ABSTRACT
PURPOSE
Over the past two decades, advancements in imaging modalities have significantly evolved the diagnosis and management of retinal diseases. Through these novel platforms, we have developed a deeper understanding of the anatomy of the choroidal vasculature and the choriocapillaris. The recently developed tools such as optical coherence tomography (OCT) and OCT angiography (OCTA) have helped elucidate the pathological mechanisms of several posterior segment diseases. In this review, we have explained the anatomy of the choriocapillaris and its close relationship to the outer retina and retinal pigment epithelium.
METHODS
A comprehensive search of medical literature was performed through the Medline/PubMed database using search terms: choriocapillaris, choroid, quantification, biomarkers, diabetic retinopathy, age-related macular degeneration, choroidal blood flow, mean blur rate, flow deficit, optical coherence tomography, optical coherence tomography angiography, fluorescein angiography, indocyanine green angiography, OCTA, Doppler imaging, uveitis, choroiditis, white dot syndrome, tubercular serpiginous-like choroiditis, choroidal granuloma, pachychoroid, toxoplasmosis, central serous chorioretinopathy, multifocal choroiditis, choroidal neovascularization, choroidal thickness, choroidal vascularity index, choroidal vascular density, and choroidal blood supply. The search terms were used either independently or combined with choriocapillaris/choroid.
RESULTS
The imaging techniques which are used to qualitatively and quantitatively analyze choriocapillaris are described. The pathological alterations in the choriocapillaris in an array of conditions such as diabetes mellitus, age-related macular degeneration, pachychoroid spectrum of diseases, and inflammatory disorders have been comprehensively reviewed. The future directions in the study of choriocapillaris have also been discussed.
CONCLUSION
The development of imaging tools such as OCT and OCTA has dramatically improved the assessment of choriocapillaris in health and disease. The choriocapillaris can be delineated from the stromal choroid using the OCT and quantified by manual or automated methods. However, these techniques have inherent limitations due to the lack of an anatomical distinction between the choriocapillaris and the stromal choroid, which can be overcome with the use of predefined segmentation slabs on OCT and OCTA. These segmentation slabs help in standardizing the choriocapillaris imaging and obtain repeatable measurements in various conditions such as diabetic retinopathy, age-related macular degeneration, pachychoroid spectrum, and ocular inflammations. Additionally, Doppler imaging has also been effectively used to evaluate the choroidal blood flow and quantifying the choriocapillaris and establishing its role in the pathogenesis of various retinochoroidal diseases. As tremendous technological advancements such as wide-field and ultra-wide field imaging take place, there will be a significant improvement in the ease and accuracy of quantifying the choriocapillaris.
INTRODUCTION
The capillary lamina of the choroid, also known as the choriocapillaris, is a single layer of fenestrated capillary bed located beneath the Bruch’s membrane and is approximately 10–30 µm in thickness.Citation1,Citation2 This choroidal vascular bed was first described by J. Hovius in 1702, and a century later, the term choriocapillaris was coined by D. Eschricht.Citation1 In 1963, Garron described the choriocapillaris as a single continuous layer of capillaries consisting of a ‘fine single-layered vascular net’.Citation3 Over the next decade, Golder and Gay developed an understanding of choriocapillaris anatomy using in vivo studies by embolizing the choroidal arteries in animal models.Citation4,Citation5 The knowledge about the choriocapillaris morphology was further evolved with the application of injectable dyes to study the choroidal vasculature.Citation6
The choriocapillaris is densely arranged with intervascular septa in the posterior pole of the eye and has a wider lumen with a large flat surface area that allows metabolic exchange with the outer retinal layer.Citation2,Citation7 Although the significance of choriocapillaris has been extensively reported in the literature, however, the understanding of the structure and function of this vascular bed continues to be limited. The diseases of the posterior pole of the eye have shown significant structural or functional changes in the choriocapillaris. These pathologies are either directly caused by the changes in the choriocapillaris anatomy or are thought to be significantly influenced by the changes in the choriocapillaris. Altered choriocapillaris blood flow can result in loss of photoreceptor functions. Furthermore, the reduced blood flow in the retina can modulate the production and expression of vascular endothelial growth factors and consequently drive the changes in choroidal vasculature. Understanding this closed-loop feedback relationship between the choroid and retina is essential for the researchers and clinicians evaluating posterior segment pathologies.
Imaging of the choriocapillaris can be challenging due to the optical filtering effect caused by the overlying retinal pigment epithelium (RPE), which creates obstacles to observing blood flow dynamics in the choriocapillaris in vivo.Citation1 Hyper-reflectivity of the RPE, along with back-shadowing due to pre-retinal or intraretinal pathologies such as cystoid spaces or inflammation can obscure the visualization of choriocapillaris. Conventional angiographies such as fluorescein angiography (FA) and indocyanine green angiography (ICGA) have been utilized in studying the correlations between choroidal perfusion and ocular disease.Citation8,Citation9 However, FA and ICGA are invasive, time-consuming, and require a contrast agent, which may induce systemic adverse effects.Citation9,Citation10
In this comprehensive review, we have described the detailed patho-anatomy of the choriocapillaris and its close relationship with the outer retina and the RPE. Various imaging tools such as ICGA, optical coherence tomography (OCT) and OCT angiography (OCTA) used to assess the choriocapillaris perfusion have been described. Further, we have comprehensively reviewed the pathological alterations of choriocapillaris in various systemic and ocular diseases involving the posterior pole such as diabetes, age-related macular degeneration (AMD), pachychoroid spectrum of diseases, and inflammatory posterior uveitic entities. Finally, limitations and advances in imaging of the choriocapillaris have been summarized.
ANATOMY OF THE CHORIOCAPILLARIS
The choroid is a network of blood vessels, collagen fibers, fibroblasts, and melanocytes and contributes to the uveal tract posteriorly and the ciliary zone anteriorly.Citation2 Choroidal thickness is approximately 0.2 mm at the posterior pole and thinner at the anterior poles.Citation11 Traditionally, the choroid was considered the primary source of nutrition and oxygen supply for the outer retina.Citation2 However, recent studies have revealed its critical role in thermoregulation, intraocular pressure modulation, emmetropization, positional adjustment of the retina, and secreting growth factors. Additionally, the choroid functions to drain the aqueous humor from the anterior chamber through the uveoscleral pathway.Citation12
The development of human eye begins towards the end of the fourth week of gestation when the neural tube folds to form the two optic vesicles at the cranial end, which invaginates into optic cups.Citation13 The mesenchymal layer surrounding the optic cups differentiates into internal and external layers, which further differentiate into choroid and sclera, respectively. The choroidal cells, such as the melanocytes, are derived from the neural crest cells, whereas mesoderm gives rise to the endothelial cells of the choroidal blood vessels. The choriocapillaris begins to differentiate during the fourth and fifth weeks. The increasing fenestrations, luminal enlargement, and basement membrane of the capillaries appear by the ninth week of gestation. The deposition of collagen between the basement membrane of the RPE and the capillaries appears in the subsequent 3 weeks.
The choroid comprises three distinct layers – the Bruch’s membrane, vascular layer, and suprachoroidal lamina. The luminal size of the choroidal vasculature increases progressively from deep to superficial layers, consisting of the choriocapillaris, Sattler’s layer, and Haller’s layer (). The choriocapillaris is the innermost layer of the choroid found beneath the Bruch’s membrane and consists of a single layer of endothelial cells around the capillaries, with a characteristically flat and wide appearance.Citation2 The capillary endothelial cells show a functional anatomical pattern of nuclei found closer to the scleral surface, allowing an efficient exchange between the choriocapillaris and RPE.Citation14 The capillaries have a distinctly wider lumen than other capillaries bed found in the body, allowing more than one red blood cell to pass through. Additionally, there is a greater frequency of fenestrations located anteriorly facing the retina, which allow plasma protein such as vitamin A to be delivered to the RPE.Citation15 Unlike the capillaries found elsewhere in the body, choriocapillaris have a distinct planar geometry, which allows diffusion of molecules three to four times more than the kidneys. The specialized anatomy of the choriocapillaris lamina allows it to provide nourishment to the RPE, filtering metabolic waste and regulating outer ocular temperature.Citation2 The choriocapillaris are more frequently packed densely at the posterior pole of the eye. The capillary beds are organized into lobules and separated by avascular septa allowing each lobule with its precapillary arteriole and postcapillary venule flow.Citation16,Citation17 Each lobule has two peripheral venules inserted into the capillaries at a right angle. The high permeability of the fenestrated capillaries also maintains a high oncotic pressure that allows fluid to move out of the retina through the choroid layers and eventually drain out of the sclera.Citation15,Citation18
Figure 1. Histopathological specimen of the choroid in a healthy post-mortem eye. The choriocapillaris consists of small vessels under the Bruch’s membrane in the inner choroid (labeled with black arrowheads). There is no anatomical demarcation between the choriocapillaris and the deeper choroidal vessels (Haller’s and Sattler’s layers). The lumen of the deeper choroidal vessels is filled with blood (black arrows).
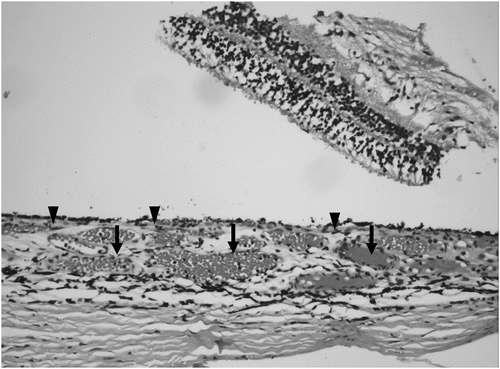
The arterial blood supply to the choroid predominantly arises from the ophthalmic artery branches from the internal carotid artery.Citation19 The ophthalmic artery branches into two posterior ciliary arteries (in 48% of eyes) or three posterior ciliary arteries (in 38% of eyes). The posterior ciliary branches into the medial and lateral posterior ciliary arteries, showing different anatomical variations. The long posterior ciliary arteries arise from the medial and lateral posterior ciliary arteries, whereas the short posterior ciliary arteries arise from other branches of the posterior ciliary arteries. These branches are further divided into choroidal arterioles, which ultimately branch and segmentally feed into the choriocapillaris lobule.Citation17 The recurrent branches of the long posterior and anterior ciliary artery supply the anterior choriocapillaris, and the short posterior ciliary arteries primarily supply the posterior choriocapillaris.
The Bruch’s membrane is a thin fibroelastic layer found between the choriocapillaris and the outer retina and is composed of elements of the outer retina and choriocapillaris. It provides a filtration barrier to regulate exchange between the retina pigment epithelium and choriocapillaris. The anatomy of the Bruch’s membrane makes it permeable to ions, metabolic waste, and glucose, and the age-related changes in its anatomy play a significant role in disease incidence.Citation11
IN VIVO TECHNIQUES OF IMAGING THE CHORIOCAPILLARIS
Imaging of the choroid can be performed in vivo using traditional dye-based angiography techniques such as FA and ICGA. However, FA is very limited in visualization of the choroid, and vascular flow through the choroid appears only as the initial “choroidal flush” within first few seconds after injecting the dye. Therefore, ICGA is considered as the gold standard in imaging of the choriocapillaris. Since the dye used in ICGA is 98% protein-bound, it has limited diffusion through the choriocapillaris. Specialized fundus cameras are needed to obtain ICGA images. ICGA is extensively used to image the choroidal vessels and choriocapillaris. Ischemia of the choriocapillaris appears as areas of hypofluorescence in the early phase ICGA, which may be hypo- or isofluorescent in the late phase. In advanced stages of the disease, there may be well-defined areas of hypofluorescence with visible large choroidal vessels suggestive of choriocapillaris atrophy. ICGA also allows qualitative analysis of large choroidal vessels; for instance, in pachychoroid spectrum of diseases, diffuse fuzziness of the large choroidal vessels on ICGA indicates hyperpermeability of the choroidal vasculature, which is considered to be pathogenic of this condition.
OCT has revolutionized imaging of the choroid and the choriocapillaris.Citation20 It provides a high-resolution three-dimensional structural image of the different layers of the choroid. There is no optical delineation of different layers of the choroid on OCT. Choriocapillaris appears as a thin hyporeflective layer with a dotted pattern underneath the RPE. Due to the hyper-reflectivity of the overlying RPE, minor alterations in the choriocapillaris may be obscured. Some of the limitations in assessing the choriocapillaris were overcome by the enhanced-depth imaging OCT (EDI-OCT).Citation21 The EDI-OCT allows deeper penetration of the eye, enabling the acquisition of quantitative information about the choroidal thickness.Citation21 Swept-source OCT (SS-OCT) provides a more precise, detailed evaluation of the choriocapillaris compared to EDI-OCT by utilizing longer and broader wavelengths and multiple images, allowing improvement in the signal-to-noise ratio and enhancing the image quality.Citation22
OCTA provides depth-resolved and high-resolution images enabling detailed observation of pathophysiological changes, monitoring disease progression, and managing outcomes in chorioretinal diseases.Citation23–27 The underlying principle of OCTA is based on detecting blood flow in the deeper layers including the choriocapillaris. It combines these findings with the structural images from OCT scans.Citation25 Using the OCTA, the lobular structure of the choriocapillaris is easily visualized with a densely packed honeycomb pattern closer to the macula. Additionally, OCTA can be used in patients with a contraindication to dyes. The newer advancements in OCTA have further added to the quality of choriocapillaris imaging and provided information on vascular changes in vivo.Citation27 The ultrahigh-speed SS-OCTA is more reliable at visualizing the deeper capillary layer by using a longer wavelength for deeper penetration into the choroid and increased image acquisition rate. The variable interscan time analysis (VISTA) method with SS-OCTA also provides relative blood flow speed measurements in diseases like diabetic retinopathy (DR) and AMD.Citation27–29
Availability of EDI-OCT, SS-OCT, and OCTA have added to the diagnostic armamentarium of retina specialists through early detection of chorioretinal vascular diseases and swift management, leading to better visual outcomes. Moreover, the sustained technological evolution provides clinicians and researchers tools to explore pathophysiology in the most devastating diseases of the posterior pole of the eye.
MEASUREMENT OF CHORIOCAPILLARIS VASCULAR FLOW
Thickness-Based Method of Assessment
The changes in the choroid thickness are believed to be an indicator of the impact of systemic diseases on the eye. The high-resolution images and precise choroidal thickness data obtained using EDI-OCT have enabled ophthalmologists to identify ophthalmic disease-associated changes in the choroid.Citation21,Citation30,Citation31 EDI-OCT has also been used to assess the treatment response by measuring choroidal thickness changes. The semiautomated segmentation of vascular and stromal tissue on this imaging platform helps distinguish the differences between the two components.Citation32,Citation33 On EDI-OCT, the choriocapillaris slab appears as a thin layer with a hyper-reflective dotted pattern beneath the RPE-Bruch’s membrane complex.Citation34 Deeper to the choriocapillaris, the larger Haller’s and Sattler’s layer vessels appear as round-to-oval hyporeflective areas corresponding to the lumen of the blood vessels. Using the EDI-OCT, Invernizzi and colleagues reported that post-ischemia, the thickness of the choriocapillaris layer increases, and there is a loss of the typical dotted pattern beneath the RPE–Bruch’s complex. This area of abnormal appearance co-localizes exactly with the hypofluorescent areas on ICGA, suggesting choriocapillaris flow loss.Citation34
The choroidal vascularity index (CVI) is another tool that can be used to assess the choroidal flow using two-dimensional OCT scans. CVI is the ratio of luminal area to total choroidal area using binarization of cross-sectional OCT images.Citation30,Citation31 It has been used in evaluating circulatory alteration in ocular diseases, particularly in the subfoveal choroid.Citation31,Citation35 However, CVI cannot be used to measure only the choriocapillaris flow.
Flow-Based Method of Assessment
Choroidal flow has been primarily assessed using the ICGA imaging in vivo.Citation18 However, ICGA is an invasive technique with limited visualization of the flow velocity in the choroidal vasculature. ICGA also cannot separately provide information on the choriocapillaris flow by segmenting it from other deeper choroidal layers. With the introduction of OCTA, high contrast, well-defined images of the choroidal, especially the choriocapillaris, are made possible.Citation21 However, despite the advances in OCTA, a significant limitation is the inability of this platform to provide an insight into the flow dynamics of choroidal vasculature.Citation36 Projection from superficial retinal vessels and hyper-reflective RPE are other limitations in employing OCTA for choriocapillaris analysis.
Recently, laser variable interscan time analysis (VISTA) imaging technique based on the SS-OCT images has shown promising data in displaying relative choroidal blood flow velocities.Citation37 VISTA coupled with SS-OCTA analyzes flow speeds by creating a colored flow speed map showing no flow, slow flow, and faster flow to help further investigate the pathophysiology of diseases.Citation7 However, the VISTA imaging technique only provides relative blood flow measurement for slower flow speeds (less than 300 μm/s).Citation27
DIABETIC EYE DISEASE
Choriocapillaris Flow Alterations in Diabetes
Diabetes mellitus (DM) is commonly associated with microvascular complications, and its impact on ocular health is taking center stage with rapidly rising global prevalence.Citation38 While DR has been addressed extensively by numerous researchers, choriocapillaris damage and associated changes in the choroidal hemodynamics are described relatively scarcely in published literature.Citation39
Before the introduction of modern technologies such as OCT and OCTA, choroidal circulation was evaluated by the measurement of pulsatile blood flow (PBF). Langham and colleagues used the first-generation pneumatic probe to report a decrease in PBF in diabetic eyes compared to healthy eyes and observed a negative trend in relation to DR progression.Citation40 On the contrary, the later work of MacKinnon and Geyer groups showed the opposite relation.Citation41,Citation42 Shmidt and colleagues utilized the Langham ocular flow system to assess PBF in younger patients with insulin-dependent DM and found no significant difference between the two groups (). Therefore, the use of PBF to measure the choroidal blood flow is controversial.
Table 1. Pulsatile blood flow in diabetic and aged eyes, studies summary.
To determine choriocapillaris blood flow in foveal regions, Nagaoka and colleagues used the laser doppler velocity flowmetry technique.Citation43 They reported that the choroid blood flow and foveolar choroidal blood velocity were significantly impaired in all diabetic groups compared to controls subjects. They found that the decrease in foveolar blood volume was only observed in NPDR with macular edema. A few other studies have hypothesized that decreased choroidal circulation precedes and further contributes to retinopathy progression to proliferative stages and macular edema development, mainly by triggering tissue hypoxia and vascular endothelial growth factor overexpression.Citation40,Citation44,Citation45
Flow Abnormalities on Imaging
Diabetic Choroidopathy
Diabetic choroid vasculature abnormalities, known as diabetic choroidopathy, were first in the 1980s using early electron microscopy imaging of human choroid.Citation46,Citation47 In 1985, Hidayat and colleagues observed basement thickening, narrowed or completely obliterated vessels, choriocapillaris “dropout,” and neovascularization in young patients. Subsequently, these findings were confirmed and further characterized by other researchers.Citation47–49 The current clinical definition of diabetic choroidopathy includes vessel wall thickening and basal laminar deposits, dilatation and obstruction of capillaries, dropout areas with diminished or absent perfusion, microaneurysms and neovascularization, increased vascular tortuosity.Citation48–50
ICGA has been used to assess the choriocapillaris flow in diabetic eyes by certain authors. Weinberger and colleagues assessed 42 patients with NPDR and reported the selective filing of choriocapillaris in ischemic areas in 12% of the eyes. In later studies, Shiragami and Hua groups used a similar ICGA-based technique for evaluating diabetic eyes and reported identical abnormalities.Citation51,Citation52 They reported a correlation between hypofluorescent spots and choroidal non-perfusion regions with DR severity (). While multiple studies have reported choroidal thickness alterations in diabetes, the conclusions remain conflicting. Choi et al. used ultra-high-speed SS-OCT to report choriocapillaris abnormalities in all diabetic eyes, with higher severity of those changes and proportion of affected eyes in the PDR group.Citation29 Interestingly, OCTA imaging of young patients did not reveal any significant difference in vessel density of the choriocapillaris.Citation53
Table 2. Choroidal flow imaging studies in diabetic retinopathy.
As highlighted in the previous sections, indirect vasculature quantification methods such as CVI and choroidal vascular density (CVD) were introduced due to the unreliability of choroidal thickness and other metrics.Citation31,Citation54–56 The studies using these metrics consistently observed decreased CVI and CVD in diabetic eyes, with and without DR, and correlated to retinopathy severity.Citation55,Citation56 The choroid blood flow alterations, as assessed by the above indices, were significantly decreased in no DR groups compared to controls, confirming the hypothesis of choroid changes preceding the development of DR.
The OCTA technology has been recently applied in assessing choriocapillaris flow in eyes with DM.Citation57 Dai et al. have evaluated choriocapillaris flow deficit areas using SS-OCTA in patients with different stages of DR and healthy control subjects.Citation58 In their study, including 45 eyes of subjects with DR, they observed that the flow deficit areas in the choriocapillaris were 1.4 times larger in diabetic eyes than in control eyes, but no significant difference was observed in the parameters in comparing eyes with NPDR and PDR.Citation58 Similar results were shown by Zhang and colleagues, who observed reduced choriocapillaris flow density in eyes with DR compared to healthy controls.Citation59
In summary, the imaging of the choriocapillaris in eyes with DR, especially in the macular region, helps in understanding the pathological effects of the disease on choroidal circulation and consequently on the health of the overlying photoreceptors. The involvement of choriocapillaris in DR may be related to the elevated levels of VEGF in the local milieu. Choriocapillaris perfusion impairment is also observed in diabetic patients without clinically detectable DR.Citation29 Furthermore, choriocapillaris flow reduction correlates with the DR stage, with advanced stages featured by greater choriocapillaris hypoperfusion ().Citation60,Citation61 Thus far, widefield OCTA has been used to assess the retinal perfusion in patients with DM.Citation62–64 In the future, widefield OCTA can be effectively used to evaluate the choriocapillaris perfusion in eyes with DR.
Figure 2. Optical coherence tomography angiography (OCTA) of two patients with diabetic macular edema. The layers of the OCTA are labeled as follows: SCP – superficial retinal capillary plexus; DCP – deep retinal capillary plexus; OR – outer retina; CC – choriocapillaris. Both patients #1 (A) and #2 (B) show an increase in the foveal avascular zone area and perifoveal increase in the flow deficit areas in the retinal microvasculature. Notably, the CC slab in both the patients shows areas of flow deficit (white arrowheads) suggestive of impaired CC perfusion.
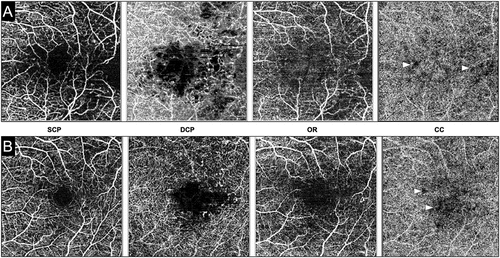
Flow Alterations after Therapies
There are very few studies that have evaluated the changes in choriocapillaris and choroidal circulation in patients with DR after therapies such as laser photocoagulation or anti-vascular endothelial growth factor (anti-VEGF) injections. Mendivil et al. published results on ophthalmic, central retinal, and short posterior ciliary arterial blood flow in diabetic eyes at 0.5, 1, and 2 years post-laser treatment with color Doppler.Citation44 Reported blood flow velocities in these vessels were significantly lower in treated eyes than in age-matched controls. Choroidal blood in the foveal region were significantly increased 1 month after panretinal photocoagulation (PRP). This increase in local blood flow was attributed to redistribution after laser coagulation or PRP-induced inflammation.Citation65 However, there is no published study analyzing the choriocapillaris flow after laser PRP or macular laser in eyes with DR.
A few studies have analyzed the effects of anti-VEGF treatment on choroidal blood flow. Okamoto and colleagues looked at the impact of ranibizumab on choroidal blood flow in treatment-naïve eyes with macular edema. They observed that the flow was significantly reduced compared to baseline within 1 month after intravitreal ranibizumab injection.Citation66 In 2019, Conti et al. showed no significant difference in choriocapillaris perfusion in diabetic eyes injected with periodic aflibercept compared to baseline during the 1-year follow-up.Citation67 Wang and colleagues also observed that prior history of anti-VEGF treatment was not associated with choroidal vascular density and volume changes.Citation55
In general, reports analyzing the choriocapillaris perfusion in eyes with DR (or in patients with DM but no DR) are scattered and do not suggest a direct relationship of the choriocapillaris flow with the disease pattern, severity, or treatment. Rather, there is a complex relationship between choriocapillaris blood flow and DR, and targeted studies are needed in the future to understand this association, if any.
AGE-RELATED MACULAR DEGENERATION
Pathology of Choriocapillaris Alteration with Age
With advancing age, there are several anatomical alterations that occur in the choroid and specifically, in the choriocapillaris. Recently, May and colleagues summarized the anatomical changes associated with aging in human choroid.Citation68 Similar findings (with variable degrees of thinning) were reported in the literature using in vivo OCT, and up to 57% reduction in choroidal thickness was observed in a post-mortem study.Citation11,Citation69,Citation70 Ramrattan and colleagues reported a decrease in choriocapillaris density and diameter in autopsy eyes from patients in the tenth decade of life compared to those in the first decade. A novel automated segmentation algorithm using SS-OCT developed by Zhou and colleagues found a significant inverse correlation between choroid thickness/volume and age consistent with previous results. Citation71 There are few reports that have segmented the choriocapillaris alone and provided data regarding the change in thickness of the choriocapillaris layer.
Choriocapillaris Alterations Associated with Age-related Macular Degeneration
AMD is the leading cause of blindness in developed countries with an estimated worldwide prevalence is 8.7%.Citation72 AMD is a multifactorial disease, and the exact pathogenesis is yet to be determined. It is traditionally classified into dry (from no drusen to geographic atrophy) and wet (neovascular, exudative) AMD forms. Among the proposed pathogenic mechanisms, the vascular theory outlined by Friedman suggests that scleral stiffening and altered choroid circulation trigger the RPE metabolic dysfunction, debris, and drusen accumulation. On examining dehydrated, mounted specimens, Friedman observed that senile drusen distribution was not random, and it was closely related to choriocapillaris associated with collecting venules. Another histopathologic study, with combined light and electron microscopical technique, showed the loss of capillaries in aged eyes and all investigated AMD stages (more profound choriocapillaris loss in later stages).Citation73 Thus, Biesemeier and colleagues concluded that choriocapillaris loss occurs with aging and precedes RPE and neuroretina degeneration.
While the role of the choroid in AMD development remains controversial, the alterations of choroidal blood flow in macular degeneration have been studied. Using PBF, certain studies have shown no significant differences in pulsatile flow between age-matched control and non-exudative AMD groups, but significantly lower flow in exudative AMD compared to control subjects.Citation74 However, Sandhu and colleagues measured PBF and found no significant difference in eyes with exudative AMD compared to fellow eyes with dry AMD. Thus, using PBF, it is not clear whether choroidal alterations take place in AMD or not. Since PBF is not considered to be a surrogate marker for choroidal or choriocapillaris flow, it is not preferred quantitative metric presently.
A few studies have utilized OCT and OCTA in studying the choroidal changes (and choriocapillaris density) in AMD.Citation24,Citation75 Several investigations have confirmed choroidal thinning in eyes with pseudodrusen, early and advanced AMD, and choriocapillaris loss in geographic atrophy eyes. Moreover, a negative correlation between subretinal deposits density and choriocapillaris density was also observed.Citation75,Citation76 In eyes with geographic atrophy, there is not only choriocapillaris dropout and impaired flow beneath the regions of photoreceptors and RPE loss but it extends beyond the margins of the atrophy in some cases.Citation29,Citation76 SS-OCT with VISTA algorithm added important details to the previous findings, specifying that the choriocapillaris flow changes as atrophic with geographic atrophy region and diminished beyond that region.Citation75,Citation77 In neovascular AMD, subfoveal choroidal thickness is reduced compared to age-matched controls.Citation24 Moult et al. studied exudative AMD with SS-OCT and found the association between choroidal neovascularization (CNV) lesions and regions of choriocapillaris alteration and suggested the CNV lesions themselves may form in these regions of reduced circulation.Citation78
In summary, the studies employing OCT and OCTA have shown more conclusively the reduced choriocapillaris perfusion in eyes with AMD, especially those with CNV compared to healthy control subjects. In addition, patients with pseudodrusen and early AMD may also have subclinical choriocapillaris changes. Similar to DR, further studies are needed to evaluate changes in choriocapillaris flow after various treatments including anti-VEGF therapy in AMD.
PACHYCHOROID SPECTRUM OF DISEASES
The term “pachychoroid” or “pachychoroid spectrum disease” was introduced in 2013 and is defined as an abnormal and permanent increase in choroid thickness, often with dilated choroidal vessels and other structural changes of the normal choroid architecture.Citation79,Citation80 The pachychoroid spectrum disease includes central serous chorioretinopathy (CSC), pachychoroid pigment epitheliopathy (PPE), pachychoroid neovasculopathy (PNV), and polypoidal choroid vasculopathy (PCV).Citation81 Dansingani and colleagues used SS-OCT with en face fragmentation to describe two significant findings of pachychoroid phenotype – pathologically dilated deep choroidal vessels that occupy the full choroid thickness and terminate abruptly, and the spatial correlation of these pachy-vessels to regions on increased choroidal thickness.
Abnormal Choroidal Flow in Pachychoroid Spectrum
While the underlying pathogenic mechanisms of pachychoroid diseases are yet to be clarified, it is speculated that the choroid changes in these cases may be intrinsic and etiologic. After careful examination of CSC patients and their relatives, Lehman and colleagues observed increased subfoveal choroid thickness in all subjects and suggested that pachychoroid could be an inherited condition.Citation82 The hallmark of pachychoroid spectrum is the presence of relative choriocapillaris ischemia along with a thickened choroid. Thus, there is a differential flow within the different choroidal layers with relative low perfusion of the inner choroid, i.e. choriocapillaris. Using OCTA, researchers from South Korea analyzed the choriocapillaris flow in eyes with pachychoroid, PPE, and control eyes.Citation83 The reported vascular density in the choriocapillaris was significantly lower in PPE eyes than in pachychoroid and control eyes, whereas the mean number of signal voids and mean total area of signal voids per eye were highest in PPE eyes. Additionally, 89% of the area of signal voids were located over pachy-vessels, with the strongest correlation in the PPE group. With these findings, Baek and colleagues concluded that the choriocapillaris flow is impaired in pachychoroid and worsens as epitheliopathy progresses and suggests the choriocapillaris flow impairment precedes RPE and outer retina changes in pachychoroid eyes.Citation83
Polypoidal Choroidal Vasculopathy (PCV)
PCV is characterized by aneurismal dilations or polypoid vascular lesions at the termination of a network of large choroid vessels (or polypoidal dilations and choroid branching vascular networks (BVN)) on ICGA and is associated with recurrent serous and hemorrhagic detachment of RPE and neuroretina.Citation84,Citation85 In the pre-OCT era, the ICGA was utilized to assess these aneurysm-like enlargements, branching networks of variable-sized vascular channels, choroidal filling delays, and choroid vascular hyperpermeability and was the only available tool for PCV diagnosis.Citation86,Citation87 Recently, OCTA was shown to be as effective as the ICGA method for PCV evaluation.Citation88,Citation89 Srour and colleagues observed hyper-flow lesions in choroid BVN using OCTA that topographically corresponds to BVN visualized by ICGA.Citation89 Cheung et al. used OCT to report marked subfoveal choroid thickening in PCV compared to age-matched controlled eyes and those with early AMD.Citation90 Alasil and Ferrara used another OCT modality, SS-OCT, and demonstrated choroidal vasculature abnormalities in all PCV eyes.Citation24,Citation85 However, there is limited information on choriocapillaris flow in patients with PCV in the literature.
Central Serous Chorioretinopathy (CSC)
CSC is a common retinal disease with a relatively high recurrence rate and is characterized by serous retinal detachment and RPE lesions.Citation91 Numerous FA and ICGA studies observed choroid blood vessel filling delays, hyper-permeability, and fluid leakage, suggesting that choriocapillaris dysfunction plays an important and likely etiologic role in CSC development.Citation80,Citation92 In fact, Kitaya et al. used FA and ICGA simultaneously in 36 patients with acute CSC and choroid flow measurement with laser Doppler flowmetry.Citation93 Besides early filling delays, focal leakage, and abnormal late-phase hyperfluorescence, the authors reported that the choroid blood flow in eyes with CSC was significantly lower than in fellow eyes, which may correlate with non-perfused choriocapillaris in angiography studies.Citation93 On OCT, the increased subfoveal choroid thickness was universal for CSC.Citation94 En face SS-OCT in eyes with chronic CSC showed diffuse and focal dilation on choroid vessels, corresponding to the changes on fundus autofluorescence images. Costanzo and colleagues reported OCTA findings with abnormal choroidal vessel patterns in CSC eyes and noticed ‘dark areas’ at the choriocapillaris that correspond flow void and suggest the focal flow reduction could cause this phenomenon. Several other authors have also used OCTA and reported similar findings in eyes with CSC (both affected and fellow eyes).Citation95–99
In 2018 and 2019, two separate groups quantified the choriocapillaris flow using OCTA in fellow eyes of patients with CSC.Citation95,Citation96 The fellow eyes are taken for analysis to avoid OCT signal artifacts, especially if subretinal fluid is present. Both studies demonstrated a significantly larger mean flow void area in unaffected eyes of CSC patients. Yun et al. specified the prevalence of flow void areas in CSC and control groups (59.6% versus 29.8%). They noted that the choriocapillaris’ flow void area in the CSC group’s eyes was significantly higher over the vascular bed than over the stromal bed.Citation98 This finding assumes that choroid structure and choriocapillaris flow distribution in CSC patients are asymmetric. Lastly, the location of epitheliopathy corresponded to the flow void area, suggesting that RPE abnormalities develop within the area of choriocapillaris hypoperfusion. In summary, findings from all studies indicate that the choriocapillaris flow reduction in CSC eyes and that focal flow impairment results in focal RPE ischemia and dysfunction.
CHOROIDITIS
White Dot Syndromes
Multimodal imaging has allowed a better understanding of the morphology and pathophysiology of the so-called white dot syndromes (WDS). WDS represent a heterogeneous group of inflammatory disorders primarily affecting the outer retina, choriocapillaris, and choroid, not secondary to infectious processes or other systemic diseases. This section analyzes the WDS, including multiple evanescent white dot syndrome (MEWDS), punctate inner choroidopathy (PIC), multifocal choroiditis with panuveitis (MCP), acute posterior multifocal placoid pigment epitheliopathy (APMPPE), serpiginous choroiditis, and Birdshot retinochoroiditis (BRC), from an imaging-based assessment perspective, and elucidates the origin of this spectrum of diseases, determining the precise localization of lesions and different levels of tissue involvement.
Multiple Evanescent White Dot Syndrome
MEWDS is an acute, idiopathic, unilateral disorder more commonly affecting young, healthy females complaining of sudden visual loss, photopsias, dyschromatopsia, and scotomas. Clinically, the disease is characterized by yellowish-white deep retinal lesions extending to the mid-peripheral retina in the macular area. The disease is typically self-resolving within weeks, with no requirement for treatment in most cases.
MEWDS was traditionally labelled as primary chorioretinitis or choriocapillaritis.Citation100–102 However, authors have debated whether the disorder represents a choroidal vasculature disease with late outer retinal involvement or a primarily outer retinal disorder. Multimodal imaging, including the most recent en face OCT and OCTA, contributed to new insights into the pathogenesis of the disease, providing a better understanding of which ocular structures are actually involved in the inflammatory process.Citation103–106 On ICGA, MEWDS is typically characterized by late hypofluorescent lesions. Such angiographic findings led to the hypothesis of the choroidal origin of the lesions resulting from choroidal hypoperfusion and corresponding to areas of the absence of flow in the choriocapillaris due to space-occupying inflammatory lesions.Citation101,Citation107 Other evidence suggested choroidal involvement in MEWDS. Different authors showed an increased choroidal thickness during the active phase of the disease, decreasing back to normal during the recovery phase.Citation108,Citation109 Fiori et al. described hyperreflective dots at the level of the inner choroid, including Sattler’s layer and choriocapillaris, disappearing during the follow-up.Citation109 Hashimoto et al analyzed choroidal circulation hemodynamics utilizing laser speckle flowgraphy and found a reduction in choroidal blood flow during active disease.Citation110
However, although there is evidence suggesting the involvement of choroid and precisely choriocapillaris in MEWDS, recent studies have provided new insights into the pathogenesis of the disease, demonstrating a primary involvement of the outer retinal layers with no choroidal disease. Pichi et al. compared en face OCT and OCTA with conventional imaging in 36 patients diagnosed with MEWDS.Citation103 OCTA demonstrated sparing of the choriocapillaris in 100% of the patients, and this is consistent with other reports describing flow preservation of the choriocapillaris in patients diagnosed with MEWDS.Citation103–106 In all patients, en face OCT did not detect any abnormalities or dilated vasculature at the level of the choriocapillaris.Citation103 However, large hyporeflective en face spots were found at the level of the ellipsoid zone. These hyporeflective spots corresponded to the lesions detected on fundus examinations. The corresponding spectral-domain (SD)-OCT conducted through the spots identified disruption of the ellipsoid zone associated with hypertrophy of the underlying RPE. The lesions at the level of the ellipsoid zone co-localized with the hypofluorescent spots detected on ICGA and with the hyper-autofluorescence seen on FAF and did not correspond to any flow void on OCTA. The authors thus hypothesized that photoreceptors could be the primary site of the inflammation and that the disruption and attenuation of the ellipsoid layer seen on SD-OCT unmask the normal underlying autofluorescence of the RPE resulting in hyper-autofluorescent lesions. The hypofluorescent lesions seen on ICGA cannot be explained by choroidal hypoperfusion, given the absence of areas of flow void at the level of choriocapillaris on OCTA. However, considering the correspondence with the areas of ellipsoid loss, the angiographic findings might be due to a disruption of indocyanine green uptake by the RPE, typically causing the physiological background hyperfluorescence.
Considering that, the previously reported findings indicating choroidal involvement in MEWDS might suggest a short-lasting inflammatory involvement of choriocapillaris and choroid during the acute phase of MEWDS and explain the absence of permanent alterations that are instead described in primary choriocapillaritis. In conclusion, recent advances in multimodal imaging suggest no choriocapillaris involvement in MEWDS. The disease primarily affects the outer retina, namely the photoreceptors and the ellipsoid zone. Associated secondary RPE abnormalities can explain the hypofluorescent spots on ICGA and the normal perfusion of the choriocapillaris on OCTA.
Acute Posterior Multifocal Placoid Pigment Epitheliopathy (APMPPE)
APMPPE is an acute, commonly bilateral, inflammatory disorder affecting young, healthy adults, presenting with bilateral visual loss, photopsias, and paracentral scotomas. Clinically, the disease manifests as multifocal yellow creamy placoid lesions, more commonly involving the posterior pole, which progressively fade over the weeks, potentially leading to hyperpigmentation and chorioretinal atrophy. APMPPE is a choriocapillaritis, where the damage occurs primarily at the level of the choriocapillaris and inner choroid, leading to secondary outer retinal changes. Since the initial description of the disease in 1968, hypotheses related to choroidal involvement and ischemia of choriocapillaris as mechanisms involved in the pathogenesis of the disease were formulated, subsequently supported by angiographic findings on ICGA.Citation111–117 Later, Spade et al. hypothesized that the simultaneous involvement of multiple patches of RPE appearing as hyper-autofluorescent on FAF could only be related to underlying choroidal vasculature involvement.Citation118 Other authors reported clinically detectable lesions with no corresponding changes in FAF to support primary choroidal involvement.Citation94 The advent of multimodal technology, including en face OCT and OCTA, provided a better understanding of the structures involved, confirming the role of choroidal microvascular blood flow in the acute phases of the disease.Citation119–121
The multifocal placoid lesions characterizing APMPPE appear hypofluorescent in the early phases of FA with late staining and hypofluorescent across all frames of ICGA. The lesions correspond to outer retinal and ellipsoid disruption on SD-OCT, with en face OCT conducted at the level of the outer retina showing hyper-reflectivity (). The outer retinal changes correspond to multifocal areas of reduced flow at the inner choroid-choriocapillaris level on OCTA, which correlates with the hypofluorescent areas on FA and ICGA.Citation119,Citation120 These findings support that the hypofluorescent lesions detected on ICGA are caused by ischemia of the choriocapillaris rather than overlying RPE abnormalities. The areas of choriocapillaris flow reduction on OCTA are more significant than the corresponding areas of outer retina involvement on en face OCT, confirming the primary involvement of inner choroid with secondary outer retina changes and RPE abnormalities. Burke et al. utilized multimodal imaging to study the evolution of chorioretinal lesions in APMPPE.Citation121 The authors proposed four stages of the disease, with the first one characterized by choriocapillaris flow reduction as detected on OCTA, ICGA, and early FA.
Figure 3. A patient with bilateral choroiditis lesions of acute posterior multifocal placoid pigment epitheliopathy (APMPPE). The right eye (A) shows indocyanine green angiography (ICGA) with hypofluorescent areas suggestive of active choroiditis and perfusion defects. The corresponding optical coherence tomography (OCT) (B) shows an increase in the height of the choriocapillaris slab (white asterisk) with loss of the normal dotted pattern, suggestive of choriocapillaris ischemia. The left eye (C) ICGA image shows a similar pattern of hypofluorescence due to perfusion loss. The OCT (D) of the left eye also shows changes in the choriocapillaris layer with thickening and loss of the normal dotted pattern (white asterisk).
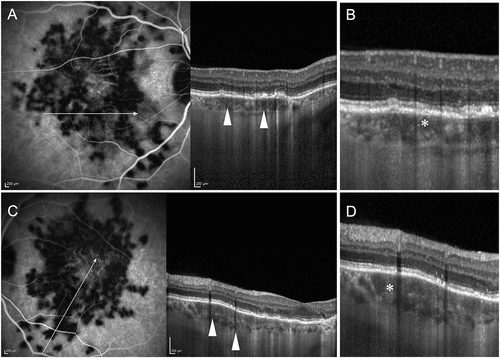
The disease can then evolve in a complete resolution of choriocapillaris hypoperfusion without any abnormalities on FAF or OCT or move to the second stage, characterized by a persistent flow void area on OCTA and classic angiographic findings. At this stage, outer retina changes, including inner segment ellipsoid zone and RPE, become visible on SD-OCT together with patches of hypo-autofluorescence on FAF. Persistent choriocapillaris flow reduction results in thinning and disrupting the outer retina, with subsequent central hyper-autofluorescence on FAF. A complete resolution of choriocapillaris flow reduction is detected on OCTA during the fourth phase. However, persistent outer retinal thinning and disruption of the RPE are detected on SD-OCT together with diffuse hypo-autofluorescence of the lesions on FAF.
The findings above thus suggest that APMPPE is a primary choriocapillaritis, where the areas of flow reduction at the level of choriocapillaritis correlated closely with both ischemic lesions seen on FA and ICGA and outer retinal disruption on SD-OCT. Furthermore, despite the resolution of choriocapillaris flow reduction, the disease can result in secondary permanent outer retina changes and RPE damages, indicating that hypoperfusion of the choriocapillaris is the primary pathological event causing the disease.
Multifocal Choroiditis with Panuveitis and Punctate Inner Choroidopathy
MFC and PIC are inflammatory disorders that can be considered variants of the same spectrum due to overlapping phenotypic features.Citation120,Citation122 Both diseases commonly affect young myopic females, complaining of blurriness, paracentral scotoma, and photopsias. Bilateral, small, discrete, well-defined, yellowish chorioretinal lesions are clinically detected. In MFC, lesions can involve both the posterior pole and periphery, associated with anterior uveitis and vitreous inflammation. In contrast, PIC is characterized by posterior pole lesions with no signs of intraocular inflammation. Both diseases are characterized by multiple recurrences, with visual impairment possibly occurring due to lesions involving the fovea or the development of choroidal neovascularization. Diagnosis is based on clinical features of active lesions and OCT findings, including elevation of the RPE with sub-RPE hyper-reflectivity and surrounding outer retina disruption ().Citation122–127 Active lesions show early hyperfluorescence with late staining on FA and hypofluorescence across all phases on ICGA. FAF shows hypo-autofluorescent spots with hyper-autofluorescent margins.Citation122,Citation123
Figure 4. A patient with punctate inner choroidopathy (PIC). The autofluorescence image is shown in A. The lesions appear hypo-autofluorescent. The optical coherence tomography (OCT) line scan passing through two lesions of PIC shows the characteristic dome-shaped elevation of the retinal pigment epithelium and fuzziness of the outer retinal layers (white arrowheads). The choriocapillaris layer underneath the lesions has increased height and loss of the normal dotted pattern (white arrow), suggestive of choriocapillaris ischemia.
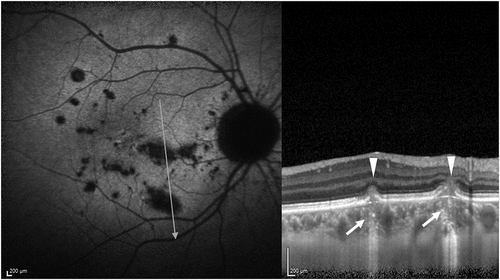
The advent of OCTA allowed us to better define the role of choriocapillaris in the pathogenesis of such diseases. Thompson et al. recently described choriocapillaris microvasculature changes in five patients diagnosed with PIC, following them with serial images before and during the treatment.Citation128 OCTA showed well-demarcated areas of flow void at the level of the choriocapillaris, co-localizing with areas of RPE elevations on OCT and hypofluorescent lesions on ICGA, corresponding to active inflammatory lesions.Citation128–130 Comparative analysis of choriocapillaris hypoperfusion before and after treatment showed a lower mean flow void area after treatment. Active lesions in the late stage of the disease result in punched-out scars with pigmented borders. Areas of flow void are described on OCTA at the level of the choriocapillaris correlating with such lesions.Citation131
Multimodal imaging findings suggest the role of choriocapillaris in the pathogenesis of both diseases. Area of choriocapillaris hypoperfusion correlating with active lesions can indicate that changes in the outer retina develop secondary to choroidal damage. However, secondary involvement of choriocapillaris following the inflammatory process of the overlying retina cannot be excluded.
Serpiginous Choroiditis
Serpiginous choroiditis is a bilateral, chronic, progressive, inflammatory disease affecting healthy male individuals slightly more than women. Patients usually complain of decreased vision, paracentral scotomas, photopsias, or metamorphopsias. The disease classically manifests with a serpiginous or geographic pattern, characterized by grey/yellow subretinal lesions centrifugally spreading from the peripapillary region and subsequently evolving into chorioretinal scars.
Serpiginous choroiditis is considered a choriocapillaritis, with choriocapillaris hypoperfusion being the most likely pathological event, leading to the secondary outer retina and RPE changes. Early hypofluorescence of active lesions on FA and hypofluorescence across all phases on ICGA already suggested the role of choroidal involvement and reduced choroidal perfusion in the pathogenesis of the disease.Citation132,Citation133 Subsequently, multimodal imaging provided new insight into the mechanisms underlying the disease, supporting the choriocapillaris primary involvement.
Several authors described active serpiginous lesions characterized by areas of flow void detected by OCTA at the level of the choriocapillaris, co-localizing with hypofluorescent lesions on ICGA.Citation134–136 Desai et al. reported pre- and post-treatment OCTA findings in six eyes of three patients diagnosed with serpiginous choroiditis.Citation136 The absence of choriocapillaris was detected in both active and inactive stages of the lesions, with the partial reappearance of the choriocapillaris flow signal following steroid treatment. Pakzad-Vaezi et al. showed that during the active stage of the disease, the choriocapillaris flow void detected on OCTA was more significant than the area of hyper-reflectivity detected on en face OCT at the level of the outer retina than the area corresponding to autofluorescence changes on FAF.40 In addition, choriocapillaris hypoperfusion on OCTA does not always correspond to abnormal autofluorescence on FAF and, thus RPE injury.Citation137 Following these findings supporting the hypothesis of choriocapillaris as the primary site of pathology and based on multimodal imaging features, the authors proposed a classification of the disease into three stages. The first grade is characterized by choriocapillaris flow void seen on OCTA with no outer retina and RPE changes detected on en face OCT and FAF. Lesions can then progress to the second grade with involvement of the overlying outer retina as seen on en face OCT. Both the stages are to some extent reversible and can resolve with proper treatment without chorioretinal scarring. The following stage corresponds to RPE injury and changes in autofluorescence seen on FAF with RPE dysfunction and clinically detectable atrophy.
Although multimodal imaging supports the hypothesis of serpiginous choroiditis as a primary choriocapillaritis, where the inflammatory damage primarily occurs at the level of the inner choroid and secondarily affects the overlying RPE and outer retina, there is still debate regarding an inflammatory process primarily occurring at the level of the outer retina and RPE and secondarily involving the choriocapillaris. Therefore, the exact pathogenic mechanisms underlying serpiginous choroiditis have yet to be fully explored.
Birdshot Retinochoroiditis (BRC)
BRC is a bilateral, chronic, progressive inflammatory disorder, more commonly affecting middle-aged Caucasian females, complaining of blurriness, floaters, photopsias, and nyctalopia. BRC is characterized by independent retinal inflammatory involvement and retinal vasculitis, often complicated by macular edema and stromal choroiditis, manifesting with subtle, creamy, round lesions, typically located around the optic disc radiating out to the equator.Citation138,Citation139
In BRC, the inflammatory damage primarily occurs at the level of choroidal stroma and secondarily affects the overlying choriocapillaris and RPE. ICGA is an essential tool for early diagnosis of the disease, providing information on inflammatory choroidal involvement manifesting as hypofluorescent lesions.Citation140,Citation141 More recently, OCTA helped determine the precise localization of inflammatory lesions in the choroid, supporting the primary stromal involvement.Citation142 Pepple et al. demonstrated that in the acute stage of the disease, the areas of flow void on OCTA co-localize with hypofluorescent lesions on ICGA and are located adjacent to large vessels in the Haller layer, with preserved choriocapillaris flow.Citation142 In the late stage of the disease characterized by chorioretinal atrophy, full-thickness flow void areas were detected in the choroid at the Haller layer, Sattler layer, and choriocapillaris. OCTA findings were reversible in the acute stage of disease and regressed with treatment, suggesting that, without proper therapeutic intervention, inflammatory damage can extend from the Haller layer to the inner choroid, eventually resulting in RPE and outer retina dysfunction. En face OCTA findings thus support that deep choroid is the primary target of inflammation and that choriocapillaris can secondarily be involved.
Tubercular Choroiditis
Tubercular choroiditis is the most common manifestation of ocular tuberculosis, including tubercular serpiginous-like choroiditis (TB-SLC) and tuberculoma.Citation143 The advent of OCTA allowed a better assessment of retinochoroidal vascular changes, providing new insight into the pathogenesis of the disease.
Tubercular Serpiginous-like Choroiditis
In TB-SLC, the inflammatory damage seems to primarily occur at the level of choriocapillaris. The disease manifests as multifocal yellowish fuzzy subretinal lesions, characterized by slightly raised active edges showing wave-like progression resembling the serpiginous pattern.Citation143–145 Different authors described areas of flow void detected on OCTA at the level of the choriocapillaris corresponding to active serpiginous-like lesions, supporting the inflammatory vascular occlusive nature of the disease primarily involving the inner choroid. Mandali et al. analyzed 11 eyes with active TB-SLC showing choriocapillaris hypoperfusion.Citation134 The affected areas on OCTA correlated consistently with hypofluorescent spots on ICGA in all eyes. However, reduced signal transmission due to the outer retina and RPE changes was noted when the areas of flow void were correlated with en face OCTA and B-scan OCT, suggesting a shadowing effect from the hyper-reflective overlying retina.Citation146 Nevertheless, Wang et al. did not find any decreased signal transmission when correlating OCTA flow void images with hyper-reflective outer retina changes on SS-OCT.Citation147 In addition, healed atrophic lesions showed prominent medium-large choroid vessels on OCTA, supporting the hypothesis that vessels from Sattler’s layer become visible due to choriocapillaris atrophy and the areas of flow void represents an actual loss of choriocapillaris blood flow and not shadowing effect.
The technique of CVI has also been used in assessing the choroidal flow in eyes with TB-SLC. Agarwal et al. showed that during the active disease, eyes with TB-SLC show a reduced CVI compared to healthy controls suggesting decreased choriocapillaris and choroidal perfusion, and as the lesions heal, the CVI is further reduced due to choriocapillaris atrophy.Citation148 Several authors correlated OCTA blood flow abnormalities with the four stages characterizing TB-SLC on FAF previously described by Gupta et al.Citation149 Areas of choriocapillaris flow void with possible signal attenuation and no view of large choroidal vessels were detected on OCTA correlating with stage 1 lesions, characterized by diffuse hyper-autofluorescence on FAF. Progressive restoration of choriocapillaris blood flow characterized by reduction of the size of the hypoperfusion area was seen during the recovery phases, correlating with the transition to diffuse hypo-autofluorescence of the lesions on FAF.Citation150 Agarwal et al. described OCTA findings during paradoxical worsening in TB-SLC.Citation150 Progression of choroidal lesions manifested as increasing areas of choriocapillaris hypoperfusion on OCTA, correlating with the direction of the leading edge of the advancing lesion. The multifocal patches of choriocapillaris hypoperfusion tended to coalesce as clinically detected, and the areas of flow void on OCTA correlated with hyper-autofluorescence changes on FAF reflecting RPE injury. As the lesions began to heal, partial recovery of choriocapillaris blood flow together with choriocapillaris atrophy resulting in medium-large choroidal vessels’ visualization was detected on OCTA.Citation146
Tuberculoma
Tuberculoma appears as a yellowish subretinal lesion with indistinct borders, corresponding to a round lesion located in the choroidal stroma.Citation143,Citation151–153 Involvement of choriocapillaris depends on the size of the lesion. Full-thickness granulomas are characterized by early and late hypofluorescence on ICGA corresponding to choriocapillaris flow void on OCTA.Citation154 By contrast, partial-thickness granulomas not involving the inner choroid manifest as early hypofluorescent and late iso-fluorescent lesions on ICGA with no microvasculature changes detected on OCTA at the level of the choriocapillaris. In conclusion, multimodal imaging findings confirm choriocapillaris involvement in tubercular choroiditis, supporting the hypothesis of primary pathologic changes occurring at the level of the inner choroid in TB-SLC and choriocapillaris involvement occurring secondary to stromal disease in tuberculoma.
Toxoplasma Retinochoroiditis
Ocular toxoplasmosis manifests as a focal necrotizing retinochoroiditis, characterized by retinal and choroidal tissue destruction. SD-OCT of active lesions typically shows disorganization and hyper-reflectivity of the neurosensory retina, together with thickening and hypo-reflectivity of the choroid.Citation155 Active lesions appear as early hypofluorescent and late hyperfluorescent on FA and hypofluorescent across all phases on ICGA. Toxoplasma lesions typically evolve in chorioretinal scars with thinning of both retina and underlying choroid detected on SD-OCT.
Few studies analyzed choriocapillaris involvement and OCTA findings in toxoplasma retinochoroiditis. OCTA usually shows a localized hypointense signal in both superficial and deep capillary plexus and choriocapillaris, corresponding to the masking effect of active focal lesion.Citation131,Citation156 As the lesion starts to heal, the dark area on OCTA regresses in size proportionally to the regression of retinochoroiditis clinically detectable. Once healed, complete and permanent loss of choriocapillaris is seen at the site of chorioretinal scarring due to atrophy, and Sattler’s layer together with large choroidal vessels are detected on OCTA at the level of choriocapillaris. Permanent destruction of the choriocapillaris at the site of focal toxoplasma lesion is thus confirmed by OCTA findings. On OCTA, eyes with toxoplasmosis may show a larger extent of choroid involvement than fundus photography, FA, and ICGA.Citation157
In summary, analysis of the choriocapillaris in ocular toxoplasmosis is largely limited due to the intraretinal pathology, which causes back-shadowing and poor visualization of the choriocapillaris. In the healed stage, OCTA can be used to assess the extent of choriocapillaris ischemia in eyes with toxoplasmosis.
Vogt–Koyanagi–Harada Disease and Sympathetic Ophthalmia
Stromal choroiditis such as Vogt–Koyanagi–Harada (VKH) disease and sympathetic ophthalmia is characterized by bilateral granulomatous inflammation involving the deep choroid. The diseases are characterized by stromal choroidal granulomas with bilateral multifocal exudative retinal detachments.Citation158–164 The phenotype presentation for both the conditions is similar, with the only difference being a history of trauma in patients with sympathetic ophthalmia.Citation165–169 Trauma leads to a trigger of antigenic reaction in the fellow eye (‘sympathizing eye’) as well as the involved eye. In the case of VKH disease, the patients demonstrate a similar immunological change resulting in severe inflammation, leading to permanent vision loss due to RPE and photoreceptor damage if not treated adequately.
Assessment of the choroid is of paramount importance in eyes with VKH and sympathetic ophthalmia. Conventionally, the assessment of the choroid is performed using ICGA.Citation170–175 ICGA provides a host of information, including the detection of choroidal granulomas (full-thickness versus partial thickness) and other chorioretinal abnormalities such as fuzziness of the vasculature. With the advent of EDI-OCT, it is now possible to detect choroidal granulomas non-invasively.Citation154,Citation176–180 EDI-OCT can detect granulomas and help in monitoring their reduction with therapy. When corticosteroid and immunosuppressive therapies are initiated, the granulomas reduce rapidly, first in the anteroposterior direction and then laterally. In other words, the granulomas first become partial thickness of the choroid (from full-thickness) and then reduce in size laterally. EDI-OCT may also give insights into the etiology of the condition, with lobulated granulomas likely to be tubercular and rounded lesions likely to be sarcoid in nature.Citation179 Serial quantification of the granuloma on OCT corresponds well with the ICGA and can be used to monitor these patients longitudinally. Such measurements can be performed manually using the inbuilt measurement tools on OCT devices.Citation179
Aggarwal et al. demonstrated the utility of OCTA in serial imaging of the choroid in VKH disease ().Citation181 The authors showed that in VKH disease, OCTA reveals flow deficit areas in the choriocapillaris directly above the choroidal granulomas. These flow deficit areas were judged as true flow deficit areas since they corresponded well with the hypofluorescence on ICGA imaging.Citation181 On the contrary, in other conditions with bilateral serous retinal detachments such as CSC, the flow deficit areas on OCTA were false and attributed to abnormalities such as pigment epithelial detachments.Citation182 In patients with VKH disease, the flow deficit areas tend to decrease when the patient is initiated on systemic anti-inflammatory therapy. Serial imaging revealed a progressive reduction in the flow deficit areas with a corresponding decrease in the subretinal fluid on OCT and improvement in visual acuity on objective assessment. The authors also showed that in certain patients in whom the initial immunosuppressive therapy was tapered quickly, the disease recurrence could be detected earlier on OCTA, with an increase in flow deficit areas in the choriocapillaris slab developing before the subretinal fluid accumulation.Citation181 Thus, OCTA may play an invaluable role in managing VKH disease non-invasively. It also provides quantitative measurements of the choriocapillaris over time in these patients.
Figure 5. Indocyanine green angiography (ICGA) (A) and optical coherence tomography angiography (OCTA) (B) of a patient with active Vogt–Koyanagi–Harada’s (VKH) disease. The ICGA shows areas of hypofluorescence suggestive of choroidal granulomas characteristic of VKH disease. The corresponding OCTA of the choriocapillaris layer shows hypo-reflective areas that exactly co-localize with the hypofluorescent areas on ICGA, suggestive of true flow deficit areas in the choriocapillaris slab.
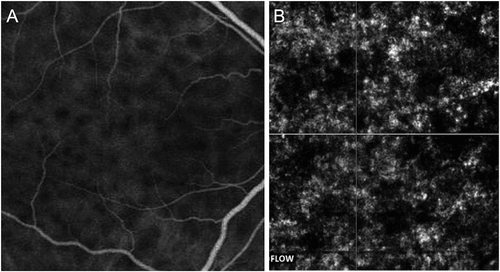
Recently, widefield OCTA has been applied to evaluate the choroid and the choriocapillaris in VKH disease. Vingopoulos et al. in a report of a single case demonstrated reduced choriocapillaris perfusion in a patient with acute VKH disease on SS-OCTA.Citation183 In a study of 133 eyes, Qian et al. observed flow void areas in 98.1% of the eyes with active VKH and 61.7% of the eyes with inactive VKH on 12 mm × 12 mm OCTA.Citation184 Similarly, Ye et al. performed 15 × 9 mm widefield OCTA (macular and peripapillary regions) in 50 eyes with VKH disease.Citation185 Compared to healthy control eyes, the authors observed significantly reduced choriocapillaris perfusion and flow void areas in the choriocapillaris. These changes were reversed in the convalescent phase when the disease healed.
In sympathetic ophthalmia, OCTA imaging has been applied less commonly. Patients with sympathetic ophthalmia generally present with severe inflammation associated with media haze due to dense vitritis and a history of trauma/surgery. Thus, these eyes may not be amenable to good-quality OCTA imaging. A limited number of studies have assessed the choroidal blood flow in patients with sympathetic ophthalmia and have shown changes similar to VKH disease.Citation186
Thus, both VKH and SO show diffuse thickening of the choroid and presence of stromal choroidal granulomas. Therefore, this results in compromised choriocapillaris perfusion and ischemia, which can be reversed once the stromal granulomas heal and resolve. The changes on OCTA co-localize well with ICGA imaging, which can also reveal choriocapillaris ischemia in addition to the stromal granulomas.
CAVEATS IN CHOROIDAL IMAGING
One must be consider that OCT scans obtained for the choroidal analysis are often two-dimensional, unless volumetric scans are obtained.Citation187 Without volumetric scans, there will be significant limitations in the capability of assessing the choriocapillaris/choroidal flow in the eye. In addition, the OCT does not have any demarcation of the choriocapillaris from the deeper layers of the choroid, unlike the retinal layers which have easily identifiable differentiation. Therefore, one must be careful in analyzing the choriocapillaris flow when studying OCT images alone.
Modalities such as OCTA can be employed to obtain repeatable choroidal blood flow measurements. These can be useful in monitoring the disease activity and progression. Technological advances such as SS-OCTA help in imaging the choroidal vasculature with higher accuracy. To date, there are no head-to-head studies comparing SD-OCTA with SS-OCTA in uveitis. SS-OCTA can adequately image the choriocapillaris, provide useful information related to flow deficit areas in choroiditis, and identify choroidal neovascular membranes (both type 1 and type 2), which may often not be detectable clinically, or on FA or ICGA.Citation188–192 However, one must be careful in analyzing the choroidal vasculature on SS-OCTA as there may be several fallacies in the assessment.
The most important caveat in assessing the choriocapillaris on OCTA is understanding the irregularity of this layer, especially in eyes with posterior uveitis in the presence of choroiditis lesions. Lumpy-bumpy nature of the inner choroid may make the segmentation slabs inaccurate, resulting in false assessments of the choriocapillaris flow deficit areas ().Citation193–197 These irregular segmentation slabs can result in fallacious quantitative measurements of the choroid. Parameters such as CVI and other automated measurements may be inaccurate. When applied in quantitative studies, without correcting the segmentation slabs, the analysis may be inaccurate and non-reproducible. There are several ways of minimizing the errors due to incorrect segmentation. One way is to use a standardized offset of the segmentation slab beneath the Bruch’s membrane.Citation195,Citation198–200 However, this may not completely control for the errors.Citation201 Manual segmentation of each line scan may be necessary to correct these errors. However, this is a tedious and time-consuming process.
Figure 6. Optical coherence tomography (OCT) scans of different patients with posterior uveitis. The OCT line scan in panel A shows a patient with multifocal choroiditis with an irregular choriocapillaris slab demonstrated by white parallel lines. The OCT scans in panels B and C show two patients with differences in the reflectivity of the retinal pigment epithelium (RPE) and the outer retina. The scan (B) shows relative atrophy and thinning of the RPE and consequent hyper-reflective signal transmission masking the choriocapillaris layer (white arrowheads). The scan (C) shows hyper-reflectivity of the RPE and the outer retina resulting in back shadowing and poor visibility of the choriocapillaris layer (white arrowheads).
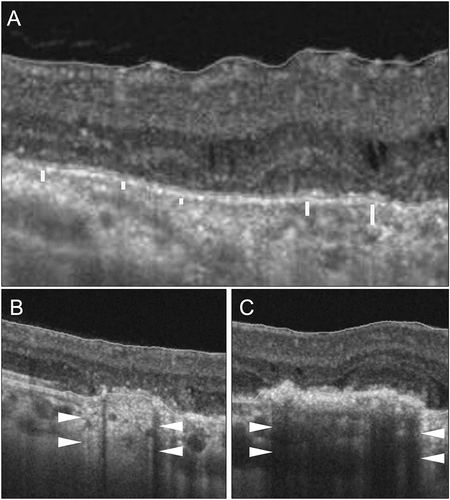
A significant challenge in eyes with posterior uveitis (and other conditions such as drusen in AMD) arises due to the irregularity (both structural and optical) of the RPE and the outer retina.Citation152,Citation202–204 These layers may be thickened or bumpy, leading to abnormal hyper-reflectivity on structural OCT. This may result in back shadowing and poor assessment of the underlying choriocapillaris (). On the contrary, atrophy or thinning of the RPE and the outer retina may increase reflectance. The underlying layers may incorrectly appear to be high-flow, and some normal vessels may be misdiagnosed as CNV.Citation205 It is important to consider the corresponding OCT B-scan in such eyes to detect flow signals, which may be absent if there is no CNV.
Stromal choroidal lesions such as tubercular or sarcoid choroidal granulomas may not be readily imaged using OCTA.Citation144,Citation151,Citation152,Citation203 Granulomas of VKH disease and sympathetic ophthalmia may not be assessed using OCTA imaging. Since the granulomas are deep in the choroid, even SS-OCTA cannot capture them adequately, and they only result in flow deficit areas of the overlying choriocapillaris. These flow deficit areas result due to compression from the mass effect of the granuloma. Thus, these lesions need to be assessed using SS-OCT or EDI-OCT (apart from OCTA) and other techniques such as ICGA.
CONCLUSIONS
The visualization of the choriocapillaris using imaging tools such as OCT and OCTA has dramatically improved the quantification of this layer in health and disease. Using OCT, the choriocapillaris can be reasonably delineated from the stromal choroid and quantified using either manual or automated methods. Since there is no anatomical distinction between the choriocapillaris and the stromal choroid, each quantification technique has its inherent limitations. However, the use of predefined segmentation slabs on OCT and OCTA help in standardizing the imaging of the choriocapillaris and obtain repeatable measurements in various conditions such as DR, AMD, pachychoroid spectrum, and ocular inflammations. Additionally, Doppler imaging and other methods to assess the choroidal blood flow have unearthed the relevance of imaging and quantifying the choriocapillaris and establishing its role in the pathogenesis of various retinochoroidal diseases. Understanding choriocapillaris blood flow has numerous implications such as redefining disease pathogenesis, better understanding of outer retinal and RPE structure/function, treatment outcomes and endpoint analysis, as well as employing novel treatment strategies to minimize choriocapillaris ischemia. As tremendous technological advancements take place (such as wide-field and ultra-wide field imaging), it is likely that the ease and accuracy of imaging and quantifying the choriocapillaris will improve. The field of imaging of the choriocapillaris has several areas options for research, and many advances are likely in the future.
METHOD OF LITERATURE SEARCH
A comprehensive search of medical literature was performed through the Medline/PubMed database (https://www.ncbi.nlm.nih.gov/pubmed) search terms: choriocapillaris, choroid, quantification, biomarkers, diabetic retinopathy, age-related macular degeneration, choroidal blood flow, mean blur rate, flow deficit, optical coherence tomography, optical coherence tomography angiography, fluorescein angiography, indocyanine green angiography, OCTA, Doppler imaging, uveitis, choroiditis, white dot syndrome, tubercular serpiginous-like choroiditis, choroidal granuloma, pachychoroid, toxoplasmosis, central serous chorioretinopathy, multifocal choroiditis, choroidal neovascularization, choroidal thickness, choroidal vascularity index, choroidal vascular density, and choroidal blood supply. The search was performed in August 2021, and publications from 1950 were selected. Only selected articles before 1990 were used for historical perspectives. The search terms were used either independently or combined with choriocapillaris/choroid. Manuscripts that focused on imaging of the choriocapillaris were selected for the review. Publications in non-English language were excluded from the search.
Disclosure statement
No potential conflict of interest was reported by the author(s).
Additional information
Funding
References
- Zouache MA, Eames I, Klettner CA, et al. Form, shape and function: segmented blood flow in the choriocapillaris. Sci Rep. 2016;6(1):6. doi:10.1038/srep35754.
- Nickla DL, Wallman J. The multifunctional choroid. Prog Retin Eye Res. 2010;29(2):144–168. doi:10.1016/j.preteyeres.2009.12.002.
- Garron LK. The ultrastructure of the retinal pigment epithelium with observations on the choriocapillaris and bruch’s membrane. Trans Am Ophthalmol Soc. 1963;61:61.
- Goldor H, Gay AJ, Gay AJ. Chorioretinal vascular occlusions with latex microspheres (A long-term study). II. Invest Ophthalmol. 1967;6:6.
- Gay AJ, Goldor H, Smith M. Chorioretinal vascular occlusions with latex spheres. Invest Ophthalmol. 1964;3:647–56.
- Lejoyeux R, Benillouche J, Ong J, et al. Choriocapillaris: fundamentals and advancements. Prog Retin Eye Res. 2022;87. doi:10.1016/j.preteyeres.2021.100997.
- Torczynski E, Tso MOM. The architecture of the choriocapillaris at the posterior pole. Am J Ophthalmol. 1976;81:428–440. doi:10.1016/0002-9394(76)90298-1.
- Uji A, Balasubramanian S, Lei J, Baghdasaryan E, Al-Sheikh M, Sadda SR. Choriocapillaris imaging using multiple en face optical coherence tomography angiography image averaging. JAMA Ophthalmol. 2017;135(11):1197. doi:10.1001/jamaophthalmol.2017.3904.
- Stanga PE, Lim JI, Hamilton P. Indocyanine green angiography in chorioretinal diseases: indications and interpretation. Ophthalmology. 2003;110(1):15–21. doi:10.1016/s0161-6420(02)01563-4.
- Meira J, Marques ML, Falcão-Reis F, Rebelo Gomes E, Carneiro A. Immediate reactions to fluorescein and indocyanine green in retinal angiography: review of literature and proposal for patient’s evaluation. Clin Ophthalmol. 2020;14:171–178. doi:10.2147/opth.s234858.
- Ramrattan RS, Van der Schaft TL, Mooy CM, De Bruijn WC, Mulder PGH, De Jong PTVM. Morphometric analysis of Bruch’s membrane, the choriocapillaris, and the choroid in aging. Invest Ophthalmol Vis Sci. 1994;35:2857–2864.
- Reiner A, Fitzgerald MEC, Del Mar N, Li C. Neural control of choroidal blood flow. Prog Retin Eye Res. 2018;64:96–130. doi:10.1016/j.preteyeres.2017.12.001.
- Sellheyer K. Development of the choroid and related structures. Eye. 1990;4(2):255–261. doi:10.1038/eye.1990.37.
- Olver JM. Functional anatomy of the choroidal circulation: methyl methacrylate casting of human choroid. Eye. 1990;4(2):262–272. doi:10.1038/eye.1990.38.
- Bill A, Sperber G, Ujiie K. Physiology of the choroidal vascular bed. Int Ophthalmol. 1983;6(2):101–107. doi:10.1007/bf00127638.
- Zouache MA, Eames I, Luthert PJ. Blood flow in the choriocapillaris. J Fluid Mech. 2015;774:37–66. doi:10.1017/jfm.2015.243.
- Hayreh SS. In vivo choroidal circulation and its watershed zones. Eye. 1990;4(2):273–289. doi:10.1038/eye.1990.39.
- Marmor MF, Cohen DS, Abdul-Rahim AS. Metabolic factors in the maintenance of retinal adhesion, Oct 14–19, 1979. Proc Conf Subretinal Space, 1981:77–85, Jerusalem. doi:10.1007/978-94-009-8653-4_6.
- Hayreh SS. Physiological anatomy of the choroidal vascular bed. Int Ophthalmol. 1983;6(2):85–93. doi:10.1007/bf00127636.
- Drexler W, Fujimoto J. State-of-the-art retinal optical coherence tomography. Prog Retin Eye Res. 2008;27(1):45–88. doi:10.1016/j.preteyeres.2007.07.005.
- Mrejen S, Spaide RF. Optical coherence tomography: imaging of the choroid and beyond. Surv Ophthalmol. 2013;58(5):387–429. doi:10.1016/j.survophthal.2012.12.001.
- Vira J, Marchese A, Singh RB, Agarwal A. Swept-source optical coherence tomography imaging of the retinochoroid and beyond. Expert Rev Med Devices. 2020;17(5):413–426. doi:10.1080/17434440.2020.1755256.
- Borrelli E, Sarraf D, Freund KB, Sadda SR. OCT angiography and evaluation of the choroid and choroidal vascular disorders. Prog Retin Eye Res. 2018;67:30–55. doi:10.1016/j.preteyeres.2018.07.002.
- Ferrara D, Waheed NK, Duker JS. Investigating the choriocapillaris and choroidal vasculature with new optical coherence tomography technologies. Prog Retin Eye Res. 2016;52:130–155. doi:10.1016/j.preteyeres.2015.10.002.
- Spaide RF, Fujimoto JG, Waheed NK, Sadda SR, Staurenghi G. Optical coherence tomography angiography. Prog Retin Eye Res. 2018;64:1–55. doi:10.1016/j.preteyeres.2017.11.003.
- Jia Y, Bailey ST, Wilson DJ, et al. Quantitative optical coherence tomography angiography of choroidal neovascularization in age-related macular degeneration. Ophthalmology. 2014;121(7):1435–1444.doi:10.1016/j.ophtha.2014.01.034.
- Kashani AH, Chen C-L, Gahm JK, et al. Optical coherence tomography angiography: a comprehensive review of current methods and clinical applications. Prog Retin Eye Res. 2017;60:66–100. doi:10.1016/j.preteyeres.2017.07.002.
- Ploner SB, Moult EM, Choi W, et al. Toward quantitative optical coherence tomography angiography. Retina. 2016;36(Supplement 1):S118–26.doi:10.1097/iae.0000000000001328.
- Choi W, Waheed NK, Moult EM, et al. Ultrahigh speed swept source optical coherence tomography angiography of retinal and choriocapillaris alterations in diabetic patients with and without retinopathy. Retina. 2017;37(1):11–21.doi:10.1097/iae.0000000000001250.
- Velaga SB, Nittala MG, Vupparaboina KK, et al. Choroidal vascularity index and choroidal thickness in eyes with reticular pseudodrusen. Retina. 2019;40(4):612–617.doi:10.1097/iae.0000000000002667.
- Agrawal R, Gupta P, Tan K-A, Cheung CMG, Wong T-Y, Cheng C-Y. Choroidal vascularity index as a measure of vascular status of the choroid: measurements in healthy eyes from a population-based study. Sci Rep. 2016;6(1):6. doi:10.1038/srep21090.
- Sonoda S, Sakamoto T, Yamashita T, et al. Choroidal structure in normal eyes and after photodynamic therapy determined by binarization of optical coherence tomographic images. Investig Opthalmol Vis Sci. 2014;55(6):3893.doi:10.1167/iovs.14-14447.
- Keenan TD, Klein B, Agrón E, Chew EY, Cukras CA, Wong WT. Choroidal thickness and vascularity vary with disease severity and subretinal drusenoid deposit presence in nonadvanced age-related macular degeneration. Retina. 2020;40(4):632–642. doi:10.1097/iae.0000000000002434.
- Invernizzi A, Agarwal A, Cozzi M, Viola F, Nguyen QD, Staurenghi G. Enhanced depth imaging optical coherence tomography features in areas of choriocapillaris hypoperfusion. Retina. 2016;36(10):2013–2021. doi:10.1097/IAE.0000000000001031.
- Betzler BK, Ding J, Wei X, et al. Choroidal vascularity index: a step towards software as a medical device. Br J Ophthalmol. 2021. doi:10.1136/bjophthalmol-2021-318782.
- Urs R, Ketterling JA, Yu ACH, Lloyd HO, Yiu BYS, Silverman RH. Ultrasound imaging and measurement of choroidal blood flow. Transl Vis Sci Technol. 2018;7(5):5. doi:10.1167/tvst.7.5.5.
- Arya M, Rashad R, Sorour O, Moult EM, Fujimoto JG, Waheed NK. Optical coherence tomography angiography (OCTA) flow speed mapping technology for retinal diseases. Expert Rev Med Devices. 2018;15(12):875–882. doi:10.1080/17434440.2018.1548932.
- Cavallerano A, Katalinic P, Cavallerano J. Joslin vision network examination for diabetic retinopathy in a veterans hospital - PHASE 2. Optom Vis Sci. 2002;79(Supplement):160. doi:10.1097/00006324-200212001-00307.
- Saeedi P, Petersohn I, Salpea P, et al. Global and regional diabetes prevalence estimates for 2019 and projections for 2030 and 2045: results from the International Diabetes Federation Diabetes Atlas, 9th edition. Diabetes Res Clin Pract. 2019;157:107843. doi:10.1016/j.diabres.2019.107843.
- Langham ME, Grebe R, Hopkins S, Marcus S, Sebag M. Choroidal blood flow in diabetic retinopathy. Exp Eye Res. 1991;52(2):167–173. doi:10.1016/0014-4835(91)90256-e.
- MacKinnon JR, O’Brien C, Swa K, Aspinall P, Butt Z, Cameron D. Pulsatile ocular blood flow in untreated diabetic retinopathy. Acta Ophthalmol Scand. 2009;75(6):661–664. doi:10.1111/j.1600-0420.1997.tb00626.x.
- Geyer O, Neudorfer M, Snir T, et al. Pulsatile ocular blood flow in diabetic retinopathy. Acta Ophthalmol Scand. 1999;77(5):522–525.doi:10.1034/j.1600-0420.1999.770507.x.
- Nagaoka T, Kitaya N, Sugawara R, et al. Alteration of choroidal circulation in the foveal region in patients with type 2 diabetes. Br J Ophthalmol. 2004 88;88(8):1060–1063. doi:10.1136/bjo.2003.035345.
- Mendívil A, Cuartero V, Mendívil MP. Ocular blood flow velocities in patients with proliferative diabetic retinopathy before and after scatter photocoagulation: a prospective study. Eur J Ophthalmol. 1995;5(4):259–264. doi:10.1177/112067219500500411.
- Arai T, Numata K, Tanaka K, et al. Ocular arterial flow hemodynamics in patients with diabetes mellitus. J Ultrasound Med. 1998;17(11):675–681.doi:10.7863/jum.1998.17.11.675.
- Hidayat AA, Fine BS. Diabetic Choroidopathy. Ophthalmology. 1985;92(4):512–522. doi:10.1016/s0161-6420(85)34013-7.
- Fryczkowski AW, Hodes BL, Walker J. Diabetic choroidal and iris vasculature scanning electron microscopy findings. Int Ophthalmol. 1989;13(4):269–279. doi:10.1007/bf02280087.
- Cao J. Choriocapillaris degeneration and related pathologic changes in human diabetic eyes. Arch Ophthalmol. 1998;116(5):589. doi:10.1001/archopht.116.5.589.
- Lutty GA. Diabetic choroidopathy. Vision Res. 2017;139:161–167. doi:10.1016/j.visres.2017.04.011.
- Melancia D, Vicente A, Cunha JP, Abegão Pinto L, Ferreira J. Diabetic choroidopathy: a review of the current literature. Graefe’s Arch Clin Exp Ophthalmol. 2016;254(8):1453–1461. doi:10.1007/s00417-016-3360-8.
- Shiragami C, Shiraga F, Matsuo T, Tsuchida Y, Ohtsuki H. Risk factors for diabetic choroidopathy in patients with diabetic retinopathy. Graefe’s Arch Clin Exp Ophthalmol. 2002;240(6):436–442. doi:10.1007/s00417-002-0451-5.
- Hua R, Liu L, Wang X, Chen L. Imaging evidence of diabetic choroidopathy in vivo: angiographic pathoanatomy and choroidal-enhanced depth imaging. PLoS One. 2013;8(12):e83494. doi:10.1371/journal.pone.0083494.
- Carnevali A, Sacconi R, Corbelli E, et al. Optical coherence tomography angiography analysis of retinal vascular plexuses and choriocapillaris in patients with type 1 diabetes without diabetic retinopathy. Acta Diabetol. 2017;54(7):695–702.doi:10.1007/s00592-017-0996-8.
- Tan K-A, Laude A, Yip V, Loo E, Wong EP, Agrawal R. Choroidal vascularity index - a novel optical coherence tomography parameter for disease monitoring in diabetes mellitus?. Acta Ophthalmol. 2016;94(7):e612–6. doi:10.1111/aos.13044.
- Wang JC, Laíns I, Providência J, et al. Diabetic choroidopathy: choroidal vascular density and volume in diabetic retinopathy with swept-source optical coherence tomography. Am J Ophthalmol. 2017;184:75–83. doi:10.1016/J.AJO.2017.09.030.
- Kim M, Ha MJ, Choi SY, Park Y-H, Rana V. Choroidal vascularity index in type-2 diabetes analyzed by swept-source optical coherence tomography. Sci Rep. 2018;8(1):8. doi:10.1038/s41598-017-18511-7.
- Borrelli E, Sacconi R, Querques G, Bandello F. Optical coherence tomography angiography in the management of diabetic retinopathy. Indian J Ophthalmol. 2021;69(11):3009. doi:10.4103/IJO.IJO_1367_21.
- Dai Y, Zhou H, Zhang Q, et al. Quantitative assessment of choriocapillaris flow deficits in diabetic retinopathy: A swept-source optical coherence tomography angiography study. PLoS One. 2020;15. doi:10.1371/JOURNAL.PONE.0243830.
- Zhang Y, Qin Y, Wang S, Liu Y, Li X, Sun X. Higher choroidal thickness and lower choriocapillaris blood flow signal density based on optical coherence tomography angiography in diabetics. Sci Rep. 2021;11(1):11. doi:10.1038/S41598-021-85065-0.
- Borrelli E, Palmieri M, Viggiano P, Ferro G, Mastropasqua R. Photoreceptor damage in diabetic choroidopathy. Retina. 2020;40(6):1062–1069. doi:10.1097/IAE.0000000000002538.
- Dodo Y, Suzuma K, Ishihara K, et al. Clinical relevance of reduced decorrelation signals in the diabetic inner choroid on optical coherence tomography angiography. Sci Rep. 2017;7(1):7.doi:10.1038/S41598-017-05663-9.
- Sawada O, Ichiyama Y, Obata S, et al. Comparison between wide-angle OCT angiography and ultra-wide field fluorescein angiography for detecting non-perfusion areas and retinal neovascularization in eyes with diabetic retinopathy. Graefe’s Arch Clin Exp Ophthalmol. 2018;256(7):1275–1280.doi:10.1007/s00417-018-3992-y.
- Cui Y, Zhu Y, Wang JC, et al. Comparison of widefield swept-source optical coherence tomography angiography with ultra-widefield colour fundus photography and fluorescein angiography for detection of lesions in diabetic retinopathy. Br J Ophthalmol. 2021;105(4):577–581.doi:10.1136/bjophthalmol-2020-316245.
- You QS, Guo Y, Wang J, et al. Detection of clinically unsuspected retinal neovascularization with wide-field optical coherence tomography angiography. Retina. 2020;4(1):40.doi:10.1097/IAE.0000000000002487.
- Takahashi A, Nagaoka T, Sato E, Yoshida A. Effect of panretinal photocoagulation on choroidal circulation in the foveal region in patients with severe diabetic retinopathy. Br J Ophthalmol. 2008;92(10):1369–1373. doi:10.1136/bjo.2007.136028.
- Okamoto M, Yamashita M, Ogata N. Effects of intravitreal injection of ranibizumab on choroidal structure and blood flow in eyes with diabetic macular edema. Graefe’s Arch Clin Exp Ophthalmol. 2018;256(5):885–892. doi:10.1007/s00417-018-3939-3.
- Conti FF, Song W, Rodrigues EB, Singh RP. Changes in retinal and choriocapillaris density in diabetic patients receiving anti-vascular endothelial growth factor treatment using optical coherence tomography angiography. Int J Retin Vitr. 2019 5; 5(1). doi:10.1186/s40942-019-0192-9
- May CA. Chronologic versus biologic aging of the human choroid. Sci World J. 2013;2013:1–7. doi:10.1155/2013/378206.
- Ikuno Y, Kawaguchi K, Nouchi T, Yasuno Y. Choroidal thickness in healthy Japanese subjects. Investig Opthalmol Vis Sci. 2010;51(4):2173. doi:10.1167/iovs.09-4383.
- Bin WW, Xu L, Jonas JB, et al. Subfoveal choroidal thickness: the Beijing eye study. Ophthalmology. 2013;120(1):175–180.doi:10.1016/j.ophtha.2012.07.048.
- Zhou H, Dai Y, Shi Y, et al. Age-Related changes in choroidal thickness and the volume of vessels and stroma using Swept-Source OCT and fully automated algorithms. Ophthalmol Retina. 2020;4(2):204–215.doi:10.1016/j.oret.2019.09.012.
- Wong WL, Su X, Li X, et al. Global prevalence of age-related macular degeneration and disease burden projection for 2020 and 2040: a systematic review and meta-analysis. Lancet Glob Health. 2014;2(2):e106–16.doi:10.1016/s2214-109x(13)70145-1.
- Lutty G, Grunwald J, Majji AB, Uyama M, Yoneya S. Changes in choriocapillaris and retinal pigment epithelium in age-related macular degeneration. Mol Vis. 1999;5:35.
- Mori F. Pulsatile ocular blood flow study: decreases in exudative age related macular degeneration. Br J Ophthalmol. 2001;85(5):531–533. doi:10.1136/bjo.85.5.531.
- Arya M, Sabrosa AS, Duker JS, Waheed NK. Choriocapillaris changes in dry age-related macular degeneration and geographic atrophy: a review. Eye Vis. 2018 5; 5(1). doi:10.1186/s40662-018-0118-x
- Curcio CA, Messinger JD, Sloan KR, McGwin G, Medeiros NE, Spaide RF. Subretinal drusenoid deposits in non-neovascular age-related macular degeneration. Retina. 2013;33(2):265–276. doi:10.1097/iae.0b013e31827e25e0.
- Moreira-Neto CA, Moult EM, Fujimoto JG, Waheed NK, Ferrara D. Choriocapillaris loss in advanced age-related macular degeneration. J Ophthalmol. 2018;2018:1–6. doi:10.1155/2018/8125267.
- Moult E, Choi W, Waheed NK, et al. Ultrahigh-speed Swept-Source OCT angiography in exudative AMD. Ophthalmic Surg Lasers Imaging Retina. 2014;45(6):496–505.doi:10.3928/23258160-20141118-03.
- Warrow DJ, Hoang QV, Freund KB. Pachychoroid pigment epitheliopathy. Retina. 2013;33(8):1659–1672. doi:10.1097/iae.0b013e3182953df4.
- Gallego-Pinazo R, Dolz-Marco R, Freund KB. Pachychoroid. Choroidal Disord. 2017:161–170. doi:10.1016/b978-0-12-805313-3.00010-7
- Dansingani KK, Balaratnasingam C, Naysan J, Freund KB. En face imaging of pachychoroid spectrum disorders with swept-source optical coherence tomography. Retina. 2016;36(3):499–516. doi:10.1097/iae.0000000000000742.
- Lehmann M, Bousquet E, Beydoun T, Behar-Cohen F. Pachychoroid. Retina. 2015;35(1):10–16. doi:10.1097/iae.0000000000000287.
- Baek J, Kook L, Lee WK, Müri RM, Knoch D. Choriocapillaris flow impairments in association with pachyvessel in early stages of pachychoroid. Sci Rep. 2019;9(1):9. doi:10.1038/s41598-019-42052-w.
- Okubo A, Ito M, Sameshima M, Uemura A, Sakamoto T. Pulsatile blood flow in the polypoidal choroidal vasculopathy. Ophthalmology. 2005;112(8):1436–1441. doi:10.1016/j.ophtha.2005.03.017.
- Alasil T, Ferrara D, Adhi M, et al. En face imaging of the choroid in polypoidal choroidal vasculopathy using swept-source optical coherence tomography. Am J Ophthalmol. 2015;159(4):634–643.e2.doi:10.1016/j.ajo.2014.12.012.
- Costa RA, Navajas EV, Farah ME, Calucci D, Cardillo JA, Scott IU. Polypoidal choroidal vasculopathy: angiographic characterization of the network vascular elements and a new treatment paradigm. Prog Retina Eye Res. 2005;24(5):560–586. doi:10.1016/j.preteyeres.2005.01.001.
- Sasahara M, Tsujikawa A, Musashi K, et al. Polypoidal choroidal vasculopathy with choroidal vascular hyperpermeability. Am J Ophthalmol. 2006;142(4):601–607.e1.doi:10.1016/j.ajo.2006.05.051.
- Inoue M, Balaratnasingam C, Freund KB. Optical coherence tomography angiography of polypoidal choroidal vasculopathy and polypoidal choroidal neovascularization. Retina. 2015;35(11):2265–2274. doi:10.1097/iae.0000000000000777.
- Srour M, Querques G, Semoun O, et al. Optical coherence tomography angiography characteristics of polypoidal choroidal vasculopathy. Br J Ophthalmol. 2016;100(11):1489–1493.doi:10.1136/bjophthalmol-2015-307892.
- Wong CW, Yanagi Y, Lee WK, et al. Age-related macular degeneration and polypoidal choroidal vasculopathy in Asians. Prog Retina Eye Res. 2016;53. doi:10.1016/j.preteyeres.2016.04.002.
- Semeraro F, Morescalchi F, Russo A, et al. Central serous chorioretinopathy: pathogenesis and management. Clin Ophthalmol. 2019;13:2341–2352. doi:10.2147/opth.s220845.
- Saito W, Hashimoto Y, Hirooka K, Ishida S. Changes in choroidal blood flow velocity in patients diagnosed with central serous chorioretinopathy during follow-up for pachychoroid pigment epitheliopathy. Am J Ophthalmol Case Rep. 2020;18:100651. doi:10.1016/j.ajoc.2020.100651.
- Kitaya N, Nagaoka T, Hikichi T, et al. Features of abnormal choroidal circulation in central serous chorioretinopathy. Br J Ophthalmol. 2003;87(6):709–712.doi:10.1136/bjo.87.6.709.
- Mrejen S, Sarraf D, Chexal S, Wald K, Freund KB. Choroidal involvement in acute posterior multifocal placoid pigment epitheliopathy. Ophthalmic Surg Lasers Imaging Retina 2016;47:20–26. doi:10.3928/23258160-20151214-03.
- Shinojima A, Kawamura A, Mori R, Fujita K, Yuzawa M. Findings of optical coherence tomographic angiography at the choriocapillaris level in central serous chorioretinopathy. Ophthalmologica. 2016;236(2):108–113. doi:10.1159/000448436.
- Qu Y, Gong D, Yu W, Dong F. Characteristics of the choriocapillaris layer in optical coherence tomography angiography of acute central serous chorioretinopathy. Ophthalmic Surg Lasers Imaging Retina. 2017;48(12):1000–1005. doi:10.3928/23258160-20171130-07.
- Rochepeau C, Kodjikian L, Garcia M-A, et al. Optical coherence tomography angiography quantitative assessment of choriocapillaris blood flow in central serous chorioretinopathy. Am J Ophthalmol. 2018;194:26–34. doi:10.1016/j.ajo.2018.07.004.
- Yun C, Huh J, Ahn SM, et al. Choriocapillaris flow features and choroidal vasculature in the fellow eyes of patients with acute central serous chorioretinopathy. Graefe’s Arch Clin Exp Ophthalmol. 2018;257(1):57–70.doi:10.1007/s00417-018-4179-2.
- Matet A, Daruich A, Hardy S, Behar-Cohen F. Patterns of choriocapillaris flow signal voids in central serous chorioretinopathy. Retina. 2019;39(11):2178–2188. doi:10.1097/iae.0000000000002271.
- Dell’omo R, Wong R, Marino M, Konstantopoulou K, Pavesio C. Relationship between different fluorescein and indocyanine green angiography features in multiple evanescent white dot syndrome. Br J Ophthalmol. 2009;94(1):59–63. doi:10.1136/bjo.2009.163550.
- Herbort CP, LeHoang P, Guex-Crosier Y. Schematic interpretation of indocyanine green angiography in posterior uveitis using a standard angiographic protocol. Ophthalmology 1998;105:432–440. doi:10.1016/s0161-6420(98)93024-x.
- Ie D, Glaser BM, Murphy RP, Gordon LW, Sjaarda RN, Thompson JT. Indocyanine green angiography in multiple evanescent white-dot syndrome. Am J Ophthalmol. 1994;117(1):7–12. doi:10.1016/s0002-9394(14)73008-9.
- Pichi F, Srvivastava SK, Chexal S, et al. En face optical coherence tomography and optical coherence tomography angiography of multiple evanescent white dot syndromE. Retina. 2016;36(Supplement 1):S178–88.doi:10.1097/iae.0000000000001255.
- Yannuzzi NA, Swaminathan SS, Zheng F, et al. Swept-Source OCT angiography shows sparing of the choriocapillaris in multiple evanescent white dot syndrome. Ophthalmic Surg Lasers Imaging Retina. 2017;48(1):69–74.doi:10.3928/23258160-20161219-10.
- Pereira F, Lima LH, Sah, et al. Swept-source OCT in patients with multiple evanescent white dot syndrome. J Ophthalmic Inflamm Infect. 2018;8(1):8. doi:10.1186/s12348-018-0159-2.
- Zicarelli F, Mantovani A, Preziosa C, Staurenghi G. Multimodal imaging of multiple evanescent white dot syndrome: a new interpretation. Ocul Immunol Inflamm. 2019;28(5):814–820. doi:10.1080/09273948.2019.1635169.
- Obana A, Kusumi M, Miki T. Indocyanine green angiographic aspects of multiple evanescent white dot syndrome. Retina. 1996;16(2):97–104. doi:10.1097/00006982-199616020-00002.
- Aoyagi R, Hayashi T, Masai A, et al. Subfoveal choroidal thickness in multiple evanescent white dot syndrome. Clin Exp Optom. 2012;95(2):212–217.doi:10.1111/j.1444-0938.2011.00668.x.
- Fiore T, Iaccheri B, Cerquaglia A, et al. Outer retinal and choroidal evaluation in multiple evanescent white dot syndrome (MEWDS): an enhanced depth imaging optical coherence tomography study. Ocul Immunol Inflamm. 2016;26(3):428–434.doi:10.1080/09273948.2016.1231329.
- Hashimoto Y, Saito W, Saito M, et al. Decreased choroidal blood flow velocity in the pathogenesis of multiple evanescent white dot syndrome. Graefe’s Arch Clin Exp Ophthalmol. 2014;253(9):1457–1464.doi:10.1007/s00417-014-2831-z.
- Gass JDM. Acute posterior multifocal placoid pigment epitheliopathy. Arch Ophthalmol 1968;80:177–185. doi:10.1001/archopht.1968.00980050179005.
- Van Buskirk EM, Lessell S, Friedman E. Pigmentary epitheliopathy and erythema nodosum. Arch Ophthalmol. 1971;85(3):369–372. doi:10.1001/archopht.1971.00990050371025.
- Deutman AF, Boen-Tan TN, Oosterhuis JA. Acute posterior multifocal placoid pigment epitheliopathy. Ophthalmologica 1973;167:368–372. doi:10.1159/000306976.
- Yuzawa M, Kawamura A, Matsui M. Indocyanine green video angiographic findings in acute posterior multifocal placoid pigment epitheliopathy. Acta Ophthalmol 2009;72:128–133. doi:10.1111/j.1755-3768.1994.tb02753.x.
- Park D, Schatz H, McDonald HR, Johnson RN. Indocyanine green angiography of acute mult-if-ocal posterior placoid pigment epitheliopathy. Ophthalmology 1995;102:1877–1883. doi:10.1016/s0161-6420(95)30780-4.
- Dhaliwal RS, Maguire AM, Flower RW, Arribas NP. Acute posterior multifocal placoid pigment epitheliopathy. Retina. 1993;13(4):317–325. doi:10.1097/00006982-199313040-00009.
- Park D, Schatz H, Mcdonald HR, Johnson RN. Acute multifocal posterior placoid pigment epitheliopathy: a theory of pathogenesis. Retina. 1995;15(4):351–352. doi:10.1097/00006982-199515040-00013.
- Spaide RF. Autofluorescence imaging of acute posterior multifocal placoid pigment epitheliopathy. Retina. 2006;26(4):479–482. doi:10.1097/00006982-200604000-00020.
- Furino C, Shalchi Z, Grassi MO, et al. OCT angiography in acute posterior multifocal placoid pigment epitheliopathy. Ophthalmic Surg Lasers Imaging Retina 2019;50:428–436. doi:10.3928/23258160-20190703-04.
- Klufas MA, Phasukkijwatana N, Iafe NA, et al. Optical coherence tomography angiography reveals choriocapillaris flow reduction in placoid chorioretinitis. Ophthalmol Retina. 2017;1(1):77–91.doi:10.1016/j.oret.2016.08.008.
- Burke TR, Chu CJ, Salvatore S, et al. Application of OCT-angiography to characterise the evolution of chorioretinal lesions in acute posterior multifocal placoid pigment epitheliopathy. Eye 2017;31:1399–1408. doi:10.1038/eye.2017.180.
- Spaide RF, Goldberg N, Freund KB. Redefining multifocal choroiditis and panuveitis and punctate inner choroidopathy through multimodal imaging. Retina. 2013;33(7):1315–1324. doi:10.1097/iae.0b013e318286cc77.
- Campos J, Campos A, Beselga D, Mendes S, Neves A, Sousa JPC. Punctate inner choroidopathy: a clinical case report. Case Rep Ophthalmol. 2013;4(3):155–159. doi:10.1159/000355389.
- Li J, Li Y, Li H, Zhang L. Imageology features of different types of multifocal choroiditis. BMC Ophthalmol. 201919. doi:10.1186/s12886-019-1045-x
- Tavallali A, Yannuzzi L. Idiopathic multifocal choroiditis. J Ophthalmic Vis Res. 2016;11(4):429. doi:10.4103/2008-322x.194141.
- Zahid S, Chen KC, Jung JJ, et al. Optical coherence tomography angiography of chorioretinal lesions due to idiopathic multifocal choroiditis. Retina. 2017;37(8):1451–1463.doi:10.1097/iae.0000000000001381.
- Cheng L, Chen X, Weng S, et al. Spectral-Domain optical coherence tomography angiography findings in multifocal choroiditis with active lesions. Am J Ophthalmol. 2016;169:145–161. doi:10.1016/j.ajo.2016.06.029.
- Thompson IA, Caplash S, Akanda M, et al. Optical coherence tomography angiography changes in choroidal vasculature following treatment in punctate inner choroidopathy. Ocul Immunol Inflamm. 2020:1–7. doi:10.1080/09273948.2019.1705986.
- Kim EL, Thanos A, Yonekawa Y, et al. Optical coherence tomography angiography findings in punctate inner choroidopathy. Ophthalmic Surg Lasers Imaging Retina. 2017;48(10):786–792.doi:10.3928/23258160-20170928-02.
- Hampton BM, Aderman CM, Flynn HW, Sridhar J. Optical coherence tomography angiography of punctate inner choroidopathy. Case Rep Ophthalmol Med. 2017;2017:1–3. doi:10.1155/2017/4754231.
- Vezzola D, Allegrini D, Borgia A, et al. Swept-source optical coherence tomography and optical coherence tomography angiography in acquired toxoplasmic chorioretinitis: a case report. J Med Case Rep. 2018;12(1):12.doi:10.1186/s13256-018-1902-x.
- Mansour AM, Jampol LEEM, Packo KH, Hrisomalos NF. Macular serpiginous choroiditis. Retina. 1988;8(2):125–131. doi:10.1097/00006982-198808020-00008.
- Giovannini A, Mariotti C, Ripa E, Scassellati-Sforzolini B. Indocyanine green angiographic findings in serpiginous choroidopathy. Br J Ophthalmol. 1996;80(6):536–540. doi:10.1136/bjo.80.6.536.
- Mandadi SKR, Agarwal A, Aggarwal K, et al. Novel findings on optical coherence tomography angiography in patients with tubercular serpiginous-like choroiditis. Retina. 2017;37(9):1647–1659.doi:10.1097/iae.0000000000001412.
- El Ameen A, Herbort CP. Serpiginous choroiditis imaged by optical coherence tomography angiography. Retin Cases Brief Rep. 2018;12(4):279–285. doi:10.1097/icb.0000000000000512.
- Desai R, Nesper P, Goldstein DA, Fawzi AA, Jampol LM, Gill M. OCT Angiography imaging in serpiginous choroidopathy. Ophthalmol Retin. 2018;2(4):351–359. doi:10.1016/j.oret.2017.07.023.
- Pakzad-Vaezi K, Khaksari K, Chu Z, Van Gelder RN, Wang RK, Pepple KL. Swept-Source OCT angiography of serpiginous choroiditis. Ophthalmol Retin. 2018;2(7):712–719. doi:10.1016/j.oret.2017.11.001.
- Minos E, Barry RJ, Southworth S, et al. Birdshot chorioretinopathy: current knowledge and new concepts in pathophysiology, diagnosis, monitoring and treatment. Orphanet J Rare Dis. 2016;11:11. doi:10.1186/s13023-016-0429-8.
- Shah KH, Levinson RD, Yu F, et al. Birdshot chorioretinopathy. Surv Ophthalmol. 2005;50(6):519–541.doi:10.1016/j.survophthal.2005.08.004.
- Papadia M, Herbort C. Indocyanine green angiography (ICGA) is essential for the early diagnosis of birdshot chorioretinopathy. Klin Monbl Augenheilkd. 2012;229(4):348–352. doi:10.1055/s-0031-1299224.
- Reddy AK, Gonzalez MA, Henry CR, Yeh S, Sobrin L, Albini TA. Diagnostic sensitivity of indocyanine green angiography for birdshot chorioretinopathy. JAMA Ophthalmol. 2015;133(7):840. doi:10.1001/jamaophthalmol.2015.0822.
- Pepple KL, Chu Z, Weinstein J, Munk MR, Van Gelder RN, Wang RK. Use of en face swept-source optical coherence tomography angiography in identifying choroidal flow voids in 3 patients with birdshot chorioretinopathy. JAMA Ophthalmol. 2018;136(11):1288. doi:10.1001/jamaophthalmol.2018.3474.
- Agrawal R, Agarwal A, Jabs DA, et al. Standardization of Nomenclature for Ocular Tuberculosis–Results of Collaborative Ocular Tuberculosis Study (COTS) Workshop. Ocul Immunol Inflamm. 2019. doi:10.1080/09273948.2019.1653933.
- Agrawal R, Gunasekeran DV, Agarwal A, et al. The collaborative ocular tuberculosis study (COTS)-1: a multinational description of the spectrum of choroidal involvement in 245 patients with tubercular uveitis. Ocul Immunol Inflamm. 2018. doi:10.1080/09273948.2018.1489061.
- Agarwal A, Marchese A, Rabiolo A, Agrawal R, Bansal R, Gupta V. Clinical and imaging factors associated with the outcomes of tubercular Serpiginous-like choroiditis. Am J Ophthalmol. 2020;220:160–169. doi:10.1016/J.AJO.2020.07.024.
- Agarwal A, Aggarwal K, Mandadi SKR, et al. Longitudinal follow-up of tubercular serpiginous-like choroiditis using optical coherence tomography angiography. Retina. 2021;41(4):793–803.doi:10.1097/IAE.0000000000002915.
- Wang H, Tan SZ, Aslam T, Jones NP, Steeples LR. Multimodal evaluation of presumed tuberculous serpiginous-like choroiditis. Ocul Immunol Inflamm. 2018;27(7):1149–1153. doi:10.1080/09273948.2018.1501497.
- Agarwal A, Agrawal R, Khandelwal N, et al. Choroidal structural changes in tubercular multifocal serpiginoid choroiditis. Ocul Immunol Inflamm. 2018;26. doi:10.1080/09273948.2017.1370650.
- Gupta A, Bansal R, Gupta V, Sharma A. Fundus autofluorescence in serpiginouslike choroiditis. Retina. 2012;32(4):814–825. doi:10.1097/iae.0b013e3182278c41.
- Agarwal A, Aggarwal K, Deokar A, et al. Optical coherence tomography angiography features of paradoxical worsening in tubercular multifocal serpiginoid choroiditis. Ocul Immunol Inflamm. 2016;24(6):621–630.doi:10.1080/09273948.2016.1207785.
- Agarwal A, Aggarwal K, Pichi F, et al. Clinical and multimodal imaging clues in differentiating between tuberculomas and sarcoid choroidal granulomas. Am J Ophthalmol. 2021;226:42–55. doi:10.1016/j.ajo.2021.01.025.
- Agarwal A, Invernizzi A, Markan A, et al. Imaging in tubercular choroiditis: current concepts. Ocul Immunol Inflamm. 2020;28. doi:10.1080/09273948.2020.1817500.
- Jain S, Agarwal A, Gupta V. Resolution of large choroidal tuberculoma following monotherapy with intravitreal ranibizumab. Ocul Immunol Inflamm. 2020;28. doi:10.1080/09273948.2019.1582786.
- Aksoy FE, Altan C, Basarir B. Multimodal imaging of a choroidal granuloma as a first sign of tuberculosis. Photodiagn Photodyn Ther. 2020;29:101580. doi:10.1016/j.pdpdt.2019.101580.
- Goldenberg D, Goldstein M, Loewenstein A, Habot-Wilner Z. Vitreal, retinal, and choroidal findings in active and scarred toxoplasmosis lesions: a prospective study by spectral-domain optical coherence tomography. Graefe’s Arch Clin Exp Ophthalmol. 2013;251(8):2037–2045. doi:10.1007/s00417-013-2334-3.
- Park JH, Lee S-Y, Lee EK. Morphological characteristics of ocular toxoplasmosis and its regression pattern on swept-source optical coherence tomography angiography: a case report. BMC Ophthalmol. 2019;19(1):19. doi:10.1186/s12886-019-1209-8.
- Zicarelli F, Pichi F, Parrulli S, et al. Acute posterior ocular toxoplasmosis: an optical coherence tomography angiography and dye angiography study. Ocul Immunol Inflamm. 2021. doi:10.1080/09273948.2021.1977831.
- Du L, Kijlstra A, Yang P. Vogt-Koyanagi-Harada disease: novel insights into pathophysiology, diagnosis and treatment. Prog Retin Eye Res. 2016;55:52. doi:10.1016/j.preteyeres.2016.02.002.
- Yang P, Zhong Y, Du L, et al. Development and evaluation of diagnostic criteria for Vogt-Koyanagi-Harada disease. JAMA Ophthalmol. 2018;136. doi:10.1001/jamaophthalmol.2018.2664.
- Damico FM, Kiss S, Young LH. Vogt-Koyanagi-Harada disease. Semin Ophthalmol. 2005;20(3):183–190. doi:10.1080/08820530500232126.
- Read R, … NR-C opinion in, 2000 undefined. Vogt-Koyanagi-Harada disease. JournalsLwwCom. n.d.
- Sakata VM, Da Silva FT, Hirata CE, De Carvalho JF, Yamamoto JH. Diagnosis and classification of Vogt-Koyanagi-Harada disease. Autoimmun Rev. 2014;13. doi:10.1016/j.autrev.2014.01.023.
- Rao NA. Pathology of Vogt-Koyanagi-Harada disease. Int Ophthalmol. 2007;27. doi:10.1007/s10792-006-9029-2.
- Das D, Boddepalli A, Biswas J. Clinicopathological and immunohistochemistry correlation in a case of Vogt-Koyanagi-Harada disease. Indian J Ophthalmol. 2019;67. doi:10.4103/ijo.IJO_1800_18.
- Yang P, Liu S, Zhong Z, et al. Comparison of clinical features and visual outcome between sympathetic ophthalmia and Vogt–Koyanagi–Harada disease in Chinese patients. Ophthalmology. 2019;126. doi:10.1016/j.ophtha.2019.03.049.
- Chan CC. Relationship between Sympathetic Ophthalmia, Phacoanaphylactic Endophthalmitis, and Vogt-Koyanagi-Harada Disease. Ophthalmology. 1988;95. doi:10.1016/S0161-6420(88)33146-5.
- Al-Halafi A, Dhibi HA, Hamade IH, Bou Chacra CT, Tabbara KF. The association of systemic disorders with Vogt-Koyanagi-Harada and sympathetic ophthalmia. Graefe’s Arch Clin Exp Ophthalmol. 2011;249. doi:10.1007/s00417-011-1727-4.
- Shah DN, Al-Moujahed A, Newcomb CW, et al. Exudative retinal detachment in ocular inflammatory diseases: risk and predictive factors. Am J Ophthalmol. 2020;218:279–287. doi:10.1016/J.AJO.2020.06.019.
- Rao NA, Marak GE, Ishibashi T, Tawara A, Harada S. Sympathetic ophthalmia simulating Vogt-Koyanagi-Harada’s disease: a clinico-pathologic study of four cases. Jpn J Ophthalmol. 1983;27:27.
- Da Silva FT, Hirata CE, Sakata VM, et al. Indocyanine green angiography findings in patients with long-standing Vogt-Koyanagi-Harada disease: a cross-sectional study. BMC Ophthalmol. 2012;12:12. doi:10.1186/1471-2415-12-40.
- Bouchenaki N, Herbort CP. The contribution of indocyanine green angiography to the appraisal and management of Vogt-Koyanagi-Harada disease. Ophthalmology. 2001;108. doi:10.1016/S0161-6420(00)00428-0.
- Bouchenaki N, Herbort CP. Indocyanine green angiography guided management of Vogt-Koyanagi-Harada disease. J Ophthalmic Vis Res. 2011;6(4):241–8.
- Abouammoh MA, Gupta V, Hemachandran S, Herbort CP, Abu El-Asrar AM. Indocyanine green angiographic findings in initial-onset acute Vogt–Koyanagi–Harada disease. Acta Ophthalmol. 2016;94. doi:10.1111/aos.12974.
- Herbort CP, Mantovani A, Bouchenaki N. Indocyanine green angiography in Vogt-Koyanagi-Harada disease: angiographic signs and utility in patient follow-up. Int Ophthalmol. 2007;27. doi:10.1007/s10792-007-9060-y.
- Miyanaga M, Kawaguchi T, Miyata K, Horie S, Mochizuki M, Herbort CP. Indocyanine green angiography findings in initial acute pretreatment Vogt-Koyanagi-Harada disease in Japanese patients. Jpn J Ophthalmol. 2010;54. doi:10.1007/s10384-010-0853-6.
- Jeyaraman VA, Sudharshan S, Selvakumar A, Bassi S, Noronha OV. Isolated cortical blindness without simultaneous neurological involvement in progressive multifocal leukoencephalopathy in a patient with human immune deficiency virus infection. J Ophthalmic Inflamm Infect. 2013;3(1):3. doi:10.1186/1869-5760-3-3.
- Rostaqui O, Querques G, Haymann P, Fardeau C, Coscas G, Souied EH. Visualization of sarcoid choroidal granuloma by enhanced depth imaging optical coherence tomography. Ocul Immunol Inflamm. 2014;22. doi:10.3109/09273948.2013.835428.
- Invernizzi A, Mapelli C, Viola F, et al. Choroidal granulomas visualized by enhanced depth imaging optical coherence tomography. Retina. 2015 35;35(3):525–531. doi:10.1097/IAE.0000000000000312.
- Invernizzi A, Agarwal A, Mapelli C, Nguyen QD, Staurenghi G, Viola F. Longitudinal follow-up of choroidal granulomas using enhanced depth imaging optical coherence tomography. Retina. 2017;37. doi:10.1097/IAE.0000000000001128.
- Ishihara M, Shibuya E, Tanaka S, Mizuki N. Diagnostic and therapeutic evaluation of multiple choroidal granulomas in a patient with confirmed sarcoidosis using enhanced depth imaging optical coherence tomography. Int Ophthalmol. 2018;38. doi:10.1007/s10792-017-0720-2.
- Aggarwal K, Agarwal A, Mahajan S, et al. The role of optical coherence tomography angiography in the diagnosis and management of acute Vogt–Koyanagi–Harada disease. Ocul Immunol Inflamm. 2018 26;26(1):142–153. doi:10.1080/09273948.2016.1195001.
- Aggarwal K, Agarwal A, Deokar A, et al. Distinguishing features of acute Vogt-Koyanagi-Harada disease and acute central serous chorioretinopathy on optical coherence tomography angiography and en face optical coherence tomography imaging. J Ophthalmic Inflamm Infect. 2017;7(1):7.doi:10.1186/s12348-016-0122-z.
- Vingopoulos F, Cui Y, Katz R, et al. Widefield swept-source OCTA in Vogt-Koyanagi- Harada disease. Ophthalmic Surg Lasers Imaging Retina. 2020:51. doi:10.3928/23258160-20200702-06.
- Qian Y, Yang J, Liang A, Zhao C, Gao F, Zhang M. Widefield Swept-Source optical coherence tomography angiography assessment of choroidal changes in Vogt-Koyanagi-Harada disease. Front Med. 2021;8. doi:10.3389/fmed.2021.698644.
- Ye X, Zhang H, Xiao P, et al. Microvasculature features of Vogt-Koyanagi-Harada disease revealed by widefield Swept-Source optical coherence tomography angiography. Front Med. 2021;8. doi:10.3389/fmed.2021.719593.
- Brar M, Sharma M, Grewal SPS, Grewal DS. Treatment response in sympathetic ophthalmia as assessed by widefield OCT angiography. Ophthalmic Surg Lasers Imaging Retina. 2018;49. doi:10.3928/23258160-20180831-13.
- Sekiryu T. Choroidal imaging using optical coherence tomography: techniques and interpretations. Jpn J Ophthalmol. 2022;66. doi:10.1007/s10384-022-00902-7.
- Aggarwal K, Agarwal A, Sharma A, Sharma K, Gupta V. Detection of Type 1 choroidal neovascular membranes using optical coherence tomography angiography in tubercular posterior uveitis. Retina. 2019;39. doi:10.1097/IAE.0000000000002176.
- Aggarwal K, Agarwal A, Gupta V. Type 2 choroidal neovascularization in a choroidal granuloma detected using swept-source optical coherence tomography angiography. Ophthalmic Surg Lasers Imaging Retina. 2018;49. doi:10.3928/23258160-20180628-11.
- Arora A, Agarwal A, Bansal R, et al. Subretinal hyperreflective material (SHRM) as biomarker of activity in exudative and Non- exudative inflammatory choroidal neovascularization. Ocul Immunol Inflamm. 2021;1–8. doi:10.1080/09273948.2021.1980813.
- Zicarelli F, Azzolini C, Cornish E, et al. Optical coherence tomography features of choroidal neovascularization and their correlation with age, gender, and underlying disease. Retina. 2021;5(1):41.doi:10.1097/IAE.0000000000002984.
- Agarwal A, Invernizzi A, Singh RB, et al. An update on inflammatory choroidal neovascularization: epidemiology, multimodal imaging, and management. J Ophthalmic Inflamm Infect. 2018;8(1):8.doi:10.1186/s12348-018-0155-6.
- Campbell JP, Zhang M, Hwang TS, et al. Detailed vascular anatomy of the human retina by projection-resolved optical coherence tomography angiography. Sci Rep. 2017;7(1):7.doi:10.1038/srep42201.
- Chu Z, Cheng Y, Zhang Q, et al. Quantification of choriocapillaris with phansalkar local thresholding: pitfalls to avoid. Am J Ophthalmol. 2020;213:161–176. doi:10.1016/j.ajo.2020.02.003.
- Dabir S, Bhatt V, Bhatt D, et al. Need for manual segmentation in optical coherence tomography angiography of neovascular age-related macular degeneration. PLoS One. 2020;15. doi:10.1371/journal.pone.0244828.
- Sambhav K, Grover S, Chalam KV. The application of optical coherence tomography angiography in retinal diseases. Surv Ophthalmol. 2017;62. doi:10.1016/j.survophthal.2017.05.006.
- Singh SR, Vupparaboina KK, Goud A, Dansingani KK, Chhablani J. Choroidal imaging biomarkers. Surv Ophthalmol. 2019;64. doi:10.1016/j.survophthal.2018.11.002.
- Corvi F, Corradetti G, Sadda SVR. Correlation between the Angiographic Choriocapillaris and the Structural Inner Choroid. Curr Eye Res. 2021;46. doi:10.1080/02713683.2020.1846756.
- Preziosa C, Corvi F, Staurenghi G, Pellegrini M. Extended field imaging optical coherence tomography angiography for the study of retinal and choroidal changes after radiation therapy for choroidal melanoma: comparison with wide-field angiography. Retina. 2021;5(1):41. doi:10.1097/IAE.0000000000002848.
- Scharf J, Corradetti G, Corvi F, Sadda S, Sarraf D. Optical coherence tomography angiography of the choriocapillaris in age-related macular degeneration. J Clin Med. 2021 10;10(4):751. doi:10.3390/jcm10040751.
- Corvi F, Sadda SVR, Staurenghi G, Pellegrini M. Thresholding strategies to measure vessel density by optical coherence tomography angiography. Can J Ophthalmol. 2020;55. doi:10.1016/j.jcjo.2020.03.011.
- Marchese A, Agarwal A, Moretti AG, et al. Advances in imaging of uveitis. Ther Adv Ophthalmol. 2020;12. doi:10.1177/2515841420917781.
- Agarwal A, Mahajan S, Khairallah M, Mahendradas P, Gupta A, Gupta V. Multimodal imaging in ocular tuberculosis. Ocul Immunol Inflamm. 2017 25;25(1):134–145. doi:10.1080/09273948.2016.1231332.
- Zhang X, Zuo C, Li M, Chen H, Huang S, Wen F. Spectral-domain optical coherence tomographic findings at each stage of punctate inner choroidopathy. Ophthalmology. 2013;120. doi:10.1016/j.ophtha.2013.05.012.
- Nesper PL, Ong JX, Fawzi AA. Exploring the relationship between multilayered choroidal neovascularization and choriocapillaris flow deficits in AMD. Invest Opthalmol Vis Sci. 2021;62(3):12. doi:10.1167/iovs.62.3.12.