ABSTRACT
Monocytes are innate immune cells that play a dual role in protection of host against pathogens and initiation and perpetuation of inflammatory disorders including joint diseases. During inflammation, monocytes migrate from peripheral blood to tissues via chemokine receptors where they produce inflammatory factors. Monocytes are classified into three subsets, namely: classical, intermediate and non-classical, each subset has particular function. Synovium of patients with inflammatory joint diseases, such as rheumatoid arthritis and psoriatic arthritis as well as osteoarthritis, is enriched by monocytes that differ from circulatory ones by distinct subsets distribution. Several therapeutic agents used systemically or locally through intra-articular injections in arthritis management modulate monocyte subsets. This scoping review summarized the existing literature delineating the effect of common therapeutic agents used in arthritis management on circulating and synovial monocytes/macrophages. As certain agents have an inhibitory effect on monocytes, we propose to test their potential to inhibit synovial monocytes via an ex-vivo platform based on cultured synovial fluid mononuclear cells derived from patients with rheumatic diseases. Information obtained from the ex-vivo platform can be applied to explore the therapeutic potential of medications in clinical practice.
Introduction
Alterations in blood and inflamed joints monocytes are the predominant feature of arthritis and regulation of inflammation through monocytes could lead to new therapeutic strategies. This scoping review is based upon a literature search and aims to describe monocyte populations in blood and inflamed joints and underscore the effect of anti-rheumatic drugs on circulating and synovial monocytes, mainly in rheumatoid arthritis (RA), psoriatic arthritis (PsA), and osteoarthritis (OA).
Monocytes are involved in the initiation of inflammation and bone erosions in RA (Davignon et al., Citation2013). Infiltration of monocytes from peripheral blood into the joints is an early event in RA development. Circulating monocytes in patients with RA are hyper-inflammatory, with significantly higher expressions of inflammatory cytokines/chemokines than those of healthy controls (McGarry et al., Citation2021). Therapies that will inhibit monocytes/macrophages could restore homeostasis. Without proper treatment, RA can cause persistent synovial inflammation together with bone and cartilage destruction and, ultimately, long-term disability and extra-articular manifestations (Firestein, Citation2003). Current RA management guidelines recommend using synthetic disease-modifying anti-rheumatic drugs (sDMARDs), such as methotrexate (MTX), as the first-line therapy. Several biologic and targeted synthetic DMARDs targeting key activated immune pathways, such as tumor necrosis factor (TNF), interleukin-6 (IL‐6) receptor, T cell co-stimulation (CTLA-4 Ig), B cells (CD20), and Janus kinases (JAK) inhibitors are available in cases of no response (Smolen et al., Citation2017). However, although biologics and small molecules, such as JAK inhibitors, improve RA outcomes, response rates are not always adequate or long-lasting. Up to 30% of RA patients do not respond to TNF inhibitors (TNFi) therapy considered as a cornerstone therapy for inflammatory arthritis (Tak, Citation2012).
Monocytes play an important role in the pathogenesis of both psoriasis and joint inflammation in PsA. The mainstay systemic therapy approved for PsA is MTX, followed by anti-cytokines biologics targeting the key inflammatory cytokines, such as TNF, IL-17, and IL-23, and JAK inhibitors (Chao & Kavanaugh, Citation2019). While RA and PsA are considered systemic inflammatory diseases with high levels of inflammatory cytokines, such as TNF and IL-6 (McInnes & Schett, Citation2007), low-grade synovial inflammation has been observed in OA patients at both early and late disease stages (Benito et al., Citation2005). Monocytes contribute to inflammation (Haubruck et al., Citation2021), severity, and progression of OA (Gomez-Aristizabal et al., Citation2019). Even though OA is a universally leading cause of disability, no cure or disease-modifying treatments are available (Hunter et al., Citation2020). Symptoms are managed through a combination of non-pharmacological methods and non-steroidal anti-inflammatory drugs (NSAIDs) (Mushtaq et al., Citation2011). Surgical intervention through joint replacement remains the only option for end-stage disease, emphasizing the need for specific alternative treatment strategies.
Intra-articular (IA) injections deliver therapeutics directly to the joint space in joint disorders. IA injections of glucocorticoids (GCs) are widely used to reduce inflammation and pain, assist in increasing mobility, and improve the quality of life in patients with RA, PsA and OA (Bellamy et al., Citation2005; Eder et al., Citation2010; Hajialilo et al., Citation2016; Schumacher & Chen, Citation2005). The most frequently used IA injections in the treatment of OA are GCs, followed by IA hyaluronic acid (HA) injections. Platelet-rich plasma (PRP) (Ayhan et al., Citation2014) and mesenchymal stem cells (MSCs) are considered experimental IA treatment options (Ma et al., Citation2020).
Monocytes are abundantly present in arthritic joints. Pro-inflammatory monocytes release cytokines that activate the inflammatory process in the synovium. Understanding the direct effect of therapeutic agents on those cells and, subsequently, their ability to modulate other inflammatory cell populations could help to control inflammation within the joints. Here, we provide an overview of the inflammatory process, with a focus on the involvement of monocytes in different arthropathies. illustrates the therapeutic agents discussed in this review and their administration route in RA, PsA, and OA, including experimental agents that might target monocytes and are not yet approved for arthritis management.
Figure 1. Therapeutics in arthritis management detailed in this review and their route of administration.
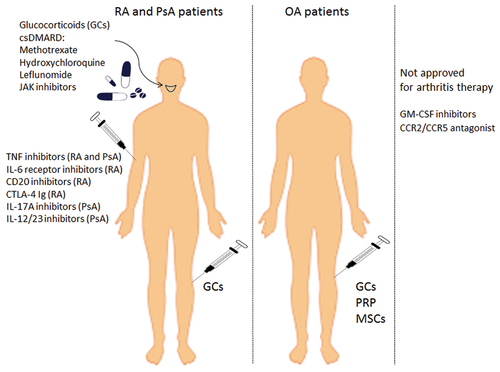
Types of monocyte subsets in the circulation and the synovial compartments
Blood monocytes are innate immune cells originating in the bone marrow and released into peripheral blood where they circulate for several days (Guilliams et al., Citation2018). Monocytes represent 5–10% of peripheral circulating leukocytes. They are categorized into three subsets based upon cell surface marker expression of lipopolysaccharide (LPS)-receptor (CD14) and the Fc gamma III receptor (CD16): CD14++CD16− (“classical”), CD14++CD16+ (“intermediate”), and CD14+CD16++ (“non-classical”; ). Similar subsets exist in mice, as evidenced by LY6C, CCR2, and CX3CR1 expression (Ziegler-Heitbrock, Citation2015; Ziegler-Heitbrock et al., Citation2010). Classical monocytes (CD14++CD16−) in peripheral blood can differentiate into monocyte-derived macrophages (Boyette et al., Citation2017). Intermediate monocytes (CD14++CD16+) express high levels of HLA-DR and Toll-like receptors (TLRs) and are, therefore, considered as an effector pro-inflammatory subset that contributes to antigen presentation and inflammatory cytokine production (Boyette et al., Citation2017). The non-classical cells (CD14+CD16++) play a role in removing damaged cells from blood vessels during inflammation (Thomas et al., Citation2015). The distribution of monocytes subsets in the blood is described as being classical (~85%), intermediate (~5%), and non-classical (~10%) (Ziegler-Heitbrock et al., Citation2010; ). Plasticity between different subsets has also been demonstrated. Classical monocytes have been shown to mature into intermediate and then into non-classical monocytes (Ancuta et al., Citation2009). Specifically, CD16+ monocytes are the main source of the pro-inflammatory cytokines, TNF and IL-1β, and have a diminished ability to produce the anti-inflammatory cytokine IL-10 (Belge et al., Citation2002).
Monocytes can differentiate into two macrophages types, M1 and M2. M1 macrophages are induced by LPS and by inflammatory cytokines, such as interferon-γ (Chabaud et al., Citation2001), and they secrete pro-inflammatory cytokines, such as IL-1β, IL-6, and TNF, as well as express TLR2, TLR4, CD80, and CD86 (Quero et al., Citation2017). Conversely, M2 macrophages secrete the anti-inflammatory cytokines IL-10 and TGF-β, and express typical CD204, CD163, and CD206 markers (Tardito et al., Citation2019). Induction of monocytes/macrophage activation is mediated through the CC-chemokine ligand 2 (CCL2)/CC-chemokine receptor 2 (CCR2), a monocyte-activating chemokine, ligand and its receptor. CCL2, also known as monocyte chemoattractant protein-1 (MCP-1), induced locomotion and recruitment of monocytes and macrophages to injury sites (Moadab et al., Citation2021). Likewise, CCL2/MCP-1 levels were significantly elevated not only in RA and PsA patients’ sera and synovial fluid (SF) (Stankovic et al., Citation2009), but also in sera and SF of OA patients compared to healthy controls (Longobardi et al., Citation2018). CCR2 is expressed by classical CD14++CD16− monocytes, and ligation with CCL2 can directly trigger migration of those cells to inflammatory sites (Tacke & Randolph, Citation2006). Mice with either CCL2 or CCR2 knocked out showed reduced histological OA changes and local macrophage infiltration (Raghu et al., Citation2017).
Changes in blood monocytes in rheumatic diseases
Circulating monocytes are recruited from the bloodstream and infiltrate into inflamed sites. The circulating CD14+CD16+ monocyte level was shown to be increased in RA patients compared to healthy controls. The CD14+CD16+ monocyte level positively correlated with higher disease activity in RA, whereas the CD14+CD16− monocyte level correlated with lower disease activity (Tsukamoto et al., Citation2017). Following MTX therapy, the CD14+CD16− subtype increased, while the CD14+CD16+ subtype decreased (Tsukamoto et al., Citation2017), indicating that the former could play some protective role. Thus, CD14+CD16+ and CD14+CD16− monocyte subsets might possess different functions in RA. CD14+CD16− monocytes are the primary source of osteoclasts (Xue et al., Citation2020), whereas CD14+CD16+ monocytes migrate to the synovium and produce high levels of TNF, IL-6, and IL-1β. These cytokines promote IL-17 cytokine production, thus playing a critical role in osteoclast formation in the synovium (Belge et al., Citation2002; Yoon et al., Citation2014). Moreover, circulating CD14+CD16+ monocytes were more prevalent in PsA and psoriasis (PsO) patients compared to healthy controls (Chiu et al., Citation2010). The CD16 expression level correlated with bone resorption activity in PsA, whereupon CD16 expression in the peripheral blood could potentially be used as a susceptibility marker for arthritis in PsO patients and an indicator for treatment response among PsA patients with erosive arthritis (Chiu et al., Citation2010). In OA, monocytes display an enhanced capacity to generate osteoclasts as was shown by the increased osteoclast numbers, probably due to decreased apoptosis (Durand et al., Citation2013).
Synovial fluid and tissue monocytes
Peripheral blood and SF compartments share similarities. In SF, monocytes are highly abundant, representing at least 20% of the SF cells. In RA, intermediate and classical monocytes comprise about 70% and 30% of the SF monocytes, respectively, whereas the non-classical monocytes are infrequent (Burbano et al., Citation2018; Yoon et al., Citation2014). Intermediate CD14+CD16+ monocytes are enriched in the SF of PsA (Yager et al., Citation2021), juvenile idiopathic arthritis (JIA; Cren et al., Citation2020; Schmidt et al., Citation2020), and OA patients (Gomez-Aristizabal et al., Citation2019; ).
In PsA, mass cytometry (CyTOF) combined with transcriptomic analysis found that CD14+ cells were enriched in PsA SF and monocytes/macrophages spontaneously produced substantial levels of pro-inflammatory proteins, including osteopontin and CCL2 (Kurowska-Stolarska & Alivernini, Citation2022). In addition, downregulated CD14+CD6− classical and upregulated CD14+CD16+ intermediate monocytes were found in PsA compared to RA and OA. The protease-activated receptor 2 (PAR2) expression in PsA monocytes/macrophages significantly affected MCP-1 production (Abji et al., Citation2020).
Figure 2. Distribution and main characteristics of circulating and synovial monocyte subsets in joint inflammation. (a) Prevalence (%) of monocytes among total leukocytes and monocytes subsets according to CD14 and CD16 expression (b) Monocytes from the circulation migrate to the synovium and lead to imbalance of M1/M2 macrophages responsible for inflammation. CCL2 influx leads to monocyte migration from the circulation to the joints. As a consequence, M1 macrophages producing the pro-inflammatory cytokines IL-1β, IL-6, and TNF are up-regulated, while the anti-inflammatory M2 macrophages producing TGF-β and IL-10 are down-regulated. This event promotes synovial tissue damage instead of tissue repair. Abbreviations: Chemokine (C-C motif) ligand 2 (CCL2) cluster differentiation (CD), interleukin (IL), macrophage (M) transforming growth factor-beta (TGF-β) and tumor necrosis factor (TNF).
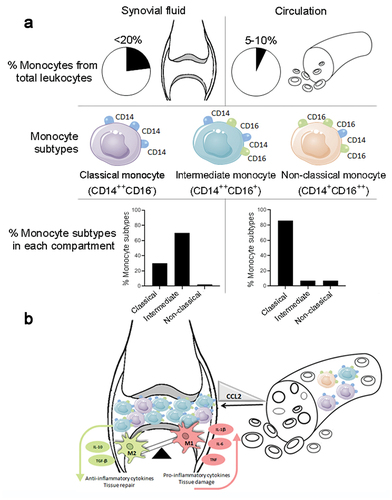
There is a significant correlation between SF CD14+CD16+ monocyte frequency and disease activity parameters (Gomez-Aristizabal et al., Citation2019). Joint destruction correlates with synovial monocytes/macrophage infiltration in RA (Mulherin et al., Citation1996). SF monocytes differentiate into osteoclasts that mediate bone erosion (Adamopoulos et al., Citation2006) and promote CD4+ T cell polarization to Th1 and Th17 cells, which are considered as critical mediators of RA (Roberts et al., Citation2015).
Monocytes are abundant not only in the SF but also in the synovium itself. In RA synovial tissue can be divided into three distinct pathology groups: (1) lympho-myeloid dominated by lymphoid lineage infiltration (T cells, B cells, plasma cells) (2) diffuse-myeloid with myeloid lineage predominance (3) pauci-immune characterized mainly by stromal cells (Humby et al., Citation2019). Using single cell RNA sequencing (sc-RNA seq) technology that enables a high-resolution characterization of key cellular phenotypes, CD14+CD16+ monocytes were found to be abundant in the diffuse-myeloid and lympho-myeloid compared to pauci-immune group in treatment naïve early RA patients. Further dissection of the synovium monocytes in RA showed that in leucocyte rich RA synovium monocyte populations were characterized by high expression of IL-1β+ and IFN activated genes relative to OA and leucocyte poor RA synovium, suggesting that cytokine activation drives expansion of unique monocyte population that are potentially key mediators in RA pathogenesis (Zhang et al., Citation2019).
Moreover, specific monocyte/macrophages subpopulation has been identified in RA synovium with correlation to clinical phenotype. A unique cluster of inflammatory macrophage/monocyte identified as CD14+HBEGF+ (heparin binding epidermal growth factor) was enriched in the synovial tissue of RA patients (Kuo et al., Citation2019). These cells were induced by TNF and promoted fibroblast mediated joint destruction. On the other hand, MER tyrosine kinase (MerTKpos) synovial macrophages subpopulation correlated with resolution of inflammation and, therefore, could be potential treatment biomarkers in RA (Alivernini et al., Citation2020). Sc-RNA seq analysis showed similar findings in OA, where monocytes/macrophages were the most abundant immune cells in OA synovium responsible for production of inflammatory cytokines including TNF, IL-1β and IL-18 (Liu et al., Citation2021).
To date there are no data regarding the monocyte population in the synovial tissue of PsA patients.
Mechanisms and effects of therapeutic agents on circulating and synovial monocytes
Understanding the mechanisms and effects of anti-rheumatic agents on synovial monocytes may assist in reducing synovial inflammation. We refer below to the therapeutic agents that have been shown to modulate monocytes in the circulation and in synovial compartments.
Effect of glucocorticoids on monocytes
GCs are steroid hormones endogenously produced in the adrenal cortex. GCs penetrate the plasma membrane of target cells due to their lipophilic structure and bind to the cytosolic GC-receptor (GR) in the cytoplasm. The GCs/GR complex in the nucleus can bind as a homodimer to GC response elements (GREs) that promote target genes, leading to the activation of anti-inflammatory genes, such as IL-10, a process known as “transactivation.” Binding to negative GREs can result in suppression of target gene transcription, a process known as “transrepression.” Moreover, the monomeric GCs/GR complex can inhibit gene transcription independently from DNA binding by direct interaction with other transcription factors, such as nuclear factor-κB (NF-κB) and activator protein-1 (AP-1). These protein-protein interactions are known as “tethering” (Barnes, Citation2017). GCs have been the mainstay therapy of rheumatic diseases due to their potency as anti-inflammatory agents that modulate apoptosis of immune cells, among other mechanisms. In vitro synthetic GCs induce T cell apoptosis (Kirsch et al., Citation1999) and promote expansion of T regulatory cells (Karagiannidis et al., Citation2004). Their effect on monocyte phenotype and function in this context has not been well characterized.
Effect of GCs on monocytes in circulation
GC treatment of peripheral human monocytes was shown to induce a CD14+CD16+ intermediate subset phenotype with an attenuated capacity to promote both naive CD4+ T cell proliferation and polarization into Th1 and Th17 cells. In particular, CD14+CD16+ monocytes exhibited the lowest TNF level and the highest IL-10 level compared to the other monocyte subsets (Liu et al., Citation2015). GC-treated ex-vivo monocytes have shown enhanced survival, phagocytosis and chemotaxis, while displaying an anti-inflammatory phenotype (Barczyk et al., Citation2010). GCs inhibit the transcription of several pro-inflammatory cytokines produced by human monocytes and macrophages, including IL-1β, IL-6, IL-12, TNF, or GM-CSF, and down-regulate the expression of IL-8, RANTES, and MCP-1 chemokines (Cain & Cidlowski, Citation2017). In addition, GCs induce IL-10 secretion from monocytes but not from T cells (Mozo et al., Citation2004).
Effect of GCs on monocytes in synovium
IA injections of GCs have been used for decades in clinical practice to relieve pain and to control local inflammation in RA, PsA, and OA (Blyth et al., Citation1994). However, there are some concerns about potentially deleterious long-term effects of repeated IA injections of GCs (McAlindon et al., Citation2017). Local GC administration decreased the lymphocytes number without changing the monocyte population in the synovium (Makrygiannakis et al., Citation2008). The GC compound, triamcinolone acetonide (TAA), administered in IA injections to a collagenase-induced OA (CiOA) mouse model was found to increase long-term synovial macrophage/monocytes numbers (Ferrao Blanco et al., Citation2022). Hence, GCs induce anti-inflammatory effects both in the circulation and in the synovium by inhibition of the pro-inflammatory monocytes/macrophages phenotype without an overall reduction in the monocyte population.
Effect of conventional DMARDs on monocytes
Conventional disease-modifying anti-rheumatic drugs (cDMARDs) comprise a class of drugs used in RA and PsA therapy. They include methotrexate (MTX), hydroxychloroquine, leflunomide, and sulfasalazine.
Effect of methotrexate (MTX) on monocytes
MTX is a folic acid antagonist and the principal drug in RA and PsA therapy (Chara et al., Citation2015; Lopez-Olivo et al., Citation2014). Although the effect of MTX on RA is not completely understood, it is thought to derive from pathways other than folic acid antagonism, such as from adenosine or JAK/STAT pathway modulation (Ptacek et al., Citation2021). The pre-treatment monocyte distribution might predict response to treatment with MTX in RA. Higher pre-treatment numbers of circulating monocytes, especially of CD14++CD16− and CD14++CD16+ subsets, predicted a lack of clinical response to MTX compared to responders (Chara et al., Citation2015). Another study demonstrated that a higher CD16 level (FcγIIIa receptor) on CD14++ monocytes allowed their binding to the immune complex found abundantly in RA patients, enhancing TNF production and disease perpetuation. Moreover, an elevated CD16 level correlated negatively with treatment response to MTX in RA, suggesting that increased CD16 on monocytes may be a biomarker of no-response to MTX therapy in RA (Chara et al., Citation2015).
In vitro testing of MTX’s ability to inhibit proliferation and induce apoptosis in cultured human monocytic myeloid cells (THP-1) and synovial macrophages showed that MTX had a dose-dependent effect. Significant proliferation inhibition and apoptosis induction was shown with MTX at concentrations within a range of 75–500 µg/ml compared with untreated controls. However, there were no differences in monocyte proliferation and apoptosis compared to untreated cells at lower MTX concentrations (within a range of 5 ng/ml to 5 µg/ml; Cutolo et al., Citation2000). Given that the MTX plasma concentration in RA patients is approximately 0.05 µg/ml (Kivity et al., Citation2014), the MTX doses required for adequate RA disease control seem to possess only a minimal effect on monocytes.
Effect of hydroxychloroquine on monocytes
Hydroxychloroquine (HCQ) has been hypothesized to target endosomal NADPH oxidase (NOX), which is fundamental in the transduction inflammation pathway (Muller-Calleja et al., Citation2017). HCQ acts to reduce the induction of endosomal NOX by TNF, IL-1β and antiphospholipid antibodies (apL) in human monocytes, thereby preventing certain cytokine production by these cells (Muller-Calleja et al., Citation2017). However, no data on the effect of HCQ effect on monocytes are available.
Effect of leflunomide on monocytes
Leflunomide reversibly inhibits the enzyme, dihydro-orotate dehydrogenase, which is the rate-limiting step in the de novo synthesis of pyrimidines mainly in T cells. Leflunomide also influences the signaling between T lymphocytes and monocytes by suppressing TNF and IL-1β production. Leflunomide metabolites inhibit the glycosylation of the intercellular adhesion molecule 1 (ICAM-1) and vascular cell adhesion 1 (VCAM-1), which decreases the number of macrophages in the synovial tissue. This was confirmed by in vitro and dynamic gadolinium-enhanced magnetic resonance imaging (DRMRI) studies (Cutolo et al., Citation2003). Leflunomide in combination with MTX showed additive inhibitory effects on the production of inflammatory mediators in synovial macrophages co-cultured with a T cell line derived from RA patients (Cutolo et al., Citation2006).
Effect of biologic agents on monocytes
TNFi used in RA and PsA management
The TNF cytokine is expressed either as a membrane-bound form (mTNF) or cleaved into a soluble TNF. TNFi acts by inhibiting soluble TNF, but they have the supplementary action of binding to mTNF, which is mainly expressed by monocytes/macrophages. While soluble TNF elicits signals through TNFR1 and TNFR2, mTNF can also function as a receptor and, upon engaging with TNFR2 or TNFi, sends signals into mTNF-expressing cells, a process known as reverse signaling (Meusch et al., Citation2015). mTNF crosslinking with TNFi can induce apoptosis and inhibition of the excessive IL-1β secretion by monocytes of RA patients (Meusch et al., Citation2009). This action may contribute to the efficacy of TNFi (Deora et al., Citation2017). Moreover, mTNF reverse signaling was recently demonstrated in vivo in a triple transgenic mouse model (3TG) lacking TNF receptor R1 and R2 expression (TNFR1/R2 KO) (Diallo et al., Citation2021). Those mice expressed TNF at a physiological level exclusively in a tmTNF form due to knock-in mutations. Following the induction of arthritis, the 3TG mice were treated with TNFi which led to disease attenuation. Furthermore, pro-inflammatory IL-1β was inhibited, and neutrophils were reduced in the treated mice joints, thus demonstrating for the first time the reverse signaling significance in vivo and implicating a novel interpretation of the TNFi effect in inflammatory disease therapy (Diallo et al., Citation2021). The potential value of blood monocyte mTNF expression as a predictor of the response to TNFi merits further investigation. It was recently shown that whole-blood assay measuring mTNF-induced IL-10 predicted the clinical response to a TNFi therapy. The IL-10 level was significantly higher in TNFi responders than in non-responders before treatment initiation. mTNF crosslinking-induced IL-10 production correlated with the initial disease activity and the subsequent response to TNFi. Interestingly, the assay’s predictive value was unique to TNFi but not predictive for JAK inhibitors (Krasselt et al., Citation2022). After the induction of arthritis, infliximab-treated transgenic mice expressing human TNF (hTNF-Tg) demonstrated a significant reduction in arthritis symptoms. Ly6C+ macrophages were reduced in the ankles and lymph nodes, and subsequent reduction was accompanied by increased apoptosis induced by infliximab (Huang et al., Citation2018). TNFi decreased the peripheral classical CD14hiCD16− monocytes in the circulation in RA and ankylosing spondylitis patients. Circulating non-classical CD14dimCD16+ monocytes were significantly increased. Although the numbers of CD14dimCD16+ monocytes were also increased by TNFi, the expression of CD11b and CD11c integrin on their surface was significantly reduced (Batko et al., Citation2019). The monocytes of RA patients had a defect in differentiating into M2-macrophages as well as a tendency towards preferential maturation toward M1-macrophages, thus contributing to synovial inflammation. A specific short RNA overexpression (mir-155) in monocytes/macrophages was responsible for this differentiation defect, and it was reversed by TNFi treatment (Paoletti et al., Citation2019).
A study by Chara et al. (Citation2012) found that absolute circulating monocyte counts and the three monocyte subsets at three months of treatment with TNFi (adalimumab) in combination with MTX predicted the clinical response after six months of treatment in RA patients. Non-responder patients exhibited an increased number of monocytes and of those monocytes’ three subsets after three months of TNFi treatment. Furthermore, there was no difference in the absolute or in the three subsets of monocytes in the group of RA patients treated with MTX alone compared to non-treated patients. These results point toward the potential predictive value of monitoring monocyte count and distribution among TNFi-treated patients to determine the best clinical management (Chara et al., Citation2012).
Furthermore, the circulating monocyte count could be used to predict long-term remission status in RA patients treated with TNFi. In a retrospective cohort study spanning 10 years, Shipa et al. (Citation2021). found that a decrease in monocyte levels was the strongest predictor of sustained remission. Patients with a relatively small decrease in monocyte counts at 6 months from baseline pre-TNFi therapy were at the greatest risk for a loss of remission in the future. Those authors suggested that TNFi could be tapered in patients with a significant decrease in monocytes level at six months after TNFi therapy initiation.
Synovial monocytes are also modulated by systemic TNFi administration. TNFi reduced the number of infiltrating granulocytes and monocytes in the RA synovium, and also the expression of IL-8, MCP-1, IL-1, IL-6, GM-CSF, and other chemokines (Taylor et al., Citation2000).
Systemic TNFi therapy with either soluble TNF receptor (etanercept) or TNF chimeric monoclonal antibody (infliximab) led to a significant decrease in the synovial monocyte/macrophage population mediated by increased apoptosis in RA. Circulating monocyte/macrophages were less susceptible to TNFi-mediated apoptosis (Catrina et al., Citation2005), potentially due to elevated monocyte numbers in the synovium compared to the periphery.
We found that TNFi reduced the CD14+CD16+ cells (intermediate monocytes) in synovial fluid mononuclear cells (SFMCs) derived from PsA and RA patients ex-vivo. This activity of TNFi was selective and not mediated by other tested drugs: GCs or by other biologics used for PsA management (IL-17A or IL-12/23 inhibitors) or RA management (IL-6 R or CD20 inhibitors). Moreover, analysis of gene expression revealed a differential mechanism of action for TNFi compared to GCs. IL-1β was down-modulated by TNFi and up-regulated by GCs, whereas inflammatory IL-8 and MMP-9, as well as, the protective IL-10 were modulated in a similar fashion by TNFi and GCs, however GCs modulated them to a greater extent (Gertel et al., Citation2023).
IL-6 receptor and CD20 inhibitors and CTLA4-Ig
IL-6 is a pro-inflammatory cytokine elevated in the sera and SF of RA patients, and its levels correlate with disease activity and joint destruction (Dayer & Choy, Citation2010). The baseline level of circulating intermediate CD14+CD16+ monocytes was significantly higher in RA patients compared to healthy controls (Tsukamoto et al., Citation2018). During therapy with IL-6 receptor inhibitor, tocilizumab, the circulating level of CD14+CD16+ monocytes was significantly decreased, correlating with improved disease activity (Tsukamoto et al., Citation2018). Tocilizumab reduced T cell counts but not macrophage counts in RA patients synovium (Chatzidionysiou et al., Citation2021).
Anti-CD20 (rituximab), a chimeric monoclonal antibody, initially affects B cells by decreasing B cell numbers and immunoglobulin levels, correlating with improved RA disease outcomes (Cambridge et al., Citation2006). Skewing of macrophage function towards anti-inflammatory phenotype was observed in rituximab-treated RA patients (Toubi et al., Citation2007). Rituximab treatment resulted in a significant decrease of synovial B cells as well as other inflammatory cells, such as T cells and macrophages, leading to diminished synovial inflammation (Thurlings et al., Citation2008).
Abatacept represents a soluble recombinant human fusion protein comprising the extracellular domain of human CTLA-4 and a fragment of the modified Fc domain of human IgG1 (CTLA-4-Ig). Abatacept inhibits the binding of CD28 due to its interaction with CD80/CD86 and thereby impairs effector T cell activation. In addition, inhibition of CD80/CD86 binding might also exert effects on antigen-presenting cells, including monocytic lineage cells. CTLA-4-Ig treatment induces an M1-M2 shift in cultured monocyte-derived macrophages from healthy subjects as well as from RA patients (Cutolo et al., Citation2021). One of abatacept’s mechanisms on circulating monocytes derived from both RA patients and healthy controls is the down-regulation of CD64/FcγRI on circulating monocytes via direct binding to CD86 and the suppression of the production of immune complex-mediated inflammatory cytokines (Fukue et al., Citation2022). Abatacept interferes with monocytes migration into the synovial tissue which might contribute to the beneficial effects of abatacept in RA treatment (Bonelli et al., Citation2013).
IL-17A and IL-23/12 inhibitors
Th17 cells play an important role in the pathogenesis of skin and joint disease in PsA, with elevated levels of Th17 cells having been found in the SF and peripheral blood of PsA patients, pointing to treatment strategies aimed at targeting Th17 cells (Benham et al., Citation2013). Anti-IL-17 therapy demonstrated efficacy in PsA (McInnes et al., Citation2015). IL-17 was shown to be chemotactic for monocytes into the synovial tissue in both in vitro and in vivo studies, and both IL-17 and IL-17 receptor inhibitors prevented this migration (Shahrara et al., Citation2009). The IL-23 pathway has a pivotal role in PsA, and ustekinumab, an IL-12p40/IL-23p40 inhibitor, reduced CD68+-expressing cells in the synovial tissue of PsA patients (Fiechter et al., Citation2021).
Effect of JAK inhibitors on monocytes
The Janus kinase/signal transduction and activator of transcription (JAK – STAT) signaling pathway is implicated in the pathogenesis of inflammatory and autoimmune diseases including RA. Type I/II cytokine receptor family used by several cytokines employs JAK – STAT pathway through downstream activation and phosphorylation of STATs which translocate to the nucleus to modulate transcription (Yamaoka, Citation2016). The development of targeted small-molecule therapies such as JAK inhibitors, have emerged as an effective treatment in RA and PsA (Fragoulis et al., Citation2019). JAK inhibitors suppressed macrophage activation in both synovium and peripheral blood, leading to suppression of the production of certain cytokines/chemokines, including TNF (Yarilina et al., Citation2012). In the periphery, baricitinib, a JAK1 and JAK2 inhibitor, reduced the monocyte frequency only in responders. Baricitinib reduced the cytokine-induced by STAT1 in monocytes of responders compared with non-responders. IFN signature was higher at baseline in responders compared with non-responders. These results suggest a higher baseline expression of IFN-related genes and STAT1 phosphorylation in baricitinib responders (Tucci et al., Citation2022). The activity of tofacitinib, a JAK1 and JAK3 inhibitor, was demonstrated by inhibition of the IL-6/JAK/STAT mechanism that affected synovial monocytes from JIA patients and helped to restore synovial homeostasis (Schmidt et al., Citation2023).
Experimental therapies for arthritis management
Novel therapeutics that block essential molecules on monocytes might represent inhibitory pathways to avoid excessive monocytes accumulation in the synovium. Here, we describe two types of monocyte inhibitors.
Effect of GM-CSF inhibitors on monocytes
GM-CSF cytokine converts myeloid stem cells into mature cells and activates mature neutrophils, eosinophils, and macrophages (Greven et al., Citation2015). Furthermore, it is a pro-inflammatory cytokine that can promote the conversion of monocytes into M1 macrophages at certain inflammation sites, including the synovium. GM-CSF binds to the 2 subunits of its receptor, a receptor (GM-CSFRα) and a signal-transducing subunit β-chain (βc), with the latter being shared with IL-3 and IL-5. GM-CSF and its receptor were detected in RA patients sera and SF (Greven et al., Citation2015). The most well-known GM-CSF antagonists are two monoclonal antibodies, mavrilimumab and otilimab, which target the GM-CSF alpha chain of the receptor and GM-CSF itself, respectively (Sachsenweger, Citation1960). CD68-positive macrophages in the synovial sublining layer correlated with RA disease activity (Haringman et al., Citation2005). The synovial expression of GM-CSF receptor was reduced following the administration of CAM-3003, a GM-CSFR antagonist, in collagen-induced arthritis mice model (Greven et al., Citation2015).
Effect of a CCR2/CCR5 antagonist (cenicriviroc, CVC) on monocytes
A novel orally administered drug which targets CCR and CCR5 molecules on monocytes (Lalezari et al., Citation2011) in liver fibrosis is currently under evaluation (clinical trial NCT02217475) (Qian et al., Citation2022). Monocytes derived from OA SF that were assessed in an organotypic microfluidic model ex-vivo were inhibited by a CCR2/CCR5 antagonist (Mondadori et al., Citation2021).
Disease-modifying OA agents
Pharmacological treatments are mostly related to symptom relief, and there are currently no disease-modifying OA agents to slow down or stop disease progression.
Effect of mesenchymal stem cells (MSCs) on monocytes
Human embryonic stem cell-induced mesenchymal stem cells (ESC-MSC) were shown to greatly reduce OA in an in vitro and in vivo mouse model. Exosomes extracted from culture media of ESC-MSCs assisted the chondrocytes in balancing the synthesis and degradation of the extracellular matrix, whose dysfunction is a major element in OA pathogenesis (Wang et al., Citation2017). While there does not appear to be any study on the linking of MSCs to monocytes, MSCs were shown to promote an anti-inflammatory cell phenotype (Mancuso et al., Citation2019).
Effect of platelet-rich plasma on M1/M2 macrophage polarization
Platelet rich plasma (PRP) is an autologous blood product that is produced by centrifuging whole blood and concentrating platelets. It contains growth factors and cytokines, and its high anti-inflammatory factor concentration is of special importance in relation to OA. PRPs have been shown to increase M2 predominance over M1 macrophages in vitro (Uchiyama et al., Citation2021).
summarizes the effects of the above-cited therapeutic agents on monocyte populations.
Table 1. Effect of therapeutic agents on monocyte populations.
Ex-vivo assay of cultured synovial monocytes for evaluating therapeutic modulation of synovial monocytes by therapeutic agents
Targeting monocytes may represent a promising therapeutic strategy to alleviate arthritis. Using ex-vivo model to culture synovial monocytes derived from SF enables mimicking the synovial environment and testing disease-specific drug effects. As was shown in various experimental studies on peripheral blood monocytes, the level and alteration of monocyte subsets over time may have a predictive value for determining drug effectiveness. In view of differences between the synovial and circulatory monocytes, there is a knowledge gap related to modulation of synovial monocytes, monocytes subsets, and their polarization to M1 and M2 macrophages.
In clinical practice, SF is obtained by arthrocentesis procedure performed to reduce joint pain. SF is more accessible for research than synovial tissue, which can be obtained only via invasive procedure, such as arthroscopy or synovial biopsy. SF specimens can be collected from patients with active synovial disease. The wide clinical use of arthrocentesis in clinical practice enables to set cohort studies using ex-vivo assays. For studies on SF monocytes, the SFMCs are separated on Ficoll gradient centrifugation similarly to the protocol applied for the extraction of peripheral blood mononuclear cells (PBMCs). The extracted cells from middle phase can be immediately freeze or cultured for further assays. Studies on monocytes cultured ex-vivo revealed that monocytes remain vital in culture up to 7 days (Safi et al., Citation2016).
Monocytes/macrophages and fibroblast-like synoviocytes (FLS) are predominant synovial cell types (Tu et al., Citation2018). Monocytes derived from SFMCs are cultured in suspension permitting the analysis of surface markers expression by flow cytometry. In contrast, FLS adhere to plastic culture-dishes and are passaged by trypsinization to detach from plate surface (Neumann et al., Citation2010), which may affect expression of certain cell surface markers (Zhang et al., Citation2012). Therefore, the effect of drugs on synovial monocytes versus FLS might yield different results.
We propose to use an ex-vivo assay to assess monocytes response to a variety of therapeutic agents including therapy combinations. The assay will enable an analysis of SF monocytes response during the culture period, including cell surface markers, cytokine secretion, and gene expression. The activity of the tested therapeutic agents on the monocytes should be compared to the effect of GCs, the only intra-articular agent used to inhibit synovial inflammation. To test the effect of diverse therapeutic agents, their supplementation to the SFMCs culture should be adjusted to their therapeutic doses. The proposed model is shown in .
Using this model we tested the effect of TNFi versus GCs on %CD14+CD16+ monocytes in SFMCs derived from PsA and RA patients. TNFi but not GCs reduced the %CD14+CD16+ monocytes (Gertel et al., Citation2023). Along with our results showing the applicability of TNFi in reducing synovial monocytes, it was shown that IA injection of TNFi was an effective method for the treatment of recurrent synovitis (Chen et al., Citation2023). It is important to note that biologics were not approved for intra-articular treatment.
Figure 3. Schematic overview of ex-vivo system to analyze drugs effect on SFMCs derived from arthritis patients. The synovial cells are extracted and cultured ex-vivo with the drugs of interest. The activity of the drugs is then compared to control drugs with known mechanisms of action currently in use to treat joint disease (e.g., GCs: methylprednisolone (MPA) and betamethasone (BET)). Next, the cells are analyzed for changes of surface markers by flow cytometry, cytokine secretion, and gene expression. The effect of each drug on monocyte populations is then determined. This ex-vivo system could be used for analyzing the potential utilization of therapeutic agents for the treatment of different inflammatory arthritis types.
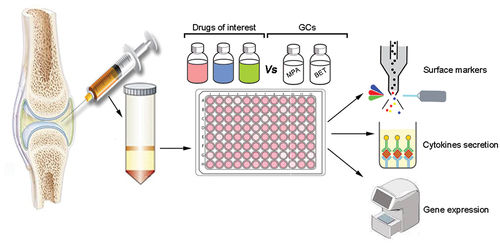
Conclusions and recommendations
This review presents the alterations in the circulatory and synovial monocyte population in patients with different arthropathies. We described the effects of systemic and IA anti-rheumatic therapies on monocyte population subsets. In light of the unmet need to discover therapeutic agents suitable to control synovial inflammation, we developed an ex-vivo monocyte model resembling the synovial microenvironment derived from SF. This ex-vivo system can be used for screening of new therapeutic candidates for the treatment of arthritis. Based on the current knowledge, we suggest that synovial monocytes could serve as pivotal indicators for DMARD selection and finding alternative drugs for IA injections.
Author’s contribution
Adam Slavick and Smadar Gertel were involved in planning the review, literature survey, manuscript writing and creation of figures. Victoria Furer, Ari Polachek, Reut Tzemach and Ori Elkayam reviewed and edited the manuscript. All authors read and approved the final manuscript.
Disclosure statement
No potential conflict of interest was reported by the author(s).
Additional information
Funding
References
- Abji, F., Rasti, M., Gomez-Aristizabal, A., Muytjens, C., Saifeddine, M., Mihara, K., Motahhari, M., Gandhi, R., Viswanathan, S., Hollenberg, M. D., Oikonomopoulou, K., & Chandran, V. (2020). Proteinase-mediated macrophage signaling in psoriatic arthritis. Frontiers in Immunology, 11, 629726. https://doi.org/10.3389/fimmu.2020.629726
- Adamopoulos, I. E., Sabokbar, A., Wordsworth, B. P., Carr, A., Ferguson, D. J., & Athanasou, N. A. (2006, January). Synovial fluid macrophages are capable of osteoclast formation and resorption. The Journal of Pathology, 208(1), 35–43. https://doi.org/10.1002/path.1891
- Alivernini, S., MacDonald, L., Elmesmari, A., Finlay, S., Tolusso, B., Gigante, M. R., Petricca, L., DiMario, C., Bui, L., Perniola, S., Attar, M., Gessi, M., Fedele, A. L., Chilaka, S., Somma, D., Sansom, S. N., Filer, A., McSharry, C., Millar, N. L., ... Kurowska-Stolarska, M. (2020, August). Distinct synovial tissue macrophage subsets regulate inflammation and remission in rheumatoid arthritis. Nature Medicine, 26(8), 1295–1306. https://doi.org/10.1038/s41591-020-0939-8
- Ancuta, P., Liu, K. Y., Misra, V., Wacleche, V., Gosselin, A., Zhou, X., & Gabuzda, D. (2009, August 27). Transcriptional profiling reveals developmental relationship and distinct biological functions of CD16+ and CD16- monocyte subsets. BMC Genomics, 10, 403. https://doi.org/10.1186/1471-2164-10-403
- Ayhan, E., Kesmezacar, H., & Akgun, I. (2014, July). Intraarticular injections (corticosteroid, hyaluronic acid, platelet rich plasma) for the knee osteoarthritis. World Journal of Orthopedics, 5(3), 351–361. https://doi.org/10.5312/wjo.v5.i3.351
- Barczyk, K., Ehrchen, J., Tenbrock, K., Ahlmann, M., Kneidl, J., Viemann, D., & Roth, J. (2010, July). Glucocorticoids promote survival of anti-inflammatory macrophages via stimulation of adenosine receptor A3. Blood, 116(3), 446–455. https://doi.org/10.1182/blood-2009-10-247106
- Barnes, P. J. (2017). Glucocorticosteroids. Handbook of Experimental Pharmacology, 237, 93–115. https://doi.org/10.1007/164_2016_62
- Batko, B., Schramm-Luc, A., Skiba, D. S., Mikolajczyk, T., & Siedlinski, M. (2019, January). TNF-α inhibitors decrease classical CD14(hi)CD16− Monocyte subsets in highly active, conventional treatment refractory rheumatoid arthritis and ankylosing spondylitis. International Journal of Molecular Sciences, 20(2), 291. https://doi.org/10.3390/ijms20020291
- Belge, K. U., Dayyani, F., Horelt, A., Siedlar, M., Frankenberger, M., Frankenberger, B., Espevik, T., & Ziegler-Heitbrock, L. (2002, April). The proinflammatory CD14+CD16+DR++ monocytes are a major source of TNF. Journal of Immunology, 168(7), 3536–3542. https://doi.org/10.4049/jimmunol.168.7.3536
- Bellamy, N., Campbell, J., Robinson, V., Welch, V., Gee, T. L., Bourne, R., & Wells, G. A. (2005, April). Intraarticular corticosteroid for treatment of osteoarthritis of the knee. Cochrane Database of Systematic Reviews, 18(2), CD005328.
- Benham, H., Norris, P., Goodall, J., Wechalekar, M. D., FitzGerald, O., Szentpetery, A., Smith, M., Thomas, R., & Gaston, H. (2013, September). Th17 and Th22 cells in psoriatic arthritis and psoriasis. Arthritis Research & Therapy, 15(5), R136. https://doi.org/10.1186/ar4317
- Benito, M. J., Veale, D. J., & FitzGerald, O., Van Den Berg, W. B., & Bresnihan, B. (2005, September). Synovial tissue inflammation in early and late osteoarthritis. Annals of the Rheumatic Diseases, 64 (9), 1263–1267. https://doi.org/10.1136/ard.2004.025270
- Blyth, T., Hunter, J. A., & Stirling, A. (1994, May). Pain relief in the rheumatoid knee after steroid injection. A single-blind comparison of hydrocortisone succinate, and triamcinolone acetonide or hexacetonide. British Journal of Rheumatology, 33(5), 461–463. https://doi.org/10.1093/rheumatology/33.5.461
- Bonelli, M., Ferner, E., Goschl, L., Blüml, S., Hladik, A., Karonitsch, T., Kiener, H. P., Byrne, R., Niederreiter, B., Steiner, C. W., Rath, E., Bergmann, M., Smolen, J. S., & Scheinecker, C. (2013, March). Abatacept (CTLA-4IG) treatment reduces the migratory capacity of monocytes in patients with rheumatoid arthritis. Arthritis & Rheumatism, 65(3), 599–607. https://doi.org/10.1002/art.37787
- Boyette, L. B., Macedo, C., Hadi, K., Elinoff, B. D., Walters, J. T., Ramaswami, B., Chalasani, G., Taboas, J. M., Lakkis, F. G., & Metes, D. M. (2017). Phenotype, function, and differentiation potential of human monocyte subsets. PLoS One, 12(4), e0176460. https://doi.org/10.1371/journal.pone.0176460
- Burbano, C., Rojas, M., Munoz-Vahos, C., Vanegas-García, A., Correa, L. A., Vásquez, G., & Castaño, D. (2018, December). Extracellular vesicles are associated with the systemic inflammation of patients with seropositive rheumatoid arthritis. Scientific Reports, 8(1), 17917. https://doi.org/10.1038/s41598-018-36335-x
- Cain, D. W., & Cidlowski, J. A. (2017, April). Immune regulation by glucocorticoids. Nature Reviews: Immunology, 17(4), 233–247. https://doi.org/10.1038/nri.2017.1
- Cambridge, G., Stohl, W., Leandro, M. J., Migone, T.-S., Hilbert, D. M., & Edwards, J. C. W. (2006, March). Circulating levels of B lymphocyte stimulator in patients with rheumatoid arthritis following rituximab treatment: Relationships with B cell depletion, circulating antibodies, and clinical relapse. Arthritis & Rheumatism, 54(3), 723–732. https://doi.org/10.1002/art.21650
- Catrina, A. I., Trollmo, C., Af Klint, E., Engstrom, M., Lampa, J., Hermansson, Y., Klareskog, L., & Ulfgren, A. K. (2005, January). Evidence that anti-tumor necrosis factor therapy with both etanercept and infliximab induces apoptosis in macrophages, but not lymphocytes, in rheumatoid arthritis joints: Extended report. Arthritis & Rheumatism, 52(1), 61–72. https://doi.org/10.1002/art.20764
- Chabaud, M., Lubberts, E., Joosten, L., van den Berg, W., & Miossec, P. (2001). IL-17 derived from juxta-articular bone and synovium contributes to joint degradation in rheumatoid arthritis. Arthritis Research, 3(3), 168–177. https://doi.org/10.1186/ar294
- Chao, R., & Kavanaugh, A. (2019, December). Psoriatic arthritis: Newer and older therapies. Current Rheumatology Reports, 21(12), 75. https://doi.org/10.1007/s11926-019-0866-1
- Chara, L., Sanchez-Atrio, A., Perez, A., Cuende, E., Albarrán, F., Turrión, A., Chevarria, J., Del Barco, A. A., Sánchez, M. A., Monserrat, J., Prieto, A., de la Hera, A., Sanz, I., Diaz, D., & Alvarez-Mon, M. (2015, January 16). The number of circulating monocytes as biomarkers of the clinical response to methotrexate in untreated patients with rheumatoid arthritis. Journal of Translational Medicine, 13, 2. https://doi.org/10.1186/s12967-014-0375-y
- Chara, L., Sanchez-Atrio, A., Perez, A., Cuende, E., Albarrán, F., Turrión, A., Chevarria, J., Sánchez, M. A., Monserrat, J., de la Hera, A., Prieto, A., Sanz, I., Diaz, D., & Alvarez-Mon, M. (2012, July). Monocyte populations as markers of response to adalimumab plus MTX in rheumatoid arthritis. Arthritis Research & Therapy, 14(4), R175. https://doi.org/10.1186/ar3928
- Chatzidionysiou, K., Circiumaru, A., Rethi, B., Joshua, V., Engstrom, M., Hensvold, A., Af Klint, E., & Catrina, A. (2021, June). Tocilizumab decreases T cells but not macrophages in the synovium of patients with rheumatoid arthritis while it increases the levels of serum interleukin-6 and RANKL. RMD Open, 7(2), e001662. https://doi.org/10.1136/rmdopen-2021-001662
- Chen, Y., Yuan, J., Cai, Z., & Ma, Y. (2023, July). Efficacy of tumor necrosis factor inhibitor combined with intra-articular injection of triamcinolone acetonide in the treatment of refractory rheumatoid arthritis synovitis: A retrospective study. Clinical Rheumatology, 42(7), 1793–1799. https://doi.org/10.1007/s10067-023-06530-x
- Chiu, Y. G., Shao, T., Feng, C., Mensah, K. A., Thullen, M., Schwarz, E. M., & Ritchlin, C. T. (2010). CD16 (FcRγiii) as a potential marker of osteoclast precursors in psoriatic arthritis. Arthritis Research & Therapy, 12(1), R14. https://doi.org/10.1186/ar2915
- Cren, M., Nziza, N., Carbasse, A., Mahe, P., Dufourcq-Lopez, E., Delpont, M., Chevassus, H., Khalil, M., Mura, T., Duroux-Richard, I., Apparailly, F., Jeziorski, E., & Louis-Plence, P. (2020). Differential accumulation and activation of monocyte and dendritic cell subsets in inflamed synovial fluid discriminates between Juvenile idiopathic arthritis and septic arthritis. Frontiers in Immunology, 11, 1716. https://doi.org/10.3389/fimmu.2020.01716
- Cutolo, M., Bisso, A., Sulli, A., Felli, L., Briata, M., Pizzorni, C., & Villaggio, B. (2000, November). Antiproliferative and antiinflammatory effects of methotrexate on cultured differentiating myeloid monocytic cells (THP-1) but not on synovial macrophages from patients with rheumatoid arthritis. The Journal of Rheumatology, 27(11), 2551–2557.
- Cutolo, M., Capellino, S., Montagna, P., Sulli, A., Seriolo, B., & Villaggio, B. (2006, June). Anti-inflammatory effects of leflunomide in combination with methotrexate on co-culture of T lymphocytes and synovial macrophages from rheumatoid arthritis patients. Annals of the Rheumatic Diseases, 65 (6), 728–735. https://doi.org/10.1136/ard.2005.045641
- Cutolo, M., Soldano, S., Gotelli, E., Montagna, P., Campitiello, R., Paolino, S., Pizzorni, C., Sulli, A., Smith, V., & Tardito, S. (2021, December). CTLA4-Ig treatment induces M1–M2 shift in cultured monocyte-derived macrophages from healthy subjects and rheumatoid arthritis patients. Arthritis Research & Therapy, 23(1), 306. https://doi.org/10.1186/s13075-021-02691-9
- Cutolo, M., Sulli, A., Ghiorzo, P., Pizzorni, C., Craviotto, C., & Villaggio, B. (2003, April). Anti-inflammatory effects of leflunomide on cultured synovial macrophages from patients with rheumatoid arthritis. Annals of the Rheumatic Diseases, 62 (4), 297–302. https://doi.org/10.1136/ard.62.4.297
- Davignon, J. L., Hayder, M., Baron, M., Boyer, J.-F., Constantin, A., Apparailly, F., Poupot, R., & Cantagrel, A. (2013, April). Targeting monocytes/macrophages in the treatment of rheumatoid arthritis. Rheumatology (Oxford), 52(4), 590–598. https://doi.org/10.1093/rheumatology/kes304
- Dayer, J. M., & Choy, E. (2010, January). Therapeutic targets in rheumatoid arthritis: The interleukin-6 receptor. Rheumatology (Oxford), 49(1), 15–24. https://doi.org/10.1093/rheumatology/kep329
- Deora, A., Hegde, S., Lee, J., Choi, C.-H., Chang, Q., Lee, C., Eaton, L., Tang, H., Wang, D., Lee, D., Michalak, M., Tomlinson, M., Tao, Q., Gaur, N., Harvey, B., McLoughlin, S., Labkovsky, B., & Ghayur, T. (2017, May/June). Transmembrane TNF-dependent uptake of anti-TNF antibodies. Mabs, 9(4), 680–695. https://doi.org/10.1080/19420862.2017.1304869
- Diallo, K., Simons, N., Sayegh, S., Baron, M., Degboé, Y., Boyer, J.-F., Kruglov, A., Nedospasov, S., Novarino, J., Aloulou, M., Fazilleau, N., Constantin, A., Cantagrel, A., Davignon, J.-L., & Rauwel, B. (2021, April). Evidence for tmTNF reverse signaling in vivo: Implications for an arginase-1-mediated therapeutic effect of TNF inhibitors during inflammation. iScience, 24(4), 102331. https://doi.org/10.1016/j.isci.2021.102331
- Durand, M., Komarova, S. V., Bhargava, A., Trebec-Reynolds, D. P., Li, K., Fiorino, C., Maria, O., Nabavi, N., Manolson, M. F., Harrison, R. E., Dixon, S. J., Sims, S. M., Mizianty, M. J., Kurgan, L., Haroun, S., Boire, G., Lucena-Fernandes, M. D. F., & de Brum-Fernandes, A. J. (2013, January). Monocytes from patients with osteoarthritis display increased osteoclastogenesis and bone resorption: The in vitro Osteoclast Differentiation in Arthritis study. Arthritis & Rheumatism, 65(1), 148–158. https://doi.org/10.1002/art.37722
- Eder, L., Chandran, V., Ueng, J., Bhella, S., Lee, K.-A., Rahman, P., Pope, A., Cook, R. J., & Gladman, D. D. (2010, July). Predictors of response to intra-articular steroid injection in psoriatic arthritis. Rheumatology (Oxford), 49(7), 1367–1373. https://doi.org/10.1093/rheumatology/keq102
- Ferrao Blanco, M. N., Bastiaansen Jenniskens, Y. M., Kops, N., Chavli, A., Narcisi, R., Botter, S. M., Leenen, P. J. M., Osch, G. J. V. M., & Fahy, N. (2022, June). Intra-articular injection of triamcinolone acetonide sustains macrophage levels and aggravates osteophytosis during degenerative joint disease in mice. British Journal of Pharmacology, 179(11), 2771–2784. https://doi.org/10.1111/bph.15780
- Fiechter, R. H., de Jong, H. M., van Mens, L. J. J., Fluri, I. A., Tas, S. W., Baeten, D. L. P., Yeremenko, N. G., & van de Sande, M. G. H. (2021). IL-12p40/IL-23p40 blockade with ustekinumab decreases the synovial inflammatory infiltrate through modulation of multiple signaling pathways including MAPK-ERK and Wnt. Frontiers in Immunology, 12, 611656. https://doi.org/10.3389/fimmu.2021.611656
- Firestein, G. S. (2003, May). Evolving concepts of rheumatoid arthritis. Nature, 423(6937), 356–361. https://doi.org/10.1038/nature01661
- Fragoulis, G. E., McInnes, I. B., & Siebert, S. (2019, February). JAK-inhibitors. New players in the field of immune-mediated diseases, beyond rheumatoid arthritis. Rheumatology (Oxford), 58(Suppl 1), i43–i54. https://doi.org/10.1093/rheumatology/key276
- Fukue, R., Okazaki, Y., Gono, T., & Kuwana, M. (2022, August). Abatacept downregulates Fcγ receptor I on circulating monocytes: A potential therapeutic mechanism in patients with rheumatoid arthritis. Arthritis Research & Therapy, 24(1), 194. https://doi.org/10.1186/s13075-022-02886-8
- Gertel, S., Polachek, A., Furer, V., Paran, D., Tzemach, R., Levartovsky, D., Litinsky, I., Anouk, M., Meridor, K., Nochomovitz, H., Wollman, J., Berman, M., Borok, S., & Elkayam, O. (2023, March 2). Synovial monocytes from psoriatic and rheumatoid arthritis patients are modulated differently by TNF inhibitors and glucocorticoids: An ex-vivo study. Clinical and Experimental Rheumatology. https://doi.org/10.55563/clinexprheumatol/d3mfat
- Gomez-Aristizabal, A., Gandhi, R., Mahomed, N. N., Marshall, K. W., & Viswanathan, S. (2019, January). Synovial fluid monocyte/macrophage subsets and their correlation to patient-reported outcomes in osteoarthritic patients: A cohort study. Arthritis Research & Therapy, 21(1), 26. https://doi.org/10.1186/s13075-018-1798-2
- Greven, D. E., Cohen, E. S., Gerlag, D. M., Campbell, J., Woods, J., Davis, N., van Nieuwenhuijze, A., Lewis, A., Heasmen, S., McCourt, M., Corkill, D., Dodd, A., Elvin, J., Statache, G., Wicks, I. P., Anderson, I. K., Nash, A., Sleeman, M. A., & Tak, P. P. (2015, October). Preclinical characterisation of the GM-CSF receptor as a therapeutic target in rheumatoid arthritis. Annals of the Rheumatic Diseases, 74(10), 1924–1930. https://doi.org/10.1136/annrheumdis-2014-205234
- Guilliams, M., Mildner, A., & Yona, S. (2018, October). Developmental and functional heterogeneity of monocytes. Immunity, 49(4), 595–613. https://doi.org/10.1016/j.immuni.2018.10.005
- Hajialilo, M., Ghorbanihaghjo, A., Valaee, L., Kolahi, S., Rashtchizadeh, N., Amirkhiz, M. B., Malekmahdavi, I., & Khabbazi, A. (2016, December). A double-blind randomized comparative study of triamcinolone hexacetonide and dexamethasone intra-articular injection for the treatment of knee joint arthritis in rheumatoid arthritis. Clinical Rheumatology, 35(12), 2887–2891. https://doi.org/10.1007/s10067-016-3397-4
- Haringman, J. J., Gerlag, D. M., Zwinderman, A. H., Smeets, T. J., Kraan, M. C., Baeten, D., McInnes, I. B., Bresnihan, B., & Tak, P. P. (2005, June). Synovial tissue macrophages: A sensitive biomarker for response to treatment in patients with rheumatoid arthritis. Annals of the Rheumatic Diseases, 64 (6), 834–838. https://doi.org/10.1136/ard.2004.029751
- Haubruck, P., Pinto, M. M., Moradi, B., Little, C. B., & Gentek, R. (2021). Monocytes, macrophages, and their potential niches in synovial joints – Therapeutic targets in post-traumatic osteoarthritis? Frontiers in Immunology, 12, 763702. https://doi.org/10.3389/fimmu.2021.763702
- Huang, Q. Q., Birkett, R., Doyle, R., Shi, B., Roberts, E. L., Mao, Q., & Pope, R. M. (2018, January). The role of macrophages in the response to TNF inhibition in experimental arthritis. Journal of Immunology, 200(1), 130–138. https://doi.org/10.4049/jimmunol.1700229
- Humby, F., Lewis, M., Ramamoorthi, N., Hackney, J. A., Barnes, M. R., Bombardieri, M., Setiadi, A. F., Kelly, S., Bene, F., DiCicco, M., Riahi, S., Rocher, V., Ng, N., Lazarou, I., Hands, R., van der Heijde, D., Landewé, R. B. M., van der Helm van Mil, A., Alberto Cauli, A., ... Pitzalis, C. (2019, June). Synovial cellular and molecular signatures stratify clinical response to csDMARD therapy and predict radiographic progression in early rheumatoid arthritis patients. Annals of the Rheumatic Diseases, 78(6), 761–772. https://doi.org/10.1136/annrheumdis-2018-214539
- Hunter, D. J., March, L., & Chew, M. (2020, November). Osteoarthritis in 2020 and beyond: A Lancet Commission. Lancet (London, England), 396(10264), 1711–1712. https://doi.org/10.1016/S0140-6736(20)32230-3
- Karagiannidis, C., Akdis, M., Holopainen, P., Woolley, N. J., Hense, G., Rückert, B., Mantel, P.-Y., Menz, G., Akdis, C. A., Blaser, K., & Schmidt-Weber, C. B. (2004, December). Glucocorticoids upregulate FOXP3 expression and regulatory T cells in asthma. The Journal of Allergy and Clinical Immunology, 114(6), 1425–1433. https://doi.org/10.1016/j.jaci.2004.07.014
- Kirsch, A. H., Mahmood, A. A., Endres, J., Bohra, L., Bonish, B., Weber, K., & Fox, D. A. (1999,). Apoptosis of human T-cells: Induction by glucocorticoids or surface receptor ligation in vitro and ex vivo. Journal of Biological Regulators and Homeostatic Agents, 13(2), 80–89.
- Kivity, S., Zafrir, Y., Loebstein, R., Pauzner, R., Mouallem, M., & Mayan, H. (2014, November). Clinical characteristics and risk factors for low dose methotrexate toxicity: A cohort of 28 patients. Autoimmunity Reviews, 13(11), 1109–1113. https://doi.org/10.1016/j.autrev.2014.08.027
- Krasselt, M., Gruz, N., Pierer, M., Baerwald, C., & Wagner, U. (2022, June 19). IL-10 induced by mTNF Crosslinking-Mediated reverse signaling in a whole blood assay is predictive of response to TNFi therapy in rheumatoid arthritis. Journal of Personalized Medicine, 12(6). https://doi.org/10.3390/jpm12061003
- Kuo, D., Ding, J., Cohn, I. S., Zhang, F., Wei, K., Rao, D. A., Rozo, C., Sokhi, U. K., Shanaj, S., Oliver, D. J., Echeverria, A. P., DiCarlo, E. F., Brenner, M. B., Bykerk, V. P., Goodman, S. M., Raychaudhuri, S., Rätsch, G., Ivashkiv, L. B., & Donlin, L. T. (2019, May 8). HBEGF + macrophages in rheumatoid arthritis induce fibroblast invasiveness. Science Translational Medicine, 11(491). https://doi.org/10.1126/scitranslmed.aau8587
- Kurowska-Stolarska, M., & Alivernini, S. (2022, July). Synovial tissue macrophages in joint homeostasis, rheumatoid arthritis and disease remission. Nature Reviews: Rheumatology, 18(7), 384–397. https://doi.org/10.1038/s41584-022-00790-8
- Lalezari, J., Gathe, J., Brinson, C., Thompson, M., Cohen, C., Dejesus, E., Galindez, J., Ernst, J. A., Martin, D. E., & Palleja, S. M. (2011, June). Safety, efficacy, and pharmacokinetics of TBR-652, a CCR5/CCR2 antagonist, in HIV-1–infected, treatment-experienced, CCR5 antagonist–naive subjects. Journal of Acquired Immune Deficiency Syndromes (1999), 57(2), 118–125. https://doi.org/10.1097/QAI.0b013e318213c2c0
- Liu, B., Dhanda, A., Hirani, S., Williams, E. L., Sen, H. N., Martinez Estrada, F., Ling, D., Thompson, I., Casady, M., Li, Z., Si, H., Tucker, W., Wei, L., Jawad, S., Sura, A., Dailey, J., Hannes, S., Chen, P., Chien, J. L., ... Nussenblatt, R. B. (2015, June). CD14++CD16+ monocytes are enriched by glucocorticoid treatment and are functionally attenuated in driving effector T cell responses. Journal of Immunology, 194(11), 5150–5160. https://doi.org/10.4049/jimmunol.1402409
- Liu, W., Chen, Y., Zeng, G., Yang, S., Yang, T., Ma, M., & Song, W. (2021). Single-cell profiles of age-related osteoarthritis uncover underlying heterogeneity associated with disease progression. Frontiers in Molecular Biosciences, 8, 748360. https://doi.org/10.3389/fmolb.2021.748360
- Longobardi, L., Jordan, J. M., Shi, X. A., Renner, J. B., Schwartz, T. A., Nelson, A. E., Barrow, D. A., Kraus, V. B., & Spagnoli, A. (2018, September). Associations between the chemokine biomarker CCL2 and knee osteoarthritis outcomes: The Johnston County osteoarthritis project. Osteoarthritis & Cartilage / OARS, Osteoarthritis Research Society, 26(9), 1257–1261. https://doi.org/10.1016/j.joca.2018.04.012
- Lopez-Olivo, M. A., Siddhanamatha, H. R., Shea, B., Tugwell, P., Wells, G. A., & Suarez-Almazor, M. E. (2014, June). Methotrexate for treating rheumatoid arthritis. Cochrane Database of Systematic Reviews, 2014(6), CD000957. https://doi.org/10.1002/14651858.CD000957.pub2
- Ma, W., Liu, C., Wang, S., Xu, H., Sun, H., & Fan, X. (2020, December). Efficacy and safety of intra-articular injection of mesenchymal stem cells in the treatment of knee osteoarthritis: A systematic review and meta-analysis. Medicine (Baltimore), 99(49), e23343. https://doi.org/10.1097/MD.0000000000023343
- Makrygiannakis, D., Revu, S., Neregard, P., Af Klint, E., Snir, O., Grundtman, C., & Catrina, A. (2008). Monocytes are essential for inhibition of synovial T-cell glucocorticoid-mediated apoptosis in rheumatoid arthritis. Arthritis Research & Therapy, 10(6), R147. https://doi.org/10.1186/ar2582
- Mancuso, P., Raman, S., Glynn, A., Barry, F., & Murphy, J. M. (2019). Mesenchymal stem cell therapy for osteoarthritis: The critical role of the cell secretome. Frontiers in Bioengineering and Biotechnology, 7, 9. https://doi.org/10.3389/fbioe.2019.00009
- McAlindon, T. E., LaValley, M. P., Harvey, W. F., Price, L. L., Driban, J. B., Zhang, M., & Ward, R. J. (2017, May). Effect of intra-articular triamcinolone vs saline on knee cartilage volume and pain in patients with knee osteoarthritis: A randomized clinical trial. JAMA, 317(19), 1967–1975. https://doi.org/10.1001/jama.2017.5283
- McGarry, T., Hanlon, M. M., Marzaioli, V., Cunningham, C. C., Krishna, V., Murray, K., Hurson, C., Gallagher, P., Nagpal, S., Veale, D. J., & Fearon, U. (2021). Rheumatoid arthritis CD14 + monocytes display metabolic and inflammatory dysfunction, a phenotype that precedes clinical manifestation of disease. Clinical & Translational Immunology, 10(1), e1237. https://doi.org/10.1002/cti2.1237
- McInnes, I. B., Mease, P. J., Kirkham, B., Kavanaugh, A., Ritchlin, C. T., Rahman, P., van der Heijde, D., Landewé, R., Conaghan, P. G., Gottlieb, A. B., Richards, H., Pricop, L., Ligozio, G., Patekar, M., & Mpofu, S. (2015, September). Secukinumab, a human anti-interleukin-17A monoclonal antibody, in patients with psoriatic arthritis (FUTURE 2): A randomised, double-blind, placebo-controlled, phase 3 trial. Lancet (London, England), 386(9999), 1137–1146. https://doi.org/10.1016/S0140-6736(15)61134-5
- McInnes, I. B., & Schett, G. (2007, June). Cytokines in the pathogenesis of rheumatoid arthritis. Nature Reviews: Immunology, 7(6), 429–442. https://doi.org/10.1038/nri2094
- Meusch, U., Krasselt, M., Rossol, M., Baerwald, C., Klingner, M., & Wagner, U. (2015, August 7). In vitro response pattern of monocytes after tmTNF reverse signaling predicts response to anti-TNF therapy in rheumatoid arthritis. Journal of Translational Medicine, 13, 256. https://doi.org/10.1186/s12967-015-0620-z
- Meusch, U., Rossol, M., Baerwald, C., Hauschildt, S., & Wagner, U. (2009, September). Outside-to-inside signaling through transmembrane tumor necrosis factor reverses pathologic interleukin-1β production and deficient apoptosis of rheumatoid arthritis monocytes. Arthritis & Rheumatism, 60(9), 2612–2621. https://doi.org/10.1002/art.24778
- Moadab, F., Khorramdelazad, H., & Abbasifard, M. (2021, March 15). Role of CCL2/CCR2 axis in the immunopathogenesis of rheumatoid arthritis: Latest evidence and therapeutic approaches. Life Sciences, 269, 119034. https://doi.org/10.1016/j.lfs.2021.119034
- Mondadori, C., Palombella, S., Salehi, S., Talò, G., Visone, R., Rasponi, M., Redaelli, A., Sansone, V., Moretti, M., & Lopa, S. (2021, July). Recapitulating monocyte extravasation to the synovium in an organotypic microfluidic model of the articular joint. Biofabrication, 13(4), 045001. https://doi.org/10.1088/1758-5090/ac0c5e
- Mozo, L., Suarez, A., & Gutierrez, C. (2004, March). Glucocorticoids up-regulate constitutive interleukin-10 production by human monocytes. Clinical and Experimental Allergy: Journal of the British Society for Allergy and Clinical Immunology, 34(3), 406–412. https://doi.org/10.1111/j.1365-2222.2004.01824.x
- Mulherin, D., Fitzgerald, O., & Bresnihan, B. (1996, January). Synovial tissue macrophage populations and articular damage in rheumatoid arthritis. Arthritis & Rheumatism, 39(1), 115–124. https://doi.org/10.1002/art.1780390116
- Muller-Calleja, N., Manukyan, D., Canisius, A., Strand, D., & Lackner, K. J. (2017, May). Hydroxychloroquine inhibits proinflammatory signalling pathways by targeting endosomal NADPH oxidase. Annals of the Rheumatic Diseases, 76(5), 891–897. https://doi.org/10.1136/annrheumdis-2016-210012
- Mushtaq, S., Choudhary, R., & Scanzello, C. R. (2011, September). Non-surgical treatment of osteoarthritis-related pain in the elderly. Current Reviews in Musculoskeletal Medicine, 4(3), 113–122. https://doi.org/10.1007/s12178-011-9084-9
- Neumann, E., Riepl, B., Knedla, A., Lefèvre, S., Tarner, I. H., Grifka, J., Steinmeyer, J., Schölmerich, J., Gay, S., & Müller-Ladner, U. (2010). Cell culture and passaging alters gene expression pattern and proliferation rate in rheumatoid arthritis synovial fibroblasts. Arthritis Research & Therapy, 12(3), R83. https://doi.org/10.1186/ar3010
- Paoletti, A., Rohmer, J., Ly, B., Pascaud, J., Rivière, E., Seror, R., Le Goff, B., Nocturne, G., & Mariette, X. (2019, October). Monocyte/macrophage abnormalities specific to rheumatoid arthritis are linked to miR-155 and are differentially modulated by different TNF inhibitors. Journal of Immunology, 203(7), 1766–1775. https://doi.org/10.4049/jimmunol.1900386
- Ptacek, J., Hawtin, R. E., Sun, D., Louie, B., Evensen, E., Mittleman, B. B., Cesano, A., Cavet, G., Bingham III, C. O., Cofield, S. S., & Curtis, J. R. (2021). Diminished cytokine-induced Jak/STAT signaling is associated with rheumatoid arthritis and disease activity. PLoS One, 16(1), e0244187. https://doi.org/10.1371/journal.pone.0244187
- Qian, T., Fujiwara, N., Koneru, B., Ono, A., Kubota, N., Jajoriya, A. K., Tung, M. G., Crouchet, E., Song, W.-M., Marquez, C. A., Panda, G., Hoshida, A., Raman, I., Li, Q.-Z., Lewis, C., Yopp, A., Rich, N. E., Singal, A. G., Nakagawa, S., ... Hoshida, Y. (2022, April). Molecular signature predictive of long-term liver fibrosis progression to inform antifibrotic drug development. Gastroenterology, 162(4), 1210–1225. https://doi.org/10.1053/j.gastro.2021.12.250
- Quero, L., Hanser, E., Manigold, T., Tiaden, A. N., & Kyburz, D. (2017, November). TLR2 stimulation impairs anti-inflammatory activity of M2-like macrophages, generating a chimeric M1/M2 phenotype. Arthritis Research & Therapy, 19(1), 245. https://doi.org/10.1186/s13075-017-1447-1
- Raghu, H., Lepus, C. M., Wang, Q., Wong, H. H., Lingampalli, N., Oliviero, F., Punzi, L., Giori, N. J., Goodman, S. B., Chu, C. R., Sokolove, J. B., & Robinson, W. H. (2017, May). CCL2/CCR2, but not CCL5/CCR5, mediates monocyte recruitment, inflammation and cartilage destruction in osteoarthritis. Annals of the Rheumatic Diseases, 76(5), 914–922. https://doi.org/10.1136/annrheumdis-2016-210426
- Roberts, C. A., Dickinson, A. K., & Taams, L. S. (2015). The interplay between monocytes/macrophages and CD4(+) T cell subsets in rheumatoid arthritis. Frontiers in Immunology, 6, 571. https://doi.org/10.3389/fimmu.2015.00571
- Sachsenweger, R. (1960). The variability of the apparent angle of strabismus. Albrecht von Graefe’s Archiv fur Ophthalmologie, 162, 166–177. https://doi.org/10.1007/BF00682419
- Safi, W., Kuehnl, A., Nussler, A., Eckstein, H.-H., & Pelisek, J. (2016, April). Differentiation of human CD14+ monocytes: An experimental investigation of the optimal culture medium and evidence of a lack of differentiation along the endothelial line. Experimental & Molecular Medicine, 48(4), e227. https://doi.org/10.1038/emm.2016.11
- Schmidt, T., Berthold, E., Arve-Butler, S., Gullstrand, B., Mossberg, A., Kahn, F., Bengtsson, A. A., Månsson, B., & Kahn, R. (2020, August). Children with oligoarticular juvenile idiopathic arthritis have skewed synovial monocyte polarization pattern with functional impairment—A distinct inflammatory pattern for oligoarticular juvenile arthritis. Arthritis Research & Therapy, 22(1), 186. https://doi.org/10.1186/s13075-020-02279-9
- Schmidt, T., Dahlberg, A., Berthold, E., Król, P., Arve-Butler, S., Rydén, E., Najibi, S. M., Mossberg, A., Bengtsson, A. A., Kahn, F., Månsson, B., & Kahn, R. (2023). Synovial monocytes contribute to chronic inflammation in childhood-onset arthritis via IL-6/STAT signalling and cell-cell interactions. Frontiers in Immunology, 14, 1190018. https://doi.org/10.3389/fimmu.2023.1190018
- Schumacher, H. R., & Chen, L. X. (2005, November). Injectable corticosteroids in treatment of arthritis of the knee. The American Journal of Medicine, 118(11), 1208–1214. https://doi.org/10.1016/j.amjmed.2005.05.003
- Shahrara, S., Pickens, S. R., Dorfleutner, A., & Pope, R. M. (2009, March). IL-17 induces monocyte migration in rheumatoid arthritis. Journal of Immunology, 182(6), 3884–3891. https://doi.org/10.4049/jimmunol.0802246
- Shipa, M. R. A., Amarnani, R., Yeoh, S. A., Mainuddin, M. D., & Ehrenstein, M. R. (2021, December). Early reduction in circulating monocyte count predicts maintenance of remission in patients with rheumatoid arthritis treated with anti-TNF therapy. Annals of the Rheumatic Diseases, 80(12), 1628–1629. https://doi.org/10.1136/annrheumdis-2021-220642
- Smolen, J. S., Landewe, R., Bijlsma, J., Burmester, G., Chatzidionysiou, K., Dougados, M., Nam, J., Ramiro, S., Voshaar, M., van Vollenhoven, R., Aletaha, D., Aringer, M., Boers, M., Buckley, C. D., Buttgereit, F., Bykerk, V., Cardiel, M., Combe, B., Cutolo, M., ... van der Heijde, D. (2017, June). EULAR recommendations for the management of rheumatoid arthritis with synthetic and biological disease-modifying antirheumatic drugs: 2016 update. Annals of the Rheumatic Diseases, 76(6), 960–977. https://doi.org/10.1136/annrheumdis-2016-210715
- Stankovic, A., Slavic, V., Stamenkovic, B., Kamenov, B., Bojanovic, M., & Mitrovic, D. R. (2009). Serum and synovial fluid concentrations of CCL2 (MCP-1) chemokine in patients suffering rheumatoid arthritis and osteoarthritis reflect disease activity. Bratislavske lekarske listy, 110(10), 641–646.
- Tacke, F., & Randolph, G. J. (2006). Migratory fate and differentiation of blood monocyte subsets. Immunobiology, 211(6–8), 609–618. https://doi.org/10.1016/j.imbio.2006.05.025
- Tak, P. P. (2012, April). A personalized medicine approach to biologic treatment of rheumatoid arthritis: A preliminary treatment algorithm. Rheumatology (Oxford), 51(4), 600–609. https://doi.org/10.1093/rheumatology/ker300
- Tardito, S., Martinelli, G., Soldano, S., Paolino, S., Pacini, G., Patane, M., Alessandri, E., Smith, V., & Cutolo, M. (2019, November). Macrophage M1/M2 polarization and rheumatoid arthritis: A systematic review. Autoimmunity Reviews, 18(11), 102397. https://doi.org/10.1016/j.autrev.2019.102397
- Taylor, P. C., Peters, A. M., Paleolog, E., Chapman, P. T., Elliott, M. J., McCloskey, R., Feldmann, M., & Maini, R. N. (2000, January). Reduction of chemokine levels and leukocyte traffic to joints by tumor necrosis factor α blockade in patients with rheumatoid arthritis. Arthritis & Rheumatism, 43(1), 38–47. https://doi.org/10.1002/1529-0131(200001)43:1<38:AID-ANR6>3.0.CO;2-L
- Thomas, G., Tacke, R., Hedrick, C. C., & Hanna, R. N. (2015, June). Nonclassical patrolling monocyte function in the vasculature. Arteriosclerosis, Thrombosis, and Vascular Biology, 35(6), 1306–1316. https://doi.org/10.1161/ATVBAHA.114.304650
- Thurlings, R. M., Vos, K., Wijbrandts, C. A., Zwinderman, A. H., Gerlag, D. M., & Tak, P. P. (2008, July). Synovial tissue response to rituximab: Mechanism of action and identification of biomarkers of response. Annals of the Rheumatic Diseases, 67(7), 917–925. https://doi.org/10.1136/ard.2007.080960
- Toubi, E., Kessel, A., Slobodin, G., Boulman, N., Pavlotzky, E., Zisman, D., Rozenbaum, M., & Rosner, I. (2007, June). Changes in macrophage function after rituximab treatment in patients with rheumatoid arthritis. Annals of the Rheumatic Diseases, 66(6), 818–820. https://doi.org/10.1136/ard.2006.062505
- Tsukamoto, M., Seta, N., Yoshimoto, K., Suzuki, K., Yamaoka, K., & Takeuchi, T. (2017, February). CD14(bright)CD16+ intermediate monocytes are induced by interleukin-10 and positively correlate with disease activity in rheumatoid arthritis. Arthritis Research & Therapy, 19(1), 28. https://doi.org/10.1186/s13075-016-1216-6
- Tsukamoto, M., Suzuki, K., Seta, N., & Takeuchi, T. (2018,). Increased circulating CD14brightCD16+ intermediate monocytes are regulated by TNF-α and IL-6 axis in accordance with disease activity in patients with rheumatoid arthritis. Clinical and Experimental Rheumatology, 36(4), 540–544.
- Tu, J., Hong, W., Zhang, P., Wang, X., Körner, H., & Wei, W. (2018). Ontology and function of fibroblast-like and macrophage-like synoviocytes: How do they talk to each other and can they be targeted for rheumatoid arthritis therapy? Frontiers in Immunology, 9, 1467. https://doi.org/10.3389/fimmu.2018.01467
- Tucci, G., Garufi, C., Pacella, I., Zagaglioni, M., Pinzon Grimaldos, A., Ceccarelli, F., Conti, F., Spinelli, F. R., & Piconese, S. (2022). Baricitinib therapy response in rheumatoid arthritis patients associates to STAT1 phosphorylation in monocytes. Frontiers in Immunology, 13, 932240. https://doi.org/10.3389/fimmu.2022.932240
- Uchiyama, R., Toyoda, E., Maehara, M., Wasai, S., Omura, H., Watanabe, M., & Sato, M. (2021, February 26). Effect of platelet-rich plasma on M1/M2 macrophage polarization. International Journal of Molecular Sciences, 22(5). https://doi.org/10.3390/ijms22052336.
- Wang, Y., Yu, D., Liu, Z., Zhou, F., Dai, J., Wu, B., Zhou, J., Heng, B. C., Zou, X. H., Ouyang, H., & Liu, H. (2017, August). Exosomes from embryonic mesenchymal stem cells alleviate osteoarthritis through balancing synthesis and degradation of cartilage extracellular matrix. Stem Cell Research & Therapy, 8(1), 189. https://doi.org/10.1186/s13287-017-0632-0
- Xue, J., Xu, L., Zhu, H., Bai, M., Li, X., Zhao, Z., Zhong, H., Cheng, G., Li, X., Hu, F., & Su, Y. (2020, September). CD14+CD16− Monocytes are the main precursors of osteoclasts in rheumatoid arthritis via expressing Tyro3TK. Arthritis Research & Therapy, 22(1), 221. https://doi.org/10.1186/s13075-020-02308-7
- Yager, N., Cole, S., Lledo Lara, A., Maroof, A., Penkava, F., Knight, J. C., Bowness, P., & Al-Mossawi, H. (2021, December). Ex vivo mass cytometry analysis reveals a profound myeloid proinflammatory signature in psoriatic arthritis synovial fluid. Annals of the Rheumatic Diseases, 80(12), 1559–1567. https://doi.org/10.1136/annrheumdis-2021-220280
- Yamaoka, K. (2016, June). Janus kinase inhibitors for rheumatoid arthritis. Current Opinion in Chemical Biology, 32, 29–33. https://doi.org/10.1016/j.cbpa.2016.03.006
- Yarilina, A., Xu, K., Chan, C., & Ivashkiv, L. B. (2012, December). Regulation of inflammatory responses in tumor necrosis factor-activated and rheumatoid arthritis synovial macrophages by JAK inhibitors. Arthritis & Rheumatism, 64(12), 3856–3866. https://doi.org/10.1002/art.37691
- Yoon, B. R., Yoo, S. J., Choi, Y., Chung, Y.-H., Kim, J., Yoo, I. S., Kang, S. W., & Lee, W.-W. (2014). Functional phenotype of synovial monocytes modulating inflammatory T-cell responses in rheumatoid arthritis (RA). PLoS One, 9(10), e109775. https://doi.org/10.1371/journal.pone.0109775
- Zhang, B., Shan, H., Li, D., Li, Z.-R., Zhu, K.-S., Jiang, Z.-B., & Huang, M.-S. (2012, November). Different methods of detaching adherent cells significantly affect the detection of TRAIL receptors. Tumori Journal, 98(6), 800–803. https://doi.org/10.1177/030089161209800619
- Zhang, F., Wei, K., Slowikowski, K., Fonseka, C. Y., Rao, D. A., Kelly, S., Goodman, S. M., Tabechian, D., Hughes, L. B., Salomon-Escoto, K., Watts, G. F. M., Jonsson, A. H., Rangel-Moreno, J., Meednu, N., Rozo, C., Apruzzese, W., Eisenhaure, T. M., Lieb, D. J., Boyle, D. L., ... Raychaudhuri, S. (2019, July). Defining inflammatory cell states in rheumatoid arthritis joint synovial tissues by integrating single-cell transcriptomics and mass cytometry. Nature Immunology, 20(7), 928–942. https://doi.org/10.1038/s41590-019-0378-1
- Ziegler-Heitbrock, L. (2015). Blood monocytes and their subsets: Established features and open questions. Frontiers in Immunology, 6, 423. https://doi.org/10.3389/fimmu.2015.00423
- Ziegler-Heitbrock, L., Ancuta, P., Crowe, S., Dalod, M., Grau, V., Hart, D. N., Leenen, P. J. M., Liu, Y.-J., MacPherson, G., Randolph, G. J., Scherberich, J., Schmitz, J., Shortman, K., Sozzani, S., Strobl, H., Zembala, M., Austyn, J. M., & Lutz, M. B. (2010, October). Nomenclature of monocytes and dendritic cells in blood. Blood, 116(16), e74–80. https://doi.org/10.1182/blood-2010-02-258558