Abstract
The eastern part of the northern margin of the North China Craton is a key metal metallogenic province in China, hosting many important rare metal and rare earth element (REE) deposits and having great resource potential. This study investigated the Saima deposit, a niobium (Nb) and tantalum (Ta) deposit and the Baerzhe deposit hosting zirconium (Zr), Nb and REEs. Based on previous LA-ICP-MS zircon U–Pb dating results obtained by the authors’ team, this study compared the whole-rock geochemistry and whole-rock Sr–Nd–Pb isotopes of an orebody with its surrounding rocks. The geochemical characteristics of both deposits are used to systematically review the evolution of the alkaline magma and the differences in the physical and chemical conditions for enrichment and mineralisation of late-stage rare elements and REEs such as Nb, Ta and Zr. Comparing the mineralisation of the Saima and Baerzhe deposits, the alkaline magmatic evolution plays a crucial role in the enrichment of rare-earth elements, which are generally formed in the late stage of alkaline magmatic evolution.
KEY POINTS
The Saima deposit, which has a metallogenic age of 224.40 ± 6.10 Ma, formed in nepheline syenites with high alkali (Na2O + K2O) and low MgO and CaO contents, with high TFeO/MgO ratios. The A-type granites formed in an extensional tectonic setting after the closure of the Paleo-Asian Ocean.
The Baerzhe deposit, which has a metallogenic age of 125.2–122.15 Ma, formed in A-type alkali granites (orebody and surrounding rocks) that have high alkali (Na2O + K2O) and low MgO and CaO contents with high TFeO/MgO ratios, strong uranium (U) depletion and typical V-shaped distribution of REE. The mineralisation is controlled by melt–fluid interactions in the later stage of highly evolved magmas.
The enrichment and mineralisation of rare elements and REEs in the eastern part of the north margin of the North China Craton are mainly associated with the high degree of evolution of alkaline magmas.
Introduction
With an increase in the value and importance of strategic key metals, research on the metallogenic regularity of three types of rare deposits (i.e. rare element deposits, REE deposits and scattered element deposits) has received extensive attention worldwide. Early rare earth resource potential evaluation included the first quantitative evaluation project of USGS in the 1990s and the subsequent global mineral resource evaluation project, EURARE and ASTER programs in Europe and the potential evaluation of important mineral resources in China (Bagas et al., Citation2017; Xiao et al., Citation2017). At present, the known rare earth minerals are mainly concentrated in China, the United States, India, Australia, the Commonwealth of Independent States, South Africa and a few other countries. The United States is an important global source of rare earth resources. It is generally believed that China’s endogenous rare metal deposits and REE deposits are roughly divided into alkaline, granite, granite pegmatite, skarn (or ribbon rock), quartz vein, leptite, migmatite and acid volcanic types. Among them, alkaline rock type, granite type and granite pegmatite type deposits are the most important and most abundant, formed from the Proterozoic to Mesozoic (Deng et al., Citation2018; Mao et al., Citation2019; Wang et al., Citation2012; Zhai et al., Citation2019; Zhang et al., Citation2019, Citation2022, Citation2023). In China, a key zone for rare metal and REE deposits is the eastern part of the northern margin of the North China Craton. However, owing to the small scale of the deposits and the low level of research, only preliminary research on the geological characteristics, genetic types and exploration potential has been undertaken (Ju et al., Citation2019; Li et al., Citation2022; B. Wu et al., Citation2016, Citation2018; F. Y. Wu et al., Citation2000; Yang, Giester, et al., 2012; Zhang et al., Citation2023; Zhu et al., Citation2016), with no systematic studies on the genesis of deposits and the sources of metallogenic materials. This study investigated the Saima and Baerzhe deposits in the eastern part of the northern margin of the North China Craton, aiming to explore diagenesis and mineralisation, and define its tectonic setting using petrology, element geochemistry and isotopic chronology.
Regional geological setting
Rare and REE mineral resources in the eastern part of the northern margin of the North China Craton (also referred to as the study area) are mainly distributed on plate margins or at junctions of structures with different ages. They are controlled by large regional faults, such as the Tanlu and Suolun–Xilamulun–Changchun–Yanji fault zones, that experienced Sibaoian, Indosinian and Yanshanian tectonic–magmatic events. The rare and REE minerals are closely associated with Mesoproterozoic and Mesozoic magmatic rocks (F. Y. Wu et al., Citation2000; Yang et al., Citation2007, Citation2012). Eight rare metal and REE deposits and another 10 ore occurrences have been discovered mainly in the Liaoning Province and eastern Inner Mongolia (). This study investigated the most typical deposits in the study area, i.e. the Baerzhe and Saima deposits, compared the geochemical characteristics of the orebodies and host rocks, and assessed the role of alkaline magmatism on the enrichment and mineralisation of rare elements and REEs.
Saima deposit
The Saima deposit lies at the intersection of the Taizihe-Hunjiang sag and the Yingkou-Kuandian uplift in the Liaodong block. The exposed strata in the deposit include the Paleo-proterozoic Gaixian and Dashiqiao formations, the Neo-proterozoic Xihe Group and the Cambrian, Ordovician and Jurassic strata (Ma et al., Citation2023; Y. Wu et al., Citation2023). The mining area features intense magmatism that can be divided into the Proterozoic and Mesozoic tectonomagmatic cycles. The alkaline intrusions associated with mineralisation are in the core of the Mesozoic tectonomagmatic zone and are mostly exposed as dykes. Their rock types are dominated by nepheline syenites, which are rich in green, grass green and greyish-brown aegirine and pegmatite, which formed in the late stage with a higher degree of alteration. The complex tectonism includes W–E-, nearly S–N-, NE- and NW-trending fault structures, of which the NE-trending faults are ore-controlling structures (). The main body of the Saima alkaline rock mass has been metamorphosed (Y. Wu et al., Citation2023) with alteration types including microclinisation, nephelinisation, natrolitisation, cancrinitisation and carbonatisation. Zoning is not significant, and the Nb–Ta mineralisation is closely associated with nephelinisation and cancrinitisation. Zirconium-bearing minerals include zircon in phonite, potassium zircon in nepheline syenite, and potassium zircon, sodium zircon and heterolite in isonite syenite (B. Wu et al., Citation2015, Citation2016).
Figure 2. Geological map of Saima uranium–thorium–niobium polymetallic deposit (modified after Ju et al., Citation2019).
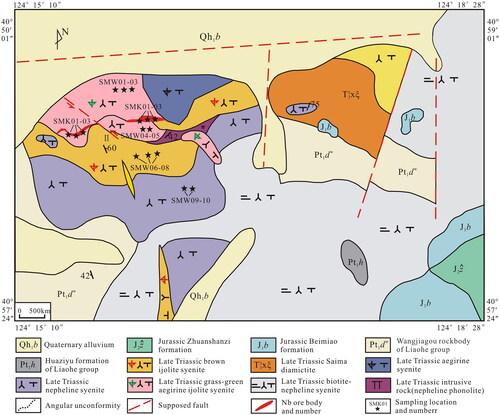
Presently, eight orebodies occur in the greyish-brown–grass-green nepheline syenites. No. I orebody is 500 m long and 6 m wide, and No. II orebody is 160 m long and 18 m wide. The orebodies have parallel stratiform and lensoid forms up to 40 m thick (), with dip directions of 45–115° and dip angles of 25–75°. The orebodies gradually transition into surrounding rocks, without obvious boundaries. The ore minerals mainly include columbite–tantalite, fergusonite and brocenite that have high Nb and low Ta contents. Gangue minerals include nepheline, aegirine, rutile and pyrite, with Nb content of generally 0.03–0.06 wt% and Ce content of generally 0.1 wt%.
The nepheline syenites (orebody) are composed of orthoclase, nepheline, biotite and aegirine. Orthoclase (50 vol%) is hypidiomorphic tabular or anhedral granular, with a particle size of 1.06–9.1 mm, with carlsbad twinning and some nepheline inclusions. Nepheline (30 vol%) is hypidiomorphic-anhedral tabular and columnar, with a particle size of 0.3–7.5 mm. Biotite (10 vol%) is hypidiomorphic tabular, with a particle size of 0.6–3.5 mm and some metasomatised by nepheline. Aegirine (7 vol%) is idiomorphic-hypidiomorphic tabular, with a long axis of 0.3–2 mm. Other components include arfvedsonite (3 vol%) ().
Figure 3. Representative outcrop-pictures and photomicrographs of the ore-forming rocks in Saima niobium deposit. (a) Nepheline syenite; (b) aegirine syenite (containing niobium and tantalum); (c) aegirine syenite; and (d) aegirine syenite. Mineral abbreviations: Aeg, aegirine; Bt, biotite; Kfs, K-feldspar; Ne, nepheline.
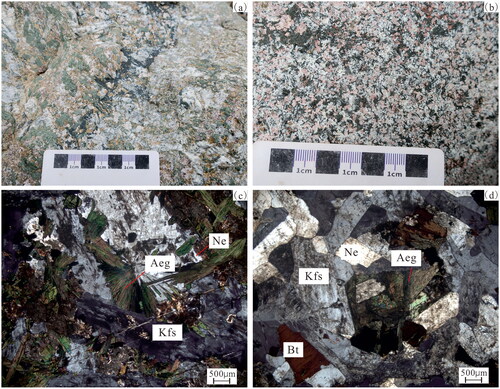
Baerzhe deposit
The Baerzhe deposit, located in the eastern part of the southern Da Hinggan Mountains, lies in the composite part of the eastern wing of the Mongolian arc structure and the NNE-trending tectonic zone of the Da Hinggan Mountains on the northern margin of the North China Continent. This deposit is both a super-large REE–Zr–Nb–Ta–Be polymetallic deposit and a large U–Th deposit (Su et al., Citation2021). The mining area of the Baerzhe deposit has simple strata, only including the Baiyingaolao and Meletu formations. Magmatic rocks mostly occur in groups and belts, with limited outcrop area (M. Wu et al., Citation2021), are mainly distributed in the axis and two wings of anticlines, and occur as small stocks and dykes. The lithologies include alkali granites, granite porphyry, monzonite porphyry, diorite porphyrite and andesitic porphyrite. The ore-hosting rock is dominated by alkali granite, with an outcrop area of about 0.35 km2 with two discontinuous exposed rock masses in the east and west with an area of 0.24 km2 and 0.11 km2, respectively. Drilling has shown that the two rock masses are connected at depth. The alkali granites show significant zoning in vertical and horizontal directions. In the horizontal direction, they are divided into three lithofacies zones from the edge to the centre: a miarolitic riebeckite granite, a pegmatoidal granite and a strongly altered riebeckite granite. In the vertical direction, they are divided into six lithofacies zones from top to bottom: a miarolitic riebeckite granite, a pegmatoidal granite, a strongly altered riebeckite granite, a moderately altered riebeckite granite, the weakly altered porphyritic riebeckite granite and a porphyritic riebeckite granite. The surrounding rocks include alkaline rhyolitic crystal and lithic tuffs and intermediate acid pyroclastic rocks of the Upper Jurassic Baiyingaolao Formation (). The alkaline granite is divided into 801 and 802, of which 801 is alkaline granite and Zr–Nb–REE ore-forming rock, and 802 is a porphyritic alkaline granite with local silicification and sodium alteration, and Nb and Y mineralisation (Yang et al., Citation2011).
Figure 4. Geological map of the Baerzhe rare earth deposit (modified after Yang, Sun, et al., Citation2012).
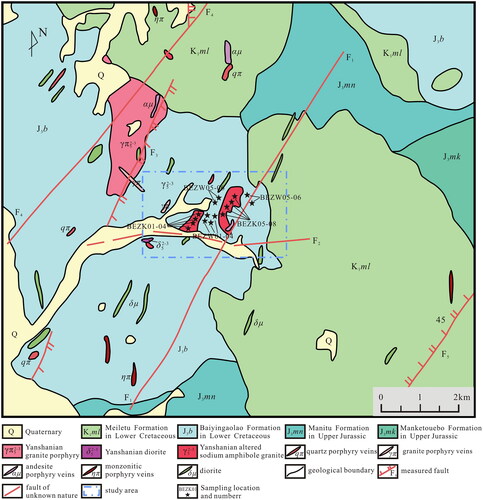
Petrological characteristics
The nepheline syenites (orebodies) in the Saima deposit have a high degree of idiomorphism and a complete crystal form under a microscope. It is composed of orthoclase, nepheline, biotite, and aegirine. Orthoclase (Or) in the nepheline syenites, which is hypidiomorphic tabular or anhedral granular, with a particle size of 1.06–9.1 mm and contains carlsbad twin and some nepheline inclusions, accounts for ∼50% of the total nepheline syenites. Nepheline (Ne) in the nepheline syenites, which is hypidiomorphic-anhedral tabular and columnar, with a particle size of 0.3–7.5 mm, accounts for 30% of the total nepheline syenites. Biotite (Bt) in the nepheline syenites, which is hypidiomorphic tabular, with a particle size of 0.6–3.5 mm, accounts for 10% of the total nepheline syenites, with some metasomatised by nepheline. Aegirine in the nepheline syenites, which is idiomorphic-hypidiomorphic tabular with a long axis of 0.3–2 mm, accounts for 7% of the total nepheline syenites. The other components are arfvedsonite (Arf), which accounts for 3% of the total nepheline syenites ().
The rock masses associated with the mineralisation of the Baerzhe deposit are greyish-white alkali granites with medium–fine-grained granitic textures (pegmatitic or graphic textures locally) and miarolitic structures. The main minerals of the rock masses include perthite (50–60 vol%), quartz (30–35 vol%), albite (10–15 vol%), aegirine (2–7 vol%) and albite oligoclase (1–3 vol%), and their accessory minerals include magnetite and hematite (1–3 vol%) ().
Figure 5. Representative outcrop-pictures and photomicrographs of the ore-forming rocks in Baerzhe deposit. (a) Alkali feldspar granite; (b) alkali feldspar granite (ore); (c) alkali feldspar granite; (d) alkali feldspar granite; (e) alkali feldspar granite (hornblende is metasomatised by zircon); and (f) alkali feldspar granite.
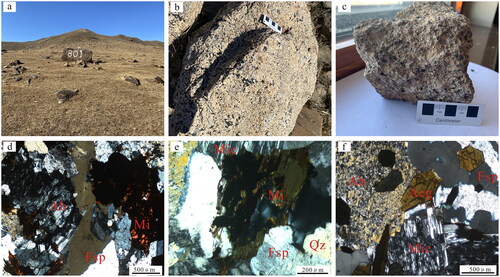
Testing and analysis results
Analysis methods
Whole-rock sEs and trace elements was completed at the Element Geochemistry Laboratory under the Institute of Earth Sciences of China University of Geosciences (Beijing). The main element content was determined using a Prodigy ICP-OES spectrometer produced by Leeman Labs Inc. For some low-content elements, the K, P, Ti and Mn contents were verified in an acid-soluble solution using an Agilent 7500a ICP-MS produced by Agilent Technologies Inc. The contents of REEs and trace elements were also determined using the Agilent 7500a ICP-MS. During the analysis, the standard solutions Std-1, Std-2 and Std-4 prepared by the Equipment Laboratory of the American Bureau of Standards were used as the external standard samples, and Rh added at a fixed proportion as the internal standard samples.
The chemical separation and testing of Sr, Nd and Pb isotopes were completed at the laboratory of Beijing Createch Testing Technology Co., Ltd using a Neptune Plus MC-ICP-MS produced by ThermoFisher. The instrumental mass fractionation correction for Sr, Nd and Pb isotopes was carried out using exponential equations 88Sr/86Sr = 8.375209,146Nd/144Nd = 0.7219 and 203Tl/205Tl = 2.3889, respectively.
Zircon U–Pb chronology
Saima deposit
Zircons have Th/U values of 0.5–1.0 (>0.1; Supplemental data, Table S1; Ju et al., Citation2019), indicating a magmatic origin (Hoskin & Schaltegger, Citation2003). On the 206Pb/238U–207Pb/235U concordia diagram, all data plot on or near the concordant curve (), and 16 analyses gave 206Pb/238U ages of 233–200 Ma, with a weighted average age of 224.40 ± 6.10 Ma (MSWD = 8.0).
Baerzhe deposit
Wang and Zhao (Citation1997) and Jahn et al. (Citation2001) determined the Rb–Sr isochron age of the ore-bearing alkali granites at 125.2 ± 2 Ma and 122 ± 5 Ma, respectively. Qiu et al. (Citation2014) determined a zircon U–Pb age of alkali granite (orebody) of 122.7 ± 1.8 Ma using LA-ICP-MS. Yang et al. (Citation2014) determined U–Pb ages of magmatic zircons and hydrothermal zircons in alkali granites (orebody) to be 123.9 ± 1.2 Ma and 123.5 ± 3.2 Ma, respectively. Chen et al. (Citation2019) determined the 40Ar/39Ar plateau age of riebeckite in strongly altered riebeckite granites to be 122.15 ± 0.65 Ma. These previous chronological data show that the metallogenic age of the Baerzhe deposit is 125.2–122.15 Ma.
Whole-rock geochemistry
Saima deposit
The nepheline syenite (orebody) samples have SiO2 contents of 51.89–54.97 wt% (average: 53.31), Al2O3 contents of 17.07–19.88 wt% (average: 18.40), total alkali content (Na2O + K2O) of 14.25–16.12 wt% (average: 14.80), K2O/Na2O ratios of 1.10–2.44 (average: 1.75), TFeO/MgO ratios of 40.55–757.72 (average: 316.64), A/NK ratios of 0.89–1.06 (average: 0.96), A/CNK ratios of 0.85–1.01 (average: 0.92), Rittman index (σ) of 19.34–22.83 (average: 21.36), peralkaline index (PI) of 2.92–6.87 (average: 4.10) and low contents of CaO, MnO, P2O5 and TiO2 (Supplemental data, Table S1). These samples fall into the range of the nepheline syenites on the TAS diagram (), the peralkaline region on the A/NK–A/CNK diagram (), the tholeiite series on the FAM diagram () and the ferroan zone on the FeOT/(FeOT+MgO)–SiO2 diagram () demonstrating these samples are syenites of the ferrous peralkaline tholeiitic series.
Figure 7. Major elements of plutons from the Saima and Baerzhe deposits. (a) Magmatite TAS diagram (after Middlemost, Citation1994); (b) A/NK–A/CNK diagram (after Maniar & Piccoli, Citation1989); (c) FAM diagram; and (d) FeOt/(FeOt + MgO)–SiO2 diagram.
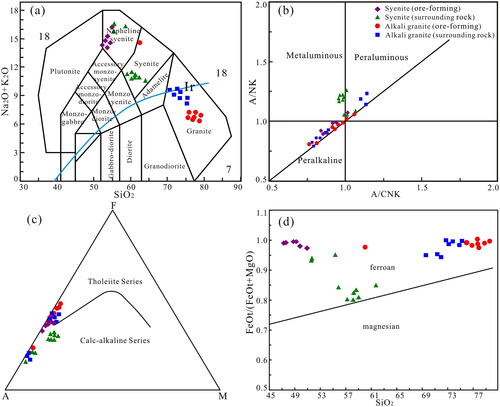
The nepheline syenite orebody samples have total REE (ΣREE) of 828.22 × 10−6–1266.52 × 10−6 (average: 1027.20 × 10−6), total LREE of 734.78 × 10−6–1187.28 × 10−6 (average: 915.03 × 10−6) and total heavy rare earth element (HREE) of 77.24 × 10−6–158.79 × 10−6 (average: 112.17 × 10−6) with rightward chondrite-normalised REE patterns, indicating LREE enrichment and HREE depletion (). The LREEs and HREEs show a moderate degree of fractionation, with LREE/HREE ratios of 5.80–15.37 (average: 8.76) and (La/Yb)N = 7.14–25.23 (average: 11.16). The samples have a flat HREE curve, with (Gd/Yb)N = 1.45–2.37, with no Eu or Ce anomalies (δEu = 0.83–0.90, average: 0.87; δCe = 0.74–1.13, average: 0.98). On the spidergram of primitive mantle-normalised trace elements (), the rock samples are enriched in elements such as Th, U, Nd, La, Ce, Sr, Nd, Zr and Hf, and depleted in P and Ti.
Figure 8. Chondrite-normalised trace element patterns and primitive mantle-normalised spider diagrams samples from the (a, b) Saima and (c, d) Baerzhe deposits (normalisation values after Boynton Citation1984; primitive mantle-normalised values after Sun and McDonough Citation1989).
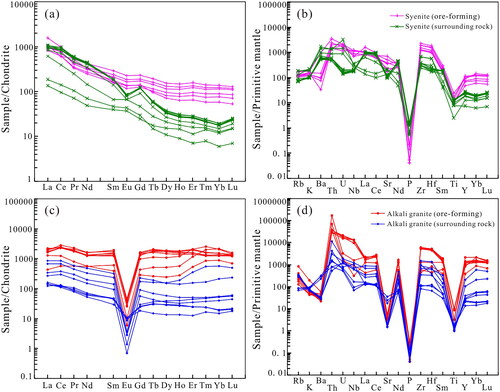
The nepheline syenite host rock samples have SiO2 contents of 55.52–64.04 wt% (average: 59.84), Al2O3 contents of 16.61–20.32 wt% (average: 18.06) and total alkali contents (Na2O + K2O) of 10.53–16.47 wt% (average: 12.49), K2O/Na2O ratios of 1.39–10.05 (average: 2.94), TFeO/MgO ratios of 4.13–21.57 (average: 8.36), A/NK ratios of 1.03–1.27 (average: 1.16), A/CNK ratios of 0.96–1.07 (average: 1.00), Rittman index (σ) of 5.27–21.48 (average: 10.41), PI of 2.53–10.50 (average: 4.77) and low contents of MnO, P2O5 and TiO2. These samples mainly fall on the syenite zone on the TAS diagram (), the meta-aluminous to peraluminous zone in the A/NK–A/CNK diagram (), the calc-alkaline series zone on the FAM diagram () and the ferroan zone on the FeOT/(FeOT+MgO)–SiO2 diagram (), demonstrating they are syenites of the ferrous meta-aluminous to peraluminous calc-alkaline series.
The host rock nepheline syenite samples have total REE (ΣREE) of 142.72 × 10−6–1175.38 × 10−6 (average: 839.06 × 10−6), total LREE of 127.74 × 10−6–1129.03 × 10−6 (average: 802.91 × 10−6) and total HREE contents of 12.39 × 10−6–47.69 × 10−6 (average: 36.15 × 10−6). They have rightward chondrite-normalised REE patterns, indicating LREE enrichment and HREE depletion () and high degrees of fractionation of LREEs and HREEs, with LREE/HREE = 8.53–24.36 (average: 20.78) and (La/Yb)N = 10.64–55.14 (average: 44.36) with a flat HREE curve, with (Gd/Yb)N = 2.02–6.72. Moreover, these samples have weak negative anomalies to weak positive Eu anomalies (δEu = 0.53–1.43, average: 0.76) but no Ce anomaly (δCe = 0.90–1.15, average: 1.05). As shown in the spidergram of primitive mantle-normalised trace elements (), these samples are enriched in elements including Ba, Th, Nd, Zr and Hf, and depleted in elements such as P, Ti, Y, Yb and Lu.
Baerzhe deposit
The Balger alkaline granites are mainly divided into superfused granites, which are unaltered and boundary melt granites that are altered by sodium metasomatism and hematisation (Ma et al., Citation2023).
The alkali granite (orebody) samples have SiO2 contents of 62.64–79.01 wt% (average: 75.65), Al2O3 contents of 8.18–16.31 wt% (average: 9.53), total alkali (Na2O + K2O) of 6.27–14.46 wt% (average: 7.76), K2O/Na2O ratios of 0.67–8.98 (average: 2.10), TFeO/MgO ratios of 46.87–1979.01 (average: 463.50), A/NK ratios of 0.80–1.05 (average: 0.94), A/CNK ratios of 0.78–1.05 (average: 0.93), Rittman index (σ) of 1.11–10.65 (average: 2.51), PI of 3.77–16.43 (average: 8.53) and low contents of CaO, MnO, P2O5 and TiO2 (Supplemental data, Tables S1 and S2). The rock samples plot onto the granite zone in the TAS diagram (), mostly into the peralkaline region on the A/NK–A/CNK diagram (), the tholeiitic series on the FAM diagram () and the ferroan zone on the FeOT/(FeOT+MgO)–SiO2 diagram () suggesting these samples are from a ferrous peralkaline tholeiitic series.
The alkali granite (orebody) samples had total REE content (ΣREE) of 1759.36 × 10−6–4711.23 × 10−6 (average: 3803.69 × 10−6), total LREE content of 850.09 × 10−6–3400.69 × 10−6 (average: 2540.59 × 10−6) and total HREE content of 420.35 × 10−6–1644.80 × 10−6 (average: 1263.11 × 10−6). They showed V-shaped chondrite-normalised REE patterns, indicating strong Eu depletion (δEu = 0.02–0.03) and no Ce anomaly (δCe = 1.12–1.29, average: 1.21; ). As shown in the spidergram of primitive mantle-normalised trace elements (), these samples were relatively enriched in elements such as Th, U, Nb, La, Ce, Nd, Zr and Hf, and were relatively depleted in elements such as Ba, Sr, P and Ti.
The alkali granite (surrounding rock) samples have SiO2 contents of 70.64–75.50 wt% (average: 73.40), Al2O3 contents of 9.58–15.16 wt% (average: 11.67), total alkali (Na2O + K2O) of 8.10–9.53 wt% (average: 8.97), K2O/Na2O ratios of 0.89–1.73 (average: 1.28), TFeO/MgO ratios of 17.63–1017.16 (average: 212.62), A/NK ratios of 0.79–1.23 (average: 0.97), A/CNK ratios of 0.79–1.14 (average: 0.94), Rittman index (σ) of 2.03–3.29 (average: 2.67), PI of 2.60–23.49 (average: 9.69) and low contents of CaO, MnO, P2O5 and TiO2. These samples plot on the granite zone in the TAS diagram (), within the peralkaline zone into the peraluminous zone on the A/NK–A/CNK diagram (), within the zone of the tholeiite series into the zone of the calc-alkaline series on the FAM diagram () and onto the ferroan zone on the FeOT/(FeOT+MgO)–SiO2 diagram () suggesting a ferrous peralkaline tholeiitic series.
The alkali granite (surrounding rock) samples had a total REE content (ΣREE) of 151.43 × 10−6–1212.13 × 10−6 (average: 487.15 × 10−6), total LREE content of 137.59 × 10−6–1041.76 × 10−6 (average: 406.94 × 10−6), total HREE content of 13.84 × 10−6–274.35 × 10−6 (average: 80.21 × 10−6) and V-shaped chondrite-normalised REE patterns, indicating strong Eu depletion (δEu = 0.01–0.04, average: 0.13) and no Ce anomaly (δCe = 0.91–1.26, average: 1.10; ). On the spidergram of primitive mantle-normalised trace elements (), the samples are enriched in Th, U, Nb, La, Ce, Nd, Zr and Hf, and relatively depleted in Sr, P and Ti.
Sr–Nd–Pb isotopes
Saima deposit
The nepheline syenite (orebody) samples had Rb and Sr contents of 256.11 × 10−6–383.32 × 10−6 and 1237.99 × 10−6–2817.35 × 10−6, respectively,87Sr/86Sr and 143Nd/144Nd ratios of 0.709334–0.711340 and 0.511855–0.511938 (Supplemental data, Table S2), respectively, and 208Pb/204Pb, 207Pb/204Pb and 206Pb/204Pb ratios of 36.9803–37.6198, 15.5020–15.5235 and 16.9282–17.3383 (Supplemental data, Table S3), respectively. After corrections for the weighted average crystallisation age of the nepheline syenites in the Saima deposit (224 Ma), the compositions of Rb, Sr and Pb, the initial ISr and INd values were 0.708360–0.708510 and 0.511666–0.511753, respectively; the corresponding εNd(t) values were −13.34 to −11.65, and the Nd model age (TDM) and two-stage model age (T2DM) were 2319–1666 Ma and 2080–1944 Ma, respectively. Moreover, the initial (208Pb/204Pb)i, (207Pb/204Pb)I and (206Pb/204Pb)i ratios were 36.932–37.019, 15.500–15.508 and 16.891–17.036, respectively.
The nepheline syenite (surrounding rock) samples had Rb and Sr contents of 160.28 × 10−6–433.97 × 10−6 and 684.91 × 10−6–1501.88 × 10−6, respectively, 87Sr/86Sr and 143Nd/144Nd ratios of 0.710845–0.720246 and 0.511074–0.511865, respectively, and 208Pb/204Pb, 207Pb/204Pb and 206Pb/204Pb ratios of 35.7990–37.3475, 15.0334–15.5368 and 15.0631–17.5763, respectively. The initial ISr and INd values (224 Ma) were 0.708380–0.717520 and 0.510953–0.511690, respectively, the corresponding εNd(t) values were −27.26 to −12.87, and the Nd TDM and T2DM ages were 2425–1652 Ma and 3209–2047 Ma, respectively. Moreover, the initial (208Pb/204Pb)i, (207Pb/204Pb)i and (206Pb/204Pb)i ratios were 35.480–36.953, 15.029–15.499 and 14.984–16.846, respectively.
Baerzhe deposit
The alkali granite (orebody) samples had Rb and Sr contents of 290.39 × 10−6–1900.00 × 10−6 and 14.94 × 10−6–39.52 × 10−6, respectively, 87Sr/86Sr and 143Nd/144Nd ratios of 0.710865–1.344385 and 0.512737–0.512797, respectively, and 208Pb/204Pb, 207Pb/204Pb and 206Pb/204Pb ratios of 38.1321–38.3839, 15.5390–15.5584 and 18.2161–18.6394, respectively. The initial ISr and INd values of the alkali granites in the Baerzhe deposit (122 Ma) were 0.62109–0.93931 and 0.51257–0.51265, respectively, the corresponding εNd(t) values were 1.68–3.20, and the Nd TDM and T2DM ages were −18 392 (–1512) to 71 082 Ma and 818–641 Ma, respectively. Moreover, the initial (208Pb/204Pb)i, (207Pb/204Pb)I and (206Pb/204Pb)i ratios were 37.300–38.120, 15.539–15.548 and 18.211–18.424, respectively.
The alkali granite (surrounding rock) samples had Rb and Sr contents of 162.60 × 10−6–791.71 × 10−6 and 10.44 × 10−6–256.04 × 10−6, respectively, 87Sr/86Sr and 143Nd/144Nd ratios of 0.708259–2.431830 and 0.512653–0.512701, respectively, and 208Pb/204Pb, 207Pb/204Pb and 206Pb/204Pb ratios of 38.2144–38.4404, 15.5414–15.5704 and 18.2319–18.8503, respectively. The initial ISr and INd values (122 Ma) were 0.70444–2.05108 and 0.51253–0.51259, respectively, the corresponding εNd(t) values were 0.96–2.15, and the Nd TDM and T2DM ages were 1265–795 Ma and 839–743 Ma, respectively. Moreover, the initial (208Pb/204Pb)i, (207Pb/204Pb)i and (206Pb/204Pb)i ratios were 36.781–38.241, 15.541–15.557 and 18.220–18.578, respectively.
Discussion
Metallogenic epoch
In recent years, three phases of large-scale Mesozoic mineralisation have been identified in northeastern China (Ju et al., Citation2019; Ren et al., Citation2012; F. Y. Wu et al., Citation2000; Zhang et al., Citation2015). (1) At 255–190 Ma, a strong continental–continental collision between the North China Craton and the Paleo-Asian Plate resulted in crustal compression, shortening and stacked and thickened orogenesis. Moreover, the crustal materials were metamorphosed, dehydrated and partially melted, primarily forming crust-derived high-viscosity granites accompanied by the formation of Indosinian Cu–Mo–REE polymetallic deposits, such as Xishadegai Molybdenum deposit (Hou et al., Citation2010). (2) At 200–130 Ma, there was a subduction of the Paleo-Pacific Plate toward the Eurasian Plate from the Early Jurassic to the Early Cretaceous with a peak in the Late Jurassic. The tectonic system transition led to the development of a series of E–W- and NE-trending deep fault structures and many secondary structures, which provided favourable conditions for magma intrusion and migration of ore fluids, such as the Xiaojiayingzi Molybdenum (Fe) deposit (Dai et al., Citation2007). (3) At 150–20 Ma, the Siberian Plate collided with the North China Plate, causing renewed crustal compression and thickened orogenesis, forming the intermediate acid magmas and ore fluids, such as the Chalukou porphyry Mo polymetallic deposit (Nie et al., Citation2011). Subduction of the Pacific Plate peaked (140 Ma), and a major transformation of the tectonic framework of eastern China evolved into the circum-West Pacific tectonic domain and the developments of the ore system of the circum-Pacific tectonic system. The three phases of Mesozoic intense magmatic-metallogenic events in northeastern China corresponded to the beginning and peaking of the Paleo-Pacific Plate subduction, which coincided with the subduction and closure of the Paleo-Asian Ocean and are consistent with the documented tectonic evolution of the eastern section of the Central Asian Orogenic Belt. The Late Indosinian–Yanshanian metallogenic events in northeastern China resulted from the combined action of multiple plates.
Sixteen single-grain zircons from the nepheline syenites of the Saima deposit were all idiomorphic–hypidiomorphic long-columnar and had significant magmatic oscillatory zoning, with Th/U ratios greater than 0.1, suggesting the typical characteristics of zircons from magmatic crystallisation at 224.40 ± 6.10 Ma (Ju et al., Citation2019). The Baerzhe deposit has a metallogenic age of 125.2–122.15 Ma and formed as a part of the large-scale Yanshanian metallogenic event in northeastern China (Yang et al., Citation2014).
Genetic types of rocks
Both the nepheline syenites (orebody and surrounding rocks) and alkali granites (orebody and surrounding rocks) have high alkali content (Na2O + K2O), high TFeO/MgO ratios, low MgO and CaO contents, PI values of greater than 0.85 and Zr content greater than 250 × 10−6. Therefore, they are consistent with the geochemical characteristics of A-type granites (Whalen et al., Citation1987; Xu & Qiu, Citation2010), which are widely considered to have high crystallisation temperatures around 800–900 °C (Liu et al., Citation2003). The crystallisation temperatures of the Saima nepheline syenites and the Baerzhe alkali granites were determined using the Ti-in-zircon thermometer (Watson et al., Citation2006). The crystallisation temperatures of zircons from the nepheline syenites (orebody and surrounding rock) in the Saima deposit were 1091–1173 °C and 940–1053 °C, respectively, whereas zircons from the alkali granites (orebody and surrounding rock) in the Baerzhe deposit were 1035–1545 °C and 856–1201 °C, respectively comparable with A-type granites. Moreover, the crystallisation temperatures of nepheline syenites (orebody) and alkali granites (orebody) are significantly higher than those of surrounding rocks bearing no ores. The nepheline syenite and alkali granite samples plot into the A-type granite region in the (Na2O + K2O)–10 000Ga/Al (), Ce–10 000Ga/Al () and Zr–10 000Ga/Al () diagrams and into the A1-type granite zone and its adjacent zones in the Yb/Ta–Y/Nb diagram ().
Figure 9. Discrimination diagrams of ore-forming samples from the Saima and Baerzhe deposits [after Whalen et al., Citation1987; the evolution trend line in (b) after M. Wu et al. Citation2017]. (a) (Na2O + K2O)–10 000Ga/Al; (b) Ce–10 000Ga/Al; (c) Zr–10 000Ga/Al; and (d) Yb/Ta–Y/Nb.
![Figure 9. Discrimination diagrams of ore-forming samples from the Saima and Baerzhe deposits [after Whalen et al., Citation1987; the evolution trend line in (b) after M. Wu et al. Citation2017]. (a) (Na2O + K2O)–10 000Ga/Al; (b) Ce–10 000Ga/Al; (c) Zr–10 000Ga/Al; and (d) Yb/Ta–Y/Nb.](/cms/asset/e3ea68da-015a-49e9-a7ff-d5757a0a83d9/taje_a_2353286_f0009_c.jpg)
Magma sources
Magma sources of nepheline syenites in the Saima deposit
The nepheline syenite (orebody) samples from the Saima deposit had ISr values of 0.708360–0.708510, INd values of 0.511666–0.511753, εNd(t) values of −13.34 to −11.65 and T2DM ages of 2080–1944 Ma, indicating that the magmas were derived from ancient crust or enriched mantle. The samples plot near the mantle evolution trend line in the ISr–εNd(t) diagram (), into the lower crustal marginal region in the 207Pb/204Pb–206Pb/204Pb diagram (), near the I-type enriched mantle (EMI) and lower crust regions in the 207Pb/204Pb–206Pb/204Pb diagram () and into the EMI region in the 208Pb/204Pb–206Pb/204Pb diagram (), indicating that the magmas of the nepheline syenite (orebody) in the Saima deposit originate from EMI. The nepheline syenite (orebody) lithogeochemistry have characteristics of alkali-rich and high-potassium alkaline magmas, with extremely high LREE content (734.78 × 10−6–1187.28 × 10−6). Moreover, their Rb and Sr element contents (Rb = 256.11 × 10−6–383.32 × 10−6; Sr = 1237.99 × 10−6–2817.35 × 10−6) are higher than average continental crust (Rb = 49 × 10−6, Sr = 320 × 10−6 (Rudnick & Gao, Citation2003) indicating that the magmas originated from a mantle source enriched in LREEs and large-ion lithophile elements. The low compatible element contents of Ni = 0.25–1.86 × 10−6, Co = 1.98–9.34 × 10−6 and Sc = 0.63–4.92 × 10−6 are associated with crystallisation and differentiation of the original mantle solution. The strong HREE depletion and absence of an Eu anomaly indicate garnet in the residual facies in the magma source.
Figure 10. Magmatic source area discrimination diagrams of samples from the Saima and Baerzhe deposits (EMI, EMII, HIMU and Primitive after Zindler and Hart, Citation1986; lower crust, mantle after Zartman and Doe Citation1981): (a) ISr–εNd(t); (b) 207Pb/204Pb–206Pb/204Pb; (c) 207Pb/204Pb–206Pb/204Pb; and (d) 208Pb/204Pb–206Pb/204Pb.
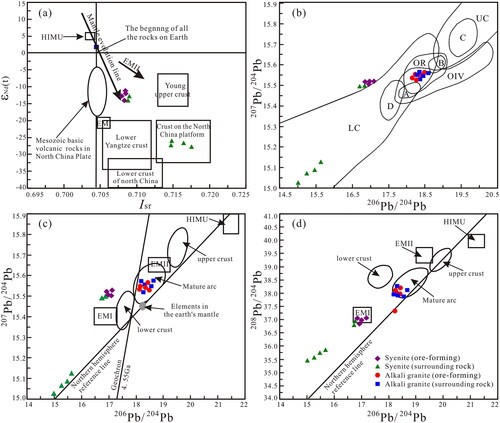
The nepheline syenite (surrounding rock) samples from the Saima deposit have an ISr of 0.708380–0.717520, INd of 0.510953–0.511690, εNd(t) of −27.26 to −12.87 (average: −22.05) and T2DM ages of 3209–2047 Ma, similar to the ISr values (0.7050–0.7110) and εNd(t) values (<–20) of the crustal materials of the North China Platform and indicating that the magmas may have originated from a lower crustal source of the North China Platform. The Saima nepheline syenite (surrounding rock) lithogeochemistry samples have characteristics of alkali-rich and high-potassium alkaline magmas with enriched LHEEs, Rb and Sr compared with continental crust rocks and a mantle source enriched in LREEs and large-ion lithophile elements. The nepheline syenite (surrounding rock) samples plot into the upper crust zone of the North China Platform and near the mantle evolution trend line in the ISr–εNd(t) diagram (), into the lower crust and its marginal zones on the 207Pb/204Pb–206Pb/204Pb diagram (), near the EMI and the lower crust zones and near the reference line in the 207Pb/204Pb–206Pb/204Pb discrimination diagram () and into the EMI zone and the reference line in the northern hemisphere on the 208Pb/204Pb–206Pb/204Pb discrimination diagram (), indicating that the magmas of the nepheline syenites (surrounding rocks) originate from the crust–mantle mixing source.
The field geological observations show that the nepheline syenites (orebody) and the syenites (surrounding rocks) of the Saima deposit belong to the same magmatic body and thus should have the same magma source. Compared with syenites (orebody), the nepheline syenites (surrounding rocks) of the Saima deposit have lower INd and εNd(t) values, higher ISr values and stronger LREE and HREE fractionation, indicating that the nepheline syenites (surrounding rocks) were more strongly affected by the crustal contamination during the magmatic evolution.
Sources of alkali granites in the Baerzhe deposit
A-type granites are widely associated with crustal extension (Clemens et al., Citation1986; Ostendorf et al., Citation2014) with magmas from a range of sources, including the depleted mantle, primitive mantle, enriched mantle, crust–mantle mixing and crust (S. P. Wu et al., Citation2007). The alkali granites (orebody and surrounding rock) in the Baerzhe deposit have a similar lithogeochemistry, such as enrichment of Si and Na + K, depletion of Ca and Mg, strong Eu negative anomalies, no Ce anomaly, enrichment of elements including Th, U, Nb, La, Ce, Nd, Zr and Hf, and the depletion of Ba, Sr, P and Ti, indicating a common magma source. Based on field geological observations, experimental petrology, element geochemistry and Sr–Nd–Pb isotope tracing, the alkali granites (orebody and surrounding rock) of the Baerzhe deposit originated from a depleted lithospheric mantle with contamination by crustal materials. The alkali granite (orebody and surrounding rock) samples from the Baerzhe deposit had positive εNd(t) values (1.68–3.20 and 0.96–2.15, respectively) and young Nd T2DM ages (818–641 Ma and 839–743 Ma, respectively), indicating the presence of a juvenile depleted mantle source (King et al., Citation1997; Qiu et al., Citation2005; F. Y. Wu et al., Citation2002). Unlike the enriched mantle with high Sr content of up to 1100 × 10−6 (Chen & Zhai, Citation2003), the alkali granite (orebody and surrounding rocks) samples had a low Sr content, ranging from 13.79 × 10−6 to 39.52 × 10−6 and 10.44 × 10−6 to 256.04 × 10−6, respectively. Therefore, the alkali granites are unlikely to have formed from the partial melting of the enriched mantle. The Sr content of the depleted mantle and the lower crust is 20 × 10−6 and 290 × 10−6, respectively (Wang et al., Citation2006), similar to the Sr content of the alkali granites, so likely resulted from the mixing of lower crust with depleted mantle.
Tectonic setting and mineralisation
The northern margin of the North China Craton is adjacent to the Central Asian Orogenic Belt, and the magmatic activities on the northern margin are closely associated with the tectonic evolution of the Paleo-Asian Ocean (Fan et al., Citation2019; Liu et al., Citation2017; Windley et al., Citation2007; F. Y. Wu et al., Citation2007; Xiao et al., Citation2003). The Paleo-Asian Ocean closed in a scissor pattern from west to east along the Xilamulun–Changchun–Yanji area between the late Silurian and the late Permian (Chen et al., Citation2000; Li et al., Citation2007, Citation2015; Miao et al., Citation2007, Citation2008; F. Y. Wu et al., Citation2007). The timing of closure of the Paleo-Asian Ocean is still disputed, varying from the end of the Permian to the beginning of the Triassic (Song et al., Citation2015; Xiao et al., Citation2003, Citation2015; Zhou et al., Citation2017), but most researchers consider that the closure occurred in the late Permian (Chen et al., Citation2009; Fan et al., Citation2019; F. Y. Wu et al., Citation2011; Zhang et al., Citation2009) with the northern margin of the North China Craton in a post-collision extensional tectonic setting during the Middle–Late Triassic (Fan et al., Citation2019; Yang, Sun, et al., Citation2012; Zhang et al., Citation2014). Existing research results show that the Carboniferous–Permian magmatic rocks in the eastern part of the Central Asian Orogenic Belt have subduction-related geochemical characteristics (F. Y. Wu et al., Citation2011; Zhang et al., Citation2009, Citation2016; Zhong et al., Citation2020), while the Late Triassic magmatic rocks have geochemical characteristics related to the post-orogenic extensional environment (Yang, Sun, et al., Citation2012; Zhang et al., Citation2014), confirming that the northern margin of the North China Craton was in the extensional tectonic setting after the closure of the Paleo-Asian Ocean during the Late Triassic.
Rare-element and REE deposits and ore-bearing rock masses are mostly distributed on plate margins or at the junctions of structures with different ages that are controlled by regional large faults with ore-controlling structures mostly derived secondary structures. Since the deposits of this type are closely related to magmatism, the rare-element and REE metallogenic belts are spatially consistent with active zones of alkaline magmatic rocks (Ju et al., Citation2019). The formation of Early Cretaceous A-type granites on the margin of the North China Craton is related to the subduction of the Paleo-Pacific Plate (F. Y. Wu et al., Citation2002) in the Early Cretaceous, thinning the lithosphere on the northern margin of the North China Craton associated with upwelling of mantle magmas along irregular channels formed by extensional failure in the shallow crust (Sun et al., Citation2007; Zheng et al., Citation2007). F. Y. Wu et al. (Citation2002) showed the Permian–Cretaceous A-type granites (300–120 Ma) in northeastern China resulted from the rupture of the subducting slab of the Paleo-Asian Ocean in the Permian; Triassic–Early Jurassic A-type granites were formed by asthenosphere upwelling and mantle melting under the post-orogenic large-scale lithospheric delamination after subduction–collision; and the Cretaceous A-type granites were associated with the lithosphere delamination during extension, which was accompanied by late Mesozoic subduction of the Pacific Plate (F. Y. Wu et al., Citation2002).
The host rocks of the Saima and Baerzhe deposits are nepheline syenites and alkali granites, respectively, both of which are alkaline magmatic rocks of A-type granite affinity. The occurrence of A-type granites is closely related to the extensional thinning of the lithosphere, while the decompression and unloading of the lithosphere are closely associated with the upwelling and underplating of mantle materials. As revealed by the zircon U–Pb isotope dating in this study and previous chronological studies, the metallogenic epochs of the Saima and Baerzhe deposits are the late stage of the Late Triassic and the late stage of the Early Cretaceous, respectively. The Saima deposit nepheline syenites and the Baerzhe deposit alkali granites plot onto the intraplate granite zone in the Ta–Yb diagram (), and the Rb–(Y + Nb) () diagrams, except for the nepheline syenite (surrounding rock) samples, which plot into the post-collision granite region. In addition, they plot near the extensional structural setting zone on the log(CaO/(Na2O + K2O))–SiO2 diagram (). In the R1–R2 diagram (), the nepheline syenite (orebody) samples plot into the non-orogenic A-type granite region, the nepheline syenite (surrounding rock) samples plot in the late orogenic granite region, and all the alkali granite samples plot in the post-orogenic A-type granite zone. Therefore, the nepheline syenites in the Saima deposit were formed in an extensional tectonic setting after the closure of the Paleo-Asian Ocean, whereas the alkali granites in the Baerzhe deposit formed after the subduction of the Paleo-Pacific Ocean in an extensional tectonic setting.
Figure 11. Tectonic setting discrimination diagrams of samples from the Saima and Baerzhe deposits. (a) Ta–Yb (after Pearce et al., Citation1984); (b) Rb–(Y + Nb) (after Pearce et al., Citation1984); (c) log(CaO/(Na2O + K2O))–SiO2 (after Brown et al., Citation1984); and (d) R1–R2 (after Brown et al., Citation1984).
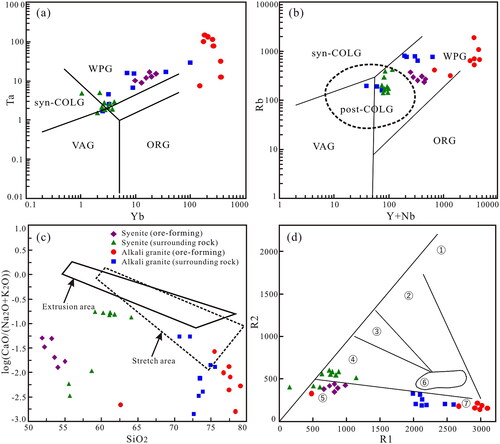
In the post-orogenic stage, deep faults, which were formed or resurrected by crustal extension and strike-slip motion, serve as upwelling channels for a mantle magma rich in LREEs and large-ion lithophile elements, forming the orebody hosted in nepheline syenites. The magma for the alkali-feldspar granites in the Baerzhe deposit, which were intruded in an intracontinental extensional tectonic setting, were derived from a depleted mantle that upwelled, underplated and partially melted lower crustal materials. The tectonic setting of the alkali-feldspar granites in the Baerzhe deposit is interpreted to be associated with subduction of the Paleo-Pacific Plate.
Magmatic evolution and rare-earth element mineralisation
The REE tetrad effect of granites has been shown to be associated with a high degree of crystallisation and differentiation of granite magmas that enriches the residual in volatile constituents including F, H2O and Cl, and enriches halogen-rich fluids in residual melts, forming a melt–fluid system. The melt–fluid interactions present an M-type REE tetrad effect with extreme enrichment of rare elements in the melt–fluid forming superlarge and large, rare metal deposits (Fan et al., Citation2019; Li et al., Citation2015; M. Wu et al., Citation2021; Yang et al., Citation2009). A system with an REE tetrad effect deviates significantly from chondrites in terms of Zr/Hf and Y/Ho ratios and is constrained by the highly evolved magmas rich in volatile constituents, indicating that the magmatic system has entered an aqueous-like fluid system (Bau, Citation1996). Irber (Citation1999) took the TE1,3 values of 1.0 and 1.1 as the boundaries and showed that rocks with TE1,3 values greater than 1.1 had an apparent REE tetrad effect and that rocks with TE1,3 values less than 1.0 had no REE tetrad effect. As shown in , the alkali granite samples from the Baerzhe deposit had TE1,3 values of 1.02–1.29 (orebody) and 1.00–1.16 (surrounding rock) and obvious and slight REE tetrad effects, respectively. The nepheline syenite from the Saima deposit has TE1,3 values of 0.91–0.98 (orebody) and 0.90–0.95 (surrounding rock), and no REE tetrad effect. The Y/Ho, Zr/Hf and K/Rb ratios () of the nepheline syenite and alkali granite samples decreased with increasing TE1,3 values, whereas the K/Ba ratio increased with increasing TE1,3 values. Moreover, this trend is greatest in the orebody samples, which have significantly higher TE1,3 values than their corresponding surrounding rock samples. These results indicate a significantly higher magma evolution in the orebodies than their corresponding surrounding rocks. As shown in the Ba–Eu, Sr–Eu, Ba–Sr and 1/Sr–Rb/Sr covariation diagrams (), in both deposits the linear trends indicate that the magmas have undergone strong differentiation after emplacement. In the Saima deposit, this is primarily controlled by crystallisation of biotite, feldspar and hornblende, whereas in the Baerzhe deposit, the linear trend is mainly controlled by crystallisation of feldspars.
Figure 12. Numerical modelling diagrams for trace elements of samples from the Saima and Baerzhe deposits (modified after Xu et al., Citation2015). (a) Y/Ho–TE1,3; (b) Zr/Hf–TE1,3; (c) K/Rb–TE1,3; and (d) K/Ba–TE1,3. Amp, amphibole; Bt, biotite; Grt, garnet; Kf, K-feldspar; Ms, muscovite; Pl, plagioclase.
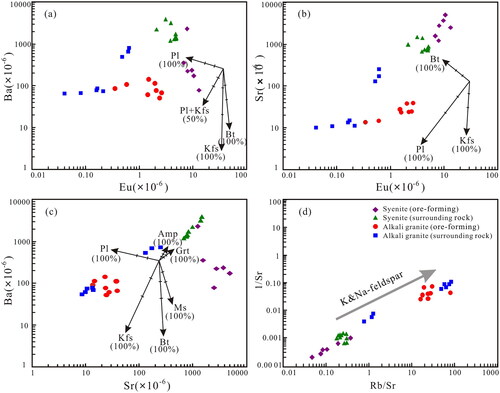
Figure 13. Discrimination diagrams for degree of magma evolution for samples from the Saima and Baerzhe deposits (after F. Y. Wu et al., Citation2021): (a) Ba–Eu; (b) Sr–Eu; (c) Ba–Sr; and (d) 1/Sr–Rb/Sr.
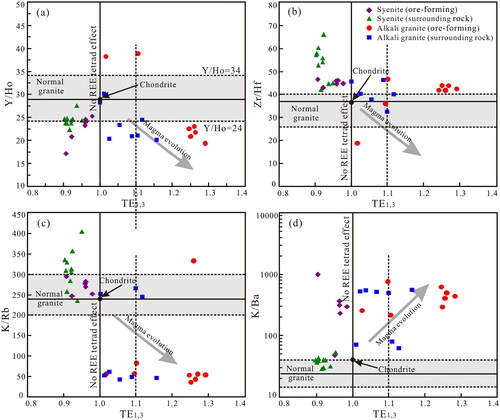
In the Baerzhe deposit, the higher TE1,3 values in the orebody samples (1.02–1.29, mostly >1.10) than in the surrounding rocks (1.00–1.16, mostly 1.00–1.10) suggest that the original magmas of alkali granites (orebody) are a solution rich in volatile constituents (F, H2O and Cl) that included significant melt–fluid interactions in addition to fractional crystallisation. The Baerzhe alkali granite orebody contains many geodes confirming formation associated with the magma-hydrothermal stage (Yang et al., Citation2009) and that mineralisation is controlled by melt–fluid interactions in the later stages of highly evolved magmas.
In contrast to the alkali granite (surrounding rock) samples from the Baerzhe deposit, the nepheline syenite samples from the Saima deposit have normal chondrite-normalised REE patterns (TE1,3 values <1.00) with Zr/Hf and Y/Ho ratios in the range of normal magmatic rocks. Zhu et al. (Citation2016) found that the alkaline complex area in the Saima deposit experienced a differentiation process from silica-saturated syenites (trachytes and quartz syenites) to syenites not saturated with silicon (nepheline syenites, lujavrites and phonolites). Based on the study of eudialytes in nepheline syenites, B. Wu et al. (Citation2018, Citation2020) showed that the ore-forming magmatic solution experienced the superposition of the autometasomatic fluids rich in Na, K, Al and F (predominant) with small amounts of Ca-rich fluids (secondary) and that the mineralisation resulted from crystallisation and differentiation. Zhong et al. (Citation2020) considered that the initial nepheline syenites magmas had a high oxygen fugacity and high temperature, and that after undergoing biotite crystallisation, the residual magmas had a lower oxygen fugacity and higher Na content, laying a foundation for the formation of the Saima deposit. In the Saima deposit, the nepheline syenites (orebody) have a significantly higher degree of magmatic evolution than their surrounding rocks ( and ) and with biotite, feldspar and amphibole crystallisation and differentiation.
Both the Saima and Baerzhe deposits have the characteristics of alkali-rich and high-potassium alkaline magmas with extremely high LREE content, rich in Th, U, Nb, La, Ce, Nd, Zr and Hf, and relatively deficient in P and Ti, and have undergone strong differentiation after emplacement. The differentiation of nepheline syenites in the Saima deposit is primarily controlled by the crystallisation of biotite, feldspar and hornblende, while the differentiation in the Baerzhe deposit is mainly controlled by feldspar crystallisation. Moreover, the orebodies in the Saima and Baerzhe deposits are formed by significantly higher degrees of magmatic evolution than their corresponding surrounding rocks. Therefore, mineralisation in both deposits is closely related to magmatic evolution with magmatic crystallisation and differentiation playing a crucial role in the mineralisation process.
Conclusions
The nepheline syenites (orebody) in the Saima deposit have a crystallisation age of 224.40 ± 6.10 Ma. They are A-type granites and formed in an extensional tectonic setting after the closure of the Paleo-Asian Ocean. The alkali granites (orebody) in the Baerzhe deposit have a crystallisation age of 125.2–122.15 Ma. They are also A-type granites that formed in an intracontinental extensional tectonic setting associated with the subduction of the Paleo-Pacific Plate.
The magmas of the nepheline syenites (Saima orebody) originate from the mantle source rich in LREEs and large-ion lithophile elements, whereas the magmas of the nepheline syenites (surrounding rocks) originate from a crust–mantle mixing source. The magmas of the alkali granites (Baerzhe orebody and surrounding rocks) originate from a mixing source of the lower crust and depleted mantle.
The nepheline syenites (orebody) in the Saima deposit have a higher degree of crystallisation and differentiation than the corresponding surrounding rocks, indicating that the magmatic crystallisation and differentiation play a crucial role in the mineralisation process of the deposit. The alkali granites (orebody) in the Baerzhe deposit have a significant REE tetrad effect, whereas alkali granites (surrounding rocks) have a slight REE tetrad effect. Therefore, mineralisation in the Baerzhe deposit is closely associated with magmatic evolution and is controlled by melt–fluid interactions in the later stages of highly evolved magmas.
The comparison of mineralisation in the Saima and Baerzhe deposits demonstrates that alkaline magmatic evolution plays a crucial role in the enrichment and mineralisation of rare-earth elements, and these rare-earth element deposits formed during the late stage of alkaline magmatic evolution.
Supplemental Material
Download MS Excel (54 KB)Acknowledgements
The authors thank Professor Yu Haocheng of China University of Geosicences, as well as the reviewers for their valuable comments.
Disclosure statement
No potential conflict of interest was reported by the author(s).
Data availability statement
The authors confirm that the data supporting the findings of this study are available within the article supplemental data files.
Additional information
Funding
References
- Bagas, L., Xiao, K., Jessell, M., & Li, N. (2017). Quantitative assessment of China’s mineral resources (Part 1). Ore Geology Reviews, 91, 1081–1083. https://doi.org/10.1016/j.oregeorev.2017.11.010
- Bau, M. (1996). Controls on the fractionation of isovalent trace elements in magmatic and aqueous systems: Evidence from Y/Ho, Zr/Hf, and lanthanide tetrad effect. Contributions to Mineralogy and Petrology, 123(3), 323–333. https://doi.org/10.1007/s004100050159
- Brown, G. C., Thorpe, R. S., & Webb, B. C. (1984). The geochemical characteristics of granitoids in contrasting arcs and comments on magma sources. Journal of the Geological Society, 141(3), 413–426. https://doi.org/10.1144/gsjgs.141.3.0413
- Boynton, W. V. (1984). Cosmochemistry of the rare earth elements: Meteorite studies. In P. Henderson (Ed.), Rare earth element geochemistry (pp. 63–114). Elsevier. https://doi.org/10.1016/B978-0-444-42148-7.50008-3
- Chen, B., & Zhai, M. G. (2003). Geochemistry of late Mesozoic lamprophyre dykes from the Taihang Mountains, north China, and implications for the sub-continental lithospheric mantle. Geological Magazine, 140(1), 87–93. https://doi.org/10.1017/S0016756802007124
- Chen, B., Jahn, B. M., & Tian, W. (2009). Evolution of the Solonker Suture Zone: Constraints from zircon U–Pb ages, Hf isotopic ratios and whole-rock Nd–Sr isotope compositions of subduction and collision related magmas and forearc sediments. Journal of Asian Earth Sciences, 34(3), 245–257. https://doi.org/10.1016/j.jseaes.2008.05.007
- Chen, B., Jahn, B. M., Wilde, S. A., & Xu, B. (2000). Two contrasting Paleozoic magmatic belts in northern Inner Mongolia, China: Petrogenesis and tectonic implications. Tectonophysics, 328(1-2), 157–182. https://doi.org/10.1016/S0040-1951(00)00182-7
- Chen, J. Y., Fan, H. H., Wang, S. Y., & Zhang, J. (2019). Geochemical characteristics and chronology of alkali-granites in Baerzhe area, Inner Mongolia, and geological significance. Geological Review, 65(S1), 45–46. (In Chinese with English abstract). https://doi.org/10.16509/j.georeview.2019.s1.020
- Clemens, J. D., Holloway, J. R., & White, A. J. R. (1986). Origin of an A-type granites: Experimental constrains. American Mineralogist, 71(3-4), 317–324.
- Dai, Z., Mao, J., Du, A., Xie, G., Bai, J., Yang, F., & Qu, W. (2007). Re–Os dating of molybdenite from the Xiaojiayingzi Mo (Fe) deposit in Western Liaoning and its geological significance. Acta Geologica Sinica, 81(7), 917–923. (in Chinese with English abstract)
- Deng, K., Li, Q. G., Chen, Y. J., Zhang, C., Zhu, X. F., & Xu, Q. W. (2018). Geochronology, geochemistry and Sr–Nd–Pb–Hf isotopes of the Early Jurassic granodiorite from the Sankuanggou intrusion, Heilongjiang Province, northeastern China: Petrogenesis and geodynamic implications. Lithos, 296-299, 113–128. https://doi.org/10.1016/j.lithos.2017.10.016
- Fan, W. B., Jiang, N., Zhai, M. G., & Hu, J. (2019). Phanerozoic garnet-bearing leucogranite in the northern margin of North China Craton: Characters, timing and preliminary petrogenesis study. Acta Petrologica Sinica, 35(07), 2237–2258. (In Chinese with English abstract). https://doi.org/10.18654/1000-0569/2019.07.18
- Hoskin, P. W. O., & Schaltegger, U. (2003). The composition of zircon and igneous and metamorphic petrogenesis. Reviews in Mineralogy and Geochemistry, 53(1), 27–62. https://doi.org/10.2113/0530027
- Hou, W. R., Nie, F., Du, A., Li, C., Jiang, S., Bai, D., & Liu, Y. (2010). Re–Os isotopic dating of molybdenite from Xishadegai molybdenum deposit in Urad Front Banner of Inner Mongolia and its geological significance. Mineral Deposits, 29(6), 1045–1053. (in Chinese with English abstract)
- Irber, W. (1999). The lanthanide tetrad effect and its correlation with K/Rb, Eu/Eu*, Sr/Eu, Y/Ho, and Zr/Hf of evolving peraluminous granite suites. Geochimica et Cosmochimica Acta, 63(3-4), 489–508. https://doi.org/10.1016/s0016-7037(99)00027-7
- Jahn, B. M., Wu, F. Y., Capdevila, R., Martineau, F., Zhao, Z. H., & Wang, Y. X. (2001). Highly evolved juvenile granites with tetrad REE patterns: The Woduhe and Baerzhe granites from the Great Xing’an Mountains in NE China. Lithos, 59(4), 171–198. https://doi.org/10.1016/S0024-4937(01)00066-4
- Ju, N., Ren, Y. S., Zhang, S., Bi, Z. W., Shi, L., Zhang, D., Shang, Q. Q., Yang, Q., Wang, Z. G., Gu, Y. C., Sun, Q. S., & Wu, T. (2019). Metallogenic epoch and tectonic setting of Saima niobium deposit in Fengcheng, Liaoning Province, NE China. Minerals, 9(2), 80. https://doi.org/10.3390/min9020080
- King, P. L., White, A. J. R., Chappell, B. W., & Allen, C. M. (1997). Characterization and origin of aluminous A-type granites from the Lachlan Fold Belt, southeastern Australia. Journal of Petrology, 38(3), 371–391. https://doi.org/10.1093/petroj/38.3.371
- Li, J. Y., Gao, L. M., Sun, G. H., Li, Y. P., & Wang, Y. B. (2007). Shuangjingzi Middle Triassic syn-collisional crust-derived granite in the East Inner Mongolia and its constraint on the timing of collision between Siberian and Sino-Korean paleo-plates. Acta Petrologica Sinica, 23(03), 565–582. (In Chinese with English abstract). https://doi.org/10.3321/j.issn:1000-0569.2007.03.004
- Li, S. C., Liu, Z. H., Xu, Z. Y., Li, G., & Zhang, C. (2015). Age and tectonic setting of volcanic rocks of the Tamulangou Formation in the Great Xing’an Range, NE China. Journal of Asian Earth Sciences, 113, 471–480. https://doi.org/10.1016/j.jseaes.2014.09.014
- Li, X., Zheng, Y. C., Yang, Z. S., Hou, Z. Q., Wu, C. D., Xu, P. Y., & Wang, L. (2022). Discovery of Miocene pegmatite type Be–Nb–Ta(–Rb) mineralization in the Yangbajain of Central Lhasa subterrane, Tibet, China. China Geology, 5(0), 1–3. https://doi.org/10.31035/cg2022037
- Liu, J., Li, Y., Zhou, Z. H., & Yang, H. G. (2017). The Ordovician igneous rocks with high Sr/Y at the Tongshan porphyry copper deposit, satellite of the Duobaoshan deposit, and their metallogenic role. Ore Geology Reviews, 86, 600–614. https://doi.org/10.1016/j.oregeorev.2017.02.036
- Liu, X. M., Chen, P. R., Wang, R. C., & Hu, H. (2003). Discrimination criteria and genesis for A type rock suites. Geological Journal of China Universities, 9(04), 573–591. (In Chinese with English abstract). https://geology.nju.edu.cn/EN/Y2003/V9/I4/573
- Ma, J. D., Wu, M. Q., Diao, X., He, D. Y., Cui, T., Long, Z. Y., & Qiu, K. F. (2023). Na-metasomatism and hematization implication of Zr and Nb–Be–REE mineralization: Case study of the Baerzhe deposit in Inner Mongolia. Acta Petrologica Sinica, 39(2), 432–444. https://doi.org/10.18654/1000-0569/2023.02.09
- Maniar, P. D., & Piccoli, P. M. (1989). Tectonic discrimination of granitoids. Geological Society of America Bulletin, 101(5), 635–643. https://doi.org/10.1130/0016-7606(1989)101<0635:TDOG>2.3
- Mao, J. W., Yuan, S. D., Xie, G. Q., Song, S. W., Zhou, Q., Gao, Y. B., Liu, X., Fu, X. F., Cao, J., Zeng, Z. L., Li, T. G., & Fan, X. Y. (2019). New advances on metallogenic studies and exploration on critical minerals of China in 21st century. Mineral Deposits, 38(05), 935–969. (In Chinese with English abstract). https://doi.org/10.16111/j.0258-7106.2019.05.001
- Miao, L. C., Fan, W. M., Liu, D. Y., Zhang, F. Q., Shi, Y. R., & Guo, F. (2008). Geochronology and geochemistry of the Hegenshan ophiolitic complex: Implications for late-stage tectonic evolution of the Inner Mongolia-Daxinganling Orogenic Belt, China. Journal of Asian Earth Sciences, 32(5-6), 348–370. https://doi.org/10.1016/j.jseaes.2007.11.005
- Miao, L. C., Liu, D. Y., Zhang, F. Q., Fan, W. M., Shi, Y. R., & Xie, H. Q. (2007). Zircon SHRIMP U–Pb ages of the “Xinghuadukou Group” in Hanjiayuanzi and Xinlin areas and the “Zhalantun Group” in Inner Mongolia, Da Hinggan Mountains. Chinese Science Bulletin, 52(8), 1112–1124. https://doi.org/10.1007/s11434-007-0131-2
- Middlemost, E. A. K. (1994). Naming materials in the magma/igneous rock system. Earth-Science Reviews, 37(3-4), 215–224. https://doi.org/10.1016/0012-8252(94)90029-9
- Nie, F., Sun, Z., Li, C., Liu, Y., Lv, K., Zhang, K., & Liu, Y. (2011). Re–Os isotopic dating of molybdenite separates from Chalukou Porphyry Mo polymetallic deposit in Heilongjiang Province. Mineral Deposits, 30(5), 828–836. (in Chinese with English abstract) https://doi.org/10.16111/j.0258-7106.2011.05.005
- Ostendorf, J., Jung, S., Berndt, G. J., & Hauff, F. (2014). Syn-orogenic high-temperature crustal melting: Geochronological and Nd–Sr–Pb isotope constraints from basement-derived granites (Central Damara Orogen, Namibia). Lithos, 192-195, 21–38. https://doi.org/10.1016/j.lithos.2014.01.007
- Pearce, J. A., Harris, N. B. W., & Tindle, A. G. (1984). Trace element discrimination diagrams for the tectonic interpretation of granitic rocks. Journal of Petrology, 25(4), 956–983. https://doi.org/10.1093/petrology/25.4.956
- Qiu, J. S., McInnes, B. I. A., Jiang, S. Y., & Hu, J. (2005). Geochemistry of the Minkengshan pluton in Huichang County, Jiangxi Province and new recognition about its genetic type. Geochimica, 34(01), 20–32. (In Chinese with English abstract). https://doi.org/10.3321/j.issn:0379-1726.2005.01.003
- Qiu, Z. L., Liang, D. Y., Wang, Y. F., Sun, Y., & Li, L. F. (2014). Zircon REE, trace element characteristics and U–Pb chronology in the Baerzhe alkaline granite: Implications to the petrological genesis and mineralization. Acta Petrologica Sinica, 30(06), 1757–1768. (In Chinese with English abstract).
- Ren, Y. S., Ju, N., Zhao, H. L., Wang, H., Hou, K. J., & Liu, S. (2012). Geochronology and geochemistry of metallogenetic porphyry bodies from Nongping Au–Cu deposit in Eastern Yanbian, NE China: Implications for depositional environment. Acta Geologica Sinica (English Edition), 86(3), 619–629. https://doi.org/10.1111/j.1755-6724.2012.00690.x
- Rudnick, R. L., & Gao, S. (2003). Composition of the continental crust. In H. D. Holland & K. K. Turekian (Eds.), Treatise on geochemistry, Vol. 3, The crust (pp. 1–64). Elsevier-Pergamon. https://doi.org/10.1016/b0-08-043751-6/03016-4
- Song, S. G., Wang, M. M., Xu, X., Wang, C., Niu, Y. L., Allen, M. B., & Su, L. (2015). Ophiolites in the Xing’an-Inner Mongolia accretionary belt of the CAOB: Implications for two cycles of seafloor spreading and accretionary orogenic events. Tectonics, 34(10), 2221–2248. https://doi.org/10.1002/2015TC003948
- Su, H. M., Jiang, S. Y., Zhu, X. Y., Duan, Z. P., Huang, X. K., & Zou, T. (2021). Magmatic-hydrothermal processes and controls on rare-metal enrichment of the Baerzhe peralkaline granitic pluton, inner Mongolia, northeastern China. Ore Geology Reviews, 131, 103984. https://doi.org/10.1016/j.oregeorev.2021.103984
- Sun, S. S., & McDonough, W. F. (1989). Chemical and isotopic systematics of oceanic basalts: Implications for mantle composition and processes. In A. D. Saunders & M. J. Norry (Eds.), Magmatism in the ocean basins (pp. 313–345). Geological Society London Special Publication 42. https://doi.org/10.1144/GSL.SP.1989.042.01.19
- Sun, W. D., Ding, X., Hu, Y. H., & Li, X. H. (2007). The golden transformation of the Cretaceous plate subduction in the west Pacific. Earth and Planetary Science Letters, 262(3-4), 533–542. https://doi.org/10.1016/j.epsl.2007.08.021
- Wang, D. H., Wang, R. J., Li, J. K., Zhao, Z., Yu, Y., Zheng, G. D., Li, X. S., Sun, Y., Li, D. X., & Zhao, D. (2012). The basic characteristics and research status of three mineral resources in China. Mineral Deposits, 31(S1), 41–42. (In Chinese with English abstract) https://doi.org/10.16111/j.0258-7106.2012.s1.023
- Wang, Y. J., Fan, W. M., Zhang, H. F., & Peng, T. P. (2006). Early Cretaceous gabbroic rocks from the Taihang Mountains: Implications for a paleo subduction-related lithospheric mantle beneath the central North China Craton. Lithos, 86(3-4), 281–302. https://doi.org/10.1016/j.lithos.2005.07.001
- Wang, Y. X., & Zhao, Z. H. (1997). Geochemistry and genesis of the Balzhe ultra-large rare earth niobium–beryllium–zirconium deposit. Geochimica, 26(01), 24–35. (In Chinese with English abstract). https://doi.org/10.19700/j.0379-1726.1997.01.003
- Watson, E. B., Wark, D. A., & Thomas, J. B. (2006). Crystallization thermometers for zircon and rutile. Contributions to Mineralogy and Petrology, 151(4), 413–433. https://doi.org/10.1007/s00410-006-0068-5
- Whalen, J. B., Currie, K. L., & Chappell, B. W. (1987). A-type granites: Geochemical characteristics, discrimination and petrogenesis. Contributions to Mineralogy and Petrology, 95(4), 407–419. https://doi.org/10.1007/BF00402202
- Windley, B. F., Alexeiev, D., Xiao, W., Kröner, A., & Badarch, G. (2007). Tectonic models for accretion of the Central Asian Orogenic Belt. Journal of the Geological Society, 164(1), 31–47. https://doi.org/10.1144/0016-76492006-022
- Wu, B., Wang, R. C., Guo, G. L., Li, C. X., & Song, Z. T. (2020). Compositional variations of rinkite in the Saima Alkaline Complex, Liaoning Province, and its implications for alkaline magma evolution. Earth Science, 45(02), 467–478. (In Chinese with English abstract).
- Wu, B., Wang, R. C., Liu, X. D., Guo, G. L., & Song, Z. T. (2018). Chemical composition and alteration assemblages of eudialyte in the Saima alkaline complex, Liaoning Province, and its implication for alkaline magmatic-hydrothermal evolution. Acta Petrologica Sinica, 34(006), 1741–1757. (In Chinese with English abstract). CNKI:SUN:YSXB.0.2018-06-012
- Wu, B., Wang, R. C., Yang, J. H., Wu, F. Y., Zhang, W. L., Gu, X. P., & Zhang, A. C. (2015). Wadeite (K2ZrSi3O9), an alkali-zirconosilicate from the Saima agpaitic rocks in northeastern China: Its origin and response to multi-stage activities of alkaline fluids. Lithos, 224-225, 126–142. https://doi.org/10.1016/j.lithos.2015.02.008
- Wu, B., Wang, R. C., Yang, J. H., Wu, F. Y., Zhang, W. L., Gu, X. P., & Zhang, A. C. (2016). Zr and REE mineralization in sodic lujavrite from the Saima alkaline complex, northeastern China: A mineralogical study and comparison with potassic rocks. Lithos, 262, 232–246. https://doi.org/10.1016/j.lithos.2016.07.013
- Wu, F. Y., Jahn, B. M., Wilde, S., & Sun, D. Y. (2000). Phanerozoic continental crustal growth: U–Pb and Sr–Nd isotopic evidence from the granites in northeastern China. Tectonophysics, 328(1-2), 89–113. https://doi.org/10.1016/S0040-1951(00)00179-7
- Wu, F. Y., Liu, X. C., Ji, W. Q., Wang, J. M., & Yang, L. (2017). Highly fractionated granites: Recognition and research. Scientia Sinica (Terrae), 47(7), 745–765. (in Chinese with English abstract). CNKI:SUN:JDXK.0.2017-07-001
- Wu, F-Y., Sun, D-Y., Ge, W-C., Zhang, Y-B., Grant, M. L., Wilde, S. A., & Jahn, B-M. (2011). Geochronology of the Phanerozoic granitoids in northeastern China. Journal of Asian Earth Sciences, 41(1), 1–30. https://doi.org/10.1016/j.jseaes.2010.11.014
- Wu, F. Y., Sun, D. Y., Li, H. M., Jahn, B. M., & Wilde, S. A. (2002). A-type granites in northeastern China: Age and geochemical constraints on their petrogenesis. Chemical Geology, 187(1-2), 143–173. https://doi.org/10.1016/S0009-2541(02)00018-9
- Wu, F. Y., Zhao, G. C., Sun, D. Y., Wilde, S. A., & Yang, J. H. (2007). The Hulan Group: Its role in the evolution of the Central Asian Orogenic Belt of NE China. Journal of Asian Earth Sciences, 30(3-4), 542–556. https://doi.org/10.1016/j.jseaes.2007.01.003
- Wu, G., Chen, Y. H., Sun, F. Y., Liu, J., Wang, G. R., & Xu, B. (2015). Geochronology, geochemistry, and Sr–Nd–Hf isotopes of the early Paleozoic igneous rocks in the Duobaoshan area, NE China, and their geological significance. Journal of Asian Earth Sciences, 97, 229–250. https://doi.org/10.1016/j.jseaes.2014.07.031
- Wu, G., Liu, R. L., Chen, G. Z., Li, T. G., Li, H. H., Li, Y. L., Yang, F., & Zhang, T. (2021). Mineralization of the Weilasituo rare metal–tin–polymetallic ore deposit in Inner Mongolia: Insights from fractional crystallization of granitic magmas. Acta Petrologica Sinica, 37(3), 637–664. (In Chinese with English abstract). https://doi.org/10.18654/1000-0569/2021.03.01
- Wu, M., Samson, I. M., Qiu, K., & Zhang, D. (2021). Concentration mechanisms of rare earth element–Nb–Zr–Be mineralization in the Baerzhe deposit, northeast China: Insights from textural and chemical features of arfvedsonite and rare metal minerals. Economic Geology, 116(3), 651–679. https://doi.org/10.5382/econgeo.4789
- Wu, S. P., Wang, M. Y., & Qi, K. J. (2007). Present situation of researchers on A-type granites: A review. Acta Petrologica et Mineralogica, 26(01), 57–66. (In Chinese with English abstract). https://doi.org/10.3969/j.issn.1000-6524.2007.01.009
- Wu, Y., Ju, N., Liu, X., Shi, L., Feng, Y. H., & Ma, D. Z. (2023). Source of metallogenic materials of the Saima alkaline rock-hosted niobium–tantalum deposit in the Liaoning region: Evidence from the Sr–Nd–Pb and Li isotopes. Minerals, 13(11), 1443–1464. https://doi.org/10.3390/min13111443
- Xiao, K., Xing, S., Bagas, L., Sun, L., Li, N., Yin, J., Cui, N., Cong, Y., Li, J., Chen, Y., & Ye, TZhu. (2017). The China national mineral assessment initiative. Ore Geology Reviews, 91, 1084–1093. https://doi.org/10.1016/j.oregeorev.2017.08.036
- Xiao, W. J., Windley, B. F., Hao, J., & Zhai, M. G. (2003). Accretion leading to collision and the Permian Solonker suture, Inner Mongolia, China: Termination of the central Asian orogenic belt. Tectonics, 22(6), 1069–1076. https://doi.org/10.1029/2002TC001484
- Xiao, W. J., Windley, B. F., Sun, S., Li, J. L., Huang, B. C., Han, C. M., Yuan, C., Sun, M., & Chen, H. L. (2015). A tale of amalgamation of three Permo-Triassic collage systems in Central Asia: Oroclines, sutures, and terminal accretion. Annual Review of Earth and Planetary Sciences, 43(1), 477–507. https://doi.org/10.1146/annurev-earth-060614-105254
- Xu, B., Jiang, S. Y., Wang, R., Ma, L., Zhao, K. D., & Yan, X. (2015). Late Cretaceous granites from the giant Dulong Sn–polymetallic ore district in Yunnan Province, South China: Geochronology, geochemistry, mineral chemistry and Nd–Hf isotopic compositions. Lithos, 218-219, 54–72. https://doi.org/10.1016/j.lithos.2015.01.004
- Xu, X. S., & Qiu, J. S. (2010). Igneous petrology (pp. 215–220). Science Press. (In Chinese with English abstract)
- Yang, J. H., Sun, J. F., Zhang, M., Wu, F. Y., & Wilde, S. A. (2012). Petrogenesis of silica-saturated and silica-undersaturated syenites in the northern North China Craton related to post-collisional and intraplate extension. Chemical Geology, 328, 149–167. https://doi.org/10.1016/j.chemgeo.2011.09.011
- Yang, J. H., Wu, F. Y., Xie, L. W., & Liu, X. M. (2007). Petrogenesis and implications of Kuanggdonggou syenltes in the Liaodong Peninsula, east North China Craton: Constraints from in-situ zircon U–Pb ages and Hf isotopes. Acta Petrologica Sinica, 23(02), 263–276. (In Chinese with English abstract). https://doi.org/10.3321/j.issn:1000-0569.2007.02.007
- Yang, W., Shan, Q., Zhao, Z., Luo, Y., Yu, X., Li, N., & Niu, H. (2011). Petrogenic and metallogenic action of the alkaline granitoids in Baerzhe area: A comparison between mineralized and barren plutons. Journal of Jilin University (Earth Science Edition), 41(6), 1689–1704. (In Chinese with English abstract) http://xuebao.jlu.edu.cn/dxb/EN/Y2011/V41/I6/1689
- Yang, W. B., Niu, H. C., Shan, Q., Sun, W. D., Zhang, H., Li, N. B., Jiang, Y. H., & Yu, X. Y. (2014). Geochemistry of magmatic and hydrothermal zircon from the highly evolved Baerzhe alkaline granite: Implications for Zr–REE–Nb mineralization. Mineralium Deposita, 49(4), 451–470. https://doi.org/10.1007/s00126-013-0504-1
- Yang, W. D., Niu, H. C., Shan, Q., Luo, Y., Yu, X. Y., & Qiu, Y. Z. (2009). Ore-forming mechanism of the Baerzhe super-large rare and rare earth elements deposit. Acta Petrologica Sinica, 25(11), 2924–2932. (In Chinese with English abstract). CNKI:SUN:YSXB.0.2009-11-023
- Yang, Z. M., Giester, G., Ding, K. S., & Tillmanns, E. (2012). Hezuolinite, (Sr,REE)4Zr(Ti,Fe3+,Fe2+)2Ti2O8–(Si2O7)2, a new mineral species of the chevkinite group from Saima alkaline complex, Liaoning Province, NE China. European Journal of Mineralogy, 24(1), 189–196. https://doi.org/10.1127/0935-1221/2011/0023-2158
- Zartman, R. E., & Doe, B. R. (1981). Plumbotectonics – The model. Tectonophysics, 75(1-2), 135–162. https://doi.org/10.1016/0040-1951(81)90213-4
- Zhai, G. M., Wu, F. Y., Hu, R. Z., Jiang, S. Y., Li, W. C., Wang, R. C., Wang, D. H., Qi, T., Qing, K. Z., & Wen, H. J. (2019). Critical metal mineral resources: Current research status and scientific issues. Bulletin of National Natural Science Foundation of China, 33(02), 106–111. (In Chinese with English abstract). https://doi.org/10.16262/j.cnki.1000-8217.2019.02.002
- Zhang, B., Qi, F. Y., Gao, X. Z., Li, X. L., Shang, Y. T., Kong, Z. Y., Jia, L. Q., Meng, J., Guo, H., Fang, F. K., Liu, Y. B., Jiang, X., Chai, H., Liu, Z., Ye, X. T., & Wang, G. D. (2022). Geological characteristics, metallogenic regularity, and research progress of lithium deposits in China. China Geology, 5, 734–767. https://doi.org/10.31035/cg2022054
- Zhang, H., Lu, Z. H., & Tang, Y. (2019). Metallogeny and prospecting model as well as prospecting direction of pegmatite-type rare metal ore deposits in Altay orogenic belt, Xinjiang. Mineral Deposits, 38(04), 792–814. (In Chinese with English abstract) https://doi.org/10.16111/j.0258-7106.2019.04.008
- Zhang, S., Ju, N., Zhang, G. B., Zhao, Y. D., Ren, Y. S., Liu, B. S., Wang, H., Guo, R. R., Yang, Q., Sun, Z. M., Xu, F. M., Wang, K. Y., & Hao, Y. J. (2023). Geology and mineralization of the Duobaoshan supergiant porphyry Cu–Au–Mo–Ag deposit (2.36 Mt) in Heilongjiang Province, China: A review. China Geology, 6(1), 100–136. https://doi.org/10.31035/cg2023006
- Zhang, S. H., Zhao, Y., Davis, G. A., Ye, H., & Wu, F. (2014). Temporal and spatial variations of Mesozoic magmatism and deformation in the North China craton: Implications for lithospheric thinning and decratonization. Earth-Science Reviews, 131, 49–87. https://doi.org/10.1016/j.earscirev.2013.12.004
- Zhang, S. H., Zhao, Y., Liu, J. M., & Hu, Z. C. (2016). Different sources involved in generation of continental arc volcanism: The Carboniferous–Permian volcanic rocks in the northern margin of the North China block. Lithos, 240-243, 382–401. https://doi.org/10.1016/j.lithos.2015.11.027
- Zhang, S. H., Zhao, Y., Song, B., Hu, J. M., Liu, S. W., Yang, Y. H., Chen, F. K., Liu, X. M., & Liu, J. (2009). Contrasting late Carboniferous and late Permian Middle Triassic intrusive suites from the northern margin of the North China Craton: Geochronology, petrogenesis, and tectonic implications. Geological Society of America Bulletin, 121(1-2), 181–200. https://doi.org/10.1130/B26157.1
- Zhang, Y., Sun, J. G., Xing, S. W., Zhao, K. Q., & Ma, Y. B. (2015). Geochronology and metallogenesis of porphyry Mo deposits in east-central Jilin province, China: Constraints from molybdenite Re–Os isotope systematics. Ore Geology Reviews, 71, 363–372. https://doi.org/10.1016/j.oregeorev.2015.06.014
- Zheng, J. P., Griffin, W. L., O’Reilly, S. Y., Yu, C. M., Zhang, H. F., Pearson, N., & Zhang, M. (2007). Mechanism and timing of lithospheric modification and replacement beneath the eastern North China Craton: Peridotitic xenoliths from the 100 Ma Fuxin basalts and a regional synthesis. Geochimica et Cosmochimica Acta, 71(21), 5203–5225. https://doi.org/10.1016/j.gca.2007.07.028
- Zhong, J., Fan, H. H., Chen, J. Y., Meng, Y. N., Zhao, J. Y., Shi, C. H., & Wang, Y. S. (2020). Geochemistry characteristics and 40Ar–39Ar age of biotite from the Saima aegirine-nepheline syenite and its geological significance. Earth Science, 45(01), 131–144. (In Chinese with English abstract). https://doi.org/10.3799/dqkx.2018.298
- Zhou, Z. B., Pei, F. P., Wang, Z. W., Cao, H. H., Xu, W. L., Wang, Z. J., & Zhang, Y. (2017). Using detrital zircons from Late Permian to Triassic sedimentary rocks in the south-eastern Central Asian Orogenic Belt (NE China) to constrain the timing of the final closure of the Paleo-Asian Ocean. Journal of Asian Earth Sciences, 144, 82–109. https://doi.org/10.1016/j.jseaes.2016.12.007
- Zhu, Y. S., Yang, J. H., Sun, J. F., Zhang, J. H., & Wu, F. Y. (2016). Petrogenesis of coeval silica-saturated and silica-undersaturated alkaline rocks: Mineralogical and geochemical evidence from the Saima Alkaline Complex, NE China. Journal of Asian Earth Sciences, 117, 184–207. https://doi.org/10.1016/j.jseaes.2015.12.014
- Zindler, A., & Hart, S. R. (1986). Chemical geodynamics. Annual Review of Earth and Planetary Sciences, 14(1), 493–571. https://doi.org/10.1146/annurev.ea.14.050186.002425