ABSTRACT
The outbreak and persistence of coronavirus disease 2019 (COVID-19) threaten human health. B cells play a vital role in fighting the infections caused by severe acute respiratory syndrome coronavirus 2 (SARS-CoV-2). Despite many studies on the immune responses in COVID-19 patients, it is still unclear how B cell receptor (BCR) constituents, including immunoglobulin heavy (IGHs) and light chains (IGLs), respond to SARS-CoV-2 in patients with varying symptoms. In this study, we conducted complementarity-determining region 3 (CDR3) sequencing of BCR IGHs and IGLs from the peripheral blood of COVID-19 patients and healthy donors. The results showed significantly reduced clonal diversity, more expanded clones, and longer CDR3 lengths of IGH and IGL in COVID-19 patients than those in healthy individuals. The IGLs had a much higher percentage of VJ skew usage (47.83% IGLV and 42.86% IGLJ were significantly regulated) than the IGHs (12.09% IGHV and 0% IGHJ) between the healthy individuals and patients, which indicated the importance of BCR light chains. Furthermore, we found a largely expanded IGLV3-25 gene cluster mostly pairing with IGLJ1 and ILGJ2 in COVID-19 patients and a newly identified upregulated IGLJ1 gene and IGLJ2+IGLV13-21 recombination, both of which are potential sources of SARS-CoV-2-targeting antibodies. Our findings on specific immune B-cell signatures associated with COVID-19 have clinical implications for vaccine and biomarker development for disease diagnosis.
Introduction
Since the outbreak of coronavirus disease 2019 (COVID-19), caused by severe acute respiratory syndrome coronavirus 2 (SARS-CoV-2), >446 million people have been infected and >5.99 million have died, as of March 2022 (Coronavirus Resource Center Citation2022). COVID-19 patients are classified into three categories – asymptomatic, mildly symptomatic, and severely symptomatic (Rothe et al. Citation2020; Shi et al. Citation2020; Wang et al. Citation2020; Wu and McGoogan Citation2020). Asymptomatic patients can transmit the disease despite having no symptomatic status (Zost et al. Citation2020), which increases the risk of infection. Although several protective vaccines are available, novel vaccines need to be developed because SARS-CoV-2 continues to mutate. An in-depth understanding of the human immune responses to SARS-CoV-2 will benefit the development of vaccines and therapeutics.
B cell receptors (BCRs) are essential components of the complex immune system of human body and aid in the defense against invading viruses and other pathogens. They comprise two immunoglobulin heavy chains (IGHs) and two light chains (IGLs). Each chain has a varied (V) region and a constant (c) region. During the early B-cell development, the V region is formed from the variable (V), diversity (D), and joining (J) gene segments (Giallourakis et al. Citation2010). Because of the V(D)J gene recombination, nucleotide insertion and deletion in the V region, and the combination of two BCR chains, the diversity of BCR repertoires is as high as 1011 (Glanville et al. Citation2009). Researchers unraveled the dynamics of the BCR repertoire in adaptive immunity during organ transplant (Pineda et al. Citation2019), vaccine injection (Davydov et al. Citation2018), cancer (Hu et al. Citation2019; Zhang et al. Citation2017), and disease infection, including SARS-CoV-2 infection (Li et al. Citation2021; Schultheiss et al. Citation2020; Wen et al. Citation2020), using high-throughput sequencing. Deep profiling of the complementarity-determining regions (CDR1, CDR2, and particularly the CDR3 region) in the V regions is gaining increasing attention as a convenient approach to investigate immune repertoire changes (Han et al. Citation2016). Large datasets and newly identified immune signature responses to SARS-CoV-2 were generated using BCR sequencing. Novel BCR changes, including IGHV3–23, IGHV3–7, IGHV3–15, IGHV3–30, and IGKV3–11, formerly used for virus vaccine development, have been identified (Wen et al. Citation2020). Schultheiss et al. generated a dataset of > 14 million T-cell receptor (TCR) and BCR sequences from COVID-19 patients and found that converging IGHV3-driven BCR clusters were closely associated with SARS-CoV-2 antibodies (Schultheiss et al. Citation2020). Li et al. obtained 6.9 million BCR clones from immune cells (Li et al. Citation2021). A set of 1254 convergent clonotypes of BCR (Galson et al. Citation2020) and 38 significantly expanded clonal lineage responses to SARS-CoV-2 were identified in patients with COVID-19 (Montague et al. Citation2021). Moreover, a recent study showed that asymptomatic patients lack strong BCR clonal expansion compared with moderate patients (Zhao et al. Citation2021). Patients with severe COVID-19 infection have stronger B-cell immune responses to SARS-CoV-2 infection, exhibiting more expanded BCR clones, increased clonal abundance, and reduced clonal diversity (Fan et al. Citation2020; Jin et al. Citation2021; Li et al. Citation2021; Niu et al. Citation2020; Zhang et al. Citation2020a, Citation2020b), which protect them from reinfection (Huang et al. Citation2021). However, these studies on B-cell immune responses in COVID-19 patients focused largely on the heavy chains and rarely on the light chains, which are often overlooked (Townsend et al. Citation2016).
In this study, we revealed the BCR repertoire characteristics of COVID-19 patients via next-generation sequencing of the CDR3 region from both the heavy and light chains. The BCR diversity, CDR3 length distribution, V(D)J usage, and clonotype expansion of IGH and IGL were compared between the recovered COVID-19 patients with mild and moderate symptoms and healthy individuals to characterize the B-cell immune signature responses to SARS-CoV-2 infection. The results showed reduced clonal diversity, longer CDR3 length, a higher percentage of VJ skew usage of IGL, an expanded IGLV3–25 gene cluster, and a newly identified upregulated IGLJ1 gene and IGLJ2/IGLV13–21 pair in COVID-19 patients. Our study provides new insights into the BCR repertoire, thereby contributing to a new clue for expediting COVID-19 vaccine development and antibody therapy.
Materials and methods
Cohort recruitment and sample collection
A cohort of patients who recovered after SARS-CoV-2 (strain Wuhan-Hu-1) infection was used as the case group (n = 15). The case cohort was further divided into two subgroups according to severity: mild (n = 4, asymptomatic or mild symptoms, ML1–4) and moderate (n = 11, moderate symptoms, MD1–11). Mild cases had no or only mild symptoms, and computed tomography (CT) imaging revealed no pneumonia. The moderate group had fever, respiratory symptoms, and pneumonia, as confirmed via CT imaging. A healthy donor cohort was used as the control group (n = 4, healthy, HC1–4). Peripheral blood samples were collected one day before discharge, and laboratory characteristics were measured on the second day in an isolation hospital.
RNA extraction and BCR sequencing
Human peripheral blood mononuclear cells (PBMCs) were isolated for next-generation sequencing of IGHs and IGLs of BCRs at the gene level. Total RNA was extracted using Trizol reagent (Invitrogen, Carlsbad, CA) following the protocol for the construction of human antibody library (Chen et al. Citation2009). RNA quality was assessed using an Agilent 2100 instrument (Agilent Technologies, Australia). BCR sequencing was performed on samples with RNA integrity number (RIN) ≥ 8.0 and total RNA content ≥300 ng. First-strand cDNA was synthesized using the SuperScript II enzyme (Thermo Fisher, Massachusetts, USA) according to the manufacturer’s instructions (incubation at 50°C for 50 min and 70°C for 15 min) (Zhang et al. Citation2017). To obtain complementarity-determining region 3 (CDR3) of the BCR IGHs and IGLs, two independent multiplex PCR assays were conducted using the QIAGEN Multiplex PCR Kit (QIAGEN, Valencia, CA) according to the manufacturer’s protocol. The multiple forward primers in the V region and reverse primers in the J region provided by Zhang et al. (Citation2015) were used for the multiplex PCR under the following conditions: 95°C for 15 min; 30 cycles of 94°C for 30 s, 60°C for 90 s, 72°C for 30 s; final extension at 72°C for 5 min. The PCR products were purified and screened using Agencourt AMPure XP beads at a ratio of 1:1 and then subjected to library preparation and BCR sequencing using the BGISEQ-500 platform (PE100). Sequence files of BCR CDR3 were deposited in the NCBI Sequence Read Archive under project accession number PRJNA813898.
Bioinformatic analysis
Raw BCR sequencing reads were preprocessed by trimming adapter sequences and low-quality reads (Q value ≤15 accounted for ≥50% of the reads and ≥10% N-containing reads) using SOAPnuke. The remaining clean reads were aligned and grouped to obtain clones using the MiXCR tool (3.0.8) (Bolotin et al. Citation2015). Non-productive reads and sequences with fewer than 2 read counts were excluded from further analysis. Each unique CDR3 nucleotide sequence is defined as a clone (clonotype). The IMGT library v3 was used as a reference database for alignment and annotation. Clone diversity was estimated using the Shannon-Wiener index, which is a commonly used measure of diversity (Cui et al. Citation2018; Jin et al. Citation2021; Omayio et al. Citation2019). The higher the Shannon index, the more diverse the clones are. Further statistics and plotting within groups were conducted using VDJtools (Shugay et al. Citation2015).
To select the B-cell immune gene cluster response to SARS-CoV-2 immunization, we conducted a repertoire-wide evolutionary analysis using a phylogenetic tree. The top 20 abundant clonotypes of each sample from healthy controls and mild and moderate patients were used to construct phylogenetic trees using the maximum-likelihood method in MEGA software (version 11) (Tamura et al. Citation2021). The Newick format result was used to construct a phylogenetic tree using iTOL v6 (https://itol.embl.de/) (Letunic and Bork Citation2021). Only B-cell clusters derived from at least 10 unique BCR clonotypes as well as at least two mild and five moderate patients were considered potential sources of SARS-CoV-2-targeting antibodies and were selected for further analysis.
Statistical analysis and graphing
Wilcoxon signed-rank sum test was used for pairwise comparisons. The Kruskal-Wallis test was used to compare three groups. Statistical analysis and plotting were performed using the R software.
Results
Laboratory characteristics of cohorts
Fifteen COVID-19 patients and four healthy controls were recruited, and their PBMCs were isolated for IGH and IGL sequencing (). Patients with COVID-19 were further divided into mild and moderate groups according to disease symptom severity. The mild group comprised four patients without lung lesions, while the moderate group included 11 patients with typical radiological changes in the lung. The average age of the healthy individuals was 38.5, and 74% were women. The average age of the mild and moderate patients was 31.5 (75% female) and 40.6 years (55% female), respectively (). The leukocyte and neutrophil granulocyte counts were significantly lower in the moderate group than in the mild group. The average lymphocyte count was lower in moderate patients, which is consistent with lymphopenia in COVID-19 patients with a more serious status (Galson et al. Citation2020; Tavakolpour et al. Citation2020). Immunoglobulin A (IgA) and IgM concentrations were reduced in the moderate group, but IgG levels showed no difference between the moderate and mild groups. There was a slight reduction in CD3+ and CD8+ T cell counts, a slight increase in CD4+ T cells in the moderate, and an increase in the proportion of CD4+ and CD8+ T cells ().
Figure 1. Overview of BCR repertoire characterization and features of COVID-19 cohorts. (a) Schematic diagram of overall study design. (b) Laboratory characteristics differences between the mild and moderate COVID-19 cohorts. The total number of leukocytes, neutrophil granulocytes, lymphocytes, CD3+, CD4+, and CD8+ T cells in the blood, the ratio of CD4+:CD8+T cells, and immunoglobulin (Ig) levels including IgA, IgM and IgG in recovered COVID-19 patients with the mild or moderate symptom. The Wilcoxon rank-sum test was used to determine the statistical significance of the characteristics.
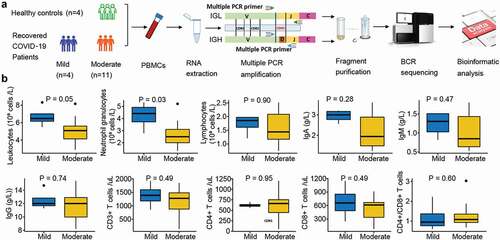
Table 1. Basic information of cohorts in this study.
Overview of BCR diversity
Next-generation sequencing of BCR IGHs yielded a total of 53.59 Gbps raw reads, of which 99.73% (53.44 Gbps) were considered as clean reads after read quality trimming. Clustering of the clean reads generated 278,886–1,114,993 clonotypes per sample (Table S1). The Shannon–Wiener index revealed that IGH diversity was lower in the mild group patients than in the healthy controls (P < .05, ). A total of 14,811 IGH clonotypes were detected in at least two healthy samples, and 30 IGH clonotypes were commonly expressed in four samples from the healthy group (). In the mild cohorts, 1,929 clonotypes were detected in at least two samples, and six were shared in all the four mild group patients (). Regarding the moderate group, 37,422 clonotypes were expressed in at least two samples, but none were shared among the 11 COVID-19 samples. The length of IGH CDR3 (HCDR3) ranged from 4–47 amino acids (AA); 7–24 AA length was more common (frequency > 0.1%). The most frequent HCDR3 length was 16 AA in healthy individuals and the mild and moderate group patients. The frequency of HCDR3 length with ≥17 AA was higher in COVID-19 patients than in healthy controls and increased with disease severity (). Conversely, the frequency of HCDR3 length ≤16 AA was lower in COVID-19 patients than in healthy controls. Overall, the average length of HCDR3 was higher in COVID-19 patients than in healthy individuals and increased with disease severity (P = .05, ).
Figure 2. Diversity of the heavy and light chains of BCR repertoire in COVID-19 patients. (a) Violin plot of Shannon Wiener-Index representing the clonal diversity of the heavy chain in healthy individuals, mild and moderate COVID-19 patients. The Wilcoxon rank-sum test was used to determine statistical significance. (b) Venn diagram of expressed clonotypes of the heavy chain in healthy controls (HC) and mild patients (Mild). (c) the frequency distribution of the length of the heavy chain CDR3 region. The Kruskal-Wallis test was used to determine the statistical significance of the average length distribution across different cohorts. (d) Violin plot of Shannon Wiener Index representing the clonal diversity of the light chain. (e) Venn diagram of expressed clonotypes of the light chain in HC and Mild groups. (f) the frequency distribution of the length of the light chain CDR3 region.
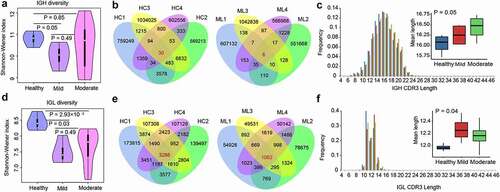
BCR light chain sequencing yielded 15.22 Gbps raw reads, of which 99.66% were clean reads (15.17 Gbps). Additionally, 32,292–192,390 clonotypes were identified in each sample (Table S2). The Shannon-Wiener index showed that IGL diversity decreased in the moderate and mild groups compared to that in healthy individuals (P < .05, ); however, no differences were observed between the mild and moderate groups. A total of 3,286, 1,062, and 393 common clonotypes from IGL were detected in the healthy, mild (), and moderate groups, respectively. The length of IGL CDR3 (LCDR3) ranged from 4–46 AA and was highly distributed (>0.1%) in 7–17 AA (). The most frequent LCDR3 length was 11 AA in healthy individuals and 13 AA in the mild and moderate groups. The frequency of LCDR3 length ≥12 AA was higher in COVID-19 patients than in the healthy controls, but the opposite was true for LCDR3 length ≤11 AA (). The average LCDR3 length increased in patients with moderate or mild COVID-19 compared with that in healthy individuals (P = .04, ).
VJ gene usage
The diversity of the BCR repertoire mainly results from VJ gene recombination. To reveal the unique VJ gene patterns and preferences for IGHs and IGLs in COVID-19 patients, we compared the expression of VJ genes among the participants. A total of 91 IGHV and six IGHJ genes were identified. The top 20 abundant IGHV genes (with an average frequency >2%) (), including the two most abundant IGHV1-18 and IGHV1-69 genes, accounted for 85–90% of the expression, with significant increase (P < .05) in COVID-19 patients compared to that in healthy individuals (). Among other IGHV genes with lower frequencies (<2%), IGHV3-15, IGHV3-72, IGHV4-28, and IGHV7-81 were significantly downregulated in COVID-19 patients, while IGHV4/OR15-8, IGHV4/OR15-7, IGHV3/OR16-15, IGHV3-54, and IGHV3-16 were significantly upregulated (P < .05, ). These contributed to a total of 12.09% significantly regulated IGHV genes, including 7.69% upregulated and 4.40% downregulated genes. The most abundant IGHJ gene was IGHJ4 (average frequency of 53.58%), followed by IGHJ5, IGHJ6, IGHJ3, IGHJ2, and IGHJ1 in the decreasing order (). There were no significant alterations in the expression of any of the IGHJ genes between healthy individuals and COVID-19 patients.
Figure 3. V and J gene usage of the heavy and light chains of BCR repertoire among healthy controls, mild and moderate COVID-19 patients. (a) Frequency distribution of overall IGHV-gene usage. (b) Heatmap of IGHV genes with significant differences (P < .05) between healthy individuals and COVID-19 patients. The Kruskal-Wallis test was used to determine statistical significance. (c) Frequency distribution of IGHJ-gene usage (d) Frequency distribution of IGLV-gene usage. (e) Heatmap of IGLV genes with significant differences (P < 0.05) between healthy individuals and COVID-19 patients. (f) Frequency distribution of IGLJ-gene usage. (g) Heatmap of IGLJ genes with significant differences (P < 0.05) between healthy individuals and COVID-19 patients. The gene name in red denotes up-regulated genes in the top 20 abundant gene types in COVID-19 patients.
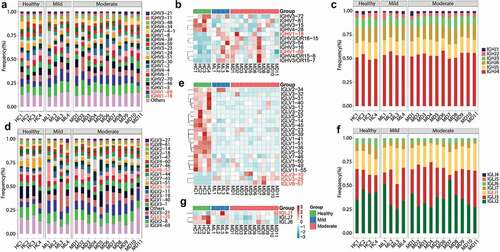
Furthermore, 46 IGLV and seven IGLJ genes were detected. The top 20 most abundant IGLV genes showed 87–97% abundance, with IGLV4-69 and IGLV2-8 being the most expressed ones (>10%, ). In particular, 22 (47.83%) IGLV genes, including 19 downregulated and three upregulated genes (P < .05, ), showed significantly different segment usages in mild or moderate COVID-19 patients compared to those in the healthy controls. Three upregulated IGLV genes (IGLV3-25, IGLV3-10, and IGLV6-57) were also included in the top 20 most abundant ones. Four IGLV genes (IGLV1-40, IGLV1-51, IGLV7-46, and IGLV2-14) were significantly downregulated within the top 20 most abundant genes and the other 15 IGLV genes were present in low frequency (<1%) (). In the decreasing order, seven IGLJ genes were IGLJ2, IGLJ3, IGLJ1, IGLJ7, IGLJ6, IGLJ5, and IGLJ4 (). Three of them (42.86%), including the upregulated IGLJ1 and the downregulated IGLJ7 and IGLJ6 (P < .05, ), showed significant regulation in COVID-19 patients.
Clonotype expansion and constitution
Immune clonal expansion is a direct consequence of the SARS-CoV-2 infection. To further investigate the features of expanded clonotypes, we first divided them into two types – low (2–10) and high (≥11) expansion. By comparing the expanded clonotypes between healthy individuals and COVID-19 patients, we found that the low-expanded clonotypes from IGH significantly decreased in the mild group, while the high-expanded clonotypes were enhanced in the mild group (P < 0.05, ). Similar results were observed for the IGL clonotypes (). Next, we examined the conserved sequence features in COVID-19 patients by selecting the top 20 most frequent BCR clonotypes from healthy controls and the mild and moderate groups to conduct phylogenetic sequence analysis. At least 10 unique BCR clonotypes and at least two mild and five moderate patients were considered potential sources of SARS-CoV-2-targeting antibodies. The phylogenetic tree revealed that the IGLV3-25 gene cluster was significantly expanded in COVID-19 patients (). IGLV3-25 was mostly rearranged with joining regions of the IGLJ1, IGLJ2, and IGLJ3 gene segments ().
Figure 4. Features of expanded clones of B-cell immune repertoires in COVID-19 patients. (a) Comparison of IGH and (b) IGL clonal expansion among the healthy, mild and moderate in low (clones with count between 2 and 10)and high expanded (clones with count ≥ 11) types. The Wilcoxon rank-sum test was used to determine the statistical significance of the frequency across different groups. (b) B cell repertoire-wide phylogenetic tree analysis in the healthy, mild, and moderate. The top 20 clones from each IGL repertoire were analyzed.
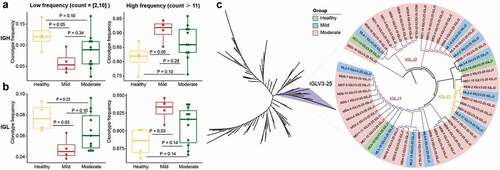
The VJ gene pairing comprises the basic structure of functional immune clones. To study biased V(D)J pairing during disease recovery, we focused on the top five V gene pairings of the most frequently used J genes from both heavy (IGHJ4) and light (IGLJ2) chains. We found that the top five paired IGHV genes of IGHJ4 varied between COVID-patients and the healthy controls. Regarding IGH, the healthy individuals possessed more IGHJ4/IGHV2–70 and IGHJ4/IGHV4–4 combinations, while the mild and moderate COVID-19 patients overexpressed the IGHJ4/IGHV1–24 pairs (). Regarding IGL, the healthy individuals expressed the five most highly expressed IGLJ2/IGLV1-51 and IGHJ2/IGHV1-40 genes, while the mild and moderate COVID-19 patients were over-represented by the IGLJ2/IGLV13–21 pairs (). Moreover, there was a difference in the top five paired IGLV genes of IGHJ2 between the mild and moderate groups – the mild group harbored IGLJ2/IGLV13–19, whereas the moderate group possessed IGLJ2/IGLV3–1 ().
Discussion
COVID-19 has caused numerous deaths globally and poses major health risks. The humoral immune system plays a vital role in disease resistance and recovery. A robust B-cell response and virus-specific antibodies can be detected several days after infection (Vabret et al. Citation2020). Further research into the BCR repertoire in COVID-19 patients is needed to understand the immune response, which is essential for both therapy and vaccine development. In this study, we compared the differences in the BCR repertoire between healthy individuals (n = 4) and COVID-19 patients with mild (n = 4, mild group) and moderate (n = 11, moderate group) symptoms using BCR sequencing of the CDR3 region on both the heavy and light chains. Our results revealed the characteristics of immune repertoire responses to SARS-CoV-2, including IGH and IGL clonotype expansion, reduction in diversity, increase in CDR3 length, dominant skew usage of IGL genes, and some newly identified upregulated genes in COVID-19 patients.
The individuals with advanced age are more vulnerable to SARS-CoV-2 infection and show different BCR repertoires with lower levels of somatic hypermutation and a less predominant IgA isotype than younger individuals (Bieberich et al. Citation2021). Together, different immune responses to vaccines between the elderly and young (Collier et al. Citation2021) indicate that age is related to the SARS-CoV-2 immune responses. The minimal age disparities between the mild and moderate groups suggested that age was not a factor affecting BCR differences between the mild and moderate groups in this study. The reduced clonal diversity and more expanded clones with a high frequency (clone count ≥11) of IGH and IGL commonly indicated an expansion of BCR clonotypes in COVID-19 patients, which was consistent with the results of previous studies (Fan et al. Citation2020; Jin et al. Citation2021; Li et al. Citation2021; Niu et al. Citation2020; Zhang et al. Citation2020a, Citation2020b). Earlier studies on disease durations showed that COVID-19 patients with moderate or severe clinical courses displayed higher BCR clonal expansion than patients with an asymptomatic clinical course, which is associated with the antibody-secreting B cells that produce antibodies to neutralize the infecting pathogen (Montague et al. Citation2021; Zhao et al. Citation2021). The diversity and richness of IGH repertoires increased slightly in the recovered group compared to those in patients with active COVID-19 (Schultheiss et al. Citation2020). In the recovery stage, there is dominant B-cell clonal expansion with reduced diversity (Niu et al. Citation2020). For example, the dominant BCR clonotypes (top 3) found in 10–30% of COVID-19 patients rapidly decreased as clinical symptoms improved (Funakoshi et al. Citation2021), as demonstrated by the biased usage of IGHV3–23 and IGHV3–7 (Wen et al. Citation2020) and a converging IGHV3-driven BCR cluster (Schultheiß et al. Citation2020) in COVID-19 patients. Moreover, COVID-19 patients in the early recovery stage have a higher percentage of expanded B cell clonotypes than those in the later recovery stage (Wen et al. Citation2020). These results suggest that BCR clonotypes probably expand their unique clonal VDJ rearrangements with increased expression over the course of COVID-19. The less commonly shared IGH clonotypes compared to IGL clones in COVID-19 patients and the healthy controls revealed more frequent changes in the heavy chains of BCRs. The differences in CDR3 region length between COVID patients and healthy individuals suggest that SARS-CoV-2 results in the selection or persistence of B cells expressing antibodies with longer IGH and IGL lengths. Light chains are largely rearranged and play active roles in the antigen selection process in chronic lymphocytic leukemia (CLL) (Blachly et al. Citation2015; Kostareli et al. Citation2010; Stamatopoulos et al. Citation2005, Citation2018) as well as hepatitis C virus (HCV)-associated B-cell lymphoproliferative disorders (Defrancesco et al. Citation2021; Minafò et al. Citation2020). For example, patients with CLL and IGLV3–21 light chain have poor prognoses (Stamatopoulos et al. Citation2018). The mutated IGL3-21R110 is a tumor promoter involved in the malignant transformation of the aggressive CLL subset (Paschold et al. Citation2022). Our results highlighted the functions of overlooked IGL and IGH, both of which play important roles in defending SARS-CoV-2. In contrast, Jin et al. (Jin et al. Citation2021) found no significant differences in the light chains between healthy individuals and COVID-19 patients. The inconsistency may be due to an insufficient sample size; thus, larger cohorts are needed for further analyses.
The specific VJ pair usage in COVID-19 patients indicates that B cells might have undergone unique and specific V(D)J rearrangements in response to the SARS-CoV-2 infection. We observed skewed usage of 11 (12.09%) IGHV genes, as previously reported (Nielsen et al. Citation2020). However, none of the IGHJ genes were skewed. It has been demonstrated that the most abundant and upregulated IGHV1-18, which is an antibody gene expressed by naive B cell clones, has higher frequencies to preferentially recognize the viral S protein during SARS-CoV-2 infection (Jennewein et al. Citation2021). The IGHV1-69 genes are the most frequently used IGHV genes in CLL B cells (Messmer et al. Citation2004) and exhibit increased usage in HCV-infected patients (Tucci et al. Citation2018). Influenza virus recognition and neutralization depend solely on the heavy chains of antibodies, which are largely derived from the IGHV1-69 gene, and have facilitated the development of universal influenza vaccines, as they target the highly conserved stem region of influenza hemagglutinin (HA) (Lingwood et al. Citation0000). In addition, IGHV1–69 B-CLL antibodies are reactive to HIV-1 envelope gp41, HCV E2 protein, and intestinal commensal bacteria (Hwang et al. Citation2014). Variants of the IGHV1-69 gene play a dominant role in protective natural antibody responses to the SARS-CoV-2 virus (Panovska-Stavridis et al. Citation2021). The abundant and significant increase in IGHV1–69 expression in COVID-19 patients further suggests its critical role in disease recovery during various viral infections, including SARS-CoV-2. In our study, the monoclonal antibody COV2–2199, comprising the IGHJ4/IGHV1–24 recombination (Voss and Hou et al. Citation2020; Zost and Gilchuk et al. Citation2020), was found to be overexpressed in COVID-19 patients.
However, many more V (22 IGLV, 47.83%) and J (3 IGLJ, 42.86%) genes from IGL displayed significantly different usage between the healthy individuals and COVID-19 patients, which indicated a critical role of IGL in the immune response to SARS-CoV-2. An increased frequency of IGLV3-10-encoded antibodies has been reported in COVID-19 patients (Leng et al. Citation2021). The highly upregulated IGLV6-57 gene is frequently used by various SARS-CoV-2 antibodies (Wang et al. Citation2021). IGLV3-25 was reported to be upregulated in the peripheral blood of patients with early symptomatic COVID-19 (Howell et al. Citation2021; McClain et al. Citation2020). We observed a huge expansion of the IGLV3-25 gene cluster at the immune repertoire level. The dominant pairing genes of IGLJ1 and IGLJ2 indicate a shared imprint of convergent B-cell response to SARS-CoV-2, which was newly identified. The strongest pairing frequencies from the light chains IGLJ2/IGLV13–21 indicated a monoclonal state associated with SARS-CoV-2 specificity, which has not previously been reported. These upregulated IGH and IGL genes may serve as potential markers for COVID-19 patients and may facilitate vaccine design.
Although we could not collect samples from patients with different severities before and during the onset of the disease, our study systematically characterized the light and heavy chains of the BCR repertoire in recovered COVID-19 patients infected with the same SARS-CoV-2 strain. With new variants identified, further investigation is needed to determine whether different SARS-CoV-2 variants have different BCR clones. Notably, in this study, we analyzed the overview of BCR from all B cells. The subsets of B-1 (B-1a and B-1b) and B-2 B cells may acquire different BCR properties (Kantor et al. Citation1997). The B-1a B cell subset contributes to an innate immune response (Montecino-Rodriguez and Dorshkind Citation2006; Sindhava and Bondada Citation2012) by generating a repertoire that is biased toward bacteria and self-antigens (Wong et al. Citation2019). During SARS-CoV-2 infection, the B-1a cells are likely to generate antibodies with reduced junctional diversity and fewer somatic hypermutations than B-2 B cells. Owing to the small sample size, the statistical power of the data must be interpreted with caution. Furthermore, large population cohort studies are needed to obtain more details on both chains of the specific B-cell immune response in COVID-19 patients in the future. In summary, our research provides a comprehensive understanding of the heavy and light chains in COVID-19 patients and critical insights into the immune responses and development of new vaccines and therapeutic strategies against COVID-19.
Ethics statement
The blood samples were provided by the Second Affiliated Hospital of Fujian Medical University (Quanzhou, Fujian, China) with the approval of the institutional research board and the donors’ consent. Procedures followed in this study were under the ethical standards of concerned institutional policies.
Supplemental Material
Download PDF (17.2 KB)Supplemental Material
Download PDF (17.2 KB)Acknowledgments
We wish to thank Yichuang Lin for his assistance in sample information collection.
Disclosure statement
No potential conflict of interest was reported by the author(s).
Supplementary material
Supplemental data for this article can be accessed online at https://doi.org/10.1080/08820139.2022.2092407.
Additional information
Funding
References
- Bieberich F, Vazquez-Lombardi R, Yermanos A, Ehling RA, Mason DM, Wagner B, Kapetanovic E, Di Roberto RB, Weber CR, and Savic M, et al. 2021. A Single-cell atlas of lymphocyte adaptive immune repertoires and transcriptomes reveals age-related differences in convalescent COVID-19 patients. Front Immunol. 12:701085.
- Blachly JS, Ruppert AS, Zhao W, Long S, Flynn J, Flinn I, Jones J, Maddocks K, Andritsos L, Ghia M, et al. 2015. Immunoglobulin transcript sequence and somatic hypermutation computation from unselected RNA-seq reads in chronic lymphocytic leukemia. Proc Natl Acad Sci USA. 112(14):4322–27.
- Bolotin D, Poslavsky S, Mitrophanov I, Shugay M, Mamedov IZ, Putintseva EV, Chudakov DM. 2015. MiXCR: Software for comprehensive adaptive immunity profiling. Nat Methods. 12(5):380–81.
- Chen W, Zhu Z, Xiao X, Dimitrov DS. 2009. Construction of a human antibody domain (VH) library. Methods Mol Biol. 525:81–99.
- Collier DA, Ferreira IATM, Kotagiri P, Datir RP, Lim EY, Touizer E, Meng B, Abdullahi A, Baker S, Dougan G, et al. 2021. Age-Related immune response heterogeneity to SARS-CoV-2 vaccine BNT162b2. Nature. 596(7872):417–22.
- Coronavirus Resource Center. 2022. [accessed 2022 Mar 7]. https://coronavirus.jhu.edu/map.html.
- Cui J-H, Lin KR, Yuan SH, Jin YB, Chen XP, Su XK, Jiang J, Pan YM, Mao SL, Mao XF, et al. 2018. TCR repertoire as a novel indicator for immune monitoring and prognosis assessment of patients with cervical cancer. Front Immunol. 9:2729.
- Davydov AN, Obraztsova AS, Lebedin MY, Turchaninova MA, Staroverov DB, Merzlyak EM, Sharonov GV, Kladova O, Shugay M, Britanova OV, et al. 2018. Comparative analysis of B-cell receptor repertoires induced by live yellow fever vaccine in young and middle-age donors. Front Immunol. 9:2309.
- Defrancesco I, Visentini M, Zibellini S, Minafò YA, Rattotti S, Ferretti VV, Rizzo E, Varettoni M, Frigeni M, Pulsoni A, et al. 2021. Mutational and immunogenetic landscape of HCV -associated B-cell lymphoproliferative disorders. Am J Hematol. 96(6):E210–E214.
- Fan X, Liu Z, Poulsen KL, Wu X, Miyata T, Dasarathy S, Rotroff DM, Nagy LE. 2020. Alcohol Consumption is Associated with Poor Prognosis in Obese Patients with COVID-19: a Mendelian Randomization Study using UK Biobank. medRxiv: The preprint server for health sciences. 2020.05.24.20101238. doi:10.1101/2020.11.25.20238915.
- Funakoshi Y, Ohji G, Yakushijin K, Ebisawa K, Arakawa Y, Saegusa J, Matsumoto H, Imanishi T, ukuda E, Matsutani T, et al. 2021. Massive surge of mRNA expression of clonal B-cell receptor in patients with COVID-19. Heliyon. 7(8):e07748.
- Galson JD, Schaetzle S, Bashford-Rogers Rachael J.M., Raybould Matthew I.J., Kovaltsuk A., Kilpatrick Gavin J, Minter R, Finch Donna K, Dias J, James Louisa K, et al. 2020. Deep sequencing of B cell receptor repertoires from COVID-19 patients reveals strong convergent immune signatures. Front Immunol 11:605170.
- Giallourakis CC, Franklin A, Guo C, Cheng HL, Yoon HS, Gallagher M, Perlot T, Andzelm M, Murphy AJ, Macdonald LE, et al. 2010. Elements between the IgH variable (V) and diversity (D) clusters influence antisense transcription and lineage-specific V(D)J recombination. Immunol Inflamm. 107(51):22207–12.
- Glanville J, Zhai W, Berka J, Telman D, Huerta G, Mehta GR, Ni I, Mei L, Sundar PD, Day GM, et al. 2009. Precise determination of the diversity of a combinatorial antibody library gives insight into the human immunoglobulin repertoire. Biochemistry. 106(48):20216–21.
- Han Y, Li H, Guan Y, and Huang J. 2016. Immune repertoire: a potential biomarker and therapeutic for hepatocellular carcinoma. Cancer Lett. 379(2):206–212.
- Howell MC, Green R, McGill AR, Kahlil RM, Dutta R, Mohapatra SS, Mohapatra S. 2021. Activation of intracellular complement in lungs of patients with severe COVID-19 disease decreases T-Cell activity in the lungs. Front Immunol. 12:700705.
- Hu X, Zhang J, Wang J, Fu J, Li T, Zheng X, Wang B, Gu S, Jiang P, Fan J, et al. 2019. Landscape of B cell immunity and related immune evasion in human cancers. Nat Genet. 51(3):560–67.
- Huang L, Shi Y, Gong B, Jiang L, Zhang Z, Liu X, Yang J, He Y, Jiang Z, and Zhong L, et al. 2021. Dynamic blood single-cell immune responses in patients with COVID-19. Signal Transduct Target Ther. 110.
- Hwang K-K, Trama AM, Kozink DM, Chen X, Wiehe K, Cooper AJ, Xia S-M, Wang M, Marshall DJ, Whitesides J, et al. 2014. IGHV1-69 B cell chronic lymphocytic leukemia antibodies cross-react with HIV-1 and Hepatitis C Virus antigens as well as intestinal commensal bacteria. PLoS ONE. 9(3):e90725.
- Jennewein MF, MacCamy AJ, Akins NR, Feng J, Homad LJ, Hurlburt NK, Seydoux E, Wan Y-H, Stuart AB, Edara VV, et al. 2021. Isolation and characterization of cross-neutralizing coronavirus antibodies from COVID-19+ subjects. Cell Rep. 36(2):109353.
- Jin X, Zhou W, Luo M, Wang P, Xu Z, Ma K, Cao H, Xu C, Huang Y, Cheng R, et al. 2021. Global characterization of B cell receptor repertoire in COVID-19 patients by single-cell V(D)J sequencing. Brief Bioinform. 22(6). doi:10.1093/bib/bbab192.
- Kantor AB, Merrill CE, Herzenberg LA, Hillson JL. 1997. An unbiased analysis of V(H)-D-J(H) sequences from B-1a, B-1b, and conventional B cells. J Immunol. 158(3):1175–86.
- Kostareli E, Sutton L-A, Hadzidimitriou A, Darzentas N, Kouvatsi A, Tsaftaris A, Anagnostopoulos A, Rosenquist R, Stamatopoulos K. 2010. Intraclonal diversification of immunoglobulin light chains in a subset of chronic lymphocytic leukemia alludes to antigen-driven clonal evolution. Leukemia. 24(7):1317–24.
- Leng L, Li M, Li W, Mou D, Liu G, Ma J, Zhang S, Li H, Cao R, Zhong W, et al. 2021. Sera proteomic features of active and recovered COVID-19 patients: potential diagnostic and prognostic biomarkers. Signal Transduction Targeted Ther. 6(1):216.
- Letunic I, Bork P. 2021. Interactive Tree of Life (iTOL) v5: an online tool for phylogenetic tree display and annotation. Nucleic Acids Res. 49(W1):W293–W296.
- Li F, Luo M, Zhou W, Li J, Jin X, Xu Z, Juan L, Zhang Z, Li Y, Liu R, et al. 2021. Single cell RNA and immune repertoire profiling of COVID-19 patients reveal novel neutralizing antibody. Protein Cell. 12(10):751–55.
- Lingwood D, McTamney PM, Yassine HM, Whittle JR, Guo X, Boyington JC, Wei CJ, Nabel GJ. 2012. Structural and genetic basis for development of broadly neutralizing influenza antibodies. Nature. 489(7417):566–570
- McClain MT, Constantine FJ, Henao R, Liu Y, Tsalik EL, Burke TW, Steinbrink JM, Petzold E, Nicholson BP, Rolfe R, et al. 2020. Dysregulated transcriptional responses to SARS-CoV-2 in the periphery support novel diagnostic approaches. medRxiv: The preprint server for health sciences. 2020.07.20.20155507.
- Messmer BT, Albesiano E, Messmer D, Chiorazzi N. 2004. The pattern and distribution of immunoglobulin VH gene mutations in chronic lymphocytic leukemia B cells are consistent with the canonical somatic hypermutation process. Blood. 103(9):3490–95.
- Minafò YA, Del Padre M, Cristofoletti C, Caprini E, Perez M, Aranburu A, Colantuono S, Gragnani L, Zignego AL, Pulsoni A, et al. 2020. A stereotyped light chain may shape virus-specific B-cell receptors in HCV-dependent lymphoproliferative disorders. Genes Immun. 21(2):131–35.
- Montague Z, Lv H, Otwinowski J, DeWitt WS, Isacchini G, Yip GK, Ng WW, Tsang OTY, Yuan M, Liu H, et al. 2021. Dynamics of B cell repertoires and emergence of cross-reactive responses in patients with different severities of COVID-19. Cell Rep. 35(8):109173.
- Montecino-Rodriguez E, Dorshkind K. 2006. New perspectives in B-1 B cell development and function. Trends Immunol. 27(9):428–33.
- Nielsen SCA, Yang F, Hoh RA, Jackson KJ, Roeltgen K, Lee JY, Rustagi A, Rogers AJ, and Powell AE, et al. 2020. B cell clonal expansion and convergent antibody responses to SARS-CoV-2. Res Square 1 . rs.3.rs–27220.
- Niu X, Li S, Li P, Pan W, Wang Q, Feng Y, Mo X, Yan Q, Ye X, Luo J, et al. 2020. Longitudinal analysis of T and B Cell receptor repertoire transcripts reveal dynamic immune response in COVID-19 patients. Front Immunol. 11:582010.
- Omayio D, and Mzungu E . 2019. Modification of shannon-wiener diversity index towards quantitative estimation of environmental wellness and biodiversity levels under a non-comparative scenario. J Environ and Earth Sci. 9(9):46–57.
- Panovska-Stavridis I, Ridova N, Stojanovska S, Stevanovic M, Stojanoska T, Demiri I, Matevska-Geshkovska N, Vujovic M, Markoska H, Filipce V, et al. 2021. Prevalence of allelic variants and clonality of IGHV1-69 expressing B-cells in patients with different severity of COVID 19 disease. Blood. 138(Suppl 1):994.
- Paschold L, Simnica D, Brito RB, Zhang T, Schultheiß C, Dierks C, Binder M. 2022. Subclonal heterogeneity sheds light on the transformation trajectory in IGLV3-21R110 chronic lymphocytic leukemia. Blood Cancer J. 12(3):49.
- Pineda S, Sigdel TK, Liberto JM, Vincenti F, Sirota M, Sarwal MM. 2019. Characterizing pre-transplant and post-transplant kidney rejection risk by B cell immune repertoire sequencing. Nat Commun. 10(1):1906.
- Rothe C, Schunk M, Sothmann P, Bretzel G, Froeschl G, Wallrauch C, Zimmer T, Thiel V, Janke C, Guggemos W, et al. 2020. Transmission of 2019-nCov Infection from an asymptomatic contact in Germany. N Engl J Med. 382(10):970–71.
- Schultheiss C, Paschold L, Simnica D, Mohme M, Willscher E, von Wenserski L, Scholz R, Wieters I, Dahlke C, Tolosa E, et al. 2020. Next-generation sequencing of T and B cell receptor repertoires from COVID-19 patients showed signatures associated with severity of disease. Immunity. 53(2):442–55 e4.
- Schultheiß C, Paschold L, Simnica D, Mohme M, Willscher E, von Wenserski L, Scholz R, Wieters I, Dahlke C, Tolosa E, et al. 2020. Next-Generation sequencing of T and B cell receptor repertoires from COVID-19 patients showed signatures associated with severity of disease. Immunity. 53(2):442–55.e4.
- Shi Y, Wang G, Cai XP, Deng JW, Zheng L, Zhu HH, Zheng M, Yang B, Chen Z. 2020. An overview of COVID-19. J Zhejiang Univ Sci B. 21(5):343–60.
- Shugay M, Bagaev DV, Turchaninova MA, Bolotin DA, Britanova OV, Putintseva EV, Pogorelyy MV, Nazarov VI, Zvyagin IV, Kirgizova VI, et al. 2015. VDJtools: Unifying Post-analysis of T Cell Receptor Repertoires. PLoS Comput Biol. 11(11):e1004503.
- Sindhava V, Bondada S. 2012. Multiple regulatory mechanisms control B-1 B cell activation. Front Immunol. 3:372.
- Stamatopoulos K, Belessi C, Hadzidimitriou A, Smilevska T, Kalagiakou E, Hatzi K, Stavroyianni N, thanasiadou A, Tsompanakou A, Papadaki T, et al. 2005. Immunoglobulin light chain repertoire in chronic lymphocytic leukemia. Blood. 106(10):3575–83.
- Stamatopoulos B, Smith T, Crompot E, Pieters K, Clifford R, Mraz M, Robbe P, Burns A, Timbs A, Bruce D, et al. 2018. The light chain IgLV3-21 defines a new poor prognostic subgroup in chronic lymphocytic leukemia: results of a multicenter study. Clin Cancer Res. 24(20):5048–57.
- Tamura K, Stecher G, Kumar S. 2021. MEGA11: molecular evolutionary genetics analysis version 11. Mol Biol Evol. 38(7):3022–27.
- Tavakolpour S, Rakhshandehroo T, Wei EX, Rashidian M. 2020. Lymphopenia during the COVID-19 infection: What it shows and what can be learned. Immunol Lett. 225:31–32.
- Townsend CL, Laffy JMJ, Wu YC, Silva O’Hare J, Martin V, Kipling D, Fraternali F, and Dunn-Walters DK. 2016. Significant differences in physicochemical properties of human immunoglobulin kappa and lambda CDR3 regions. Front Immunol. 7:388 .
- Tucci FA, Kitanovski S, Johansson P, Klein-Hitpass L, Kahraman A, Dürig J, Hoffmann D, Küppers R. 2018. Biased IGH VDJ gene repertoire and clonal expansions in B cells of chronically hepatitis C virus–infected individuals. Blood. 131(5):546–57.
- Vabret N, Britton GJ, Gruber C, Hegde S, Kim J, Kuksin M, Levantovsky R, Malle L, Moreira A, Park MD, et al. 2020. Immunology of COVID-19: current state of the science. Immunity. 52(6):910–41.
- Voss WN, Hou YJ, Johnson NV, Kim JE, Delidakis G, Horton AP, Bartzoka F, Paresi CJ, Tanno Y, Abbasi SA, Pickens W. 2020. Prevalent, protective, and convergent IgG recognition of SARS-CoV-2 non-RBD spike epitopes in COVID-19 convalescent plasma. bioRxiv. 2020.12.20.423708.
- Wang W, Xu Y, Gao R, Lu R, Han K, Wu G, Tan W. 2020. Detection of SARS-CoV-2 in different types of clinical specimens. JAMA. 323(18):1843–44.
- Wang Y, Yuan M, Lv H, Peng J, Wilson IA, Wu NC. 2021. A large-scale systematic survey of SARS-CoV-2 antibodies reveals recurring molecular features. bioRxiv. 2021.11.26.470157
- Wen W, Su W, Tang H, Le W, Zhang X, Zheng Y, Liu X, Xie L, Li J, Ye J, et al. 2020. Immune cell profiling of COVID-19 patients in the recovery stage by single-cell sequencing. Cell Discovery. 6(1):31.
- Wong JB, Hewitt SL, Heltemes-Harris LM, Mandal M, Johnson K, Rajewsky K, Koralov SB, Clark MR, Farrar MA, Skok JA. 2019. B-1a cells acquire their unique characteristics by bypassing the pre-BCR selection stage. Nat Commun. 10(1):4768.
- Wu Z, McGoogan JM. 2020. Asymptomatic and pre-symptomatic COVID-19 in China. Infect Dis of Poverty. 9(1):72.
- Zhang W, Du Y, Su Z, Wang C, Zeng X, Zhang R, Hong X, Nie C, Wu J, Cao H, et al. 2015. Imonitor: a robust pipeline for TCR and BCR repertoire analysis. Genetics. 201(2):459–72.
- Zhang W, Feng Q, Wang C, Zeng X, Du Y, Lin L, Wu J, Fu L, Yang K, Xu X, et al. 2017. Characterization of the B cell receptor repertoire in the intestinal mucosa and of tumor-infiltrating lymphocytes in colorectal adenoma and carcinoma. J Immun. 198(9):3719–28.
- Zhang F, Gan R, Zhen Z, Hu X, Li X, Zhou F, Liu Y, Chen C, Xie S, Zhang B, et al. 2020a. Adaptive immune responses to SARS-CoV-2 infection in severe versus mild individuals. Signal Transduction Targeted Ther. 5(1):156.
- Zhang J-Y, Wang X-M, Xing X, Xu Z, Zhang C, Song J-W, Fan X, Xia P, Fu J-L, Wang S-Y, et al. 2020b. Single-Cell landscape of immunological responses in patients with COVID-19. Nat Immunol. 21(9):1107–18.
- Zhao X-N, You Y, Cui X-M, Gao H-X, Wang G-L, Zhang S-B, Yao L, Duan L-J, Zhu K-L, Wang Y-L, et al. 2021. Single-Cell immune profiling reveals distinct immune response in asymptomatic COVID-19 patients. Signal Transduction Targeted Ther. 6(1):342.
- Zost SJ, Gilchuk P, Case JB, Binshtein E, Chen RE, Nkolola JP, Schäfer A, Reidy JX, Trivette A, Nargi RS, Sutton RE. 2020. Potently neutralizing and protective human antibodies against SARS-CoV-2. Nature. 584(7821):443–449.