Abstract
The ongoing COVID-19 pandemic caused by severe acute respiratory syndrome coronavirus 2 (SARS-CoV-2) has become a global threat. Despite strict control measures implemented worldwide and immunization using novel vaccines, the pandemic continues to rage due to emergence of several variants of SARS-CoV-2 with increased transmission and immune escape. The rapid spread of variants of concern (VOC) in the recent past has created a massive challenge for the control of COVID-19 pandemic via the currently used vaccines. Vaccines that are safe and effective against the current and future variants of SARS-CoV-2 are essential in controlling the COVID-19 pandemic. Rapid production and massive rollout of next-generation vaccines against the variants are key steps to control the COVID-19 pandemic and to help us return to normality. Coordinated surveillance of SARS-CoV-2, rapid redesign of new vaccines and extensive vaccination are needed to counter the current SARS-CoV-2 variants and prevent the emergence of new variants. In this article, we review the latest information on the VOCs and variants of interest (VOIs) and present the information on the clinical trials that are underway on evaluating the effectiveness of COVID-19 vaccines on VOCs. We also discuss the current challenges posed by the VOCs in controlling the COVID-19 pandemic and future strategies to overcome the threat posed by the highly virulent and rapidly transmissible variants of SARS-CoV2.
PLAIN LANGUAGE SUMMARY
The COVID-19 is a contagious respiratory disease caused by a virus known as severe acute respiratory syndrome coronavirus 2 (SARS-CoV-2) that emerged in 2019. The COVID-19 has now spread to all part of the world and has become a global threat. Even after the strict control measures and immunization programs to prevent the disease, COVID-19 is still causing destruction due to appearance of new strains of SARS-CoV-2 that transmit faster and capable of escaping the immunity. The faster spread of the new strains of viruses that cause more severe disease is the biggest challenge to control the COVID-19 pandemic by using the presently available vaccines. To control the COVID-19 pandemic we urgently need safe and effective vaccines against the corona viral variants. This can be achieved by tracking the appearance of new viral types, design and rapid production and supply of new vaccines against the virus. This article presents the latest information on the new types of SARS-CoV-2, and on the status of vaccine trials and their effectiveness against these viruses. Similarly, the information on the challenges posed by the new viral strains in controlling the COVID-19 and future strategies to overcome the threat posed by corona viruses is also provided.
1. Introduction
Since the end of 2019, the coronavirus disease 2019 (COVID-19) caused by a new type of virus called Severe Acute Respiratory Syndrome Coronavirus-2 (SARS-CoV-2) has been sweeping the world. Globally, as of May 19, 2022, there have been 520,912,257 confirmed cases of COVID-19, including 6,272,408 deaths, reported to the World Health Organization (WHO). To fight against the COVID-19, scientists around the world have tried their best to develop specific vaccines for COVID-19. According to the COVID-19 landscape of novel coronavirus candidate vaccines released by WHO, as of May 17, 2022, there are 156 vaccines in clinical development and 198 vaccines in pre-clinical development (https://www.who.int/publications/m/item/draft-landscape-of-covid-19-candidate-vaccines). Currently, more than ten COVID-19 vaccines have been authorized under an emergency use authorization (EUA), such as four vaccines from China (Sinovac vaccine, Sinopharm’s vaccine, CanSino’ Ad5-nCoV vaccine, and ZF2001), three vaccines produced in the United States (Janssen’s Ad26.COV2.S, Pfizer-BioNTech’s BNT162b2 and Moderna mRNA-1273), two vaccines from Russia (Sputnik V vaccine and EpiVacCorona vaccine), one vaccine from United Kingdom (AstraZeneca’s ChAdOx1 nCoV-19), one vaccine from Germany (CureVac), and one vaccine from India (BBV152) [Citation1–4]. As of May 16, 2022, a total of 11,713,606,779 vaccine doses have been administered.
What is worrying is that although the vaccination rate is increasing substantially, there is no obvious turning point in the incidence of COVID-19. The number of confirmed cases in the global situation () and WHO regions () has increased significantly after October 11, 2021. The high incidence globally makes the SARS-CoV-2 viral load in populations bigger, thus increasing the risk of novel mutations. It has been reported that several variants have increased the transmission and infectivity, and decreased the recognition by host antibodies, such as variant B.1.1.7 (Alpha) in United Kingdom, variant B.1.351 (Beta) in South Africa, variant P.1 (Gamma) in Brazil, variant B.1.617.2 (Delta) in India, and variant B.1.1.529 (Omicron) in South Africa [Citation2,Citation5–8]. This review will track the latest variants and mutations of SARS-CoV-2, especially the variants of concern (VOC) and variants of interest (VOI), summarize the clinical trials on evaluating the effectiveness of COVID-19 vaccines on VOC, and present some current challenges and future strategies for fighting the pandemic.
Figure 1. The global trend of confirmed COVID-19 cases/deaths (A) and the WHO regional trend of confirmed COVID-19 cases (B). The data used in this figure was collected from WHO COVID-19 Dashboard (https://covid19.who.int/) and presented by cases per weekly. The data were obtained on April 20, 2022.
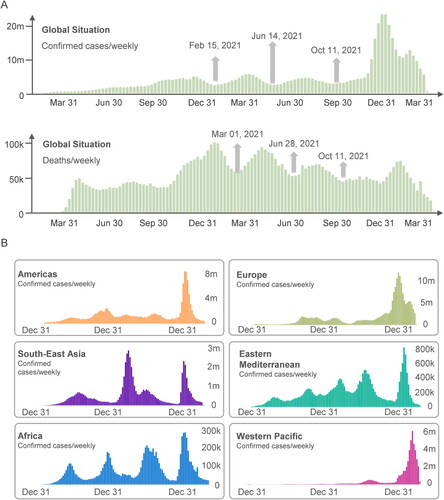
2. The variants and mutations of SARS-CoV-2
2.1. Evolutionary lineages of SARS-CoV-2
During the COVID-19 pandemic, variants of SARS-CoV-2 have been appearing and spreading around the world. According to the data released by Nextstrain, there are 2,920 genomes sampled between Dec 2019 and Apr 2022 (https://nextstrain.org/ncov/open/global?c=emerging_lineage). Although the emergence of these novel variants is inevitable and most of them have no significant effect on the behavior of the virus, some mutations may produce phenotypic changes. These SARS-CoV-2 genetic lineages were named and tracked by GISAID, Nextstrain, and Pango (Table S1). To facilitate discussions and avoid confusion, WHO gathered scientists and representatives from GISAID, Nextstrain, and Pango to name and track VOIs and VOCs using the letters of the Greek alphabet according to the global impact of these factors. As of April 20, 2022, WHO had currently designated two VOCs (Delta and Omicron, ), previously designated three VOCs (Alpha, Beta, and Gamma, ), and previously designated eight VOIs (Epsilon, Zeta, Eta, Theta, Iota, Kappa, Lambda, and Mu).
Table 1. Currently and previously designated variants of concern (VOC) by WHO.
The newly emerged VOCs and VOIs are characterized by the following features: (1) increased transmissibility; (2) difficult to be detected by diagnostic methods; (3) worse clinical manifestations; (4) escape from natural immunity or vaccine-induced immunity; and (5) reduced response to treatments. We analyzed the data of genomic epidemiology of SARS-CoV-2 with global subsampling obtained from Nextstrain database and found that B.1.1.7 (Alpha), B.1.351 (Beta), P.1 (Gamma), B.1.617.2 (Delta), B.1.1.529 (Omicron), B.1.621 (Mu), and C.37 (Lambda) were the latest and most important variants (). The evolutionary relationships of SARS-CoV-2 viruses from the ongoing COVID-19 pandemic showed that 93% of the virus samples collected before April 2021 were Alpha, Beta, and Gamma emerging lineages, after which the frequencies of Delta variant rapidly increased from 1% in April 2021 to 91% in November 2021 (). Currently, the frequency of B.1.1.529 (Omicron) is rising, accounting for 5% on 13 December 2021 ().
Figure 2. The evolutionary relationships of SARS-CoV-2 from the ongoing COVID-19 pandemic. (A) The data used herein were obtained from 2716 genomes sampled between Dec 2019 and Dec 2021. Each genomic sample was showed by a solid dot and colored by Emerging Lineages. (B) The frequencies of variants sampled between Jan 2021 and Dec 2021 were presented by different colors. All of data was obtained on April 20, 2022.
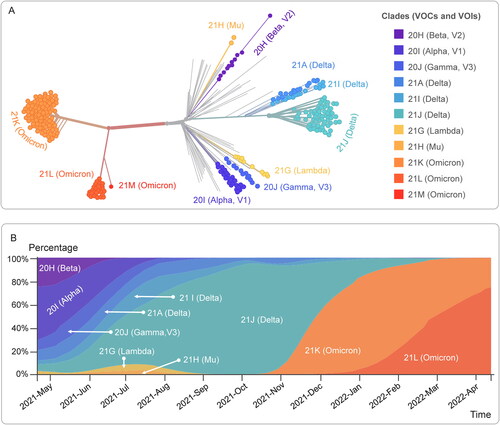
2.2. Characteristics of SARS-CoV-2 VOCs and VOIs
By analyzing the data obtained from outbreak.info, 26 mutations with >75% prevalence were observed in at least one lineage among five VOCs and two VOIs, including six mutations of interest (L18F, K417T, L452R, N501Y, P681H, and P681R, ). The mutation D614G was shared by all these seven variants, N501Y was shared by the Alpha, Beta, Gamma, Omicron, and Mu variants. Furthermore, we determined that the mutations in Spike protein Receptor Binding Domain (RBD) were present in at least 20% of countries, and found that L452R, N501Y, T478K, E484K, K417N, S477N, and E484Q mutations were identified in 191, 191, 187, 173, 134, 123, and 100 countries, respectively (). A study revealed that RBD mutations L452R, T478K and E484Q may be associated with increased ACE2 binding, and P681R resulted in a higher transmissibility [Citation9].
Figure 3. Mutation prevalence across VOC and VOI lineages and their geographical distribution on Receptor Binding Domain (RBD) of Spike protein. (A) Mutations with >95% prevalence in at least one lineage. The data were obtained from https://outbreak.info/situation-reports and accessed on Dec 14, 2021. (B) Showing mutations in Spike protein RBD present in at least 20% of countries for visualization purposes. The data were collected from COVID-Miner website (https://covid-miner.ifo.gov.it/app/countries) and accessed on Dec 14, 2021.
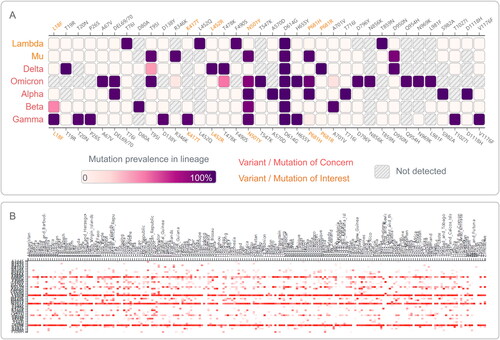
The characteristics of SARS-CoV-2 VOCs and VOIs have been listed in . The B.1.1.7 (Alpha), B.1.351 (Beta), and P.1 (Gamma) are COVs emerged between the end of 2020 and beginning of 2021 in the UK, South Africa, and Brazil, respectively [Citation2]. Both Alpha and Beta variants have a 50% increased transmission [Citation10,Citation19] and a declined neutralization effect on monoclonal antibodies against the N-terminal domain and receptor-binding domain of the spike protein [Citation14], but has no impact on susceptibility to EUA monoclonal antibody treatments [Citation13,Citation20]. Previous studies have reported that Alpha variant was characterized by increased mortality and critical care unit admission [Citation12], and was luckily found susceptible to neutralizing antibodies elicited by the vaccines which were based on the Spike protein production [Citation18]. Effectiveness after two doses of BNT162b2, ChAdOx1 nCoV-19, and NVX-CoV2373 vaccines were 93.7%, 74.5%, and 86.3% against the Alpha variant [Citation15–17]. For the Beta variant, BNT162b2 vaccine offers significant protection [Citation17], mRNA-1273 vaccination shows a reduced but still significant neutralization [Citation21], but ChAdOx1 nCoV-19 vaccine failed to show enough protection [Citation22]. Gamma variant shows a reduced susceptibility to treatments with bamlanivimab and etesevimab monoclonal antibodies [Citation37], and a reduced neutralization by convalescent and past-vaccine sera [Citation23]. Furthermore, it has been reported that BNT162b2 vaccine offered protection against the Gamma variant [Citation17].
Table 2. Characteristics of SARS-CoV-2 VOCs and VOIs.
B.1.617.2 (Delta) variant was first discovered in India at the end of 2020 and appeared to spread rapidly. Two mutations of interest with prevalence > 75% (L452R, and P681R) were observed in the lineage, which impacted antibody binding [Citation9]. Previous studies have found that the Delta variant has increased transmissibility in indoor sports settings and within households [Citation24,Citation25], and it also shows potential reduction in neutralization by some EUA monoclonal antibody treatments [Citation13,Citation20]. Effectiveness after one dose of Pfizer and AstraZeneca vaccines is only 33% against Delta variant [Citation26], and the protective efficacy of two doses of BNT162b2 vaccine is also reduced [Citation25]. Compared with the Alpha variant, the effectiveness of two doses of BNT162b2 or ChAdOx1 nCoV-19 vaccines was significantly decreased but still remained higher than 67% [Citation16].
Among the VOC, the latest SARS-CoV-2 variant to emerge is VOC “Omicron” as reported by WHO [Citation38]. The B.1.1.529, named as Omicron, was first reported from South Africa on 24 November 2021. The WHO reported that there has been an increase in SARS-CoV-2 infections in South Africa, coinciding with the detection of latest variant B.1.1.529. The first known infection by B.1.1.529 was from a sample that was collected on November 9, 2021 [Citation38]. The B.1.1.529 VOC has already spread around the world and the studies are underway to understand the consequences of mutations that far exceed the numbers in earlier variants. The B.1.1.529 contains 50 mutations, of which 32 target the spike protein (). The variant also has three mutations in the furin cleavage site that is responsible for increasing the SARS-CoV-2 infectivity [Citation41]. The details of the mutations in the B.1.1.529 are: Spike protein: A67V, Δ69–70, T95I, G142D, Δ143–145, Δ211, L212I, ins214EPE, G339D, S371L, S373P, S375F, K417N, N440K, G446S, S477N, T478K, E484A, Q493R, G496S, Q498R, N501Y, Y505H, T547K, D614G, H655Y, N679K, P681H, N764K, D796Y, N856K, Q954H, N969K, and L981F. Half (15) of these 32 changes are located in the receptor binding domain-RBD (residues 319–541). The open reading frames 1a and 1 b contains nsp3: K38R, V1069I, Δ1265, L1266I, A1892T. nsp4: T492I. nsp5: P132H. nsp6: Δ105–107, A189V. nsp12: P323L. nsp14: I42V. In the envelope protein T9I, membrane protein: D3G, Q19E, A63T and in nucleocapsid protein: P13L, Δ31–33, R203K, G204R. Initial reports showed that the B.1.1.529 is more transmissible than any known variant so far and could evade the immune protection conferred by the currently used vaccines or previous infection by SARS-CoV-2. As of December 16, 2021, the virus has already spread to 86 countries and territories and there are 6,950 cases according to GISAID. More recent studies showed that the neutralization activity of serum against Omicron variant was 41.4 times lower than against the original strain after vaccination with Pfizer BNT162b2 vaccine but the escape was incomplete [Citation29]. The Omicron variant is also associated with substantial ability to evade immunity from prior infection [Citation30].
Figure 4. The locations of the Omicron mutations in the spike protein showing amino acid substitutions (yellow), deletions (red), and insertions (green). In this trimeric structure, two monomers (gray and light blue) have their receptor-binding domains in the "down" conformation while one (dark blue) is in the "up" or "open" conformation. Mutation data from WHO structure from PDB: 6VYB (Opabinia regalis [Citation39,Citation40]).
![Figure 4. The locations of the Omicron mutations in the spike protein showing amino acid substitutions (yellow), deletions (red), and insertions (green). In this trimeric structure, two monomers (gray and light blue) have their receptor-binding domains in the "down" conformation while one (dark blue) is in the "up" or "open" conformation. Mutation data from WHO structure from PDB: 6VYB (Opabinia regalis [Citation39,Citation40]).](/cms/asset/6487bcbd-d862-4531-a736-91984729159d/iiri_a_2079642_f0004_c.jpg)
Currently, two variants were defined as VOIs by WHO, including C.37 (Lambda) and B.1.621 (Mu). C.37 (Lambda) appears to have arisen in late 2020 in Peru and Chile and was first identified in April 2021. It has now also been detected in North America, Europe, and the Middle East. Lambda variant carries a mutation L452Q in the RBD of the Spike protein, but other recent variants such as Delta, Kappa, Epsilon, and Iota carry L452R mutation. It was reported that the L452R mutation relates to immune escape and a stronger cell attachment, increasing the transmissibility, infectivity, and pathogenicity of the virus [Citation42]. Although the role of L452Q is still unknown, recent studies presented a reduction in neutralization of 3.05-fold for Lambda variant compared to the wild-type virus [Citation36], and a decreased sensitivity to neutralization by convalescent sera and REGN10987 monoclonal antibody [Citation35]. Moreover, this variant shows slightly reduced sensitivity to antibodies induced by BNT162b2, mRNA-1273, and CoronaVac vaccines, but is still sensitive to REGN10933 monoclonal antibody [Citation35,Citation36]. B.1.621 (Mu) was first observed in Colombia in January 2021. Mehul Suthar et al. analyzed antibody responses to the emerging Mu (B.1.621) variant and found that neutralizing antibody response to the Mu variant was 2.3-fold lower in comparison to the WA1 strain in day 42 sera [Citation33]. Further study examined the neutralizing efficacy of the BNT162b2 mRNA vaccine against the Mu (B.1.621) variant and reported that the BNT162b2 mRNA vaccine has 76% neutralizing effectiveness against the Mu variant compared to 96% with the original strain [Citation34], and two doses of mRNA-1273 vaccine was 90.4% (73.9–96.5%) effective against the Mu variant [Citation32].
3. The effectiveness of COVID-19 vaccines on VOCs
The COVID-19 specific vaccines authorized under EUA can be divided into four categories, including (1) inactivated vaccines (BBV152, BBIBP-CorV, and Sinovac); (2) Viral vector vaccine (ChAdOx1 nCoV-19, Sputnik V, CanSino’Ad5-nCoV, Ad26.COV2.S); (3) Nucleic acid vaccine (BNT162b1/BNT162b2, mRNA1273, CureVac); (4) Subunit vaccine (NVX-CoV2373, EpiVacCorona, and ZF2001) [Citation1–3,Citation43–46]. Although the types and development platforms of these vaccines vary widely, they are all based on the S protein of the SARS-CoV-2 virus. These COVID-19 specific vaccines induce the host to produce neutralizing antibodies that can specifically bind to the S protein of the virus to fight against the virus invasion.
However, the emergence of SARS-CoV-2 variants has brought great challenges for the development of COVID-19 specific vaccines. It was reported that some mutations on Spike protein of the virus have resulted in enhanced transmission and infectivity, more severe disease, decreased neutralization by special antibodies, and immune avoidance to Covid-19 vaccines [Citation2–4,Citation47–51]. However, these results were obtained from observational studies and small sample size research, which should be confirmed by clinical trials with larger sample size. Furthermore, there is evidence that, compared with VOIs, VOCs are more infectious. They are associated with higher hospitalization rate and mortality, weaker antibody neutralization, lower vaccine effectiveness, and higher failure rate of detection and diagnosis [Citation52]. Herein, we summarized the latest clinical trials on evaluating the effectiveness of COVID-19 vaccines on VOCs, especially the widespread variants such as B.1.1.7 (Alpha), B.1.351 (Beta), and B.1.617.2 (Delta) ( and ).
Figure 5. Forest plots of COVID-19 vaccine effectiveness on variants of concern (randomized evidence). The data were collected from COVID-NMA (https://covid-nma.com/vaccines/variants/index.php), Clinicaltrials.gov (https://clinicaltrials.gov/ct2/home). Accessed on Aug 16, 2021.
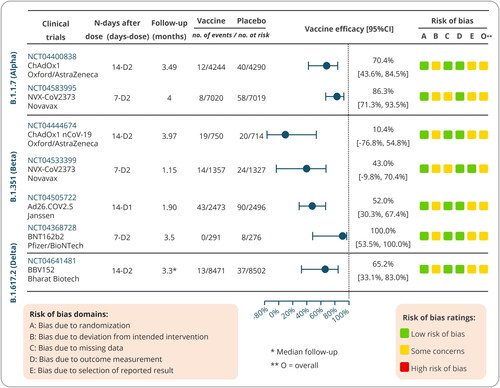
Table 3. Clinical trials on evaluating the effectiveness of COVID-19 vaccines on VOCs.
3.1. Clinical trials on Alpha variant
3.1.1. Efficacy of ChAdOx1 nCoV-19 vaccine against Alpha variant (NCT04400838)
The sharp increase in morbidity and mortality caused by B.1.1.7 (Alpha) appeared in the UK at the end of 2020. To determine the efficacy of the ChAdOx1 nCoV-19 vaccine against this variant, a phase 2/3 study was conducted to evaluate the efficacy, safety and immunogenicity of the vaccine in 8,534 healthy volunteers (aged ≥18 years) in the UK. The volunteers were randomly assigned to receive ChAdOx1 nCoV-19 vaccine (n = 4244) or an anti-meningococcal conjugate control (MenACWY, n = 4290). After two doses of immunization, the results showed that neutralization activity induced by the ChAdOx1 nCoV-19 vaccine was lower against the Alpha variant than against the non-Alpha form, and the protective efficacies against the Alpha variant and non-Alpha form were 70.4% [95% confidence interval (CI) 43.6%–84.5%] and 81.5% [67.9%–89.4%], respectively [Citation53]. These data suggest that although a depressed neutralization activity against the Alpha variant was observed in vitro, the ChAdOx1 nCoV-19 vaccine revealed an acceptable protection against this variant.
3.1.2. Efficacy of NVX-CoV2373 vaccine against Alpha variant (NCT04583995)
Previous studies reported that the protective efficacy of NVX-CoV2373 (Novavax) vaccine against the original SARS-CoV-2 strain, Alpha variant, and Beta variant were 95.6%, 85.6%, and 49.4%, respectively [Citation2,Citation57]. However, the immunogenicity, efficacy, and safety of this vaccine should be evaluated in a larger population. Recently, a phase 3, randomized, single-blinded, placebo-controlled trial was performed to assess the efficacy and safety of NVX-CoV2373 vaccine with Matrix-M1 adjuvant in 14,039 participants (18–84 years old) in the UK. Volunteers in vaccine group or placebo group were intramuscular administered with two doses of NVX-CoV2373 vaccine (5 μg) or placebo (0.5 ml of sodium chloride 0.9%), respectively. The primary efficacy end point was polymerase chain reaction (PCR)-confirmed patients with mild, moderate, or severe SARS-CoV-2 infection with onset of at least 7 days after the second injection, and the testing serologically negative for SARS-CoV-2 at baseline. The results showed an efficacy of 86.3% [71.3%–93.5%] against the Alpha variant and 96.4% [73.8%–99.5%] against non-Alpha variants [Citation15]. This clinical trial did not assess vaccine efficacy after a single dose, but previous studies have showed that NVX-CoV2373 vaccine induced an acceptable protection after the first dose in a range like those of mRNA-1273, BNT162b2, ChAdOx1 nCoV-19, and Ad26.COV2.S vaccines [Citation58–60]. Furthermore, this clinical trial also assessed the efficacy of NVX-CoV2373 vaccine against Beta variant, and the results revealed a 51.0% efficacy against the Beta variant, which is similar to a previous study [Citation44].
3.2. Clinical trials on Beta variant
3.2.1. Efficacy of ChAdOx1 nCoV-19 vaccine against Beta variant (NCT04444674)
The ChAdOx1 nCoV-19 (AZD1222) vaccine is developed by AstraZeneca in collaboration with University of Oxford and has been approved under EUA. Previous meta-analysis based on four randomized clinical trials [NCT04324606 (COV001), NCT04400838 (COV002), ISRCTN89951424 (COV003), and NCT04444674 (COV005)] found that the ChAdOx1 nCoV-19 vaccine against the original strain of SARS-CoV-2 had a 66.7% overall vaccine efficacy, and the vaccine efficacy in participants with a longer prime-boost interval (81·3%, ≥12 weeks) was higher than that in participants with a short interval (55·1%, <6 weeks) [Citation61]. However, the vaccine efficacy against SARS-CoV-2 Beta variant is unclear. To evaluate the safety, immunogenicity, and efficacy of the ChAdOx1 nCoV-19 vaccine against Beta variant, a Phase I/II, double-blinded, placebo-controlled, individually randomized trial was conducted in adults aged 18–65 years living with and without HIV in South Africa. The results were released on May 30, 2021, and showed that the ChAdOx1 nCoV-19 vaccine efficacy against Beta variant was 10.40% [−76.80%–54.80%], suggesting a two-dose regimen of the ChAdOx1 nCoV-19 vaccine presented a disappointing protection against mild-to-moderate COVID-19 caused by Beta variant [Citation22].
3.2.2. Efficacy of Ad26.COV2.S vaccine against Beta variant (NCT04505722)
The Ad26.COV2.S vaccine consists of a non-replicating human adenovirus type 26 vector, which encodes the full-length Spike protein of SARS-CoV-2. In February 2021, this single-dose vaccine was approved for preventing COVID-19 in individuals over the age of 18 under EUA by the Food and Drug Administration (FDA) of the USA. A phase 1 clinical trial (NCT04436276) showed that a single-dose vaccination with Ad26.COV2.S induced high level of neutralization antibodies and cellular immune responses [Citation62]. Recently, a randomized, double-blind, placebo-controlled Phase 3 study was performed to assess the efficacy and safety of this vaccine for the prevention of SARS-CoV-2 Beta variant in adults aged 18 years and older (NCT04505722). Globally, 19,514 and 19,544 participants were vaccinated with Ad26.COV2.S vaccine and placebo, respectively. Among them, in South African cohort, a total of 2,473 participants received Ad26.COV2.S vaccine, and 2,496 participants received placebo. The results from South African cohort reported that the efficacy of Ad26.COV2.S vaccine against moderate to severe-critical disease or severe-critical disease with onset at least 14 days after administration was 52.0% [30.3%, 67.4%] or 73.1% [40.0%, 89.4%], and the efficacy of this vaccine against moderate to severe-critical disease or severe-critical disease with onset at ≥28 days after administration was 64.0% [41.2%, 78.7%] or 81.7% [46.2%, 95.4%], respectively [Citation54].
3.2.3. Efficacy of NVX-CoV2373 vaccine against Beta variant (NCT04533399)
The NVX-CoV2373 is a subunit vaccine composed of the full-length Spike protein of SARS-CoV-2 and Matrix-M1 adjuvant. This vaccine had an acceptable safety profile and elicited strong neutralizing-antibody as well as cellular immune responses in animal models [Citation63–65]. A phase 1/2 clinical trial suggested that this vaccine was safe for participants, and induced Spike-specific IgG antibody and neutralization responses [Citation66]. It is urgent to evaluate the efficacy of NVX-CoV2373 vaccine in a setting of ongoing Beta variant transmission. A phase 2a/b, randomized, observer-blinded, placebo-controlled study was conducted to evaluate the efficacy, immunogenicity, and safety of this vaccine in South African adult subjects living without HIV and safety and immunogenicity in adults living with HIV (NCT04533399). The results showed that vaccine efficacy against Beta variant was 51.0% [−0.6%, 76.2%] in HIV-negative participants and 43.0% [−9.8%, 70.4%] in the combined HIV-negative participants and people living with HIV [Citation44].
3.2.4. Efficacy of BNT162b2 vaccine against Beta variant (NCT04368728)
BNT162b2 is a COVID-19 vaccine based on RNA and lipid nanoparticles, which encodes the full-length prefusion Spike protein of SARS-CoV-2. This vaccine has been approved for vaccination worldwide under EUA, but the data after 2 months post-vaccination is still unknown. Recently, a phase 1/2/3, placebo-controlled, randomized, observer-blind, and dose-finding clinical trial was conducted to assess six-month safety and efficacy of the BNT162b2 vaccine in healthy participants at 152 sites in Argentina, Brazil, Germany, South Africa, Turkey, and the USA (NCT04368728). The results of BNT162b2 vaccine efficacy in participants 16 years of age or older showed 95% protection [Citation59] and in 12–15 years old recipients 100% protection [Citation67]. In this report, the overall efficacy of BNT162b2 vaccine was 91.1% against COVID-19 from 7 days to 6 months post-dose 2 in participants over 12 years of age, and the results from South African cohort, where Beta lineage is prevalent, showed that the efficacy of BNT162b2 vaccine against Beta variant infection was 100.0% [53.5%, 100.0%] [Citation55]. Globally, this clinical trial recruited 44,165 volunteers over the age of sixteen and 2,264 adolescents aged 12–15, all of them were randomized to receive two doses of 30 µg BNT162b2 or placebo at 21 days apart. However, in South African cohort, 291 and 276 volunteers were included in the vaccine group and the placebo group, which accounted for only 1.2% (567/46429) of all participants. Therefore, the efficacy results against Beta variant in South Africa need to be confirmed by another clinical trial with a larger sample size.
3.3. Clinical trial on Delta variant
As mentioned above, the Delta strain rapidly became the dominant variant in the world due to its increased transmissibility in indoor sports venues and homes [Citation24]. The results from a live-virus SARS-CoV-2 neutralization assay showed that neutralizing antibody titers in 250 participants after either one or two doses of BNT162b2 vaccination were significantly decreased against the Delta and Beta variants compared with the Alpha variant [Citation25]. Similarly, other studies showed that either one dose or two doses of BNT162b2 or ChAdOx1 nCoV-19 vaccine were significantly less effective against the Delta variant than the Alpha variant [Citation16,Citation26]. Although these data depict a trend chart showing that the efficacy of existing COVID-19 vaccines against the Delta variant has decreased significantly, these results and their representativeness needs to be verified by clinical trials with larger sample sizes. On July 02, 2021, the results from a phase 3, randomized, double-blind, placebo-controlled, multicenter clinical trial were released on the medRxiv (NCT04641481). This study evaluated the efficacy, safety, and immunogenicity of BBV152 vaccine in adults over 18 years of age at 25 sites in India. The results demonstrated that 13 and 37 Delta-positive cases were identified in the BBV152 vaccine group (8471 participants) and placebo group (8502 participants), respectively, resulting in an efficacy of 65.2% [95% CI: 33.1%, 83.0%] against the Delta variant [Citation56].
4. Current challenges and future strategies
4.1. Challenges due to VOCs
The COVID-19 pandemic is the most serious pandemic that has afflicted humanity in the recent times. Controlling the pandemic is very challenging and the emergence and rapid transmission of the VOCs make the job even more demanding (). These VOCs may have a fitness advantage because they are antigenically distant enough from the original SARS-CoV-2 viruses responsible for the first wave of pandemic and have been shown to reinfect people more efficiently [Citation83–85]. The COVID-19 pandemic has already caused economic and social hardships and loss of millions of lives in the world. The emergence and spread of viral variants raise concerns that our global battle with the pandemic must continue much longer than predicted. At the same time, the existing and emerging long-term consequences manifesting as a new syndrome, long-COVID-19, may represent the next major health disaster confronting humanity.
Table 4. Impact of the SARS-CoV2 variants of concern (VOC) on the COVID-19 pandemic in the world (as of December 2021) [Citation68].
The approval of vaccines for COVID-19 in less than a year is unprecedented in human history and medical research [Citation86]. The development and deployment of vaccines have been a remarkable science success story: within 16 months of the first vaccine trials, 2.8 billion vaccine doses were administered. Despite significant improvements and encouraging results from the vaccine candidate trials, numerous challenges remain that are as follows ().
Figure 6. Current challenges and future strategies. An illustration depicting the current challenges to control the Covid-19 pandemic due to emergence of VOCs and various strategies control the combat the current and future pandemics due to corona viruses.
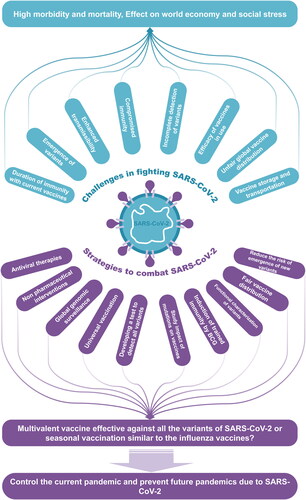
There are several vaccines that have received Emergency Use Authorization (EUA) and each one of them has unique storage requirements. The vaccines need to be distributed throughout the world and the proper transportation is a huge task. It is important to keep these vaccines in their desired storage conditions to ensure the stability and efficacy. Many tropical or subtropical countries do not have enough storage facilities, and the challenge of keeping the vaccines at their desired temperature is a huge challenge in such places [Citation87].
Hoarding and delayed supplies of vaccines in some countries create difficulties in controlling the pandemic and might create better conditions for the emergence of new variants, which in turn, can perpetuate the pandemic [Citation88–90]. There are challenges to distribute and administer the vaccine to over 7 billion people worldwide in producing the enough number of doses required for all the eligible people in the world. It is necessary to vaccinate every eligible person in the world as nobody would be safe until everyone is safe. The large differences in the rate of vaccination coverage between countries imply significant inequality in vaccine distribution, which may be caused by different national economics, difficulties with logistics and storage, lower rates of vaccination, the extent to which vaccination has been promoted by the government or health authorities [Citation91].
The variants, especially the VOCs may reduce the detection sensitivity of RT-PCR based diagnostic tools especially when mutations occur in locations where probes and primers may bind [Citation92,Citation93]. Mutations in nucleic acid can lead to changes in protein sequence and conformation, which are important determinants for antibody binding. These changes can result in a failure of diagnostic immunoassay to detect variant, leading to false negative COVID-19 detection [Citation93,Citation94].
The hesitancy of people to get safe and recommended vaccines known as “vaccine hesitancy” was a growing concern even before the COVID-19 pandemic [Citation95]. Vaccine hesitancy can also hamper efforts to fight pandemic and therefore, governments need to actively raise public confidence, especially in disadvantaged groups. The studies carried out to find the reasons across the world showed that, in high income countries concerns were raised about the safety issues due to the rapid development of the vaccines [Citation90]. The data from low- and middle-income countries (LMICs) showed that the concerns about the side effects were most common for hesitancy in taking the vaccine [Citation96].
The SARS-CoV-2 variants present new global challenges to control the COVID-19 pandemic due to increased transmission and virulence. The newly emerged SARS-CoV-2 VOCs () have brought major challenges to the effectiveness of currently used vaccines and vaccine research. As speculated earlier, the emergence of the VOCs has brought a new phase of the pandemic [Citation97]. When studied the presence of antibodies against SARS-CoV-2 after the viral infection, about 95% of the people retained the antibodies at six months post-infection [Citation98,Citation99]. Interestingly, reinfection has also been observed in these people [Citation100]. These findings suggested that reduction in immunity is evident after the immunization with current vaccines and may necessitate several booster shots to protect against future infections with SARS-CoV-2 [Citation101]. Scientists working with SARS-CoV-2 virus have suggested that there is a possibility that the COVID-19 disease will persist and become a recurrent seasonal disease [Citation102]. The SARS-CoV-2 might become endemic virus, but the pattern it will take is difficult to predict [Citation103]. As shown in , the virus may follow several routes and lead to different scenarios in the future. Studies have clearly shown that there is a significant reduction in the efficacy of vaccines against the VOCs () [Citation104].
To find a quick response to the pandemic, many COVID-19 vaccines have been developed using different technologies and platforms consisting of DNA, RNA, viral vector, inactivated virus, protein subunit, and live attenuated vaccines [Citation105,Citation106]. The main target of the current vaccines is the Spike (S) protein and especially the receptor binding domain (RBD) in S that induces antibody to block the entry of SARS-CoV-2 into host cell [Citation107]. These vaccines developed using different platforms show different levels of efficacy [Citation108,Citation109], which range between 65–95% in the vaccines that are currently in use, and the RNA vaccines have shown up to 95% efficacy levels [Citation58,Citation59]. All these vaccines require two doses with at least 20–30 days gap to obtain the expected protection in adults and in children above the age of 5 years except the vaccine from Johnson & Johnson that is given as a single dose in adults above the age of 18 years [Citation108]. Even though the efficacy of some of these vaccines is good, the virus is constantly changing, and newly emerged VOCs seem to evade the currently used vaccines ().
There is a need to monitor the appearance of SARS-CoV-2 animal species. It is difficult to predict if complete eradication of SARS-CoV-2 virus can be feasible even if herd immunity is reached with satisfactory coverage [Citation110]. The current vaccine rollout in the world may not provide complete immunity in some individuals and may not reach certain subpopulations, leaving some regions vulnerable. Transmissions within these subpopulations, the high proportion of asymptomatic COVID-19 infections, and waning of both post-infection and vaccine-induced immunity could maintain the circulation of the virus in the global population. Even if eliminated in humans, the multitude of documented non-human hosts and several animals that have been in contact with infected humans have been tested positive for SARS-CoV-2 [Citation111,Citation112]. These findings suggest that the virus could remain circulating with ongoing risks of infection and potential further spread between susceptible human hosts.
4.2. Future strategies to combat COVID19 pandemic due to VOC
The availability of several effective vaccines against SARS-CoV-2 has given hope to billions of people across the globe [Citation21,Citation22]. With the currently used vaccines, it is time-consuming to immunize enough to reach herd immunity or end the pandemic, especially given the 2- or 3-dose regimens. The current pandemic is complicated by the emergence of VOCs and there are already five SARS-CoV-2 VOCs, which have shown increased transmissibility and resistance to the efficacy of vaccines and monoclonal antibodies [Citation36,Citation37]. It is of critical importance to implement different strategies to deal with the VOCs and potential outbreaks. Some of the strategies are as follows that will help in reducing the morbidity and mortality due to SARS-CoV-2 infections and controlling the current pandemic and preventing the future outbreaks ().
Nonpharmaceutical interventions (NPIs) are practices people can use to slow down the spread of SARS-CoV-2. NPIs are among the best ways of controlling COVID-19 pandemic when vaccines are not effective or unavailable for the VOCs. With the emergence of VOCs that are spreading very fast and partially escape the immunity provided by the current vaccines, the best strategy is to maintain social distancing, maintain good personal hygiene, and wear a mask when being in contact with other people [Citation113].
Antiviral therapy has been suggested to be useful for the treatment of moderate and severe illness due to SARS-CoV-2 as the replication of virus leads to many clinical manifestations of COVID-19. The antiviral drugs such as remdesivir, ivermectin, nitazoxanide, hydroxychloroquine or chloroquine and/or azithromycin and lopinavir/ritonavir and other HIV protease inhibitors may inhibit viral entry via ACE2 receptor, function of transmembrane serine protease 2 (TMPRSS2), viral membrane fusion and endocytosis, or the activity of the SARS-CoV-2 3-chymotrypsin-like protease (3CLpro), and the RNA-dependent RNA polymerase [Citation114]. To block the entry of SARS-CoV-2 into host cells use of antimalarial drugs such as Camostat targeting host cell TMPRSS2 [Citation115,Citation116]. The drugs such as remdesivir interfere with viral replication in vitro and in vivo by inhibiting the RNA dependent RNA polymerase (RdRP) [Citation117]. The other therapeutic approaches for VOCs include engineered recombinant soluble ACE2 that is reported to inhibit SARS-CoV-2 infection in vitro by blocking viral entry when recombinant ACE2 interacts with RBD of SARS-CoV-2 S protein with high affinity [Citation117,Citation118]. This could be a powerful treatment against SARS-CoV-2 VOCs. Another approach that targets nucleocapsid protein and Redrop in SARS-CoV-2 viral genome is antiviral CRISPR in human cells (PAC-MAN), which is a Cas13d-based strategy with a potential to combat all the corona viruses and the VOCs [Citation119]. In animal models, monoclonal antibodies targeted against viral S protein and administered intranasally have been effective in COVID-19 prophylaxis [Citation120]. Recently, Mäkelä et al. described novel engineered Sherpabodies which were targeted against a conserved RBD region of SARS-CoV-2 spike protein [Citation121]. These targeting modules can be administered intranasally to people prior to any event that could possess a risk for SARS-CoV-2 exposure.
To combat the SARS-CoV-2 variants genomic surveillance of variants is another strategy in the future [Citation122]. It is important to obtain reliable information whether they are more infectious, virulent, and immune resistant to currently used vaccines well before they spread throughout the world [Citation123]. Genomic surveillance can use next generation sequencing to create the availability of whole genome data and advanced phylogenetic methods, and process further the obtained data using highly accurate structural modeling tools, such as AlphaFold [Citation124], to detect variants that are phenotypically or antigenically different. Genomic surveillance will facilitate early anticipation pandemic as well as initiation of effective strategies to reduce the spread of SARS-CoV-2 variants and prevent spread of other novel pathogenic viruses in the future [Citation123,Citation125].
The currently used vaccines have different levels of efficacy. They have been implemented in vaccination programs depending on the resources and decisions in each country. These vaccines show reduced efficacy against the VOCs (). There have been opinions that the world needs a universal vaccine, the vaccine which is effective against the SARS-COV-2 regardless of the virus sub type, antigenic drift or antigenic shift that does not require modification from year to year which could provide protection against all viruses or strains within a given virus family [Citation126–128]. However, it is challenging to develop universal as it requires more resources, and it depends on breakthroughs and discoveries with no guarantee to achieve success. Even if a universal vaccine is developed, it may not prevent the new pandemics and it is difficult to predict how long the immunity lasts against the SARS viruses. Even though that goal may not be feasible in the near future, it is important to immunize all eligible people in the world using vaccines that provide maximal protection to reduce the emergence and spread of variants.
The variants of SARS-CoV-2 can affect the sensitivity of the test used for detection of the virus in infected people. It is important to identify new variants of the SARS-CoV-2 for monitoring the pandemic and the evolution of virus. With the frequent emergence and spread of VOCs, it is important to ensure that the currently used diagnostic tests throughout the world can still detect the virus. The tests that are in use for detecting the SARS-CoV-2 work by detecting specific targets of the viral genome (molecular tests) or specific viral proteins (antigenic tests). In viral variants, a mutation may change the target that might affect the sensitivity of the test. Therefore, it is important to develop a test that can detect all the variants [Citation129].
In the past, studies have suggested that there have been fewer cases of COVID-19 disease and lower mortality in the countries with universal childhood vaccination with tuberculosis vaccine bacillus Calmette-Guerin (BCG) [Citation3,Citation130]. Immunization using BCG has been shown to increase the ability of the immune system to fight against several other pathogens, in addition to tuberculosis, due to induction of nonspecific immunity. Currently, the effects of BCG on COVID-19 are being evaluated in a number of studies [Citation131]. A recent study showed reduction in Covid-induced inflammation in elderly people vaccinated with BCG [Citation131]. Another study involving health care workers showed a significant difference between the BCG vaccinated group and unvaccinated group with respect to COVID-19 infections [Citation132]. Therefore, universal BCG vaccination could be beneficial to control the current pandemic and any future viral pandemics [Citation3,Citation130].
The current pandemic is the deadliest pandemic the world has witnessed in the last 100 years. The COVID-19 disease is no longer a problem of a single region or country but a common threat to the entire world and therefore, the countries need to work cooperatively to overcome the pandemic [Citation133]. Rapid distribution and administration of the vaccine to all eligible people in the world is the most effective way to control the pandemic. There is a need for strong international coordination and cooperation among all the stakeholders involving vaccine industry to ensure that effective vaccines can be supplied to every corner of the globe. There is also a need to distribute the vaccines fairly, under the supervision of the World Health Organization. The slogan of WHO correctly states: “If the vaccine isn’t everywhere, this pandemic isn’t going anywhere” [Citation134].
In addition to the above strategies, there is a need to vaccinate animals to prevent from reverse zoonoses and prevent the sick animals from coming in contact with humans [Citation135]. There is an immediate need to monitor the disease severity associated with VOCs and implement a long-term strategy for global genomic surveillance of SARS-CoV-2, with a goal to detect rapidly the most significant variants and predict their potential clinical significance. According to the article “Can we predict the limits of SARS-CoV-2 variants and their phenotypic consequences?” published by UK Govt at https://assets.publishing.service.gov.uk (accessed the information from the website on 20.4.2022), there is a possibility that the new variants can arise through interactions between wild type and vaccine strains. Therefore, it has been suggested that the use of live attenuated vaccines for SARS-CoV-2 should be avoided.
Apart from genomic surveillance there is a need for more comprehensive scientific evaluation of new variants involving clinical data to predict the potential risk of each new variant compared to the previously studied variants. Processes and legislation are globally needed for the rapid access and sharing of viral sequences, clinical and biological materials related to virus isolates. This information will be vital to study more efficiently novel variants and assess the threat they pose to humans. Future strategies to combat virus involve the development of multivalent vaccines to fight against the current and future VOCs. It has been shown that the cocktail therapy may provide a powerful way to minimize mutational escape by SARS-CoV-2 [Citation136]. The development of vaccines targeting more conserved protein sequences with a low chance of mutation could be another strategy to avoid the emergence of future VOCs [Citation40]. An ideal vaccine would be effective against all variants, could be administered as a single dose, would preferably be noninjectable, and would also avoid cold-chain constraints.
5. Conclusions
The development of effective vaccines against SARS-CoV-2 within only 16 months of COVID-19 pandemic is a phenomenal achievement in the history of pandemics. Some of the vaccines that are in use show up to 95% efficacy and more than 8.09 billion doses have already been administered across 184 countries. However, the emergence of SARS-CoV-2 variants have posed a huge challenge in controlling the COVID-19 pandemic, especially the variants of concerns (VOCs) made major impact on the clinical and global health outcomes. The emergence of new variants greatly depends on the success of vaccinations and control measures to prevent further spread of VOCs to different parts of the world. Currently, the variants have posed a huge challenge of tracking and controlling the spread of pandemic. The VOCs have already led to reinfections and immune escape, and the currently used vaccines appear to be only partially effective against these variants. The emergence of the latest VOC, Omicron, suggests that complete eradication of SARS-CoV-2 will be challenging in the near future. The economic and health consequences of the pandemic are already immense, and the world will need long time to recover.
With the latest addition of Omicron variant to the list of VOCs, there are five major SARS-CoV-2 variants which have been shown to be highly transmissible and response more poorly than the original virus to the currently used vaccines. To fight against the challenges of SARS-CoV-2 VOCs now and in the future, the development of novel vaccines and high-throughput platforms for vaccine production are urgently needed. The best long-term solution is to develop multivalent vaccines that can protect against all SARS-CoV-2 VOCs. In the future, there may be a need for seasonal vaccination against the SARS-coronavirus similar to the approach that has been successfully implemented for decades against the influenza virus.
Authors’ contributions
Conceptualization: Wenping Gong, Xueqiong Wu, and Ashok Aspatwar; Data extraction: Wenping Gong and Ashok Aspatwar; Funding acquisition: Wenping Gong, Seppo Parkkila, and Ashok Aspatwar; Writing—original draft, Wenping Gong, Seppo Parkkila, and Ashok Aspatwar; Writing—review and editing, Wenping Gong, Xueqiong Wu, Seppo Parkkila, and Ashok Aspatwar. All authors read and approved the manuscript.
Supplemental Material
Download MS Excel (9.2 KB)Data availability
All data generated or analyzed during this study are included in this published article and its supplementary information files.
Declaration of Interest
The authors have no relevant affiliations or financial involvement with any organization or entity with a financial interest in or financial conflict with the subject matter or materials discussed in the manuscript. This includes employment, consultancies, honoraria, stock ownership or options, expert testimony, grants, or patents received or pending, or royalties.
Additional information
Funding
References
- Doroftei B, Ciobica A, Ilie OD, et al. Mini review discussing the reliability and efficiency of COVID 19 vaccines. Diagnostics (Basel, Switzerland). 2021;11(4):579.
- Jia Z, Gong W. Will mutations in the spike protein of SARS CoV2 lead to the failure of COVID 19 vaccines? J Korean Med Sci. 2021;36(18):e124. doi:10.3346/jkms.2021.36.e124.
- Gong W, Aspatwar A, Wang S, et al. COVID 19 pandemic: SARS CoV 2 specific vaccines and challenges, protection via BCG trained immunity, and clinical trials. Expert Rev Vaccines 2021;20(7):857–880. doi:10.1080/14760584.2021.1938550.
- Aspatwar A, Gong W, Wang S, et al. Tuberculosis vaccine BCG: the magical effect of the old vaccine in the fight against the COVID 19 pandemic. Int Rev Immunol. 2021;41(2):283–296.
- Galloway SE, Paul P, MacCannell DR, et al. Emergence of SARS CoV 2 B.1.1.7 lineage United States, December 29, 2020 January 12, 2021. MMWR Morb Mortal Wkly Rep. 2021;70(3):95–99. doi:10.15585/mmwr.mm7003e2.
- Tegally H, Wilkinson E, Giovanetti M, et al. Emergence and rapid spread of a new severe acute respiratory syndrome-related coronavirus 2 (SARS-CoV-2) lineage with multiple spike mutations in South Africa. medRxiv: the preprint server for health sciences. 2020.
- Wei Lin L, Xiaoqiong G, Federica A, et al. Quantitative SARS-CoV-2 tracking of variants Delta, Delta plus, Kappa and Beta in wastewater by allele-specific RT-qPCR. medRxiv: the preprint server for health sciences. 2021.
- Qin S, Cui M, Sun S, et al. Genome characterization and potential risk assessment of the novel SARS-CoV-2 variant omicron (B.1.1.529). Zoonoses. 2021;1(1). doi:10.15212/ZOONOSES-2021-0024.
- Cherian S, Potdar V, Jadhav S, et al. SARS-CoV-2 spike mutations, L452R, T478K, E484Q and P681R, in the second wave of COVID-19 in Maharashtra, India. Microorganisms. 2021;9(7):1542. doi:10.3390/microorganisms9071542.
- Davies NG, Abbott S, Barnard RC, et al. Estimated transmissibility and impact of SARS-CoV-2 lineage B.1.1.7 in England. Science (New York, NY). 2021;372(6538):372. doi:10.1126/science.abg3055.
- Lin L, Liu Y, Tang X, et al. The disease severity and clinical outcomes of the SARS-CoV-2 variants of concern. Front Public Health. 2021;9:775224. doi:10.3389/fpubh.2021.775224.
- Patone M, Thomas K, Hatch R, et al. Mortality and critical care unit admission associated with the SARS-CoV-2 lineage B.1.1.7 in England: an observational cohort study. Lancet Infect Dis. 2021;21(11):1518–1528. doi:10.1016/S1473-3099(21)00318-2.
- FDA. Fact sheet for health care providers emergency use authorization (EUA) of bamlanivimab and etesevimab. Indianapolis, USA; 2021.
- Wang P, Nair MS, Liu L, et al. Antibody resistance of SARS-CoV-2 variants B.1.351 and B.1.1.7. Nature. 2021;593(7857):130–135. doi:10.1038/s41586-021-03398-2.
- Heath PT, Galiza EP, Baxter DN, et al. Safety and efficacy of NVX-CoV2373 Covid-19 vaccine. N Engl J Med. 2021;385(13):1172–1183. doi:10.1056/NEJMoa2107659.
- Lopez Bernal J, Andrews N, Gower C, et al. Effectiveness of Covid-19 vaccines against the B.1.617.2 (Delta) variant. N Engl J Med. 2021;385(7):585–594. doi:10.1056/NEJMoa2108891.
- Zani A, Caccuri F, Messali S, et al. Serosurvey in BNT162b2 vaccine-elicited neutralizing antibodies against authentic B.1, B.1.1.7, B.1.351, B.1.525 and P.1 SARS-CoV-2 variants. Emerg Microbes Infect. 2021;10(1):1241–1243. doi:10.1080/22221751.2021.1940305.
- Shen X, Tang H, McDanal C, et al. SARS-CoV-2 variant B.1.1.7 is susceptible to neutralizing antibodies elicited by ancestral spike vaccines. Cell Host Microbe. 2021;29(4):529–539.e3. doi:10.1016/j.chom.2021.03.002.
- Pearson CA, Russell TW, Davie NG, et al. Estimates of severity and transmissibility of novel South Africa SARS-CoV-2 variant 501Y.V2. 2021.
- FDA. Fact Sheet for Health Care Providers Emergency Use Authorization (EUA) of Regen-Covtm (Casirivimab and Imdevimab). New York, USA: U.S. Food and Drug Administration (FDA); 2021:57.
- Wu K, Werner AP, Moliva JI, et al. mRNA-1273 vaccine induces neutralizing antibodies against spike mutants from global SARS-CoV-2 variants. bioRxiv: the preprint server for biology. 2021.
- Madhi SA, Baillie V, Cutland CL, et al. Efficacy of the ChAdOx1 nCoV-19 Covid-19 Vaccine against the B.1.351 Variant. N Engl J Med. 2021;384(20):1885–1898. doi:10.1056/NEJMoa2102214.
- Wang P, Casner RG, Nair MS, et al. Increased resistance of SARS-CoV-2 variant P.1 to antibody neutralization. Cell Host Microbe. 2021;29(5):747–751.e4. doi:10.1016/j.chom.2021.04.007.
- Dougherty K, Mannell M, Naqvi O, et al. SARS-CoV-2 B.1.617.2 (Delta) variant COVID-19 outbreak associated with a gymnastics facility - Oklahoma, April–May 2021. MMWR Morb Mortal Wkly Rep. 2021;70(28):1004–1007. doi:10.15585/mmwr.mm7028e2.
- Wall EC, Wu M, Harvey R, et al. Neutralising antibody activity against SARS-CoV-2 VOCs B.1.617.2 and B.1.351 by BNT162b2 vaccination. Lancet (London, England). 2021;397(10292):2331–2333. doi:10.1016/S0140-6736(21)01290-3.
- Iacobucci G. Covid-19: local councils initiate surge vaccination to tackle B.1.617.2 variant. BMJ. 2021;373:n1361. doi:10.1136/bmj.n1361.
- Mahase E. Covid-19: hospital admission 50–70% less likely with omicron than delta, but transmission a major concern. BMJ. 2021;375:n3151. doi:10.1136/bmj.n3151.
- Vogel G, Kupferschmidt K. Early lab studies shed light on Omicron’s behavior. Science. 2021;374(6575):1543–1544. doi:10.1126/science.acz9878.
- Cele S, Jackson L, Khan K, et al. SARS-CoV-2 Omicron has extensive but incomplete escape of Pfizer BNT162b2 elicited neutralization and requires ACE2 for infection. medRxiv: the preprint server for health sciences. 2021.
- Pulliam JRC, van Schalkwyk C, Govender N, et al. Increased risk of SARS-CoV-2 reinfection associated with emergence of the Omicron variant in South Africa. medRxiv: the preprint server for health sciences. 2021.
- Xie X, Han JB, Ma G, et al. Emerging SARS-CoV-2 B.1.621/Mu variant is prominently resistant to inactivated vaccine-elicited antibodies. Zool Res. 2021;42(6):789–791. doi:10.24272/j.issn.2095-8137.2021.343.
- Katia B, Lina SS, Lei Q, et al. Effectiveness of mRNA-1273 against Delta, Mu, and other emerging variants. medRxiv: the preprint server for health sciences. 2021.
- Mehul S, Prabhu SA, Mengyun H, et al. Durability of immune responses to the BNT162b2 mRNA vaccine. bioRxiv: the preprint server for biology. 2021.
- Kei M, Sundararaj SJ, Hideaki K, et al. Neutralizing efficacy of vaccines against the SARS-CoV-2 Mu variant. medRxiv: the preprint server for health sciences. 2021.
- Tada T, Zhou H, Dcosta BM, et al. SARS-CoV-2 Lambda variant remains susceptible to neutralization by mRNA vaccine-elicited antibodies and convalescent serum. bioRxiv: the preprint server for biology. 2021.
- Acevedo ML, Alonso-Palomares L, Bustamante A, et al. Infectivity and immune escape of the new SARS-CoV-2 variant of interest Lambda. medRxiv: the preprint server for health sciences. 2021.
- Wood DA, Aleem A, Davis D. Providing Access to Monoclonal Antibody Treatment of Coronavirus (COVID-19) Patients in Rural and Underserved Areas. Treasure Island (FL): StatPearls Publishing; 2021.
- WHO. Classification of omicron (B.1.1.529): SARS-CoV-2 variant of concern. 2021.
- Torjesen I. Covid-19: omicron may be more transmissible than other variants and partly resistant to existing vaccines, scientists fear. BMJ. 2021;375:n2943. doi:10.1136/bmj.n2943.
- Walls AC, Park YJ, Tortorici MA, et al. Structure, function, and antigenicity of the SARS-CoV-2 spike glycoprotein. Cell. 2020;181(2):281–292.e6. doi:10.1016/j.cell.2020.02.058.
- Zhang L, Mann M, Syed ZA, et al. Furin cleavage of the SARS-CoV-2 spike is modulated by O-glycosylation. Proc Natl Acad Sci U S A. 2021;118(47):e2109905118.
- Tchesnokova V, Kulasekara H, Larson L, et al. Acquisition of the L452R mutation in the ACE2-binding interface of Spike protein triggers recent massive expansion of SARS-CoV-2 variants. J Clin Microbiol. 2021;59(11):e0092121. doi:10.1128/JCM.00921-21.
- COVID-19 Vaccines. Drugs and Lactation Database (LactMed). Bethesda (MD): National Library of Medicine (US); 2006.
- Shinde V, Bhikha S, Hoosain Z, et al. Efficacy of NVX-CoV2373 Covid-19 vaccine against the B.1.351 variant. N Engl J Med. 2021;384(20):1899–1909. doi:10.1056/NEJMoa2103055.
- Huang B, Dai L, Wang H, et al. Neutralization of SARS-CoV-2 VOC 501Y.V2 by human antisera elicited by both inactivated BBIBP-CorV and recombinant dimeric RBD ZF2001 vaccines. bioRxiv: the preprint server for biology. 2021.
- Zhao X, Zheng A, Li D, et al. Neutralization of recombinant RBD-subunit vaccine ZF2001-elicited antisera to SARS-CoV-2 variants including Delta. bioRxiv: the preprint server for biology. 2021.
- Jangra S, Ye C, Rathnasinghe R, et al. SARS-CoV-2 spike E484K mutation reduces antibody neutralisation. Lancet Microbe. 2021;2(7):e283–e4. doi:10.1016/S2666-5247(21)00068-9.
- Deng X, Garcia-Knight MA, Khalid MM, et al. Transmission, infectivity, and antibody neutralization of an emerging SARS-CoV-2 variant in California carrying a L452R spike protein mutation. medRxiv: the preprint server for health sciences. 2021.
- Li Q, Wu J, Nie J, et al. The impact of mutations in SARS-CoV-2 spike on viral infectivity and antigenicity. Cell. 2020;182(5):1284–1294 e9. doi:10.1016/j.cell.2020.07.012.
- Farinholt T, Doddapaneni H, Qin X, et al. Transmission event of SARS-CoV-2 Delta variant reveals multiple vaccine breakthrough infections. medRxiv: the preprint server for health sciences. 2021.
- Gong W, Wu X. Is the tuberculosis vaccine BCG an alternative weapon for developing countries to defeat COVID-19? Indian J Tuberc. 2021;68(3):401–404. doi:10.1016/j.ijtb.2020.10.012.
- Qi S, XiaoPing D. Rapid global spread of the SARS-CoV-2 delta (B.1.617.2) variant: spatiotemporal variation and public health impact. Zoonoses. 2021;1.
- Emary KRW, Golubchik T, Aley PK, et al. Efficacy of ChAdOx1 nCoV-19 (AZD1222) vaccine against SARS-CoV-2 variant of concern 202012/01 (B.1.1.7): an exploratory analysis of a randomised controlled trial. Lancet. 2021;397(10282):1351–1362. doi:10.1016/S0140-6736(21)00628-0.
- Sadoff J, Gray G, Vandebosch A, et al. Safety and efficacy of single-dose Ad26.COV2.S vaccine against Covid-19. N Engl J Med. 2021;384(23):2187–2201. doi:10.1056/NEJMoa2101544.
- Thomas SJ, Moreira ED, Kitchin N, et al. Six month safety and efficacy of the BNT162b2 mRNA COVID-19 vaccine. medRxiv: the preprint server for health sciences. 2021.
- Ella R, Reddy S, Blackwelder W, et al. Efficacy, safety, and lot to lot immunogenicity of an inactivated SARS-CoV-2 vaccine (BBV152): a, double-blind, randomised, controlled phase 3 trial. medRxiv: the preprint server for health sciences. 2021.
- Novavax. Novavax COVID-19 vaccine demonstrates 89.3% efficacy in UK phase 3 trial. Novavax; 2021. https://ir.novavax.com/news-releases/news-release-details/novavax-covid-19-vaccine-demonstrates-893-efficacy-uk-phase-3.
- Baden LR, El Sahly HM, Essink B, et al. Efficacy and safety of the mRNA-1273 SARS-CoV-2 vaccine. N Engl J Med. 2021;384(5):403–416. doi:10.1056/NEJMoa2035389.
- Polack FP, Thomas SJ, Kitchin N, et al. Safety and efficacy of the BNT162b2 mRNA Covid-19 vaccine. N Engl J Med. 2020;383(27):2603–2615. doi:10.1056/NEJMoa2034577.
- Voysey M, Clemens SAC, Madhi SA, et al. Safety and efficacy of the ChAdOx1 nCoV-19 vaccine (AZD1222) against SARS-CoV-2: an interim analysis of four randomised controlled trials in Brazil, South Africa, and the UK. Lancet (London, England). 2021;397(10269):99–111. doi:10.1016/S0140-6736(20)32661-1.
- Voysey M, Costa Clemens SA, Madhi SA, et al. Single-dose administration and the influence of the timing of the booster dose on immunogenicity and efficacy of ChAdOx1 nCoV-19 (AZD1222) vaccine: a pooled analysis of four randomised trials. Lancet (London, England). 2021;397(10277):881–891. doi:10.1016/S0140-6736(21)00432-3.
- Stephenson KE, Le Gars M, Sadoff J, et al. Immunogenicity of the Ad26.COV2.S Vaccine for COVID-19. JAMA. 2021;325(15):1535–1544. doi:10.1001/jama.2021.3645.
- Tian JH, Patel N, Haupt R, et al. SARS-CoV-2 spike glycoprotein vaccine candidate NVX-CoV2373 immunogenicity in baboons and protection in mice. Nat Commun. 2021;12(1):372. doi:10.1038/s41467-020-20653-8.
- Guebre-Xabier M, Patel N, Tian JH, et al. NVX-CoV2373 vaccine protects cynomolgus macaque upper and lower airways against SARS-CoV-2 challenge. Vaccine. 2020;38(50):7892–7896. doi:10.1016/j.vaccine.2020.10.064.
- Mukhopadhyay L, Yadav PD, Gupta N, et al. Comparison of the immunogenicity & protective efficacy of various SARS-CoV-2 vaccine candidates in non-human primates. Indian J Med Res. 2021;153(1 & 2):93–114. doi:10.4103/ijmr.IJMR_4431_20.
- Keech C, Albert G, Cho I, et al. Phase 1-2 trial of a SARS-CoV-2 recombinant spike protein nanoparticle vaccine. N Engl J Med. 2020;383(24):2320–2332. doi:10.1056/NEJMoa2026920.
- Frenck RW, Jr, Klein NP, Kitchin N, et al. Safety, immunogenicity, and efficacy of the BNT162b2 Covid-19 vaccine in adolescents. N Engl J Med. 2021;385(3):239–250. doi:10.1056/NEJMoa2107456.
- World Health Organization. Tracking SARS-CoV-2 variants. https://wwwwhoint/en/activities/tracking-SARS-CoV-2-variants/. 2021.
- Collier DA, De Marco A, Ferreira I, et al. Sensitivity of SARS-CoV-2 B.1.1.7 to mRNA vaccine-elicited antibodies. Nature. 2021;593(7857):136–141. doi:10.1038/s41586-021-03412-7.
- Giles B, Meredith P, Robson S, et al. The SARS-CoV-2 B.1.1.7 variant and increased clinical severity-the jury is out. Lancet Infect Dis. 2021;21(9):1213–1214. doi:10.1016/S1473-3099(21)00356-X.
- Khan A, Zia T, Suleman M, et al. Higher infectivity of the SARS-CoV-2 new variants is associated with K417N/T, E484K, and N501Y mutants: an insight from structural data. J Cell Physiol. 2021;236(10):7045–7057. doi:10.1002/jcp.30367.
- Funk T, Pharris A, Spiteri G, et al. Characteristics of SARS-CoV-2 variants of concern B.1.1.7, B.1.351 or P.1: data from seven EU/EEA countries, weeks 38/2020 to 10/2021. Euro surveillance: bulletin. Europeen sur les maladies transmissibles = European Communicable Disease Bulletin. 2021;(16):2100348.
- Ramanathan M, Ferguson ID, Miao W, et al. SARS-CoV-2 B.1.1.7 and B.1.351 Spike variants bind human ACE2 with increased affinity. bioRxiv: the preprint server for biology. 2021.
- Mahase E. Covid-19: Novavax vaccine efficacy is 86% against UK variant and 60% against South African variant. BMJ. 2021;372:n296. doi:10.1136/bmj.n296.
- Sabino EC, Buss LF, Carvalho MPS, et al. Resurgence of COVID-19 in Manaus, Brazil, despite high seroprevalence. Lancet (London, England). 2021;397(10273):452–455. doi:10.1016/S0140-6736(21)00183-5.
- Charmet T, Schaeffer L, Grant R, et al. Impact of original, B.1.1.7, and B.1.351/P.1 SARS-CoV-2 lineages on vaccine effectiveness of two doses of COVID-19 mRNA vaccines: results from a nationwide case-control study in France. Lancet Reg Health Eur. 2021;8:100171. doi:10.1016/j.lanepe.2021.100171.
- Deng X, Garcia-Knight MA, Khalid MM, et al. Transmission, infectivity, and neutralization of a spike L452R SARS-CoV-2 variant. Cell. 2021;184(13):3426–3437.e8. doi:10.1016/j.cell.2021.04.025.
- World Health Organization. SARS-CoV-2 Delta variant now dominant in much of European region; efforts must be reinforced to prevent transmission, warns WHO Regional Office for Europe and ECDC. https://wwweurowhoint/en/media-centre/sections/press-releases/2021/sars-cov-2-delta-variant-now-dominant-in-much-of-european-region-efforts-must-be-reinforced-to-prevent-transmission,-warns-who-regional-office-for-europe-and-ecdc. 2021.
- American S. Why do variants such as delta become dominant? https://wwwscientificamericancom/article/why-do-variants-such-as-delta-become-dominant1/. 2021.
- Chen J, Wang R, Wang M, et al. Mutations strengthened SARS-CoV-2 infectivity. J Mol Biol. 2020;432(19):5212–5226. doi:10.1016/j.jmb.2020.07.009.
- Mlcochova P, Kemp SA, Dhar MS, et al. SARS-CoV-2 B.1.617.2 Delta variant replication and immune evasion. Nature. 2021;599(7883):114–119. doi:10.1038/s41586-021-03944-y.
- World Health Organization. Classification of omicron (B.1.1.529): SARS-CoV-2 variant of concern. https://wwwwhoint/news/item/26-11-2021-classification-of-omicron-(b11529)-sars-cov-2-variant-of-concern. 2021.
- Harrington D, Kele B, Pereira S, et al. Confirmed reinfection with severe acute respiratory syndrome coronavirus 2 (SARS-CoV-2) variant VOC-202012/01. Clin Infect Dis. 2021;73(10):1946–1947. doi:10.1093/cid/ciab014.
- Coutinho RM, Marquitti FMD, Ferreira LS, et al. Model-based estimation of transmissibility and reinfection of SARS-CoV-2 P.1 variant. Commun Med (Lond). 2021;1:48. doi:10.1038/s43856-021-00048-6.
- Shastri J, Parikh S, Aggarwal V, et al. Severe SARS-CoV-2 breakthrough reinfection with delta variant after recovery from breakthrough infection by alpha variant in a fully vaccinated health worker. Front Med (Lausanne). 2021;8:737007. doi:10.3389/fmed.2021.737007.
- Kreier F. ‘Unprecedented achievement’: who received the first billion COVID vaccinations? Nature. 2021. doi:10.1038/d41586-021-01136-2.
- Li Q, Lu H. Latest updates on COVID-19 vaccines. Biosci Trends. 2021;14(6):463–466. doi:10.5582/bst.2020.03445.
- Cantón R, De Lucas Ramos P, García-Botella A, et al. New variants of SARS-CoV-2. Rev Esp Quimioter. 2021;34(5):419–428. doi:10.37201/req/071.2021.
- Eaton L. Covid-19: WHO warns against "vaccine nationalism" or face further virus mutations. BMJ. 2021;372:n292. doi:10.1136/bmj.n292.
- Wouters OJ, Shadlen KC, Salcher-Konrad M, et al. Challenges in ensuring global access to COVID-19 vaccines: production, affordability, allocation, and deployment. Lancet (London, England). 2021;397(10278):1023–1034. doi:10.1016/S0140-6736(21)00306-8.
- Mills MC, Salisbury D. The challenges of distributing COVID-19 vaccinations. EClinicalMedicine. 2021;31:100674. doi:10.1016/j.eclinm.2020.100674.
- Yang J-R, Kuo C-Y, Huang H-Y, et al. Newly emerging mutations in the matrix genes of the human influenza A(H1N1)pdm09 and A(H3N2) viruses reduce the detection sensitivity of real-time reverse transcription-PCR. J Clin Microbiol. 2014;52(1):76–82. doi:10.1128/JCM.02467-13.
- Jindal H, Jain S, Suvvari TK, et al. False-negative RT-PCR findings and double mutant variant as factors of an overwhelming second wave of COVID-19 in India: an emerging global health disaster. SN Compr Clin Med. 2021;3(12):2383–2388. doi:10.1007/s42399-021-01059-z.
- Ascoli CA. Could mutations of SARS-CoV-2 suppress diagnostic detection? Nat Biotechnol. 2021;39(3):274–275. doi:10.1038/s41587-021-00845-3.
- MacDonald NE, SAGE Working Group on Vaccine Hesitancy. Vaccine hesitancy: definition, scope and determinants. Vaccine. 2015;33(34):4161–4164. doi:10.1016/j.vaccine.2015.04.036.
- Machingaidze S, Wiysonge CS. Understanding COVID-19 vaccine hesitancy. Nat Med. 2021;27(8):1338–1339. doi:10.1038/s41591-021-01459-7.
- Callaway E. Fast-spreading COVID variant can elude immune responses. Nature. 2021;589(7843):500–501. doi:10.1038/d41586-021-00121-z.
- Dan JM, Mateus J, Kato Y, et al. Immunological memory to SARS-CoV-2 assessed for up to 8 months after infection. Science (New York, NY). 2021;371(6529):371. doi:10.1126/science.abf4063.
- Wajnberg A, Amanat F, Firpo A, et al. Robust neutralizing antibodies to SARS-CoV-2 infection persist for months. Science. 2020;370(6521):1227–1230. doi:10.1126/science.abd7728.
- Pilz S, Chakeri A, Ioannidis JP, et al. SARS-CoV-2 re-infection risk in Austria. Eur J Clin Invest. 2021;51(4):e13520. doi:10.1111/eci.13520.
- Martin DP, Weaver S, Tegally H, et al. The emergence and ongoing convergent evolution of the N501Y lineages coincides with a major global shift in the SARS-CoV-2 selective landscape. medRxiv: the preprint server for health sciences. 2021.
- Murray CJL, Piot P. The potential future of the COVID-19 pandemic: will SARS-CoV-2 become a recurrent seasonal infection? JAMA. 2021;325(13):1249–1250. doi:10.1001/jama.2021.2828.
- Phillips N. The coronavirus is here to stay - Here’s what that means. Nature. 2021;590(7846):382–384. doi:10.1038/d41586-021-00396-2.
- Iftekhar EN, Priesemann V, Balling R, et al. A look into the future of the COVID-19 pandemic in Europe: an expert consultation. Lancet Reg Health Eur. 2021;8:100185. doi:10.1016/j.lanepe.2021.100185.
- Lurie N, Saville M, Hatchett R, et al. Developing Covid-19 vaccines at pandemic speed. N Engl J Med. 2020;382(21):1969–1973. doi:10.1056/NEJMp2005630.
- Amanat F, Krammer F. SARS-CoV-2 vaccines: status report. Immunity. 2020;52(4):583–589. doi:10.1016/j.immuni.2020.03.007.
- Dai L, Gao GF. Viral targets for vaccines against COVID-19. Nat Rev Immunol. 2021;21(2):73–82. doi:10.1038/s41577-020-00480-0.
- Katella K. Comparing the COVID-19 vaccines: how are they different? https://wwwyalemedicineorg/news/covid-19-vaccine-comparison. 2021.
- He Q, Mao Q, Zhang J, et al. COVID-19 vaccines: current understanding on immunogenicity, safety, and further considerations. Front Immunol. 2021;12:669339. doi:10.3389/fimmu.2021.669339.
- Dowdle WR. The principles of disease elimination and eradication. Bull World Health Organ. 1998;76(Suppl 2):22–25.
- Abdel-Moneim AS, Abdelwhab EM. Evidence for SARS-CoV-2 infection of animal hosts. Pathogens (Basel, Switzerland). 2020;9(7):529. doi:10.3390/pathogens9070529.
- Oude Munnink BB, Sikkema RS, Nieuwenhuijse DF, et al. Transmission of SARS-CoV-2 on mink farms between humans and mink and back to humans. Science. 2021;371(6525):172–177. doi:10.1126/science.abe5901.
- Chen J, Lu H. New challenges to fighting COVID-19: virus variants, potential vaccines, and development of antivirals. Biosci Trends. 2021;15(2):126–128. doi:10.5582/bst.2021.01092.
- Sanders JM, Monogue ML, Jodlowski TZ, et al. Pharmacologic treatments for coronavirus disease 2019 (COVID-19): a review. JAMA. 2020;323(18):1824–1836. doi:10.1001/jama.2020.6019.
- Deng Q, Rasool RU, Russell RM, et al. Targeting androgen regulation of TMPRSS2 and ACE2 as a therapeutic strategy to combat COVID-19. iScience. 2021;24(3):102254. doi:10.1016/j.isci.2021.102254.
- Shang C, Zhuang X, Zhang H, et al. Inhibitors of endosomal acidification suppress SARS-CoV-2 replication and relieve viral pneumonia in hACE2 transgenic mice. Virol J. 2021;18(1):46. doi:10.1186/s12985-021-01515-1.
- Khateeb J, Li Y, Zhang H. Emerging SARS-CoV-2 variants of concern and potential intervention approaches. Crit Care. 2021;25(1):244. doi:10.1186/s13054-021-03662-x.
- Wysocki J, Ye M, Hassler L, et al. A novel soluble ACE2 variant with prolonged duration of action neutralizes SARS-CoV-2 infection in human kidney organoids. JASN. 2021;32(4):795–803. doi:10.1681/ASN.2020101537.
- Abbott TR, Dhamdhere G, Liu Y, et al. Development of CRISPR as an antiviral strategy to combat SARS-CoV-2 and influenza. Cell. 2020;181(4):865–876.e12. doi:10.1016/j.cell.2020.04.020.
- Baum A, Ajithdoss D, Copin R, et al. REGN-COV2 antibodies prevent and treat SARS-CoV-2 infection in rhesus macaques and hamsters. Science. 2020;370(6520):1110–1115. doi:10.1126/science.abe2402.
- Anna RM, Hasan U, Liina H, et al. Intranasal inhibitor blocks omicron and other variants of SARS-CoV-2. Research Square. 2022. Preprint.
- Romano CM, Melo FL. Genomic surveillance of SARS-CoV-2: a race against time. Lancet Reg Health Am. 2021;1:100029. doi:10.1016/j.lana.2021.100029.
- Robishaw JD, Alter SM, Solano JJ, et al. Genomic surveillance to combat COVID-19: challenges and opportunities. Lancet Microbe. 2021;2(9):e481–e4. doi:10.1016/S2666-5247(21)00121-X.
- Jumper J, Evans R, Pritzel A, et al. Highly accurate protein structure prediction with AlphaFold. Nature. 2021;596(7873):583–589. doi:10.1038/s41586-021-03819-2.
- Oude Munnink BB, Worp N, Nieuwenhuijse DF, et al. The next phase of SARS-CoV-2 surveillance: real-time molecular epidemiology. Nat Med. 2021;27(9):1518–1524. doi:10.1038/s41591-021-01472-w.
- Limam A. What is a universal coronavirus vaccine and is it achievable? https://newseucgtncom/news/2021-03-21/What-is-a-universal-coronavirus-vaccine-and-is-it-achievable–YN8cpkETqU/indexhtml. 2021.
- Page ML. Covid-resistant people point way to universal coronavirus vaccine. https://wwwnewscientistcom/article/2296980-covid-resistant-people-point-way-to-universal-coronavirus-vaccine/. 2021.
- Swadling L, Diniz MO, Schmidt NM, et al. Pre-existing polymerase-specific T cells expand in abortive seronegative SARS-CoV-2. Nature. 2021;601(7891):110–117.
- CORONADX. Are we able to detect all coronavirus variants? https://coronadx-projecteu/magazine/are-we-able-to-detect-all-coronavirus-variants-2/. 2021.
- Aspatwar A, Gong W, Wang S, et al. Tuberculosis vaccine BCG: the magical effect of the old vaccine in the fight against the COVID-19 pandemic bioRxiv. 2021.
- Jimenez D. A century-old tuberculosis vaccine could help fight Covid-19 in the elderly. https://wwwpharmaceutical-technologycom/features/tuberculosis-vaccine-covid-19-elderly/. 2021.
- Amirlak L, Haddad R, Hardy JD, et al. Effectiveness of booster BCG vaccination in preventing Covid-19 infection. Hum Vaccines Immunother. 2021;17(11):3913–3915.
- Sadeghi R, Masoudi MR, Khanjani N. The commitment for fair distribution of COVID-19 vaccine among all countries of the world. Res Nurs Health. 2021;44(2):266–267. doi:10.1002/nur.22112.
- World Health Organization. Vaccine equity. https://wwwwhoint/campaigns/vaccine-equity. 2021.
- Crisis TC. Zoo animals are getting their COVID vaccines, too. https://wwwnprorg/2021/11/13/1055503677/zoo-animals-are-getting-their-covid-vaccines-too. 2021.
- Baum A, Fulton BO, Wloga E, et al. Antibody cocktail to SARS-CoV-2 spike protein prevents rapid mutational escape seen with individual antibodies. Science. 2020;369(6506):1014–1018. doi:10.1126/science.abd0831.