Abstract
Purpose. Radiocontrast agents (RAs) cause renal tubular damage by hemodynamic imbalance, which could cause hypoxic stimulus and direct cytotoxicity. However, reactive oxygen species (ROS) could be an important factor in RAs' direct cytotoxicity. This study investigated the involvement of ROS in deleterious effects produced by RAs on normoxic and hypoxic renal tubular cells. Materials and Methods. LLC-PK1 and MDCK were exposed to diatrizoate and ioxaglate in normoxic and hypoxic conditions. Apoptotic and necrotic cell death were assessed by acridine orange/ethidium bromide and annexin V methods. Hydrogen peroxide, superoxide anion, and malondialdehyde levels were analyzed by, respectively, 2′,7′-dichlorofluorescein, luminal, and thiobarbituric acid. Antioxidant agents were used to prevent cellular RAs damage. Results. Diatrizoate and ioxaglate decreased cellular viability in both cells, and this effect was enhanced by hypoxic conditions. Diatrizoate induced more injury than ioxaglate to both cell lines. LLC-PK1 underwent necrosis, while MDCK cells underwent apoptosis when exposed to diatrizoate. These results could not be attributed to an increase in osmolality. RAs did not increase hydrogen peroxide, superoxide anion or malondialdehyde levels in both cells. Additionally, N-acetyl-L-cysteine (NAC), ascorbic acid, α-tocopherol, glutathione, β-carotene, allopurinol, cimetidine, and citric acid did not protect cells against RAs damage. Surprising, NAC increased the cellular damage induced by ioxaglate in the both cell lines. Conclusion. The present study shows that RAs induce damage in cultured tubular cells, especially in hypoxic conditions. ROS were not involved in the observed RAs' cytotoxicity, and NAC increased ioxaglate-induced tubular damage.
INTRODUCTION
Iodine-derived radiocontrast agents (RAs) are used in angiography, urography, and computerized tomography. They absorb a relatively large amount of radiation, which is proportional to the concentration of iodine in the solution. The most common side effects to the use of these contrast agents include systemic hyper-sensibility and cardiocirculatory and renal adverse responses. RAs are already considered the second largest nephrotoxic agent used in the clinical practice. Some factors may increase the incidence of the contrast-induced nephropathy (CIN), such as diabetes and acute renal failure (ARF).Citation[1–3] The importance of CIN study is that the exams utilizing RAs are commonly used in elderly patients with several risk factors. The incidence of CIN can range from 1% in non-selected populations to more than 50% in patients with known risk factors.Citation[4]
The use of RAs triggers a renal biphasic hemodynamic response. A period of vasodilatation that lasts a few seconds is followed by vasoconstriction that reduces kidney blood flow, glomerular filtration rate, and oxygen partial tension mainly in the medulla of the kidney.Citation[5],Citation[6] Furthermore, RAs exert direct toxicity on renal cells. Some studies suggest the generation of ROS as an important mechanism of direct RAs cytotoxicities.Citation[7],Citation[8]
The recommended clinical procedure used to reduce RA-induced damage to the kidneys is hydrating the patient before the administration of the RAs.Citation[9–12] Recently, N‐acetyl-cysteine, an antioxidant agent, has been used for RAs nephrotoxicities preventions.Citation[13],Citation[14] The present study investigated the involvement of ROS in the mechanism of the direct cytotoxicity produced by sodium diatrizoate and sodium meglumine ioxaglate exposure. In addition, we have tested the possible protective effect of antioxidant agents. Because RA‐induced vasoconstriction may cause tubular hypoxia, RAs were also added to tubular cells during hypoxic condition.
MATERIALS AND METHODS
Cell Culture
The Lewis Lung Cancer-Porcine Kidney (LLC-PK1) cell line is derived from epithelial renal cells of Hampshire pig and has tubular proximal phenotype.Citation[15] The Madin-Darby Canine Kidney (MDCK) cell line is originally derived from dog kidney and has several tubular distal characteristics.Citation[16] Both cell lines were grown in Dulbeco's Modified Eagle Medium (DMEM, Sigma) with the addition of 5% of fetal bovine serum (FBS, Gibco), 24 mM of NaHCO3, 10 mM of HEPES, and 10 000 U/L of penicillin/streptomycin. The culture was incubated at 37°C in a humidified atmosphere containing 5% carbon dioxide.
At semi-confluence stage, cells were detached from plastic flasks utilizing trypsin (0.05%), centrifuged, resuspended in DMEM, and subcultured in 25 cm2 culture plastic flasks for the experimental procedures.
Hypoxia was obtained by injecting a gaseous mixture containing 95% nitrogen and 5% carbon dioxide in the culture flasks during 20 minutes. After this period, the culture flasks were kept with tight plugs in incubator at 37°C for 24 hours.
Radiocontrast Agents
Radiocontrast agents normally used in radiographic examinations were studied, namely, sodium diatrizoate (diatrizoate), trade name Urographing 370 (Schering AG, Berlin, Germany), a high-osmolality ionic contrast medium; and sodium meglumine ioxaglate (ioxaglate), trade name Hexabrix 320 (Guerbet, Roissy CDG, France), a low-osmolality contrast medium. Diatrizoate was used at final concentration of 6% (22 mg of iodine per ml) and ioxaglate was used at final concentration of 10% (32 mg of iodine per ml). Results previously obtained have shown that these concentrations represent the minimum concentration of each RA required to cause cellular damage in this study's experimental model.
The culture medium was carefully aspirated, and diatrizoate or ioxaglate diluted in 5 ml of DMEM without FBS was added to treat the cell culture for 24 hours at 37°C. After this treatment, the cells were washed and submitted to the various assays described below.
Antioxidant Agents
N-acetyl-L-cysteine was added to the culture medium for 48 hours in cell cultures. In experimental groups, diatrizoate (6%) or ioxaglate (10%) was added 24 hours after the incubation with NAC, and cells were kept in culture during the next 24 hours, totaling the whole period 48 hours. NAC was used at final concentration of 50 μM and higher concentrations did not show any additional information.
Other antioxidant agents such as vitamin C (ascorbic acid, 1.0 mM), vitamin E (α-tocopherol, 0.5 mM), glutathione (1.0 mM), β-carotene (0.1 μM), allopurinol (xanthine-oxidase inhibitor, 0.5 mM), cimetidine (cytochrome P-450 inhibitor, 0.7 mM) and citric acid (2.0 mM) were also tested and used according to the method described previously. These agents have been currently considered as ROS scavengers in others experimental models.Citation[17–20] All antioxidant agents were purchased from Sigma.
Raffinose
The diatrizoate addition in the culture medium at final concentration of 6% enhances the osmolality to 82 mOsm/kg, while ioxaglate (10%) enhances the osmolality to 47 mOsm/kg. In order to evaluate osmolality factor, raffinose at final osmolality 82 mOsm/kg or 47 mOsm/kg was added to cell cultures for 24 hours as RAs exposure, respectively.
Lactate Dehydrogenase (LDH) Release
Cell membrane damage can be evaluated by the release of the intracellular enzyme lactate dehydrogenase (LDH), currently used as an important damage marker of plasmatic membrane.Citation[21]
Culture medium and cell homogenate samples of cell cultures treated with diatrizoate (6%) or ioxaglate (10%) were collected. Culture flasks cells were scrapped with PBS obtaining cell homogenates. Sodium pyruvate (1%), NADH (0.2 mM), Tris HCl (0.1 M) and ethylenediaminetetraacetic acid (EDTA, 0.5 mM) were added to the samples. Enzymatic activity was calculated by measuring the samples absorbance at 380 nM in a spectrophotometer Spectronic Instruments model Spectronic Genesys II. The ratio between culture medium and cellular homogenates LDH levels were obtained, and results were reported as percentage of control values.
Cell Viability Assay
Violet Crystal
The cell lines were cultured in 96-well culture microplate. After the exposure to RAs and antioxidant agents, 20 μL of a solution containing 30% acetic acid and 1% violet crystal was added to each well and incubated for 15 minutes. The plate was then gently washed and left to dry at 37°C before receiving 100 μL of methanol per well. Absorbance was measured at 570 nm on a microtiter plate reader.
Acridine Orange and Ethidium Bromide Labeling
Cellular viability was assessed by acridine orange and ethidium bromide label. Ethidium bromide stains all cells in green, while acridine orange is excluded from viable, staining only permeable cells in orange.
Cell samples (10 μl) of treated cultures with diatrizoate (6%) or ioxaglate (10%) were incubated with 0.3 μl of a solution containing acridine orange and ethidium bromide, both at 50 μg/mL, and examined under fluorescent light on an Olympus BX60 fluorescence microscope. In each sample, a total of 100 to 200 cells were counted in order to determine the percent rate of viable cells.
Necrosis/Apoptosis Rate
Cells undergoing apoptosis display phosphatidylserine (PS) in the surface of plasmatic membrane. Detection of PS was carried out by annexin V-FITC, and propidium iodide was used to distinguish between cells undergoing necrosis and those undergoing apoptosis. Later, apoptotic cells were labeled with both stains. BD Pharmingen kits with propidium iodide (PI) and annexin V‐FITC were used.Citation[22] The necrosis/apoptosis rate was determined by fluorescence-activated cell sorting (FACS).
The cell cultures treated with diatrizoate (6%) or ioxaglate (10%) were detached from culture flasks, counted (1 × 105 cells), collected by centrifugation in annexin buffer solution (BD Pharmingen, New Jersey, USA) and stained with annexin V-FITC (10 μL) and PI (4 μL). Analysis of labeled cells was performed by a FACS Caliber flow cytometer (Becton & Dickinson, New Jersey, USA). The results were expressed in percentage of annexin V-FITC and PI-labeled cells.
Determination of Reactive Oxygen Species
Hydrogen Peroxide
Hydrogen peroxide production were determined by using 2′,7′-dichlorofluorescein (DCFH) (Sigma Chemical Co, St. Louis, Missouri, USA) that undergoes oxidation and becomes fluorescentCitation[23] in the presence of hydrogen peroxide.
Cell cultures treated with diatrizoate (6%) or ioxaglate (10%) were detached from culture flasks, counted (1 × 106 cells), and resuspended in 800 μL of PBS. A solution of DCFH (200 μL) at final concentration 20 μmol/mL were added to the samples and incubated with agitation at 37°C for 30 minutes. The cells were centrifuged, resuspended in EDTA, and carried out for FACS analysis.
Superoxide Anion
The level of superoxide anion was determined by luminol (5-amino-2,3-dihydro-1,4-phenylazinedione; Sigma Chemicals Co., St Louis, Missouri, USA), which becomes fluorescent when combined with this specific ROS.
The tubular cells were detached from culture flasks and counted (1 × 106 cells per sample). Luminol (10−4 M) and RAs (diatrizoate at 6% and ioxaglate at 10%) were added to the samples. The reading were performed by a Bio Orbit 1251 luminometer and started immediately after the addition of RAs. The level of superoxide anion was continually assessed for the next 30 minutes, initially. The reading was extended for ninety minutes.
Lipid Peroxidation
The level of lipid peroxidation was determined by the malondialdehyde (MDA) quantification. The MDA combines with thiobarbituric acid (TBA) forming a red compound, the concentration of which can be assessed easily by spectrophotometry at 535 nm.Citation[24]
Culture medium and cell homogenate samples of cell cultures treated with diatrizoate (6%) or ioxaglate (10%) were collected. Scrapping cells from the flasks with PBS obtained the cell homogenates. Samples were added to a solution of 0.375% TBA, 15% trichloroacetic acid and 0.25 N HCl, kept in continual agitation, heated in 95oC for 20 minutes and subsequently cooled down by itself for room temperature. The assessment of protein level in the solution was carried out by the Bradford method.Citation[25] The positive control was obtained by incubating the cell samples with H2O2 at 1% for one hour before the assay.
Statistical Analysis
Data were expressed as mean ± SEM (standard error mean). Experimental and control groups were compared by Students' t test. Significance level for nullity hypothesis was set at 5% (p < 0.05).
RESULTS
Cellular Damage after Exposure of LLC-PK1 and MDCK Cells to RA and Hypoxia
shows the cellular damage caused by the exposition to ioxaglate (10%) or diatrizoate (6%), associated or not with hypoxia during 24 hours. Both RAs decreased cellular viability and had their effect increased by hypoxic conditions.
Figure 1. Cell viability assessed by acridine orange and ethidium bromide labeling in LLC-PK1 and MDCK cell lines. Briefly, semi-confluent LLC-PK1 or MDCK cells were treated with ioxaglate (10%, 32 mg of iodine per mL), raffinose 47 mOsm/kg (osmolality control of ioxaglate at 6%), diatrizoate (6%, 22 mg of iodine per mL) or raffinose 82 mOsm/kg (osmolality control of diatrizoate at 10%) for 24 hours. Additionally, exposure to hypoxia were performed to both cell lines by injecting a gaseous mixture of 95% of nitrogen and 5% of carbon dioxide during 20 minutes and maintaining for 24 hours, concomitantly to the chemical treatments. After this period, cells were detached from culture flasks and incubated with 0.3 μl of a solution containing acridine orange and ethidium bromide, both at 50 μg/mL, and examined under fluorescent light. In each sample, a total of 100–200 cells were counted in order to determine the percent rate of viable cells. Data are expressed as mean ± SEM. The bars assigned are significantly different from the respective control condition.
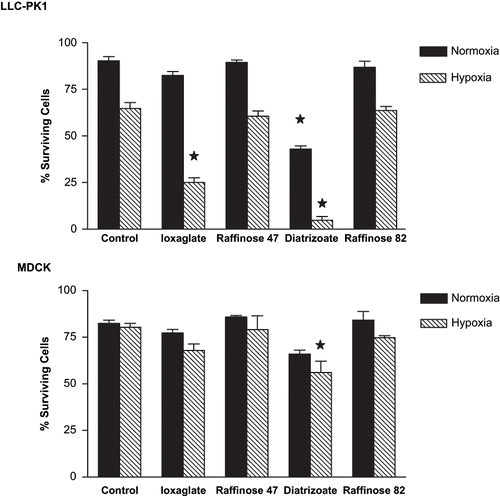
Diatrizoate (6%) enhanced the osmolality in 82 mOsm/kg, while ioxaglate (10%) enhanced the osmolality in 47 mOsm/kg. Raffinose 82 mOsm/kg and 47 mOsm/kg, used as osmolality control, did not induce cell death in normoxic or hypoxic conditions ().
shows the effect of ioxaglate (10%) or diatrizoate (6%) exposition, associated or not with gaseous hypoxia, during 24 hours on plasmatic membrane.
Figure 2. Plasmatic membrane integrity evaluation in LLC-PK1 and MDCK cells by lactate dehydrogenase (LDH) release. Briefly, semi-confluent LLC-PK1 or MDCK cells were treated with ioxaglate (10%, 32 mg of iodine per mL), diatrizoate (6%, 22 mg of iodine per mL), raffinose 47 mOsm/kg (osmolality control of ioxaglate 10%), or raffinose 82 mOsm/kg (osmolality control of diatrizoate at 6%) for 24 hours. Additionally, exposure to hypoxia were performed to both cell lines by injecting a gaseous mixture of 95% of nitrogen and 5% of carbon dioxide during 20 minutes and maintaining for 24 hours, concomitantly to the radiocontrast agents' exposure. After this period, supernatant culture medium and cell homogenate samples (obtained by scrapping cells with PBS) were collected. Sodium pyruvate (1%), NADH (0.2 mM), Tris HCl (0.1 M), and EDTA (0.5 mM) were added to the samples. Enzymatic activity was calculated by measuring the increase in absorbance at 380 nM in a spectrophotometer and reported as percentage of control values. Data are expressed as mean ± SEM. The bars assigned are significantly different from the respective control condition.
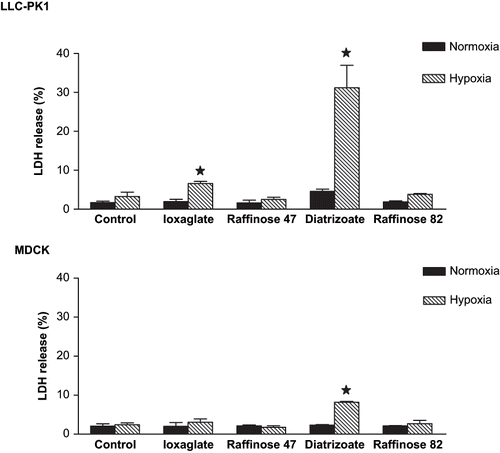
No differences were observed in LDH levels after RA exposure in MDCK under normoxic conditions, but ioxaglate (10%) and diatrizoate (6%) exposure enhanced LDH levels in LLC-PK1 cells.
Under hypoxic conditions, diatrizoate (6%) and ioxaglate (10%) caused a significant increase in LDH released to the culture medium in LLC-PK1, while only diatrizoate exposition increased LDH levels in culture medium on MDCK. Raffinose 82 mOsm/kg and 47 mOsm/kg, used as (respectively) diatrizoate (6%) and ioxaglate (10%) osmolality control, did not induce LDH released to the culture medium in both cell lines under normoxic or hypoxic conditions (see ).
Under hypoxic conditions, LLC-PK1 cells underwent necrosis (16.1%), whereas MDCK cells underwent apoptosis (22.1%) when exposed to diatrizoate (6%).
Reactive Oxygen Species
In order to investigate the cellular damage induced by RAs and hypoxia in LLC-PK1 and MDCK that could be attributed to ROS, hydrogen peroxide, superoxide anion, and lipidic peroxidation were evaluated.
shows the hydrogen peroxide levels in LLC-PK1 and MDCK cells after 24 hours exposition to diatrizoate (6%) and ioxaglate (10%). The incubation with RA did not increase the level of hydrogen peroxide in both cell lines.
Table 1 Hydrogen peroxide production by LLC-PK1 and MDCK cells exposed to ioxaglate (10%) and diatrizoate (6%)
The exposition of LLC-PK1 and MDCK to RA decreased cellular viability. Because non-viable cells are not able to produce ROS, a reduction in exposition period was carried out to assess ROS production.
Tubular cells were exposed to diatrizoate (6%) during 1 and 3 hours, and concomitant assessment of cell viability was performed using annexin V and propidium iodide (see ) . In this condition, cellular viability rates were similar to the control cultures, and no increase in hydrogen peroxide production after 1 or 3 hours exposition to diatrizoate (6%) was observed (not shown).
Figure 3. Necrosis and apoptosis assessment by annexin V and propidium iodide in LLC-PK1 cells exposed to hypoxia only; LLC-PK1 cells exposed to hypoxia and diatrizoate (6%); MDCK cells exposed to hypoxia only; MDCK cells exposed to hypoxia and diatrizoate (6%). Briefly, cells were treated with diatrizoate (6%, 22 mg of iodine per mL) for 24 hours. Additionally, exposure to hypoxia was performed to both cell lines by injecting a gaseous mixture of 95% of nitrogen and 5% of carbon dioxide during 20 minutes and maintaining for 24 hours, concomitantly to the diatrizoate exposure. After this period, cells were detached from culture flasks, counted (1 × 105 cells), collected by centrifugation in annexin buffer solution, and stained with annexin V-FITC (10 μL) and PI (4 μL). Analysis of the labeled cells was performed by a flow cytometer. Data are expressed in percentage of annexin V-FITC- and PI-labeled cells. The results represent at least four independent assays.
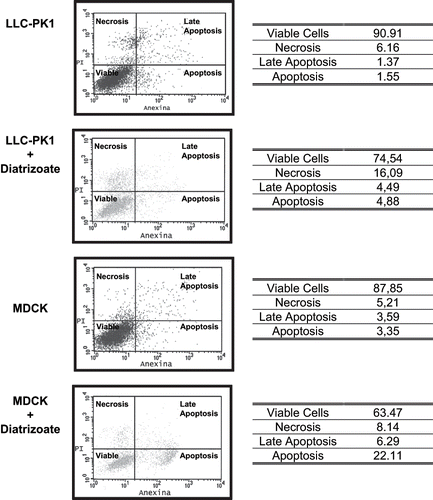
shows the superoxide anion production induced by exposition to diatrizoate (6%) and ioxaglate (10%) evaluated in LLC-PK1 cells during 30 minutes. No variation in superoxide anion level was detected. Additionally, no variation in superoxide anion production was observed when the reading period was extended to 90 minutes. The same pattern of superoxide production was observed when MDCK cells were exposed to diatrizoate (6%) and ioxaglate (10%) (data not shown).
Figure 4. Superoxide anion levels in LLC-PK1 cells exposed to diatrizoate (6%, 22 mg of iodine per mL) or ioxaglate (10%, 32 mg of iodine per mL). Briefly, cells were detached from culture flasks and counted (1 × 106 cells per sample). Luminol (10−4 M) and radiocontrast agents were added to the samples. The reading were performed by a luminometer and started immediately after the addition of diatrizoate and ioxaglate. The level of superoxide anion was continually assessed for the next 30 minutes.
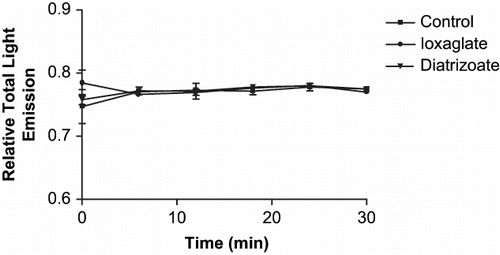
shows the lipidic peroxidation after 24-hour exposure to diatrizoate (6%) under normoxic or hypoxic conditions assessed by MDA production in LLC-PK1 and MDCK.
Figure 5. Effect of diatrizoate (6%) on lipidic peroxidation in LLC-PK1 and MDCK cells. Briefly, semi-confluent cells were treated with sodium diatrizoate (6%) for 24 hours. Additionally, exposure to hypoxia was performed to both cell lines by injecting a gaseous mixture of 95% of nitrogen and 5% of carbon dioxide during 20 minutes and maintaining for 24 hours, concomitantly to the diatrizoate exposure. After this period, supernatant culture medium and cell homogenate samples were collected. Samples were added to a solution of 0.375% TBA, 15% trichloroacetic acid, and 0.25 N HCl, kept in continual agitation, heated in 95°C for 20 minutes and subsequently cooled down by itself for room temperature. Malondialdehyde (MDA) levels were calculated by measuring the samples absorbance at 535 nm. The positive control was obtained by incubating the cell samples with hydrogen peroxide (1%) for one hour before the assay. Data are expressed as mean ± SEM. The bars assigned are significantly different from values obtained in control condition.
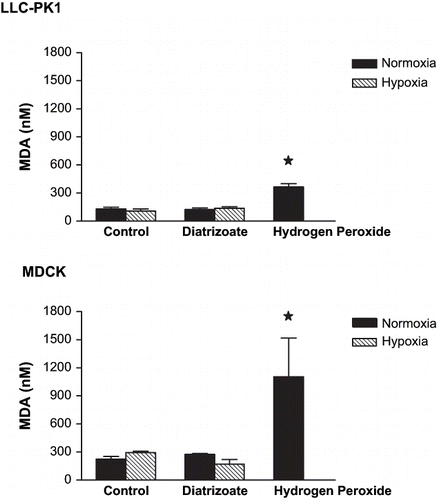
No increase in MDA level was detected after the exposure to diatrizoate (6%) under normoxic or hypoxic conditions. The same pattern of MDA production was observed when both cell lines were exposed to ioxaglate (10%) (not shown).
Antioxidant Agents
LLC-PK1 and MDCK cells were incubated with NAC and several antioxidant agents (ascorbic acid, allopurinol, cimetidine, α-tocopherol, citric acid, glutathione and β-carotene) in an attempt to test their protector effects against damage induced by exposition to RA.
shows the effects of NAC (50 μM) on the cellular death induced by ioxaglate (10%) in LLC-PK1 and MDCK cells. Tubular cells were exposed to NAC during 24 hours and after this period to NAC and ioxaglate during the next 24 hours, totaling 48 hours. The viability of LLC-PK1 and MDCK cell lines decreased significantly when exposed to ioxaglate (39.9% and 58.5% of cell death, respectively), but NAC increased the cell death induced by ioxaglate in both cell lines (57.8% and 80.2%, respectively).
Figure 6. Viability of LLC-PK1 and MDCK cells performed by violet crystal for assessment of NAC effects. Briefly, cell lines cultivated 96-well culture microplate was exposed to ioxaglate (10%, 32 mg of iodine per mL) for 24 hours (ioxaglate) and to NAC (50 μM) for 48 hours (NAC). Additionally, ioxaglate (10%) was added 24 hours after the incubation with NAC and cells were kept in culture during the next 24 hours, totaling the whole period 48 hours (ioxaglate + NAC). After this period, 20 μL of a solution containing 30% acetic acid and 1% violet crystal were added to each well and incubated for 15 minutes. The plate was washed and left to dry at 37°C before receiving 100 μL of methanol per well. Absorbance was measured at 570 nm on a microtiter plate reader. Data are expressed in mean percentage ± SEM. The stars mean statistic difference between the bars.
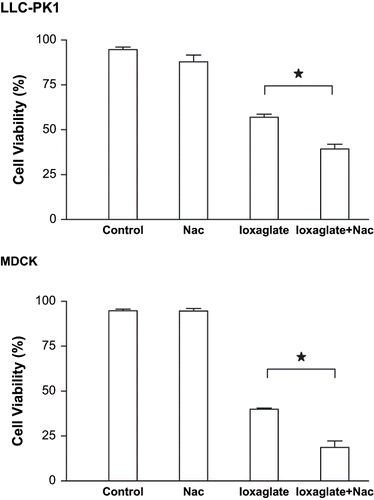
shows the antioxidant agents' exposition during the effect induced by ioxaglate in LLC-PK1. Previously, it was observed that diatrizoate (6%) interfered with the reading of the absorbance, assessed by violet crystal, so the antioxidant agent exposition was studied only under ioxaglate (10%) effects on cellular viability. Additionally, we verified that higher concentrations of each antioxidant could trigger intrinsic cytotoxicity and interfere with the results, so the concentrations showed in are the highest concentrations with no intrinsic cytotoxicity observed in both cell lines. None of the antioxidant agents induced any effect in viability or prevented cellular damage caused by ioxaglate (10%) in LLC-PK1.
Table 2 Antioxidant effects on viability of LLC-PK1 cells exposed to ioxaglate
DISCUSSION
In clinical practice, RAs (diatrizoate and ioxaglate) are administered in doses of 1.5 mL/kg, inducing a plasmatic iodine concentration of approximately 10 mg/mL. However, during the transport through the nephron, the concentration of iodine can easily increase two or three times (22 mgI/mL or 32 mgI/mL) in tubular fluid. In the specific case of RAs, urinary concentrations of 120 mg/mL of iodine in rats receiving clinically significant RA doses were found.Citation[26]
The present study indicates that diatrizoate (6%, 22 mg of iodine per ml) and ioxaglate (10%, 32 mg of iodine per ml), especially in hypoxic conditions, induced damage in LLC-PK1 and MDCK. The exposure of tubular epithelial cells to diatrizoate decreased cell viability in both cell lines and enhanced the release of LDH in LLC-PK1. The effects of both RAs were enhanced when hypoxia was associated. Other studies also indicated that RAs induce damage in tubular cell lines.Citation[27],Citation[28]
MDCK seems to be more resistant to RAs and hypoxic conditions than LLC-PK1. Additionally, MDCK cells underwent apoptosis while LLC-PK1 cells underwent necrosis when exposed to diatrizoate and hypoxia, the most cytotoxic experimental condition. Other reports also showed that MDCK cells are more resistant to anoxia than proximal tubular epithelial cells.Citation[29] Distal tubular cells are exposed to higher osmolality than proximal tubular cells. Additionally, proximal tubular cells show intense ATP-dependent activity, so it is reasonable to presume that LLC-PK1 are more sensitive to hypoxic conditions than MDCK cells. The present data obtained in vitro could reflect the different susceptibility of each portion of the nephron to RAs and hypoxia.
Diatrizoate exposition was more harmful than ioxaglate to both cell lines, especially under hypoxic conditions. It was reported that 20mgI/mL diatrizoate was more cytotoxic than the same concentration of ioxaglate. This effect was independent of the osmolality.Citation[30] This disparity could be attributed to others' physicochemical properties of both molecules as fluid viscosity, but the specific molecular mechanism remain to be determined.
The osmolality did not play a relevant role in RA-induced cytotoxicity in our model, as there was no difference in cellular viability and LDH release when the culture medium osmolality of tubular cells was increased by raffinose. The role of osmolality in CIN has been previously reported in experimental models; however, the osmolality levels were higher than those used in the present study (47 and 82 mOsm/Kg). Hizoh et al. related DNA fragmentation in MDCK cells exposed to 640 mOsm/Kg.Citation[28] Hardiek et al. related slight osmolality damage in LLC-PK1 cells exposed to 450 mOsm/Kg.Citation[31] Nevertheless, osmolality could not be pointed out as the unique mechanism in the RAs cytotoxicity, even at 2000 mOsm/Kg.Citation[32]
The results clearly indicate that ROS are not involved in RAs cytotoxicity in the in vitro model. RAs did not enhance ROS production or lipidic peroxidation in either cell line. There was no increase in hydrogen peroxide and superoxide anion when tubular cells were exposed to diatrizoate or ioxaglate, and there was no difference in malondialdehyde levels when LLC-PK1 and MDCK were exposed to diatrizoate. Additionally, antioxidant agents could not prevent the injury caused by RAs in epithelial cells. Despite that, Drager et al. showed that NAC blocked the increase in the urinary excretion of F2-isoprostane, as a measure of lipid peroxidation caused by RA administration. The possible ROS production and oxidative stress induced by RA administration in vivo cannot be excluded. The associated inflammatory process induced by RA exposition could have induced it.
RA cytotoxicity might be caused by the direct effect of these agents on plasmatic and mitochondrial membranes.Citation[27] Actually, Zager et al. found an increase of 15-KDa cytochrome-c in cell cultures exposed to RA, suggesting mitochondrial membrane injury. In the same way, they detected cytoplasmatic membrane proteins such as NaK-ATPase and caveolin, signaling damage to the plasmatic membrane.
Our findings indicate that antioxidant agents did not protected epithelial cells against damage caused by RAs. On the contrary, NAC increased the cellular damage induced by ioxaglate in both cell lines. Some reports indicated that MDCK cells exposed to NAC and diatrizoate showed increased levels of apoptosis and DNA fragmentation.Citation[26] It was reported that NAC, but not α‐tocopherol, enhanced apoptosis induced by hypoxia in murine fibroblasts, human pancreatic cancer cells, and human lung carcinoma cells by inhibition of nuclear factor NFkappaB.Citation[33] The mechanism involved in the cytotoxicity observed with NAC and ioxaglate remains to be determined.
Several experimental models point out NAC as a promising cytoprotective drug,Citation[34],Citation[35] and clinical studies suggest that NAC can prevent CIN.Citation[13],Citation[14] Under in vivo conditions, NAC may act on others factors not present in the in vitro model. NAC can act via hemodynamic balance, forming a synthetic variation of the S-nitrosothiol compound, the S-nitroso-N-acetylcysteine (SNAC). SNAC showed a potent vasodilatate effect in hypertensive and normotensive rats,Citation[36] and it is well defined that RA triggers a vasoconstrictor effect that reduces kidney blood flow, glomerular filtration rate, and oxygen partial tension, mainly in the medulla of the kidney.Citation[5],Citation[6] However, there are controversies about the potential protective role of NAC. A study on mice and human proximal tubular cell lines showed that NAC does not prevent the decrease in cellular viability, the increase in DHL release, or induced lipid peroxidation after RA exposure.Citation[27] Zager et al. suggested that the injury caused by RA is not due to oxidative stress, at least in vitro. Another clinical study shows that protective action of NAC occurred when patients received small amounts of RA, but not when the patients received higher doses.Citation[37] Other studies did not show any protective effect after the administration of NAC.Citation[38–40] Further studies are fundamental to establish the effect of NAC on CIN.
CONCLUSION
The present study indicates that diatrizoate and ioxaglate induced damage in LLC-PK1 and MDCK, especially in hypoxic conditions. Diatrizoate exposure produces different effects in proximal and distal tubular cells. ROS formation is not involved in RA-induced cytotoxicity, at least in vitro. Antioxidant agents did not prevent RA-induced tubular cell injury, and NAC actually increased the cellular damage of RA. Additional studies are necessary to clarify the mechanism of cytotoxicity induced by RA and the preventive effect of NAC.
REFERENCES
- Davidson CJ, Hlatky M, Morris KG, Pieper K, Skelton TN, Schwab SJ, Bashore TM. Cardiovascular and renal toxicity of a nonionic radiographic contrast agent after cardiac catheterization: a prospective trial. Ann Intern Med. 1989; 110: 119–124
- Parfrey PS, Griffiths SM, Barrett BJ, Paul MD, Genge M, Withers J, Farid N, McManamon PJ. Contrast material-induced renal failure to patients with diabetes mellitus, renal insufficiency, or both: a prospective controlled study. N Engl J Med. 1989; 320: 143–149
- Rudnick MR, Berns JS, Cohen RM, Goldfarb S. Contrast media associated nephrotoxicity. Semin Nephrol. 1997; 17: 15–26
- Waybill MM, Waybill PN. Contrast media-induced nephrotoxicity: identification of patients at risk and algorithms for prevention. Vasc Interv Radiol. 2001; 12: 3–9
- Weisberg LS, Kurnik PB, Kurnik BR. Radiocontrast-induced nephropathy in humans: Role of vasoconstriction. Kidney Int. 1992; 41: 1408–1415
- Tumlin JA, Wang A, Murray PT, Mathur VS. Fenoldopam mesylate blocks reductions in renal plasma flow after radiocontrast dye infusion: A pilot trial in the prevention of contrast nephropathy. Am Heart J. 2002; 143: 894–903
- Wasaki M, Sugimoto J, Shirota K. Glucose alters the susceptibility of mesangial cells to contrast media. Invest Radiol. 2001; 7: 355–362
- Katholi RE, Woods WT Jr, Taylor GJ, Deitrick CL, Womack KA, Katholi CR, McCann WP. Oxygen free radicals and contrast nephropathy. Am J Kidney Dis. 1998; 32: 64–71
- Agrawal M, Stouffer GA. Contrast induced nephrotoxicity after angiography. Am Med Sci. 2002; 323: 252–258
- Murphy SW, Barrett BJ, Parfrey PS. Contrast nephropathy. J Am Soc Nephrol. 2000; 11: 177–182
- Solomon R, Werner C, Mann D, D'Elia J, Silva P. Effects of saline, mannitol, and furosemide on acute decreases in renal function induced by radiocontrast agents. N Engl J Med. 1994; 331: 1416–1420
- Trivedi HS, Moore H, Nasr S, Aggarwal K, Agrawal A, Goel P, Hewett J. A randomized prospective trial to assess the role of saline hydration on the development of contrast nephrotoxicity. Nephron Clin Pract. 2003; 93: C29–C34
- Tepel M, van der Giet M, Schwarzfeld C, Laufer U, Liermann D, Zidek W. Prevention of radiographic-contrast-agent-induced reductions in renal function by acetylcysteine. N Engl J Med. 2000; 343: 180–184
- Drager LF, Andrade L, Barros de Toledo JF, Laurindo FR, Machado Cesar LA, Seguro AC. Renal effects of N-acetyl‐cysteine in patients at risk for contrast nephropathy: decrease in oxidant stress-mediated renal tubular injury. Nephrol Dial Transplant. 2004; 19: 1803–1807
- Hull RN, Cherry WR, Weaver GW. The origin and characteristics of a pig kidney cell strain, LLC-PK1. In Vitro. 1976; 12: 670
- American Type Culture Collection. Catalogue of Cell Lines and Hybridomas 7th, R Hay, M Mary, TR Chen, P McClintock, Y Reid. ATCC Press, Rockville, Md. 1992; 21
- Peuchant E, Delmas-Beauvieux MC, Duborg L, Thomas MJ, Peeromat A, Aparicio M, Clerc M, Combe C. Antioxidant effects of a supplemented very low protein diet in chronic renal failure. Free Radic Biol Med. 1997; 22: 131–320
- Rhoden E, Teloken C, Lucas M, Rhoden C, Mauri M, Zettler C, Bello-Klein A, Barros E. Protective effect of allopurinol in the renal ischemia-reperfusion in uninephrectomized rats. Gen Pharmacol. 2000; 35: 189–193
- Byer K, Khan SR. Citrate provides protection against oxalate and calcium oxalate crystal induced oxidative damage to renal epithelium. J Urol. 2005; 173: 640–646
- Dinu D, Nechifor MT, Movileanu L. Ethanol-induced alterations of the antioxidant defense system in rat kidney. J Biochem Mol Toxicol. 2005; 19: 386–395
- Yang CC, Hsu SP, Wu MS, Chien CT. Effects of vitamin C infusion and vitamin E-coated membrane on hemodialysis-induced oxidative stress. Kidney Int. 2006; 69: 706–714
- Zimmerhackl LB, Mesa H, Kraemer F, Koelmel C, Wiegele G, Brandis M. Tubular toxicity of cyclosporine A and the influence of endothelin-1 in renal cell culture model (LLC-PK1 and MDCK). Pediatr Nephrol. 1997; 11: 778–783
- Vermes I, Haanen C, Steffens-Nakken H, Reutelingsperger C. A novel assay for apoptosis—flow cytometric detection of phosphatidylserine expression on early apoptotic cells using fluorescein labeled Annexin V. J Immunol Methods. 1995; 184: 39–51
- Ubezio P, Civoli F. Flow cytometric detection of hydrogen peroxide production induced by doxorubicin in cancer cells. Free Radic Biol Med. 1994; 16: 509–516
- Hart MC, Tolson JK, Blocks ER. Supplemental fatty acids alter lipid peroxidation and oxidant injury in endothelial cells. Am J Physiol. 1991; 260: L481–L488
- Bradford MM. A rapid and sensitive method for the quantitation of microgram quantities of protein utilizing the principle of protein-dye binding. Anal Biochem. 1976; 72: 248–254
- Beaufils H, Idee JM, Berthommier C, Balut C, Bourbouze R, Nimier K, Chicandre-Jouanneau C, Bonnemain B. Iobitridol, a new nonionic low-osmolality contrast agent, and iohexol: impact on renal histology in the rat. Invest Radiol. 1995; 30: 33–39
- Zager RA, Johnson ACM, Hanson SY. Radiographic contrast media-induced tubular injury: Evaluation of oxidant stress and plasma membrane integrity. Kidney Int. 2003; 64: 128–139
- Hizoh I, Haller C. Radiocontrast-induced renal tubular cell apoptosis: hypertonic versus oxidative stress. Invest. Radiol. 2002; 37: 428–434
- Sheridan AM, Schwartz JH, Kroshian VM, Tercyak AM, Laraia J, Masino S, Lieberthal W. Renal mouse proximal tubular cells are more susceptible than MDCK cells to chemical anoxia. Am. J. Physiol. 1993; 265: F342–F350
- Fanning NF, Manning BJ, Buckley J, Redmond HP. Iodinated contrast media induces neutrophil apoptosis through a mitochondrial and caspase mediated pathway. Br J Radiol. 2002; 75: 861–873
- Hardiek K, Katholi RE, Ramkumar V, Dietrick C. Proximal tubule cell response to radiographic contrast media. Am J Physiol Renal Physiol. 2001; 280: F61–F70
- Schick SC, Haller C. Comparative cytotoxicity of ionic and non-ionic radiocontrast agents on MDCK cell monolayers in vitro. Nephrol Dial Transplant. 1999; 14: 342–347
- Qanungo S, Wang M, Nieminen AL. N-Acetyl-L-cysteine enhances apoptosis through inhibition of nuclear factor-kappaB in hypoxic murine embryonic fibroblasts. J Biol Chem. 2004; 279: 50455–50464
- Holt S, Goodier D, Marley R, Patch D, Burroughs A, Fernando B, Harry D, Moore K. Improvement in renal function in hepatorenal syndrome with N-acetylcysteine. Lancet. 1999; 353: 294–295
- Morley N, Curnow A, Salter L, Campbell S, Gould D. N-acetyl-L-cysteine prevents DNA damage induced by UVA, UVB and visible radiation in human fibroblasts. J. Photochem. Photobiol. B. 2003; 72: 55–60
- Ricardo KF, Shishido SM, de Oliveira MG, Krieger MH. Characterization of the effect of S-nitroso-N-acetylcysteine in normotensive and hypertensive conscious rats. Nitric Oxide. 2002; 7: 57–66
- Briguori C, Manganelli F, Scarpato P, Elia PP, Golia B, Riviezzo G, Lepore S, Librera M, Villari B, Colombo A, Ricciardelli B. Acetylcysteine and contrast agent-associated nephrotoxicity. J. Am. Coll. Cardiol. 2002; 40: 298–303
- Allaqaband S, Tumuluri R, Malik AM, Gupta A, Volkert P, Shalev Y, Bajwa TK. Prospective randomized study of N-acetylcysteine, fenoldopam, and saline for prevention of radiocontrast-induced nephropathy. Catheter Cardiovasc. Interv. 2002; 57: 279–283
- Durham JD, Caputo C, Dokko J, Zaharakis T, Pahlavan M, Keltz J, Dutka P, Marzo K, Maesaka JK, Fishbane S. A randomized controlled trial of N-acetylcysteine to prevent contrast nephropathy in cardiac angiography. Kidney Int. 2002; 62: 2202–2207
- Gomes VO, Poli de Figueredo CE, Caramori P, Lasevitch R, Bodanese LC, Araujo A, Roedel AP, Caramori AP, Brito FS Jr, Bezerra HG, Nery P, Brizolara A. N-acetylcysteine does not prevent contrast induced nephropathy after cardiac catheterization with an ionic low osmolality contrast medium: a multicentre clinical trial. Heart. 2005; 91: 774–778