ABSTRACT
Purpose
To enumerate the various diagnostic modalities used for keratoconus and their evolution over the past century.
Methods
A comprehensive literature search including articles on diagnosis on keratoconus were searched on PUBMED and summarized in this review.
Results
Initially diagnosed in later stages of the disease process through clinical signs and retinoscopy, the initial introduction of corneal topography devices like Placido disc, photokeratoscopy, keratometry and computer-assisted videokeratography helped in the earlier detection of keratoconus. The evolution of corneal tomography, initially with slit scanning devices and later with Scheimpflug imaging, has vastly improved the accuracy and detection of clinical and sub-clinical disease. Analyzing the alteration in corneal biomechanics further contributed to the earlier detection of keratoconus even before the tomographic changes became evident. Anterior segment optical coherence tomography has proven to be a helpful adjuvant in diagnosing keratoconus, especially with epithelial thickness mapping. Confocal microscopy has helped us understand the alterations at a cellular level in keratoconic corneas.
Conclusion
Thus, the collective contribution of the various investigative modalities have greatly enhanced earlier and accurate detection of keratoconus, thus reducing the disease morbidity
INTRODUCTION
The first comprehensive description of keratoconus was provided by John Nottingham in 1854 when he described a condition called ‘conical cornea’.Citation1 Since then, our understanding of the disease has evolved significantly. Keratoconus has been classically described as a bilateral, progressive, non-inflammatory disorder of the cornea leading to corneal thinning, protrusion, and irregular astigmatism, eventually resulting in impaired quality of vision.Citation2,Citation3 The usual onset of the disease is in adolescence, and it progresses until the third or fourth decade of life.Citation4
Very few articles are available in the literature that focuses on the evolution of the diagnosis of keratoconus. This review aims to provide a comprehensive analysis of the various diagnostic modalities used for keratoconus over the past century and describe their importance in ultimately diagnosing and treating the disease early.
METHODS
A thorough literature search for the components of this review was done using PubMed. The keywords in relation to keratoconus used for the search were “retinoscopy”, “corneal topography”, “pentacam”, “corneal biomechanics”, “ocular coherence tomography” and “confocal microscopy”. The search yielded a total of 3510 articles. The articles were screened, and only those focused on the diagnosis of keratoconus were included in this review.
Moderate to advanced keratoconus can be detected by clinical examination but these clinical signs () usually appear later in the course of the disease. Amongst these, a good retinoscopy can pick up keratoconus much earlier but is subjective.
Table 1. Clinical signs of Keratoconus.
RETINOSCOPY
Retinoscopy, invented by Hugo Wolff,Citation5 is an indispensable component of objective refraction. In keratoconus, a scissor reflex is usually seen on retinoscopy, comprising an “against” motion in the centre and a “with” motion in the periphery.Citation3 This split in the light reflex is called the scissor reflex. Goebels et al.Citation6 found that retinoscopy was comparable to the Pentacam and Ocular Response Analyzer in confirming the diagnosis of keratoconus. Al-Mahrouqi et al. found that the scissoring reflex on retinoscopy is very sensitive for detecting keratoconus and is an economical and easy to use method to screen for keratoconus especially in population-based screening programs.Citation7
CORNEAL TOPOGRAPHY
Helmholtz first introduced the keratometer (ophthalmometer) in 1854 as an instrument that provided information on 2–3 locations on the anterior corneal surface approximately 3 mm apart. The keratometer can detect keratoconus by identifying distortion of the reflected mires, and central or inferior steepening and is a sensitive indicator of keratoconus.Citation3 The limitations of the keratometer include lack of information regarding the topography central or peripheral to the points of measurement and mire distortion even with mild surface irregularity preventing meaningful assessment.Citation8
Marc Amsler, in 1938, used a photographic Placido disk to describe the topographic changes in keratoconus.Citation9 He classified keratoconus based on the degree of deviation of the horizontal axis of the Placido disc and documented the natural history of progression of the disease. Amsler concluded that progression was likely to occur in patients between 10 and 20 years of age, whereas progression was less likely after 30 years.Citation9,Citation10 However, reproducibility is a significant problem with this device due to instrument tilt or poor alignment resulting in incorrect interpretation.Citation11
PlacidoCitation12 and Gullstrand were the first to develop the photokeratoscope that provides topography of 55–80% of the anterior corneal surface, excluding the central 3 mm. The earliest sign detected on photokeratoscopy in keratoconus was compression of mires in the inferotemporal cornea (egg-shaped mires).Citation13
In 1880, Antonio PlacidoCitation14 introduced the principle of Placido ring-based videokeratoscopes, which use concentric rings of light and a digital camera to capture the reflection of the rings on the corneal surface, and the data is then processed using algorithms to illustrate the topography of the anterior corneal surface. These devices incorporate features of both the keratometer and a photokeratoscope. Rabinowitz and co-workers have described various patterns and indices for early detection of keratoconus using videokeratography.Citation15–18 Inferior steepening with asymmetric bowtie with a skewed radial axis (AB/SRAX) pattern was found in nearly 100% of patients with early keratoconus but only 0.5% of the normal population.Citation19 Additionally, it was shown that 50% of eyes with an AB/SRAX pattern in the normal fellow eye of unilateral keratoconus ultimately progress to keratoconus, proving that this pattern could be a marker for forme fruste keratoconus (FFKC).Citation18,Citation20 A few indices, including I-S value, the SRAX index and KISA% index, were also introduced to improve the detection of keratoconus.Citation15,Citation16,Citation18 However, none of these indices has proven to be 100% accurate in differentiating normal corneas from those that had early keratoconus.
CORNEAL TOMOGRAPHY
Corneal tomography provides a three-dimensional representation of the cornea with a detailed analysis of both the anterior and posterior corneal surfaces as well as pachymetric analysis. The evolution of corneal tomography has revolutionized the diagnosis of keratoconus and screening for refractive surgery.
The Orbscan (Bausch & Lomb; Rochester, USA) was the first corneal tomography method introduced in 1995. It uses the innovative principle of slit scanning technology to acquire information from the cornea’s anterior and posterior surfaces. In 1999, the Orbscan IIz (Bausch and Lomb, Rochester, USA) was introduced, which used a combination of Placido rings and slit scanning to assess the tomography and pachymetry. The Placido rings consist of 40 monochromatic concentric rings, and analysis of the reflection of these rings on the corneal surface provides the anterior corneal topography. The slit-scanning system utilizes 40 white light slits at angles of 45°, each measuring 12.5 × 0.3 mm, and gives information regarding the topography of the posterior corneal surface and corneal thickness. Recently, an artificial intelligence system named the Screening Corneal Objective Risk of Ectasia (SCORE) has been linked to the Orbscan IIz. This system uses a combination of 12 tomographic indices, including maximum posterior elevation, vertical decentration of thinnest point, thinnest corneal thickness and the difference between mean central and thinnest corneal thickness. These indices are used to derive a score and categorize the cornea as positive or negative for the risk of ectasia. The SCORE software has been validated in various subsets like FFKC and post LASIK ectasia.Citation21–23
Scheimpflug imaging of the cornea has more recently become the mainstay of corneal tomography. Jules Carpentier first described the Scheimpflug principle in 1901, which was cited and credited in the original patent by Theodor Scheimpflug in 1904. In Scheimpflug imaging, the planes are aligned to provide an extended depth of focus and more sharpness to points of the image with minimal distortion.Citation24
Digital rotating Scheimpflug tomography is an evolution compared to parallelopiped horizontal cross-sectioning for tomographic evaluation of the cornea and anterior segment used in the Orbscan.Citation25 The rotating system in Scheimpflug imaging has a common centre that makes image registration more accurate than horizontal slit scanning.Citation26,Citation27 The Oculus Pentacam (Wetzlar, Germany) was the first system to use a digital rotating Scheimpflug camera for corneal and anterior segment tomography.Citation28 In addition to the rotating Scheimpflug camera, the pentacam has a central frontal camera that controls fixation and compensates for alignment. This high-resolution frontal camera also records pupil measurements of white-to-white diameter and provides orientation for reconstructing a three-dimensional tomography model. In around 2 seconds, a three-dimensional cornea and anterior segment model is generated from as many as 25,000 elevation points in the Pentacam and 138,000 elevations points in the Pentacam HR. The resultant Scheimpflug images provide data from the anterior and posterior surfaces of the cornea, angle, anterior iris and lens. Although the Pentacam provides very accurate tomography data, it has a few limitations. The wavelength of light used, 475 nm, is sensitive to corneal opacities leading to inaccuracies in contour analysis. Another limitation is the inability to visualize the anterior chamber angle directly due to total internal reflection in the peripheral cornea. However, the Pentacam has extrapolation software integrated into the system, accurately estimating the anterior chamber angle.Citation29,Citation30 The other devices that use Scheimpflug photography include Galilei (Port, Switzerland), Sirius (Firenze, Italy), Tomey TMS-5 (Nagoya, Japan), Costruzione Strumenti Oftalmici (CSO; Florence, Italy) and the Preciso (iVIS Technologies, Taranto, Italy). The features of these devices in elaborated in .
Table 2. Comparison of various Scheimpflug-based tomography devices.
The elevationCitation31–33 and pachymetric indices () derived from tomographic maps have been reported to be very effective in detecting early keratoconus.Citation31,Citation32,Citation34–37 The corneal thickness spatial profile and percentage thickness increase graphs, available on Pentacam, have proven important in identifying keratoconus.Citation34,Citation38–45 Pachymetric progression indices (PPI) are assessed for all hemi-meridians across the cornea so that the average (PPI Ave) and the meridian with maximal (PPI Max) pachymetric increase are noted.Citation25 The ratio between the thinnest point and PPI gives the Ambrosio’s relational thickness (ART). The Pentacam HR also provides the Belin-Ambrósio Enhanced Ectasia Display (BAD) (), with data demonstrating nine input parameters; anterior elevation and posterior elevation at the thinnest point, change in anterior and posterior elevation, location of the thinnest point, corneal thickness at the thinnest point, pachymetric progression, ART and Kmax.Citation46 Anterior and posterior elevation data is calculated utilizing a method of enhanced best-fit spheres (BFS), which are generated by excluding an area of 3.5 mm in diameter centred on the thinnest point. The resultant enhanced BFS is flatter than a standard BFS, which has minimal effect in normal corneas but highlights the abnormal areas of an ectatic cornea.Citation47,Citation48 The BAD summarises the analyses in the form of normality indices as provided in . The software highlights values of these indices between 1.6 and 2.6 in yellow (suspect keratoconus) and values >2.6 in red (clinical keratoconus).Citation48 The final D value (BAD-D) is deduced from a regression analysis of all the parameters, and a value of more than 2.1 indicates ectasia. BAD-D has been shown to have the highest accuracy in detecting clinical and subclinical keratoconus.Citation25,Citation49–55
Figure 1. Pentacam maps of a keratoconus patient A) 4 Maps refractive output showing specific tomographic features of keratoconus. B) Belin Ambrosio enhanced ectasia display of the same patient showing all BAD indices including BAD-D in red indicating keratoconus.
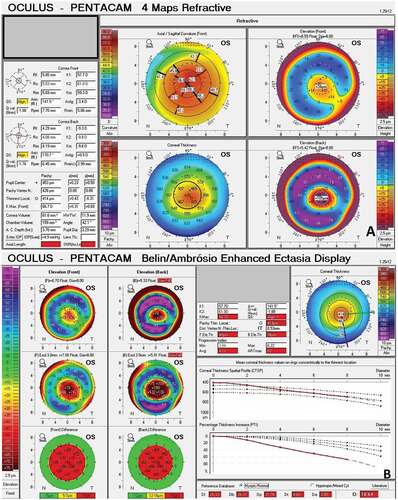
Table 3. Belin/Ambrósio enhanced ectasia display values.
CORNEAL BIOMECHANICS
A decrease in corneal biomechanical strength has been recognized as a critical factor in the pathophysiology of keratoconus.Citation56–58 A combination of factors, including a decrease in the number of collagen lamellae and their altered orientation, reduced cross-links, and alterations in keratocyte density and extracellular matrix, contribute to the decrease in biomechanical resistance of the cornea to permanent deformation.Citation58–60 Investigating these changes in biomechanical properties could help in the earlier diagnosis of keratoconus, even before topographic and tomographic changes become evident.
The Ocular Response Analyzer (ORA, Reichert Ophthalmic Instruments, NY) was the first commercially available instrument to measure the biomechanical properties of the cornea in vivo.Citation61 This instrument is a non-contact tonometer with a metered air puff to applanate the cornea. The infrared reflectance produces two distinct peaks: when the cornea moves inwards (P1) and when the cornea moves outwards (P2). The values for P1 and P2 equate to the emitted air-pulse pressure at the respective applanation events within the 3 mm sampling zone.Citation61
The two main biomechanical outputs from the ORA are corneal hysteresis (CH) and corneal resistance factor (CRF). CH is defined as the difference between pressures at P1 and P2, and CRF is calculated as (P1 – kP2), with a proprietary factor k (0.7) derived by the manufacturer.Citation61 Though these parameters have been noted to be lower in keratoconus than normal corneas,Citation61–67 they lack sensitivity and specificity for diagnosing keratoconus as a significant overlap exists between the two groups. Hence, these parameters cannot be used for the diagnosis of early keratoconus.Citation65–67 However, newer parameters were derived from the ORA waveform to improve the diagnostic accuracy.Citation68–70 Avetisov et al.Citation71 found a statistical difference in a mathematically derived coefficient of elasticity between healthy and keratoconic corneas. Luz et al.Citation72 used logistic regression analysis to produce a combined tomographic and biomechanical linear model, which improved the accuracy of diagnosis of FFKC.
The CorVis ST (CST, Oculus; Wetzlar, Germany) was introduced as an alternative to measuring the biomechanical properties of the cornea in vivo. CST is also a non-contact tonometer like the ORA and emits an air pulse. However, in contrast to ORA, it uses an ultra-high-speed Scheimpflug camera (4330 frames/sec) to record the ocular response to the air pulse across an 8 mm wide horizontal cross-section of the cornea. The data is analyzed in real-time to derive various parameters () that help provide information regarding biomechanical propertiesCitation73 (). Similar to the ORA, though several parameters of CST were significantly different in keratoconic corneas when compared with healthy corneas,Citation73–75 a substantial overlap existed between the two groups.Citation74,Citation75 However, analysis of deformation amplitude and deflection amplitude was found to differentiate suspect and keratoconic eyes from normal eyes.Citation76
Figure 2. Tomographic biomechanical display (TBI) from Pentacam and Corvis ST showing abnormal corneal biomechanics and abnormal CBI, BAD D and TBI values indicative of keratoconus.
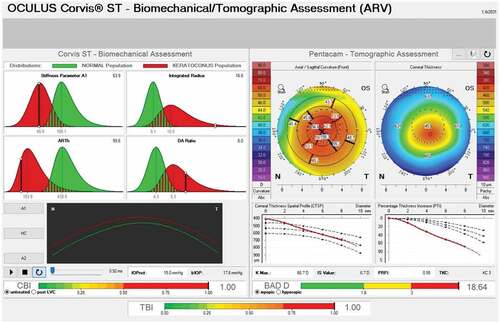
Table 4. Corneal deformation parameters provided by the corvis ST.
Vinciguerra et al.Citation77 combined corneal deformation parameters and horizontal thickness profile to develop the Corvis corneal biomechanical index (CBI), which was highly sensitive and specific to differentiate keratoconic and normal eyes. Ambrósio et al.Citation78 integrated data obtained from Scheimpflug-based corneal tomography and analysis of corneal biomechanical properties using artificial intelligence techniques to develop the tomographic biomechanical index (TBI). TBI has proven better than other investigative parameters, including CBI and BAD-D, in detecting corneal ectasia.Citation78,Citation79 Validation studies have also demonstrated that TBI increases the ability to detect early ectasia even in subclinical casesCitation80–82
Brillouin Spectroscopy is a newer modality for analyzing corneal biomechanics. The principle is based on the interaction of light (laser) and the intrinsic acoustic waves within the tissue. The laser’s interaction with the tissue causes a Doppler frequency shift associated with the elastic modulus,Citation83 thus analyzing the biomechanics of the cornea, sclera and lens.Citation84–87 Scarcelli et al.Citation88 reported ex vivo ectatic corneas having significantly smaller Brillouin frequency shift than normal corneas. It was also found that there was a significant difference between Brillouin frequency shift in the cone region compared to other areas in vivo.Citation83 Further studies have distinguished mild KC from normal and clinical KC by comparing their Brillouin frequency, proving its efficacy and accuracy.Citation89,Citation90
OPTICAL COHERENCE TOMOGRAPHY
Anterior segment optical coherence tomography (AS-OCT) helps assess the microstructure of the cornea in vivo by producing high resolution, cross-sectional images using the principle of optical light scattering in combination with low coherence interferometry.Citation91
AS-OCT has gained importance in epithelial thickness mapping in keratoconus, providing a potential avenue for early diagnosis. Early histological changes in keratoconus include reduced corneal basal epithelial cell density, Bowman’s layer instability and loss of anterior stromal collagen fibrils.Citation92,Citation93 The features that have been demonstrated in epithelial thickness maps on AS-OCT in eyes with keratoconus are apical epithelial thinning, decreased overall epithelial thickness, thicker epithelial layer supero-nasally (in a typical inferotemporal cone), greater differences between the minimum and maximum epithelial thickness and greater variability of the pattern standard deviation and map standard deviation compared with normal eyes.Citation39,Citation94–96 Apical epithelial thinning occurs due to a compensatory remodelling in response to the underlying stromal thinning to reduce anterior surface irregularityCitation94,Citation97 (). This may lead to masking the stromal thinning when the total corneal thickness is measured or cause topographic images to appear relatively normal, especially in early keratoconus.Citation39,Citation98 Therefore, analysis of the epithelium and stroma separately with the AS-OCT could help in improving the accuracy of diagnosis. However, it has been shown that epithelial thickness parameters are insufficient as independent diagnostic tools and should be used in conjunction with corneal tomography to diagnose keratoconus.Citation56,Citation99
Figure 3. AS-OCT of a keratoconic cornea showing compensatory changes in the epithelial thickness (white arrow) as a response to underlying stromal thinning thus resulting in a regular anterior surface.
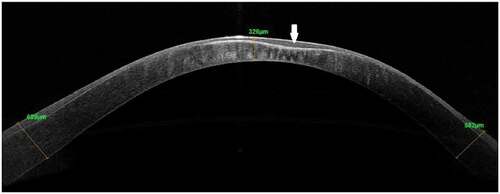
Li et al.Citation94,Citation100 used the OCT to develop an extended epithelial thickness map and various indices to detect early KC. Pahuja et al.Citation101 studied Bowman’s layer irregularity in normal and ectatic corneas using OCT and introduced the Bowman’s roughness index. This index successfully detected keratoconus and, in combination with the BAD-D and epithelial thickness data, improved the sensitivity for the detection of early keratoconus. Hwang et al.Citation102 reported that the combination of parameters from Scheimpflug imaging and OCT improved the accuracy in detecting very asymmetric keratoconus.
Polarization-sensitive optical coherence tomography (PS-OCT) enhances the contrast of fibrous tissues by measuring birefringence.Citation103 It is well known that the arrangement of collagen fibril lamellae is altered in keratoconus,Citation59,Citation104,Citation105 which results in modifications in their birefringence. Based on this principle, it was shown that phase retardation of the posterior corneal surface was increased in ectatic corneas due to an increase in birefringence.Citation106 It was also shown that phase retardation was sensitive in discriminating keratoconus suspects and can help diagnose early or subclinical keratoconus.Citation106
AS-OCT can also be used for detecting risk factors for developing acute hydrops in keratoconus. Fuentes et al.Citation107 reported features on AS-OCT such as increased epithelial thickness, a higher epithelial to stromal thickness ratio, stromal thinning at the corneal apex and hyper-reflectivity of Bowman’s layer are associated with an increased risk of developing hydrops. The authors also hypothesized that corneal scarring might increase corneal rigidity, thus protecting against developing hydrops. In cases of corneal hydrops, AS-OCT can help assess and classify the extent and edges of Descemet’s membrane (DM) detachment. Basu et al.Citation108 reported that eyes with deeper DM detachments and larger DM breaks required more time to resolve corneal oedema.
CONFOCAL MICROSCOPY
In vivo confocal microscopy (IVCM) allows imaging of the cornea at a cellular level. The features of keratoconus on IVCM include decreased keratocyte density in the anterior and posterior stroma,Citation60,Citation109–112 decreased basal epithelial cell and endothelium densities along with increased pleomorphism and polymegethism,Citation109 significantly lower nerve densityCitation113 and alteration in the arrangement of the subbasal nerve plexus.Citation114 Interestingly, keratoconus patients who use contact lenses have significantly lower anterior keratocyte density than those who do not.Citation60,Citation111,Citation112
WAVEFRONT ANALYSIS
In keratoconus, irregular astigmatism increases lower and higher-order aberrations.Citation115 Many authors have shown that corneal and total wavefront ocular aberrations are higher in ectatic corneas when compared to healthy ones.Citation115,Citation116 Higher-order aberrations, especially vertical coma and trefoil, have been reported to be significantly higher in mild keratoconus than in normal eyes.Citation115,Citation117–120 and can potentially be used for the early detection of the disease.
MACHINE LEARNING
Machine learning refers to an artificial intelligence technique where a dataset of known inputs is entered into a machine programmed to maximize output accuracy.Citation121 Over the past two decades, multiple machine learning methods have been employed to diagnose keratoconus with varying parameters to improve detection accuracy.Citation43,Citation97,Citation120,Citation122–133 Recently, Lopes et al.Citation133 reported the most extensive machine learning study to detect clinical ectasia and ectasia susceptibility. The authors introduced a new Pentacam random forest index (PRFI), proven to be statistically superior to BAD-D when assessing all ectasia cases. The advantage of machine learning methods is that many refractive indices can be evaluated at once, improving the diagnostic accuracy and decreasing the dependence of subjective operators. However, lack of standardization across the data subsets provides the most significant challenge in drawing comparative conclusions currently but has excellent potential for the future.
SUMMARY
The increasing popularity of laser refractive surgery over the past two decades has accelerated the need for accurate detection of subclinical keratoconus. The constant evolution and distinctive contribution of the diagnostic modalities in keratoconus have vastly improved the detection and aided in the timely treatment of the disease. Corneal tomography is still the most widely used tool for detecting keratoconus. However, the increasing importance of the other modalities like corneal biomechanics assessment and anterior segment optical coherence tomography has proven that a multi-pronged approach can help in increasing the diagnostic accuracy. Analysis of multiple parameters with machine learning will significantly enhance the speed and accuracy of detection and decrease subjective dependence.
DISCLOSURE STATEMENT
No potential conflict of interest was reported by the authors.
Additional information
Funding
REFERENCES
- Gokul A, Patel DV, McGhee CNJ. Dr John Nottingham’s 1854 landmark treatise on conical cornea considered in the context of the current knowledge of keratoconus. Cornea. 2016 May;35(5):673–678.
- Rabinowitz YS. Keratoconus. Surv Ophthalmol. 1998 Jan;42(4):297–319.
- Krachmer JH, Feder RS, Belin MW. Keratoconus and related non-inflammatory corneal thinning disorders. Surv Ophthalmol. 1984 Jan;28(4):293–322.
- Davidson AE, Hayes S, Hardcastle AJ, Tuft SJ. The pathogenesis of keratoconus. Eye. 2014 Feb 20;28(2):189–195.
- RUNDLES WZ. Streak Retinoscopy. Arch Ophthalmol. 1939 May 1;21(5):833–843.
- Goebels S, Käsmann-Kellner B, Eppig T, Seitz B, Langenbucher A. Can retinoscopy keep up in keratoconus diagnosis? Contact Lens Anterior Eye. 2015 Aug;38(4):234–239.
- Al-Mahrouqi H, Oraba SB, Al-Habsi S, et al. Retinoscopy as a screening tool for keratoconus. Cornea. Apr 9 2019;38(4):442–445.
- Wilson SE, Klyce SD. Advances in the analysis of corneal topography. Surv Ophthalmol. 1991 Jan;35(4):269–277.
- Amsler M. Le kératocône fruste au Javal. Ophthalmologica. 1938;96(2):77–83.
- Amsler M. Kératocône classique et kératocône fruste; arguments unitaires. Ophthalmologica. 1946;111(2–3):96–101.
- Levene JR. An evaluation of the hand keratoscope as a diagnostic instrument for corneal astigmatism. II. Br J Physiol Opt. 1962;19.
- Levene JR. The true inventors of the keratoscope and photo-keratoscope. Br J Hist Sci. 1965;2(8):324–342.
- Rowsey JJ. Corneal Topography. Arch Ophthalmol. 1981 Jun 1;99(6):1093.
- Brody J, Waller S, Wagoner M. Corneal Topography: history, technique, and clinical uses. Int Ophthalmol Clin. 1994;34(3):197–207.
- Rabinowitz YS. Videokeratographic indices to aid in screening for keratoconus. J Refract Surg. 1995;11(5):371–406.
- Rabinowitz YS, Rasheed K. KISA% index: a quantitative videokeratography algorithm embodying minimal topographic criteria for diagnosing keratoconus. J Cataract Refract Surg. 1999 Oct;25(10):1327–1335.
- Rabinowitz YS, Garbus J, McDonnell PJ. Computer-assisted corneal topography in family members of patients with keratoconus. Arch Ophthalmol. 1990 Mar 1;108(3):365.
- Li X, Rabinowitz YS, Rasheed K, Yang H. Longitudinal study of the normal eyes in unilateral keratoconus patients. Ophthalmology. 2004 Mar;111(3):440–446.
- Rabinowitz YS, Yang H, Brickman Y, et al. Videokeratography database of normal human corneas. British J Ophthalmol. Jul 1 1996;80(7):610–616.
- Li X, Yang H, Rabinowitz YS. Keratoconus: classification scheme based on videokeratography and clinical signs. J Cataract Refract Surg. 2009 Sep;35(9):1597–1603.
- Saad A, Gatinel D. Validation of a new scoring system for the detection of early form of keratoconus. Int J Keratoconus Ectatic Corneal Dis. 2012 Aug;1(2):100–108.
- Chan C, Ang M, Saad A, et al. Validation of an objective scoring system for forme fruste keratoconus detection and post-LASIK ectasia risk assessment in Asian eyes. Cornea. Sep 2015;34(9):996–1004.
- Chan C, Saad A, Randleman BJ, et al. Analysis of cases and accuracy of 3 risk scoring systems in predicting ectasia after laser in situ keratomileusis. J Cataract Refract Surg. Aug 2018;44(8):979–992.
- Wegener A, Laser-Junga H. Photography of the anterior eye segment according to Scheimpflug’s principle: options and limitations - a review. Clin Exp Ophthalmol. 2009 Jan;37(1):144–154.
- Ambrósio R, Valbon BF, Faria-Correia F, Ramos I, Luz A. Scheimpflug imaging for laser refractive surgery. Curr Opin Ophthalmol. 2013 Jul;24(4):310–320.
- Belin MW, Khachikian SS, McGhee CNJ, Patel D. New technology in corneal imaging. Int Ophthalmol Clin. 2010;50(3):177–189.
- Belin MW, Khachikian SS. New devices and clinical implications for measuring corneal thickness. Clin Exp Ophthalmol. 2006 Nov;34(8):729–731.
- Wilson SE, Ambrosio R. Computerized corneal topography and its importance to wavefront technology. Cornea. 2001 Jul;20(5):441–454.
- Kurita N, Mayama C, Tomidokoro A, Aihara M, Araie M. Potential of the pentacam in screening for primary angle closure and primary angle closure suspect. J Glaucoma. 2009 Sep;18(7):506–512.
- Aptel F, Chiquet C, Beccat S, Denis P. Biometric evaluation of anterior chamber changes after physiologic pupil dilation using pentacam and anterior segment optical coherence tomography. Invest Opthalmol Visual Sci. 2012 Jun 25;53(7):4005.
- Ambrósio R, Nogueira LP, Caldas DL, et al. Evaluation of corneal shape and biomechanics before LASIK. Int Ophthalmol Clin. 2011;51(2):11–38.
- Faria-Correia F, Ramos I, Valbon B, Luz A, Roberts CJ, Ambrósio R. Scheimpflug-based tomography and biomechanical assessment in pressure-induced stromal keratopathy. J Refract Surg. 2013 May;29(5):356–358.
- Henriquez MA, Izquierdo L, Mannis MJ. Inter-eye asymmetry detected by Scheimpflug imaging in subjects with normal corneas and keratoconus. Cornea. 2013 Jun;32(6):779–782.
- Luz A, Ursulio M, Castañeda D, Ambrósio R Jr. Corneal thickness progression from the thinnest point to the limbus: a study based on a normal and a keratoconus population to create reference values. Arq Bras Oftalmol. 2006 Aug;69(4):579–583.
- Ambrósio R, Caiado ALC, Guerra FP, et al. Novel pachymetric parameters based on corneal tomography for diagnosing keratoconus. J Refract Surg. 2011 Oct;27(10):753–758.
- Ambrósio R, Alonso RS, Luz A, Coca Velarde LG. Corneal-thickness spatial profile and corneal-volume distribution: tomographic indices to detect keratoconus. J Cataract Refract Surg. 2006 Nov;32(11):1851–1859.
- Pflugfelder SC, Liu Z, Feuer W, Verm A. Corneal thickness indices discriminate between keratoconus and contact lens-induced corneal thinning. Ophthalmology. 2002 Dec;109(12):2336–2341.
- R A Jr. Percentage thickness increase and absolute difference from thinnest to describe thickness profile. J Refract Surg. 2010;26(2):84–86.
- Reinstein DZ, Gobbe M, Archer TJ, Silverman RH, Coleman DJ. Epithelial, stromal, and total corneal thickness in keratoconus: three-dimensional display with Artemis very-high frequency digital ultrasound. J Refract Surg. 2010 Jan;26(4)
- Bühren J, Kook D, Yoon G, Kohnen T. Detection of subclinical keratoconus by using corneal anterior and posterior surface aberrations and thickness spatial profiles. Invest Opthalmol Visual Sci. 2010 Jul 1;51(7):3424.
- Li Y, Meisler DM, Tang M, et al. Keratoconus diagnosis with optical coherence tomography pachymetry mapping. Ophthalmology. Dec 2008;115(12):2159–2166.
- Reinstein DZ, Archer TJ, Gobbe M. Corneal epithelial thickness profile in the diagnosis of keratoconus. J Refract Surg. 2009;25:604–610.
- Saad A, Gatinel D. Topographic and tomographic properties of forme fruste keratoconus corneas. Invest Opthalmol Visual Sci. 2010 Nov 1;51(11):5546.
- Rabinowitz YS, Rasheed K, Yang H, Elashoff J. Accuracy of ultrasonic pachymetry and videokeratography in detecting keratoconus. J Cataract Refract Surg. 1998 Feb;24(2):196–201.
- Uçakhan ÖÖ, Özkan M, Kanpolat A. Corneal thickness measurements in normal and keratoconic eyes: pentacam comprehensive eye scanner versus non-contact specular microscopy and ultrasound pachymetry. J Cataract Refract Surg. 2006 Jun;32(6):970–977.
- Belin M, Ambrósio R. Scheimpflug imaging for keratoconus and ectatic disease. Indian J Ophthalmol. 2013;61(8):401.
- Belin MW, Khachikian SS. An introduction to understanding elevation-based topography: how elevation data are displayed - a review. Clin Exp Ophthalmol. 2009 Jan;37(1):14–29.
- Lopes BT, Ramos IC, Dawson DG, Belin MW, Ambrósio R. Detection of ectatic corneal diseases based on pentacam. Z Fur Med Phys. 2016 Jun;26(2):136–142.
- Ambrósio R, Faria-Correia F, Ramos I, et al. Enhanced screening for ectasia susceptibility among refractive candidates: the role of corneal tomography and biomechanics. Curr Ophthalmol Rep. 2013 Mar 17;1(1):28–38.
- Chan TC, Wang YM, Yu M, Jhanji V. Comparison of corneal dynamic parameters and tomographic measurements using Scheimpflug imaging in keratoconus. British J Ophthalmol. 2018 Jan;102(1):42–47.
- Hashemi H, Khabazkhoob M, Pakzad R, et al. Pentacam accuracy in discriminating keratoconus from normal corneas: a diagnostic evaluation study. Eye Contact Lens. 2019 Jan;45(1):46–50.
- Hashemi H, Beiranvand A, Yekta A, Maleki A, Yazdani N, Khabazkhoob M. Pentacam top indices for diagnosing subclinical and definite keratoconus. J Curr Ophthalmol. 2016 Mar;28(1):21–26.
- Sedaghat M-R, Momeni-Moghaddam H, Ambrósio R, et al. Diagnostic ability of corneal shape and biomechanical parameters for detecting frank keratoconus. Cornea. Aug 25 2018;37(8):1025–1034.
- Ruiseñor Vázquez PR, Galletti JD, Minguez N, et al. Pentacam Scheimpflug tomography findings in topographically normal patients and subclinical keratoconus cases. Am J Ophthalmol. Jul 2014;158(1):32–40.e2.
- Steinberg J, Aubke‐Schultz S, Frings A, et al. Correlation of the KISA % index and Scheimpflug tomography in ‘normal’, ‘subclinical’, ‘keratoconus-suspect’ and ‘clinically manifest’ keratoconus eyes. Acta Ophthalmol. May 14 2015;93(3):e199–e207.
- Gomes JAP, Tan D, Rapuano CJ, et al. Global consensus on keratoconus and ectatic diseases. Cornea. Apr 2015;34(4):359–369.
- Roberts CJ, Dupps WJ. Biomechanics of corneal ectasia and biomechanical treatments. J Cataract Refract Surg. 2014 Jun;40(6):991–998.
- Vellara HR, Patel DV. Biomechanical properties of the keratoconic cornea: a review. Clin Exp Optometry. 2015 Jan 1;98(1):31–38.
- Daxer A, Fratzl P. Collagen fibril orientation in the human corneal stroma and its implication in keratoconus. Invest Ophthalmol Vis Sci. 1997;38:121–129.
- JYF K, Niederer RL, Patel DV, Sherwin T, McGhee CNJ. Laser scanning in vivo confocal analysis of keratocyte density in keratoconus. Ophthalmology. 2008 May;115(5):845–850.
- Luce DA. Determining in vivo biomechanical properties of the cornea with an ocular response analyzer. J Cataract Refract Surg. 2005 Jan;31(1):156–162.
- Ortiz D, Piñero D, Shabayek MH, Arnalich-Montiel F, Alió JL. Corneal biomechanical properties in normal, post-laser in situ keratomileusis, and keratoconic eyes. J Cataract Refract Surg. 2007 Aug;33(8):1371–1375.
- Shah S, Laiquzzaman M, Bhojwani R, Mantry S, Cunliffe I. Assessment of the biomechanical properties of the cornea with the ocular response analyzer in normal and keratoconic eyes. Invest Opthalmol Visual Sci. 2007 Jul 1;48(7):3026.
- Kirwan C, O’Malley D, O’Keefe M. Corneal hysteresis and corneal resistance factor in keratoectasia: findings using the Reichert ocular response analyzer. Ophthalmologica. 2008;222(5):334–337.
- Fontes BM, Ambrósio R, Jardim D, Velarde GC, Nosé W. Corneal biomechanical metrics and anterior segment parameters in mild keratoconus. Ophthalmology. Apr, 2010;117(4):673–679.
- Saad A, Lteif Y, Azan E, Gatinel D. Biomechanical properties of keratoconus suspect eyes. Invest Opthalmol Visual Sci. 2010 Jun 1;51(6):2912.
- Fontes BM, Ambrósio R, Velarde GC, Nosé W. Ocular response analyzer measurements in keratoconus with normal central corneal thickness compared with matched normal control eyes. J Refract Surg. 2011 Mar;27(3)
- Luz A, Lopes B, Hallahan KM, et al. Discriminant value of custom ocular response analyzer waveform derivatives in forme fruste keratoconus. Am J Ophthalmol. Apr 2016;164:14–21. doi:10.1016/j.ajo.2015.12.020.
- Luz A, Fontes BM, Lopes B, Ramos I, Schor P, Ambrósio R Jr. ORA waveform-derived biomechanical parameters to distinguish normal from keratoconic eyes. Arq Bras Oftalmol. 2013 Apr;76(2):111–117.
- Hallahan KM, Sinha Roy A, Ambrosio R, Salomao M, Dupps WJ. Discriminant value of custom ocular response analyzer waveform derivatives in keratoconus. Ophthalmology. 2014 Feb;121(2):459–468.
- Avetisov SE, Novikov IA, Bubnova IA, Antonov AA, Siplivyi VI. Determination of corneal elasticity coefficient using the ORA database. J Refract Surg. 2010 Jul;26(7):520–524.
- Luz A, Lopes B, Hallahan KM, et al. Enhanced combined tomography and biomechanics data for distinguishing forme fruste keratoconus. J Refract Surg. 2016 Jul;32(7):479–494.
- Ambrósio R Jr, Ramos I, Luz A, et al. Dynamic ultra-high speed Scheimpflug imaging for assessing corneal biomechanical properties. Rev Bras Oftalmol. 2013 Apr;72(2):99–102.
- Tian L, Huang YF, Wang LQ, et al. Corneal biomechanical assessment using corneal visualization Scheimpflug technology in keratoconic and normal eyes. J Ophthalmol. 2014;2014:147516. doi:10.1155/2014/147516.
- Ali NQ, Patel DV, McGhee CNJ. Biomechanical responses of healthy and keratoconic corneas measured using a noncontact Scheimpflug-based tonometer. Invest Opthalmol Visual Sci. 2014 Jun 13;55(6):3651.
- Francis M, Pahuja N, Shroff R, et al. Waveform analysis of deformation amplitude and deflection amplitude in normal, suspect, and keratoconic eyes. J Cataract Refract Surg. Oct 2017;43(10):1271–1280.
- Vinciguerra R, Ambrósio R, Elsheikh A, et al. Detection of keratoconus with a new biomechanical index. J Refract Surg. 2016 Dec;32(12):803–810.
- Ambrósio R, Lopes BT, Faria-Correia F, et al. Integration of Scheimpflug-based corneal tomography and biomechanical assessments for enhancing ectasia detection. J Refract Surg. 2017 Jul;33(7):434–443.
- Salomão MQ, Hofling-Lima AL, Gomes Esporcatte LP, et al. The role of corneal biomechanics for the evaluation of ectasia patients. Int J Environ Res Public Health. Mar 23 2020;17(6):2113.
- Kataria P, Padmanabhan P, Gopalakrishnan A, Padmanaban V, Mahadik S, Ambrósio R. Accuracy of Scheimpflug-derived corneal biomechanical and tomographic indices for detecting subclinical and mild keratectasia in a South Asian population. J Cataract Refract Surg. 2019 Mar;45(3):328–336.
- Sedaghat M-R, Momeni-Moghaddam H, Ambrósio R, et al. Long-term evaluation of corneal biomechanical properties after corneal cross-linking for keratoconus: a 4-year longitudinal study. J Refract Surg. 2018 Dec;34(12):849–856.
- Ferreira-Mendes J, Lopes BT, Faria-Correia F, Salomão MQ, Rodrigues-Barros S, Ambrósio R. Enhanced ectasia detection using corneal tomography and biomechanics. Am J Ophthalmol. Jan 2019;197:7–16. doi:10.1016/j.ajo.2018.08.054.
- Seiler TG, Shao P, Eltony A, Seiler T, Yun S-H. Brillouin spectroscopy of normal and keratoconus corneas. Am J Ophthalmol. Jun 2019;202:118–125. doi:10.1016/j.ajo.2019.02.010.
- Shao P, Seiler TG, Eltony AM, et al. Effects of corneal hydration on brillouin microscopy in vivo. Invest Opthalmol Visual Sci. Jun 11 2018;59(7):3020.
- Scarcelli G, Pineda R, Yun SH. Brillouin optical microscopy for corneal biomechanics. Invest Opthalmol Visual Sci. 2012 Jan 20;53(1):185.
- Besner S, Scarcelli G, Pineda R, Yun S-H. In vivo brillouin analysis of the ageing crystalline lens. Invest Opthalmol Visual Sci. 2016 Oct 3;57(13):5093.
- Kwok SJJ, Kim M, Lin HH, et al. Flexible optical waveguides for uniform periscleral cross-linking. Invest Opthalmol Visual Sci. May 11 2017;58(5):2596.
- Scarcelli G, Besner S, Pineda R, Yun SH. Biomechanical characterization of keratoconus corneas ex vivo with brillouin microscopy. Invest Opthalmol Visual Sci. 2014 Jul 23;55(7):4490.
- Naderan M, Rajabi MT, Zarrinbakhsh P. Inter-eye asymmetry in bilateral keratoconus, keratoconus suspect and normal eyes and its relationship with disease severity. British J Ophthalmol. Nov, 2017;101(11):1475–1482.
- Shao P, Eltony AM, Seiler TG, et al. Spatially resolved brillouin spectroscopy reveals biomechanical abnormalities in mild to advanced keratoconus in vivo. Sci Rep. 2019 Dec 16;9(1). doi:10.1038/s41598-019-43811-5
- Huang D, Swanson E, Lin C, et al. Optical coherence tomography. Science. Nov 22 1991;254(5035):1178–1181.
- Somodi S, Hahnel C, Slowik C, Richter A, Weiss DG, Guthoff R. Confocal in vivo microscopy and confocal laser-scanning fluorescence microscopy in keratoconus. Ger J Ophthalmol. 1996 Nov;5(6)
- Sawaguchi S. Three-dimensional scanning electron microscopic study of keratoconus corneas. Arch Ophthalmol. 1998 Jan 1;116(1):62.
- Li Y, Tan O, Brass R, Weiss JL, Huang D. Corneal epithelial thickness mapping by Fourier-domain optical coherence tomography in normal and keratoconic eyes. Ophthalmology. 2012 Dec;119(12):2425–2433.
- Kanellopoulos J, Asimellis G. OCT corneal epithelial topographic asymmetry as a sensitive diagnostic tool for early and advancing keratoconus. Clin Ophthalmol. Nov 2014;8:2277–2287. doi:10.2147/OPTH.S67902.
- Haque S, Jones L, Simpson T. Thickness mapping of the cornea and epithelium using optical coherence tomography. Optometry Vision Sci. Oct, 2008;85(10):E963–E976.
- Silverman RH, Urs R, RoyChoudhury A, Archer TJ, Gobbe M, Reinstein DZ. Epithelial remodeling as basis for machine-based identification of keratoconus. Invest Opthalmol Visual Sci. 2014 Mar 13;55(3):1580.
- Rocha KM, Perez-Straziota CE, Stulting RD, Randleman JB. Epithelial and stromal remodeling after corneal collagen cross-linking evaluated by spectral-domain OCT. J Refract Surg. 2014 Feb;30(2):122–127.
- Temstet C, Sandali O, Bouheraoua N, et al. Corneal epithelial thickness mapping using Fourier-domain optical coherence tomography for detection of form fruste keratoconus. J Cataract Refract Surg. Apr 2015;41(4):812–820.
- Li Y, Chamberlain W, Tan O, Brass R, Weiss JL, Huang D. Subclinical keratoconus detection by pattern analysis of corneal and epithelial thickness maps with optical coherence tomography. J Cataract Refract Surg. 2016 Feb;42(2):284–295.
- Pahuja N, Shroff R, Pahanpate P, et al. Application of high-resolution OCT to evaluate irregularity of Bowman’s layer in asymmetric keratoconus. J Biophotonics. May 2017;10(5):701–707.
- Hwang ES, Perez-Straziota CE, Kim SW, Santhiago MR, Randleman JB. Distinguishing highly asymmetric keratoconus eyes using combined Scheimpflug and spectral-domain OCT analysis. Ophthalmology. 2018 Dec;125(12):1862–1871.
- de Boer JF, Milner TE. Review of polarization-sensitive optical coherence tomography and stokes vector determination. J Biomed Opt. 2002;7(3):359.
- Gotzinger E, Pircher M, Dejaco-Ruhswurm I, Kaminski S, Skorpik C, Hitzenberger CK. Imaging of birefringent properties of keratoconus corneas by polarization-sensitive optical coherence tomography. Invest Opthalmol Visual Sci. 2007 Aug 1;48(8):3551.
- Meek KM, Tuft SJ, Huang Y, et al. Changes in collagen orientation and distribution in keratoconus corneas. Invest Opthalmol Visual Sci. Jun 1 2005;46(6):1948.
- Fukuda S, Yamanari M, Lim Y, et al. Keratoconus diagnosis using anterior segment polarization-sensitive optical coherence tomography. Invest Opthalmol Visual Sci. Feb 19 2013;54(2):1384.
- Fuentes E, Sandali O, El Sanharawi M, et al. Anatomic predictive factors of acute corneal hydrops in keratoconus. Ophthalmology. 2015 Aug;122(8):1653.
- Basu S, Vaddavalli PK, Vemuganti GK, Hasnat Ali M, Murthy SI. Anterior segment optical coherence tomography features of acute corneal hydrops. Cornea. 2012 May;31(5):479–485.
- Uçakhan ÖÖ, Kanpolat A, Ylmaz N, Özkan M. In vivo confocal microscopy findings in keratoconus. Eye Contact Lens. 2006 Jul;32(4):183–191.
- Niederer RL, Perumal D, Sherwin T, McGhee CNJ. Laser scanning in vivo confocal microscopy reveals reduced innervation and reduction in cell density in all layers of the keratoconic cornea. Invest Opthalmol Visual Sci. 2008 Jul 1;49(7):2964.
- Bitirgen G, Ozkagnici A, Malik RA, Oltulu R. Evaluation of contact lens-induced changes in keratoconic corneas using in vivo confocal microscopy. Invest Opthalmol Visual Sci. 2013 Aug 9;54(8):5385.
- Yeniad B, Yilmaz S, Bilgin LK. Evaluation of the microstructure of cornea by in vivo confocal microscopy in contact lens wearing and non-contact lens wearing keratoconus patients. Contact Lens Anterior Eye. 2010 Aug;33(4):167–170.
- Patel DV, Ku JYF, Johnson R, McGhee CNJ. Laser scanning in vivo confocal microscopy and quantitative aesthesiometry reveal decreased corneal innervation and sensation in keratoconus. Eye. 2009 Mar 14;23(3):586–592.
- Patel DV, McGhee CNJ. Mapping the corneal sub-basal nerve plexus in keratoconus by in vivo laser scanning confocal microscopy. Invest Opthalmol Visual Sci. Apr 1 2006;47(4):1348–1351.
- Jafri B, Li X, Yang H, Rabinowitz YS. Higher-order wavefront aberrations and topography in early and suspected keratoconus. J Refract Surg. 2007 Oct;23(8):774–781.
- Schlegel Z, Lteif Y, Bains HS, Gatinel D. Total, corneal, and internal ocular optical aberrations in patients with keratoconus.J Refract Surg. 2009 Jan;25(10). doi: 10.3928/1081597X-20090915-10
- Naderan M, Jahanrad A, Farjadnia M. Ocular, corneal, and internal aberrations in eyes with keratoconus, forme fruste keratoconus, and healthy eyes. Int Ophthalmol. 2018 Aug 24;38(4):1565–1573.
- Colak HN, Kantarci FA, Yildirim A, et al. Comparison of corneal topographic measurements and high order aberrations in keratoconus and normal eyes. Contact Lens Anterior Eye. Oct 2016;39(5):380–384.
- Ortiz-Toquero S, Fernandez I, Martin R. Classification of keratoconus based on anterior corneal high-order aberrations: a cross-validation study. Optometry Vision Sci. 2020 Mar;97(3):169–177.
- Saad A, Gatinel D. Evaluation of total and corneal wavefront high order aberrations for the detection of forme fruste keratoconus. Invest Opthalmol Visual Sci. 2012 May 17;53(6):2978.
- Lin SR, Ladas JG, Bahadur GG, Al-Hashimi S, Pineda R. A review of machine learning techniques for keratoconus detection and refractive surgery screening. Semin Ophthalmol. 2019 May 19;34(4):317–326.
- Maeda N, Klyce SD, Smolek MK. Neural network classification of corneal topography Preliminary demonstration. Invest Ophthalmol Vis Sci. 1995 Jun;36(7)
- Smolek MK, Klyce SD. Current keratoconus detection methods compared with a neural network approach. Invest Ophthalmol Vis Sci. Oct, 1997;38(11):2290–2299.
- Smolek MK, Klyce SD. Screening of prior refractive surgery by a wavelet-based neural network. J Cataract Refract Surg. 2001 Dec;27(12):1926–1931.
- Accardo PA, Pensiero S. Neural network-based system for early keratoconus detection from corneal topography. J Biomed Inform. 2002 Jun;35(3):151–159.
- de Carvalho LAV, Barbosa MS. Neural networks and statistical analysis for classification of corneal videokeratography maps based on Zernike coefficients: a quantitative comparison. Arq Bras Oftalmol. 2008 Jun;71(3):337–341.
- Souza MB, Medeiros FW, Souza DB, Garcia R, Alves MR. Evaluation of machine learning classifiers in keratoconus detection from Orbscan II examinations. Clinics (Sao Paulo). 2010;65(12):1223–1228.
- Smadja D, Touboul D, Cohen A, et al. Detection of subclinical keratoconus using an automated decision tree classification. Am J Ophthalmol. Aug 2013;156(2):237–246.e1.
- Ruiz Hidalgo I, Rodriguez P, Rozema JJ, et al. Evaluation of a machine-learning classifier for keratoconus detection based on Scheimpflug tomography. Cornea. Jun 2016;35(6):827–832.
- Kovács I, Miháltz K, Kránitz K, et al. Accuracy of machine learning classifiers using bilateral data from a Scheimpflug camera for identifying eyes with preclinical signs of keratoconus. J Cataract Refract Surg. Feb 2016;42(2):275–283.
- Saad A, Gatinel D. Combining placido and corneal wavefront data for the detection of forme fruste keratoconus. J Refract Surg. 2016 Aug;32(8):510–516.
- Ruiz Hidalgo I, Rozema JJ, Saad A, et al. Validation of an objective keratoconus detection system implemented in a Scheimpflug tomographer and comparison with other methods. Cornea. Jun 31 2017;36(6):689–695.
- Lopes BT, Ramos IC, Salomão MQ, et al. Enhanced tomographic assessment to detect corneal ectasia based on artificial intelligence. Am J Ophthalmol. 2018:195:223–232. https://doi.org/10.1016/j.ajo.2018.08.005