Nova-Bollinger data. However, the distinct difference is noteworthy between the regional Fraser gabbros, many of which plot within the Th–Nb–Yb mantle array, and the Nova-Bollinger intrusions, indicating that the entire suite is unlikely to have been derived from a common magma source or parent magma composition.
The tectonic setting of the Fraser Zone has been discussed in detail by Smithies et al. (2013) and previously by Condie and Myers (Citation1999), who interpreted the zone as an accreted arc based on trace-element discriminant arguments. This interpretation is now largely superseded on several lines of evidence, including Hf isotopic evidence for non-juvenile components and the considerably updated trace-element geochemistry dataset compiled here (see Smithies et al., 2013, for a summary). Our favoured interpretation, consistent with the conclusions of Smithies et al. (2013) and the tectonic interpretation of Spaggiari et al. (Citation2018), is that the magmas are derived from a major asthenospheric upwelling associated with a slab delamination event, following collision and accretion of the Loongana Arc on the eastern side of the orogen (Spaggiari et al., Citation2020). The Fraser Zone magmas were probably derived from asthenospheric mantle ranging from moderately depleted n-MORB source to eMORB-like, with a superimposed signal of continental crust contamination.
Timing of sulfide melt generation
Several lines of evidence bear on the question of when and where the sulfide melt component of the Nova-Bollinger orebody was generated, some of which have previously been considered (Barnes et al., Citation2022a; Barnes & Robertson, Citation2019; Maier et al., Citation2016; Taranovic et al., Citation2022). Geological evidence and mass-balance considerations show that the sulfide liquid load must have been transported into the Nova Lower Intrusion as a slurry of already contaminated magma, sulfide droplets and olivine. The presence of entrained olivine accounts for the relatively high Ni/Cu ratio of the deposit, whereas the low PGE tenors are best explained if the parent magmas were sulfide-saturated at source, as seems to be a common feature of magmatic Ni–Cu–Co sulfide ores in orogenic belts (Barnes et al., Citation2022a; Lu et al., Citation2019). Based on a very rough mass balance based on resource tonnage and grade and the geometry of the lower intrusion, the sulfide component of the orebody represents a minimum of 20% of the mass of the entire Lower Intrusion, clearly vastly more than can have been dissolved in the magma in what appears geometrically to be a blind (rather than open-ended) intrusion (Taranovic et al., Citation2022). A high proportion of the Fraser gabbro suite appears to be contaminated, implying hybridisation in the deep basement or within the Snowys Dam basin or both, with these options being largely unresolvable by chemistry. On balance, the S isotope data for Nova specifically tends to favour Snowys Dam sources. Given that the propagation of sill-sediment complexes and the flow of magma through them is known to be largely lateral (Magee et al., Citation2016a, Citation2016b), it is likely that much of the sulfide assimilation to form the Nova-Bollinger orebody took place within lateral flow of magma through the Snowys Dam sediments, with sulfide xenomelts being generated potentially tens of kilometres away from the eventual site of entrapment and ore formation (Barnes & Robertson, Citation2019; Yao & Mungall, Citation2022).
Comparison of S isotopes with other mid–lower crust deposits
shows δ34S data from three other deposits emplaced into the mid crust or lower crust—Jinchuan, Savannah and Voisey’s Bay—for comparison. A feature of all these deposits in common with Nova-Bollinger is that they show an approximately normal distribution of values, with 90% of the data falling within a narrow range 2–3‰ wide, although at different median values. At Nova-Bollinger, the range of δ34S is similar to that in the limited number of samples measured from potential S source country rocks. At Voisey’s Bay, the range is much narrower, −4 to +2‰ VCDT, compared with −17 to +19‰ VCDT in the Tasiuyak Gneiss (Ripley & Li, Citation2011). The likely explanation for the tightly clustered distribution of δ34S in this deposit type is mixing and near-homogenisation of sulfides derived from multiple S sources along the flow pathway upstream from the eventual deposition site. This is consistent with the view that ore formation involves transport and deposition (or redeposition) of sulfides at a scale of kilometres, rather than local derivation of S from immediately adjacent wall rocks (Barnes & Robertson, Citation2019; Lesher, Citation2019; Yao & Mungall, Citation2022).
Figure 11. Comparison of δ34S values between Nova-Bollinger and other intrusion-hosted deposits emplaced into the mid-crust or lower crust. Data sources: Jinchuan (Ripley et al., Citation2005); Voisey’s Bay (Ripley et al., Citation1999); Savannah (Le Vaillant et al., Citation2020). Mantle δ34S value from various sources including Labidi et al. (Citation2013, Citation2014) and Seal (Citation2006).
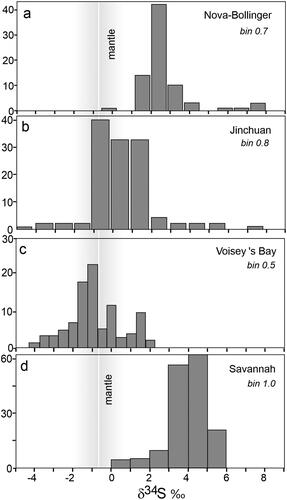
Conclusions
The Nova magmas were variably contaminated at depth prior to emplacement, most likely by typical ‘background’ Snowys Dam metasediments, assimilating up to 30% siliceous crustal material, with contamination by the immediately adjacent garnet gneisses playing a minor role at a local scale. Incorporation of proximal marble gneisses had only a localised effect at a scale of tens of metres, seen in the Sr isotopic compositions of the unusual Bollinger globular ores.
The Nova magmas had isotopic compositions and trace-element contents and ratios indicating derivation from a source somewhat on the depleted side of a PM composition. The regional dataset of Fraser gabbro compositions has trace-element ratios indicating derivation of some magmas from different, more enriched sources towards the eMORB region of the mantle array.
On the data available, the S in the Nova-Bollinger orebodies contains a high crustal component, but it is not possible to fingerprint the S source more precisely. On geological evidence, it is likely that S was derived from sulfidic metapelites and ironstones within the Snowys Dam sequence. No unequivocal trace of a reworked Archean provenance can be seen in the δ33S data, despite the regional isotopic and geochronological indications of this part of the orogen containing significant input from reworked Archean crust (Walker et al., Citation2019). The generally limited range and distribution of S isotope values, coupled with evidence for high R factors in a relatively sulfide-rich, probably blind intrusion, indicate that the ores were introduced as entrained droplets of well-equilibrated sulfide liquid, derived by pre-emplacement country rock assimilation, and transported into the intrusive complex as an emulsion. The presence of local S-rich country rocks is not likely to be a proximity indicator for orebodies. Considerable potential therefore exists for more deposits of similar size and grade to Nova-Bollinger to be discovered within the Fraser Zone.
Acknowledgements
We acknowledge the contributions of David Hammond, Sebastian Staude, Steve Rennick, Paul Polito and Tony Crawford. Mike Verrall assisted with the Tornado XRF mapping system. Sue Golding from the University of Queensland Stable Isotope Geochemistry Laboratory is thanked for assistance with S isotopic analyses; we thank David Bruce from the University of Adelaide MAS for performing the Sr and Sm–Nd isotopic analysis. Roland Maas from the University of Melbourne is thanked for analytical support and insightful discussions. Catherine Spaggiari and Hugh Smithies provided helpful advice on the regional context. Will Smith and an anonymous reviewer provided constructive reviews that considerable improved the final product.
Disclosure statement
No potential conflict of interest was reported by the author(s).
Data availability statement
Full data for S isotope analyses and whole rock trace-element analyses collected in this study are archived and available for free download on the CSIRO Data Access Portal at https://doiorg/10.25919/rcst-9d44.
Additional information
Funding
References
- Barnes, S. J., & Robertson, J. C. (2019). Time scales and length scales in magma flow pathways and the origin of magmatic Ni–Cu–PGE ore deposits. Geoscience Frontiers, 10(1), 77–87. https://doi.org/10.1016/j.gsf.2018.02.006
- Barnes, S. J., Godel, B., Gürer, D., Brenan, J. M., Robertson, J., & Paterson, D. (2013). Sulfide-olivine Fe-Ni exchange and the origin of anomalously Ni-rich magmatic sulfides. Economic Geology, 108(8), 1971–1982. https://doi.org/10.2113/econgeo.108.8.1971
- Barnes, S. J., Mungall, J. E., Le Vaillant, M., Godel, B., Lesher, C. M., Holwell, D. A., Lightfoot, P. C., Krivolutskaya, N. A., & Wei, B. (2017). Sulfide-silicate textures in magmatic Ni–Cu–PGE sulfide ore deposits: Disseminated and net-textured ores. American Mineralogist, 102(3), 473–506. https://doi.org/10.2138/am-2017-5754
- Barnes, S. J., Stanley, C. R., & Taranovic, V. (2022a). Compositions and Ni-Cu-PGE tenors of Nova-Bollinger ores with implications for the origin of Pt anomalies in PGE-poor massive sulfides. Economic Geology. https://doi.org/10.5382/econgeo.4894
- Barnes, S. J., Staude, S., Le Vaillant, M., Pina, R., & Lightfoot, P. C. (2018). Sulfide-silicate textures in magmatic Ni–Cu–PGE sulfide ore deposits: Massive, semi-massive and sulfide-matrix breccia ores. Ore Geology Reviews, 101, 629–651. https://doi.org/10.1016/j.oregeorev.2018.08.011
- Barnes, S. J., Taranovic, V., Miller, J. M., Boyce, G., & Beresford, S. W. (2020a). Sulfide emplacement and migration in the Nova-Bollinger Ni-Cu-Co deposit, Albany-Fraser Orogen, Western Australia. Economic Geology, 115(8), 1749–1776. https://doi.org/10.5382/econgeo.4758
- Barnes, S. J., Taranovic, V., Schoneveld, L. E., Mansur, E. T., Le Vaillant, M., Dare, S. A. S., Staude, S., Evans, N. J., & Blanks, D. (2020b). The occurrence and origin of pentlandite–chalcopyrite–pyrrhotite loop textures in magmatic Ni-Cu sulfide ores. Economic Geology, 115(8), 1777–1798. https://doi.org/10.5382/econgeo.4757
- Barnes, S. J., Williams, M. J., Smithies, R. H., Lowrey, J. R., & Hanski, E. (2021). Trace element contents of mantle-derived magmas through time. Journal of Petrology, 62(6), egab024. https://doi.org/10.1093/petrology/egab024
- Barnes, S. J., Yao, Z-S., Mao, Y. J., Jesus, A. P., Yang, S. H., Taranovic, V., & Maier, W. D. (2022b). Nickel in olivine as an exploration indicator for magmatic Ni–Cu sulfide deposits: a data review and re-evaluation. American Mineralogist, online. https://doi.org/10.2138/AM-2022-8327
- Bathgate, K. (2019). Geochemical and isotopic characterisation of mafic intrusions in the Fraser [thesis]. Zone, Western Australia [unpublished MScUniversity of Western Australia]. https://doi.org/10.26182/hwx6-q022
- Baublys, K. A., Golding, S. D., Young, E., & Kamber, B. S. (2004). Simultaneous determination of delta33S(V-CDT) and delta34S(V-CDT) using masses 48, 49 and 50 on a continuous flow isotope ratio mass spectrometer. Rapid Communications in Mass Spectrometry : RCM, 18(22), 2765–2769. https://doi.org/10.1002/rcm.1681
- Bennett, M., Gollan, M., Staubmann, M., & Bartlett, J. (2014). Motive, means, and opportunity: key factors in the discovery of the Nova-Bollinger magmatic Nickel–Copper sulfide deposits in Western Australia. In K. D. Kelley & H. C. Golden (Eds.), Building exploration capability for the 21st century (vol. 18, pp. 301–320). Society of Economic Geologists. https://doi.org/10.5382/SP.18.15
- Blanks, D. E., Holwell, D. A., Fiorentini, M. L., Moroni, M., Giuliani, A., Tassara, S., González-Jiménez, J. M., Boyce, A. J., & Ferrari, E. (2020). Fluxing of mantle carbon as a physical agent for metallogenic fertilization of the crust. Nature Communications, 11(1), 4342–1798. https://doi.org/10.1038/s41467-020-18157-6
- Chai, G., & Naldrett, A. J. (1992). Characteristics of Ni-Cu-PGE mineralization and genesis of the Jinchuan deposit, northwest China. Economic Geology, 87(6), 1475–1495. https://doi.org/10.2113/gsecongeo.87.6.1475
- Clark, C., Kirkland, C. L., Spaggiari, C. V., Oorschot, C., Wingate, M. T. D., & Taylor, R. J. (2014). Proterozoic granulite formation driven by mafic magmatism; an example from the Fraser Range Metamorphics, Western Australia. Precambrian Research, 240(1), 1–21. https://doi.org/10.1016/j.precamres.2013.07.024
- Condie, K. C., & Myers, J. S. (1999). Mesoproterozoic Fraser Complex; geochemical evidence for multiple subduction-related sources of lower crustal rocks in the Albany-Fraser Orogen, Western Australia. Australian Journal of Earth Sciences, 46(6), 875–882. https://doi.org/10.1046/j.1440-0952.1999.00750.x
- Ding, T., Valkiers, S., Kipphardt, H., De Bièvre, P., Taylor, P. D. P., Gonfiantini, R., & Krouse, R. (2001). Calibrated sulfur isotope abundance ratios of three IAEA sulfur isotope reference materials and V-CDT with a reassessment of the atomic weight of sulfur. Geochimica et Cosmochimica Acta, 65(15), 2433–2437. https://doi.org/10.1016/S0016-7037(01)00611-1
- Ding, X., Li, C., Ripley, E. M., Rossell, D., & Kamo, S. (2010). The Eagle and East Eagle sulfide ore-bearing mafic-ultramafic intrusions in the Midcontinent Rift System, upper Michigan: Geochronology and petrologic evolution. Geochemistry, Geophysics, Geosystems, 11(3), n/a–n/a. https://doi.org/10.1029/2009GC002546
- Ding, X., Ripley, E. M., Shirey, S. B., & Li, C. (2012). Os, Nd, O and S isotope constraints on country rock contamination in the conduit-related Eagle Cu–Ni–(PGE) deposit, Midcontinent Rift System, Upper Michigan. Geochimica et Cosmochimica Acta, 89, 10–30. https://doi.org/10.1016/j.gca.2012.04.029
- Fletcher, I. R., Myers, J. S., & Ahmat, A. L. (1991). Isotopic evidence on the age and origin of the Fraser Complex, Western Australia; a sample of mid-Proterozoic lower crust. Chemical Geology. Chemical Geology: Isotope Geoscience Section, 87(3–4), 197–216. https://doi.org/10.1016/0168-9622(91)90021-N
- Gao, J., & Zhou, M. (2013). Magma mixing in the genesis of the Kalatongke dioritic intrusion; implications for the tectonic switch from subduction to post-collision, Chinese Altay, NW China. Lithos, 162–163, 236–250. https://doi.org/10.1016/j.lithos.2013.01.007
- Glasson, K., Johnson, T., Kirkland, C., Gardiner, N. J., Clark, C., Blereau, E., Hartnady, M., Spaggiari, C. V., & Smithies, H. (2019). A window into an ancient backarc? The magmatic and metamorphic history of the Fraser Zone, Western Australia. Precambrian Research, 323, 55–69. https://doi.org/10.1016/j.precamres.2019.01.011
- Goldstein, S. L., O’Nions, R. K., & Hamilton, P. J. (1984). A Sm–Nd study of atmospheric dusts and particulates from major river systems. Earth and Planetary Science Letters, 70, 221–236. https://doi.org/10.1016/0012-821X(84)90007-4
- Jacobsen, S. B., & Wasserburg, G. J. (1978). Interpretation of Nd, Sr and Pb isotope data from Archean migmatites in Lofoten-Vesterålen, Norway. Earth and Planetary Science Letters, 41(3), 245–253. https://doi.org/10.1016/0012-821X(78)90181-4
- Kamber, B. S., Greig, A., Schoenberg, R., & Collerson, K. D. (2003). A refined solution to Earth’s hidden niobium: implications for evolution of continental crust and mode of core formation. Precambrian Research, 126(3–4), 289–308. https://doi.org/10.1016/S0301-9268(03)00100-1
- Kang, Z., Qin, K., Mao, Y., Tang, D., & Yao, Z. (2020). The formation of a magmatic Cu–Ni sulfide deposit in mafic intrusions at the Kalatongke, NW China; insights from amphibole mineralogy and composition. Lithos, 352–353, 105317. https://doi.org/10.1016/j.lithos.2019.105317
- Kirkland, C. L., Spaggiari, C. V., Pawley, M. J., Wingate, M. T. D., Smithies, R. H., Howard, H. M., Tyler, I. M., Belousova, E. A., & Poujol, M. (2011a). On the edge: U–Pb, Lu–Hf, and Sm–Nd data suggests reworking of the Yilgarn craton margin during formation of the Albany-Fraser Orogen. Precambrian Research, 187(3–4), 223–247. https://doi.org/10.1016/j.precamres.2011.03.002
- Kirkland, C. L., Spaggiari, C. V., Wingate, M. T. D., Smithies, R. H., Belousova, E. A., Murphy, R., & Pawley, M. (2011b). Inferences on crust–mantle interaction from Lu–Hf isotopes: a case study from the Albany-Fraser Orogen. Geological Survey of Western Australia Record, 2011/12, p. 25.
- Kirkland, C. L., Spaggiari, C. V., Smithies, R. H., Wingate, M. T. D., Belousova, E. A., Gréau, Y., Sweetapple, M. T., Watkins, R., Tessalina, S., & Creaser, R. (2015). The affinity of Archean crust on the Yilgarn–Albany-Fraser Orogen boundary: Implications for gold mineralisation in the Tropicana Zone. Precambrian Research, 266, 260–281. https://doi.org/10.1016/j.precamres.2015.05.023
- Labidi, J., Cartigny, P., & Moreira, M. (2013). Non-chondritic sulphur isotope composition of the terrestrial mantle. Nature, 501(7466), 208–211. https://doi.org/10.1038/nature12490
- Labidi, J., Cartigny, P., Hamelin, C., Moreira, M., & Dosso, L. (2014). Sulfur isotope budget (32S, 33S, 34S and 36S) in Pacific–Antarctic ridge basalts: A record of mantle source heterogeneity and hydrothermal sulfide assimilation. Geochimica et Cosmochimica Acta, 133, 47–67. https://doi.org/10.1016/j.gca.2014.02.023
- Latypov, R. (2015). Basal reversals in Mafic Sills and layered intrusions. In B. Charlier, O. Namur, R. Latypov, & C. Tegner (Eds.), Layered intrusions (pp. 259–293). Springer Netherlands.
- Le Vaillant, M., Barnes, S. J., Mole, D. R., Fiorentini, M. L., LaFlamme, C. K., Denyszyn, S., Austin, J., Patterson, B., Godel, B., Neaud, A., Hicks, J., Shaw-Stuart, A., & Mao, Y. J. (2020). Multidisciplinary study of a complex magmatic system: The Savannah Ni-Cu-Co Camp, Western Australia. Ore Geology Reviews, 117, 103292. https://doi.org/10.1016/j.oregeorev.2019.103292
- Lesher, C. M. (2019). Up, down, or sideways: emplacement of magmatic Fe–Ni–Cu–PGE sulfide melts in large igneous provinces. Canadian Journal of Earth Sciences, 56(7), 756–773. https://doi.org/10.1139/cjes-2018-0177
- Lesher, C. M., & Burnham, O. M. (2001). Multicomponent elemental and isotopic mixing in Ni–Cu–(PGE) ores at Kambalda, Western Australia. Canadian Mineralogist, 39, 421–446.
- Li, C., & Naldrett, A. J. (1999). Geology and petrology of the Voisey’s Bay intrusion: Reaction of olivine with sulfide and silicate liquids. Lithos, 47(1–2), 1–31. https://doi.org/10.1016/S0024-4937(99)00005-5
- Li, C., & Ripley, E. M. (2009). Sulfur contents at sulfide-liquid or anhydrite saturation in silicate melts: empirical equations and example applications. Economic Geology, 104(3), 405–412. https://doi.org/10.2113/gsecongeo.104.3.405
- Li, C., & Ripley, E. M. (2011). The giant Jinchuan Ni-Cu-(PGE) deposit; tectonic setting, magma evolution, ore genesis, and exploration implications. Reviews in Economic Geology, 17, 163–180. https://doi.org/10.5382/Rev.17.06
- Li, C., Zhang, M., Fu, P., Qian, Z. Z., Hu, P., & Ripley, E. M. (2012). The Kalatongke magmatic Ni–Cu deposits in the Central Asian Orogenic Belt, NW China: Product of slab window magmatism? Mineralium Deposita, 47(1–2), 51–67. https://doi.org/10.1007/s00126-011-0354-7
- Li, C., Zhang, Z., Li, W., Wang, Y., Sun, T., & Ripley, E. M. (2015). Geochronology, petrology and Hf–S isotope geochemistry of the newly-discovered Xiarihamu magmatic Ni–Cu sulfide deposit in the Qinghai-Tibet Plateau, western China. Lithos, 216-217, 224–240. https://doi.org/10.1016/j.lithos.2015.01.003
- Lightfoot, P. C., Keays, R. R., Evans-Lamswood, D., & Wheeler, R. (2012). S saturation history of Nain plutonic suite mafic intrusions; origin of the Voisey’s Bay Ni–Cu–Co sulfide deposit, Labrador, Canada. Mineralium Deposita, 47(1–2), 23–50. https://doi.org/10.1007/s00126-011-0347-6
- Lu, Y., Lesher, C. M., & Deng, J. (2019). Geochemistry and genesis of magmatic Ni–Cu–(PGE) and PGE–(Cu)–(Ni) deposits in China. Ore Geology Reviews, 107, 863–887. https://doi.org/10.1016/j.oregeorev.2019.03.024
- Maas, R., Grew, E. S., & Carson, C. J. (2015). On the sources of Early Cambrian pegmatites with boron and beryllium minerals in the Larsemann Hills, Prydz Bay, Antarctica. The Canadian Mineralogist, 53(2), 249–272. https://doi.org/10.3749/canmin.1400081
- Magee, C., Muirhead, J. D., Karvelas, A., Holford, S. P., Jackson, C. A. L., Bastow, I. D., Schofield, N., Stevenson, C. T. E., McLean, C., McCarthy, W., & Shtukert, O. (2016a). Lateral magma flow in mafic sill complexes. Geosphere, 12(3), 809–841. https://doi.org/10.1130/GES01256.1
- Magee, C., O’Driscoll, B., Petronis, M. S., & Stevenson, C. T. E. (2016b). Three-dimensional magma flow dynamics within subvolcanic sheet intrusions. Geosphere, 12(3), 842–866. https://doi.org/10.1130/GES01270.1
- Maier, W. D., Smithies, R. H., Spaggiari, C. V., Barnes, S. J., Kirkland, C. L., Yang, S., Lahaye, Y., Kiddie, O., & MacRae, C. M. (2016). Petrogenesis and Ni–Cu sulphide potential of mafic-ultramafic rocks in the Mesoproterozoic Fraser Zone within the Albany-Fraser Orogen, Western Australia. Precambrian Research, 281, 27–46. https://doi.org/10.1016/j.precamres.2016.05.004
- Mao, Y. J., Barnes, S. J., Godel, B., Schoneveld, L. E., Qin, K. Z., Tang, D. M., Williams, M. J., & Kang, Z. (2022). Sulfide ore formation of the Kalatongke Ni-Cu deposit as illustrated by sulfide textures. Economic Geology. https://doi.org/10.5382/econgeo.4914
- Mao, Y-J., Barnes, S. J., Qin, K. Z., Tang, D. M., Martin, L., Su, B. X., & Evans, N. J. (2019). Sector zoned orthopyroxene, olivine oxygen isotope and trace elemental stratigraphic variations in the Huangshanxi orthopyroxene-rich Ni–Cu deposit, northwest China: implications for rapid orthopyroxene growth induced by silica assimilation. Contribution to Mineralogy and Petrology, 174, 33. https://doi.org/10.1007/s00410-019-1574-6
- Morrison, J. L., Kirkland, C. L., Fiorentini, M., Beresford, S., & Polito, P. (2022). An apatite to unravel petrogenic processes of the Nova-Bollinger Ni–Cu magmatic sulfide deposit, Western Australia. Precambrian Research, 369, 106524. https://doi.org/10.1016/j.precamres.2021.106524
- Mota-e-Silva, J., Filho, C. F. F., & Giustina, M. E. S. D. (2013). The Limoeiro Deposit: Ni-Cu-PGE sulfide mineralization hosted within an ultramafic tubular magma conduit in the Borborema Province, Northeastern Brazil. Economic Geology, 108(7), 1753–1771. https://doi.org/10.2113/econgeo.108.7.1753
- Mungall, J. E., Jenkins, M. C., Robb, S. L., Yao, Z., & Brenan, J. M. (2020). Upgrading of magmatic sulfides, revisited. Economic Geology, 115(8), 1827–1833. https://doi.org/10.5382/econgeo.4775
- Naldrett, A. J., Keats, H., Sparkes, K., & Moore, R. (1996). Geology of the Voisey’s Bay Ni–Cu–Co deposit, Labrador, Canada. Exploration and Mining Geology, 5, 69–179.
- Parker, P., Bartlett, J., Hodges, K., & Thompson, A. (2017). Nova-Bollinger Ni–Cu–Co sulfide deposit. In G. N. Phillips (Ed.), Australian Ore Deposits, (Monograph 32, pp. 139–142). The Australasian Institute of Mining and Metallurgy.
- Pearce, J. A. (2008). Geochemical fingerprinting of oceanic basalts with applications to ophiolite classification and the search for Archean oceanic crust. Lithos, 100(1–4), 14–48. https://doi.org/10.1016/j.lithos.2007.06.016
- Pearce, J. A. (2014). Geochemical fingerprinting of the Earth’s oldest rocks. Geology, 42(2), 175–176. https://doi.org/10.1130/focus022014.1
- Qian, Z., Wang, J., Jiang, C., Jiao, J., He, K., Yan, H., & Sun, T. (2009). Geochemistry characters of platinum-group elements and its significances on the process of mineralization in the Kalatongke Cu–Ni sulfide deposit, Xinjiang China. Acta Petrologica Sinica, 25(4), 832–844.
- Ripley, E. M., & Li, C. (2003). Sulfur isotope exchange and metal enrichment in the formation of magmatic Cu–Ni–(PGE) deposits. Economic Geology, 98(1), 635–641. https://doi.org/10.2113/gsecongeo.98.3.635
- Ripley, E. M., & Li, C. (2011). A review of conduit-related Ni–Cu–(PGE) sulfide mineralization at the Voisey’s Bay Deposit, Labrador, and the Eagle Deposit, northern Michigan. In C. Li & E. M. Ripley (Eds.), Magmatic Ni–Cu and PGE deposits: geology, geochemistry and genesis (Reviews in Economic Geology 17, pp. 181–197). Society of Economic Geologists.
- Ripley, E. M., & Li, C. (2013). Sulfide saturation in mafic magmas; is external sulfur required for magmatic Ni-Cu-(PGE) ore genesis? Economic Geology, 108(1), 45–58. https://doi.org/10.2113/econgeo.108.1.45
- Ripley, E. M., Park, Y-R., Li, C., & Naldrett, A. J. (1999). Sulfur and oxygen isotopic evidence of country rock contamination in the Voisey’s Bay Ni–Cu–Co deposit, Labrador, Canada. Lithos, 47(1–2), 53–68. https://doi.org/10.1016/S0024-4937(99)00007-9
- Ripley, E. M., Sarkar, A., & Li, C. S. (2005). Mineralogic and stable isotope studies of hydrothermal alteration at the Jinchuan Ni–Cu Deposit, China. Economic Geology, 100(7), 1349–1361. https://doi.org/10.2113/gsecongeo.100.7.1349
- Rudnick, R. L., & Gao, S. (2003). Composition of the continental crust. In R. L. Rudnick, H. D. Holland, & K. K. Turekian (Eds.), Treatise on Geochemistry (Vol. 3, pp. 1–64). Elsevier.
- Saumur, B. M., & Cruden, A. R. (2015). On the emplacement of the Voisey’s Bay intrusion (Labrador, Canada). Geological Society of America Bulletin, 128, 147–168. https://doi.org/10.1130/B31240.1
- Seal, R. R. (2006). Sulfur isotope geochemistry of sulfide minerals. Reviews in Mineralogy and Geochemistry, 61(1), 633–663. https://doi.org/10.2138/rmg.2006.61.12
- Seat, Z., Beresford, S. W., Grguric, B. A., Gee, M. A. M., & Grassineau, N. V. (2009). Reevaluation of the role of external sulfur addition in the genesis of Ni-Cu-PGE deposits; evidence from the Nebo-Babel Ni-Cu-PGE deposit, west Musgrave, Western Australia. Economic Geology, 104(4), 521–538. https://doi.org/10.2113/gsecongeo.104.4.521
- Sippl, C., Brisbout, L. J., Spaggiari, C. V., Kennett, B. L. N., Tkalčić, H., Murdie, R., & Gessner, K. (2017). Crustal structure and tectonic evolution scenarios for the east Albany-Fraser Orogen, Western Australia, from passive seismic and gravity anomaly data. Precambrian Research, 296, 78–92. https://doi.org/10.1016/j.precamres.2017.04.041
- Smithies, R. H., Spaggiari, C. V., Kirkland, C. L., Howard, H. M., & Maier, W. D. (2013). Melting, mixing, and emplacement; evolution of the Fraser Zone, Albany-Fraser Orogen. GSWA Record 2013/5. Geological Survey of Western Australia.
- Song, X-Y., Danyushevsky, L. V., Keays, R. R., Chen, L-M., Wang, Y-S., Tian, Y-L., & Xiao, J-F. (2012). Structural, lithological, and geochemical constraints on the dynamic magma plumbing system of the Jinchuan Ni–Cu sulfide deposit, NW China. Mineralium Deposita, 47(3), 277–297. https://doi.org/10.1007/s00126-011-0370-7
- Song, X., Yi, J., Chen, L., She, Y., Liu, C., Dang, X., Yang, Q., & Wu, S. (2016). The giant Xiarihamu Ni–Co sulfide deposit in the East Kunlun orogenic belt, northern Tibet Plateau, China. Economic Geology, 111(1), 29–55. https://doi.org/10.2113/econgeo.111.1.29
- Spaggiari, C. V., Kirkland, C. L., Smithies, R. H., Wingate, M. T. D., & Belousova, E. (2015). Transformation of an Archean craton margin during Proterozoic basin formation and magmatism: the Albany-Fraser Orogen, Western Australia. Precambrian Research, 266, 440–466. https://doi.org/10.1016/j.precamres.2015.05.036
- Spaggiari, C. V., Kirkland, C., Pawley, M. J., Smithies, R. H., Wingate, M. T. D., Doyle, M. G., Blenkinsop, T. G., Clark, C., Oorschot, C. W., Fox, L. J., & Savage, J. (2011). The geology of the East Albany-Fraser Orogen – a field guide. Geological Survey of Western Australia Record, vol. 2011/23, p. 97.
- Spaggiari, C. V., Smithies, R. H., Kirkland, C. L., Wingate, M. T. D., England, R. N., & Lu, Y-J. (2018). Buried but preserved; the Proterozoic Arubiddy Ophiolite, Madura Province, Western Australia. Precambrian Research, 317, 137–158. https://doi.org/10.1016/j.precamres.2018.08.025
- Spaggiari, C. V., Smithies, R. H., Kirkland, C. L., Wingate, M. T. D., England, R. N., & Lu, Y-J. (2020). Stratigraphic and co-funded drilling of the Eucla Basement – the Proterozoic geology beneath the Nullarbor Plain. Geological Survey of Western Australia, Report, 204, 147.
- Taranovic, V., Barnes, S. J., Beresford, S. W., Williams, M. J., MacRae, C., & Schoneveld, L. E. (2022). Nova-Bollinger Ni–Cu sulfide ore deposits, Fraser Zone, Western Australia. Economic Geology, 117(2), 455–484. https://doi.org/10.5382/econgeo.4873
- Taranovic, V., Ripley, E. M., Li, C., & Rossell, D. (2015). Petrogenesis of the Ni–Cu–PGE sulfide-bearing Tamarack Intrusive Complex, Midcontinent Rift System, Minnesota. Lithos, 212–215, 16–31. https://doi.org/10.1016/j.lithos.2014.10.012
- Taranovic, V., Ripley, E. M., Li, C., & Rossell, D. (2016). Chalcophile element (Ni, Cu, PGE, and Au) variations in the Tamarack magmatic sulfide deposit in the Midcontinent Rift system; implications for dynamic ore-forming processes. Mineralium Deposita, 51(7), 937–951. https://doi.org/10.1007/s00126-016-0643-2
- Torres-Rodriguez, N., Barnes, S. J., Taranovic, V., Pearce, M. B., Verrall, M., & Schoneveld, L. E. (2021). Reaction coronas at olivine–plagioclase contacts in host rocks from the Nova-Bollinger Ni–Cu–Co deposit, Albany-Fraser Orogen, Western Australia: Evidence of a magmatic to metamorphic continuum. Journal of Petrology, 62(9), 1–24. https://doi.org/10.1093/petrology/egab055
- Walker, A. T., Evans, K. A., Kirkland, C. L., & Polito, P. A. (2022). Multiple modes of sulphur cycling within a mineralised orogen: A case study from the Fraser Zone, Western Australia, Lithos. Lithos, 408–409, 106536. https://doi.org/10.1016/j.lithos.2021.106536
- Walker, A. T., Evans, K. A., Kirkland, C. L., Martin, L., Kiddie, O. C., & Spaggiari, C. V. (2019). Tracking mineralisation with in situ multiple sulphur isotopes: A case study from the Fraser Zone, Western Australia. Precambrian Research, 332, 105379. https://doi.org/10.1016/j.precamres.2019.105379
- Yao, Z., & Mungall, J. E. (2022). Transport and deposition of immiscible sulfide liquid during lateral magma flow. Earth-Science Reviews, 227, 103964. https://doi.org/10.1016/j.earscirev.2022.103964
- Yaxley, G. M., Kamenetsky, V. S., Nichols, G. T., Maas, R., Belousova, E., Rosenthal, A., & Norman, M. (2013). The discovery of kimberlites in Antarctica extends the vast Gondwanan Cretaceous province. Nature Communications, 4, 2921. https://doi.org/10.1038/ncomms3921