Abstract
Previous observations indicate that Ficus thonningii (Blume) [Moraceae] stem-bark extracts may be useful in the control of diabetes mellitus. Accordingly, we investigated in some experimental animal paradigms the effects of F. thonningii stem-bark ethanolic extract (FTE) on renal and cardiovascular functions as complications of diabetes. Oral glucose tolerance tests were conducted in separate groups of non-diabetic and STZ-treated diabetic rats given glucose load (0.86 g.kg−1, p.o.) after 18-h fast, followed by various FTE doses (60, 120, and 240 mg.kg−1). Rats treated with deionized water (3 mL.kg−1 p.o.), or metformin (500 mg.kg−1 p.o.) acted as untreated and treated positive controls, respectively. Blood glucose was monitored at 15-min intervals for the first hour, and hourly thereafter for 3 h. Acute effects of FTE on kidney function and mean arterial blood pressure (MAP) were investigated in anaesthetized rats challenged with hypotonic saline after a 3.5-h equilibration for 4 h of 1 h control, 1.5 h treatment, and 1.5 h recovery periods. FTE was added to the infusate during the treatment period. Chronic effects of FTE were studied in individually caged rats treated daily with FTE (120 mg.kg−1, p.o.) for five weeks. Cytotoxicity of FTE was assessed by dye-reduction colorimetric (MTT) assay on MDBK and LLCPK1 kidney cell lines exposed for 24 h, 48 h, and 72 h to graded concentrations of the extract. Myocardial contractile performance was evaluated on rat isolated atrial muscle strips. FTE, like metformin, decreased blood glucose levels in non-diabetic and STZ-diabetic rats. Both acute and chronic FTE treatments did not affect renal function. In vitro studies demonstrated that FTE increased MDBK cell metabolic activity by an average of 15% (72 h), and LLCPK1 mirrored the controls. Acute intravenous infusion of FTE reduced the MAP from 119 ± 1 mmHg to 98 ± 4 mmHg. The MAP also was reduced throughout the five-week experimental study period. FTE also produced concentration-dependent, negative inotropic and chronotropic effects on rat isolated, electrically driven left-, and spontaneously beating right-, atrial muscle preparations. Our experimental findings suggest that FTE possesses reno- and cardio-protective effects in diabetes mellitus.
INTRODUCTION
We have previously reported that Ficus thonningii (Blume) [Moraceae] stem-bark ethanolic extract reduces blood glucose in non-diabetic and diabetic rats after five weeks exposure.Citation[1] Because diabetes is often associated with impaired kidney function and cardiovascular disorders, appropriate goals in the control of diabetes mellitus using F. thonningii should include not only regulating blood glucose, but also the prevention or alleviation of these complications. Accordingly, the current study was designed to establish in some experimental animal paradigms the effects of F. thonningii bark ethanolic extracts (FTE) not only on blood glucose, but also renal and cardiovascular functions. Thus, the main purpose of the present study was to investigate the effects of short-term (acute) and long-term (chronic) administration of FTE on renal fluid and electrolyte handling, as well as on blood pressure in male Wistar rats. Available evidence suggests that some herbal extracts may interfere with the concentrating and diluting mechanisms of tubular transport processes in the proximal tubule and distal tubule cells and/or on other protein components of tubular cell membranes.Citation[2],Citation[3] Indeed, previous studies in our laboratories show that some crude plant extracts impair the renal handling of fluid and electrolytes.Citation[4] Thus, we further speculated that FTE influences tubular reabsorption and secretion by altering tubular epithelial cells' viability. Therefore, the second objective was to assess the effects of FTE on cell viability on previously validated porcine proximal tubule and bovine distal tubule cell lines. Cell culture systems provide a good model for the evaluation of cytotoxicity of various compounds. We, therefore, employed the extensively used in vitro cell culture techniques of the proximal (LLC-PK1) and distal tubule (MDBK) cellsCitation[5–7] to study FTE-induced renal effects. The technique mimics the in vivo state, as these cell lines maintain similar biochemical function of levels of marker enzymes exhibited by freshly isolated cells.Citation[8] We also assessed the effects of FTE on glomerular filtration rate (GFR), an indicator of renal tubular function.Citation[9],Citation[10] Furthermore, the study investigated the influence of FTE on blood pressure, a parameter associated with the deterioration of kidney function in diabetic patients.Citation[11] In an effort to shed more light on the plausible mechanism(s) through which FTE may affect blood pressure, we have also investigated the effects of FTE on myocardial contractility in vitro.
MATERIALS AND METHODS
Preparation of Plant Extract
Pieces of Ficus thonningii stem-barks were identified by Prof. H. Baijnath, the former Chief Taxonomist/Curator of the University of Durban-Westville's Department of Botany. A voucher specimen of the plant has been deposited in the University's Botany Departmental Herbarium. The stem-barks were air-dried at room temperature and milled into fine powder with a commercial blender. The powdered stem-bark was macerated in 95% ethanol for 24 h (with occasional shaking) and filtered. The filtrate was concentrated under reduced pressure in a rotary evaporator at 60 ± 1°C. The crude, powdery Ficus thonningii stem-bark ethanolic extract (FTE) was used throughout this study without further purification.
Animals
Male Wistar rats (250–300 g body weight) maintained under laboratory conditions of temperature, humidity, and 12 h light/12 h dark regime at the Biomedical Resource Unit, University of KwaZulu-Natal, were used. The rats were exposed to both food (Epol-diet 4700, Epol, South Africa) and water ad libitum. Ethical clearance was obtained for this study from the University of KwaZulu-Natal's Ethics committee.
Experimental Design
Oral glucose tolerance test (OGTT) studies were carried out in male, non-diabetic, and streptozotocin (STZ)-treated diabetic Wistar rats (250–300 g body weight). In vivo studies on renal function and blood pressure were carried out in non-diabetic and streptozotocin (STZ)-induced diabetic rats, while the in vitro effects of the plant's extract were conducted on kidney cell lines LLC-PK1 and MDBK. Effects of FTE on myocardial contractile performance were evaluated on rat isolated atrial muscle strips.
Induction of Diabetes Mellitus
Diabetes mellitus was induced in the diabetic group of rats by intraperitoneal injections of STZ (60 mg.kg−1) in citrate buffer, pH 6.3. Vehicle (citrate buffer)-treated animals acted as controls. Animals that exhibited glucosuria after 24 h, tested by urine test strips (Rapidmed Diagnostics, Sandton, South Africa), were considered diabetic. Plasma glucose concentration of 25 mmol.L−1 measured after one week was considered as a stable diabetic state before our experimental procedures.
Series 1: OGTT
The rats used were divided into the following groups for OGTT: non-diabetic control, treated non-diabetic, control STZ-treated diabetic, and treated STZ-diabetic rats (n = 6 in each group). Rats treated with deionized water served (3 mL.kg−1, p.o.) served as control animals. All of the animals were starved for 18 h before being orally treated with glucose (0.86 g.kg−1, body weight, p.o.), followed by FTE at various doses (60, 120, and 240 mg.kg−1, p.o.). To establish whether FTE possesses pharmacological activities comparable to synthetic hypoglycemic drugs already in use, studies were conducted in separate groups of non-diabetic and STZ-diabetic rats orally treated with metformin (500 mg.kg−1, p.o.). Blood samples were collected from the tail veins of the animals at 15-min intervals for the first hour, and hourly thereafter for the subsequent 3 h for glucose measurements using Bayer's glucometer Elite® (Elite (Pty) Ltd, Health Care Division, South Africa).
Series 2: Renal Function
Acute Studies
Male Wistar rats were divided into groups of untreated control and treated rats (n = 6 in each group). Rats were anaesthetized by an introperitoneal injection of Trapanal (sodium 5- ethyl-(1-methylbutyl)-2-thiobarbiturate, Byk Gulden, Konstanz, Germany) at a dose of 0.11 g.kg−1 and tracheotomized to maintain clear airway entry. The right jugular vein was cannulated with polyethylene tubing (i.d. 0.86 mm; o.d 1.27 mm, Portex, Hythe, Kent, UK) to allow intravenous infusion of 0.077 M NaCl. The urinary bladder of each rat was also cannulated with similar calibre polyethene tubing via an incision in the abdominal wall. The body temperature of each animal was maintained at 37 ± 1oC with a heated table.
The control group of animals (n = 6) was placed on a continuous infusion of 0.077 M NaCl at 9 mL·h−1 (Harvard syringe infusion Pump 22). Following an initial equilibration period of 3.5 h, eight consecutive urine collections were made into pre-weighed plastic vials at 30-min intervals over the subsequent 4 h for measurements of urine flow and Na+ and K+ excretion rates. The control group of rats was designed to check the stability of renal function.
Treated Group
Renal effects of the crude plant's extract (FTE) were studied in a group of rats following a 3.5 h equilibration period. FTE solution was prepared by using a modified method that has been previously described. Citation[12],Citation[13] The extract was freshly dissolved in dimethyl sulfoxide (DMSO, 2 ml) and normal saline (19 ml) before use in each case. Urine samples were collected for 1 h (control period) for measurements of urine flow and Na+ and K+ excretion rates, following which the extract solution was infused at 0.06 μg·min−1 for 1.5 h (treatment period), resulting in a total dose of 18 g·kg−1 (for a 300-g rat). The animals were then switched back to the infusate alone for the last 1.5 h (recovery period).
Blood Pressure Measurements
Test groups of rats were surgically prepared as described for the renal studies, except that a heparinized cannula (Portex, i.d. 0.86 mm; o.d. 1.27 mm) was also inserted into the left common carotid artery to permit the recording of mean arterial blood pressure at 30-min intervals (Statham MLT 0380, Ad Instruments, compatible with the PowerLab System ML410/W, Australia).
Chronic Studies
Wistar rats (250–300 g body weight) were housed individually at the Biomedical Resource Unit, University of KwaZulu-Natal, in Makrolon polycarbonate metabolic cages (Techniplats, South Africa) that were cleaned daily. All animals were maintained on a 12 h dark/light cycle and allowed free access to water and food (Epol-diet 4700, Epol, South Africa). In those animals in which the effects of FTE were investigated, the rats were treated with FTE (120 mg.kg−1, p.o.) daily for five weeks at 09h00. Control rats were similarly treated with distilled water (3 mL.kg−1). Urine volume and total urinary outputs of Na+ and K+ were determined from 24 h samples for all groups.
Blood Pressure Measurements
Mean arterial blood pressure (MAP) was monitored every third consecutive day for five weeks at 09h00 using non-invasive tail cuff method with photoelectric sensors (IITC Model 31 Computerized Blood Pressure Monitor, Life Sciences, Woodland Hills, California, USA). The unit works with IITC hardware system to measure blood pressure and heart rate in conscious rats. The animals had been warmed in an enclosed chamber (IITC Model 303sc Animal Test Chamber, IITC Life Sciences, Woodland Hills, California, USA) for 30 min at ± 30°C before taking BP readings.
Terminal Studies
At the end of the five-week plants' extracts treatment period, blood glucose was measured from the tail veins of all groups of non-fasted animals using Bayer's glucometer Elite® (Elite (Pty) Ltd, Health Care Division, South Africa). Blood samples were also collected from all groups of animals by cardiac puncture into individual pre-cooled heparinized containers. Separated plasma was analyzed for Na+, K+, creatinine, and urea concentrations. Blood for insulin was collected into plain tubes, and the separated serum was stored in a Bio Ultra freezer (Mallkinckrodt, Ohio, USA) at −70°C until assayed.
Analytical Methods
Measurement of Electrolytes, Insulin, and Glomerular Filtration Rate
Urine volume was determined gravimetrically. Na+ and K+ concentrations were determined by ion activity using the Beckman Coulter (Synchron LX20 Clinical Systems, Fullerton, California, USA). Urea and creatinine analyses were performed using the Beckman Coulter instrument. Creatinine estimation employed the reaction of creatinine and sodium picrate to form creatinine picrate. Urea estimation employed the hydrolytic degradation of urea in the presence of urease. The methods used reagent kits from Beckman Coulter, Ireland, Inc., and measured using Beckman Coulter (Synchron LX20 Clinical Systems, Fullerton, California, USA). Glomerular filtration rate (GFR), as assessed by creatinine clearance, was calculated from measurements of urinary and plasma concentrations of creatinine and urine flow rate in the fifth week.
Plasma insulin concentrations were measured by Coat-A-Count procedure using a kit from Diagnostic Products Corporation, Los Angeles, USA. This is a solid-phase radioimmunoassay procedure based on insulin-specific antibody immobilized to the wall of a polypropylene tube. The lower limit of detection was 55 pg.mL−1. Inter- and intra-assay coefficients of variation were 8.1% (n = 20) and 8.3% (n = 20), respectively.
Series 3: Cell Culture Studies
LLC-PK1 and MDBK cells were grown and maintained at 37oC in Eagle's Minimum Essential Medium (EMEM) (containing 0.1 mM Hepes buffer) supplemented with 5% heat inactivated foetal calf serum, 1% L-glutamine, and 1% penstrep-fungizone [complete culture medium (CCM)] (Delta Bioproducts, South Africa). Once the cells reached confluence, they were detached from the culture flask (75 cm2) with 0.025% (w/v) trypsin and resuspended in CCM. Cell viability was determined in the presence of 0.2% (w/v) trypan blue in a haemocytometer. A 200-μl aliquot of the cell suspension (1.5 × 106 cells) was transferred into separate 96-well microtiter plates (Greiner Bio-one GmbH, Germany). Thereafter, the viability of cells incubated at 37oC for 24, 36, and 72 hours, containing various concentrations of FTE in separate wells, was assessed (0, 100, 200, 400, 600, 800, and 1000 μl mL−1, n = 6 for each dilution). The wells were aspirated after each incubation and washed with Hank's balanced salt solution (HBSS). All supernatants were discarded. The cells were resuspended in 100 μl CCM containing 10 μl of MTT [3-(4,5-dimethylthiazol-2-yl)-2,5-diphenyltetrazolium bromide] (i.e., 5mg.ml−1 MTT salt in HBSS, Calbiochem, Darmstadt, Germany) and incubated for 4 h at 37oC. After 4 h, the plates were centrifuged (20 min, 2000 rpm at room temperature). The supernatant was removed, and any resulting formazan crystals were then solubilized with 100 μl dimethylsulphoxide (DMSO). After one hour, the optical density was determined spectrophotometrically using an ELISA plate reader (Bio‐Tek Instruments) at 595 nm and a reference wavelength of 655 nm. Absorbance was expressed as percentage cleavage activity. Percentage cell viability was calculated as the mean absorbance of control cells/mean absorbance of treated cells.
Series 4: Isolated Atrial Muscle Strips
In order to throw some light on the plausible mechanism(s) by which FTE may influence blood pressure, we studied the effects of the extract on rat isolated atrial muscle strips. The Wistar rats used were sacrificed by stunning and exsanguination. The left and right atrial muscles of the animals were isolated and mounted as described by Ojewole.Citation[14] The isolated left atrium of each rat was impaled on a thin platinum wire electrode and suspended under an applied resting tension of 1.0 g in a 30-ml Ugo Basile organ-bath containing Krebs-Henseleit physiological solution (of composition, in mmol/L NaCl, 118; KCl, 4.7; NaH2PO4, 1.28; NaHCO3, 25.0; MgCl2, 1.2; CaCl2, 2.52; and glucose, 5.55, pH adjusted to 7.4) maintained at 34 ± 1°C and continuously aerated with carbogen (95% O2 + 5% CO2 gas mixture). Each left atrial muscle preparation was electrically driven with square wave pulses of 5 msec duration at a frequency of 3 Hz and supramaximal voltages of 5–10 volts, delivered by an SRI stimulator. The spontaneously beating right atrium of the animal was also set up under the same physiological, experimental conditions. Two isolated electrically driven left atrial muscle strips and two isolated spontaneously-beating right atrial muscle preparations, were always set up at a time (one used as the test and the other as the control) to allow for changes in the atrial muscle sensitivity. The atrial muscle preparations were left to equilibrate for 45–60 min (during which time the bathing physiological solution was changed every 15 min) before they were challenged with FTE or any of the reference drugs used. The test atrial muscle preparations were treated with sequentially applied, graded concentrations of the extract and/or reference drugs used, while the control atrial muscle strips were treated with volumes of distilled water equivalent to the volumes of bath-applied FTE (5–80 g.mL−1). The electrically provoked and spontaneous contractions of the atrial muscles, as well as the FTE- (and reference drug)-induced responses of the atrial muscle preparations, were recorded isometrically by means of Ugo Basile force-displacement transducers and pen-writing ‘Gemini’ recorders (model 7070).
DATA PRESENTATION
All data are expressed as means ±standard error of means (SEM). Cell viability was expressed as a percentage relative to control cells not exposed to any of the test compounds. Data obtained from test rat isolated atria treated with FTE alone, as well as those obtained from deionized water-treated control atrial strips, were pooled and compared with those of reference drugs. A statistical comparison of the differences between FTE and respective controls was performed with GraphPad InStat Software (version 3.00, GraphPad Software, San Diego, California, USA) using one-way analysis of variance (ANOVA; 95% confidence interval), followed by Tukey-Kramer multiple comparison test. A value of p < 0.05 was considered significant.
RESULTS
OGTT
compares the OGTT responses in acutely treated non-diabetic rats with respective control animals. Oral administration of various doses of FTE (60, 120, and 240 mg.kg−1) decreased the blood glucose concentrations in a dose-dependent manner, with all doses exerting maximum effects after 60 min. The hypoglycemic effect of FTE was still significant by the end of the 4 h experimental period. A similar pattern of hypoglycemic effects was observed with metformin. Untreated control non-diabetic rats exhibited significantly high plasma glucose concentrations by comparison with treated animals. The plasma glucose concentrations of the control, non-diabetic rats increased to 6.8 ± 0.3 mmol.L−1 by 30 min from a baseline value of 4.2 ± 0.1 mmol.L−1 before slowly declining to 4.3 ± 0.1 mmol.L−1 (n = 6) after 4h, a value that was significantly elevated when compared to animals administered the highest dose of FTE at the corresponding period.
Figure 1. Comparison of OGTT responses in separate groups of (A) non-diabetic and (B) streptozotocin (STZ)-treated diabetic rats treated with graded doses of FTE with control animals treated with deionized water or positive controls treated with metformin. Values are presented as means, and vertical bars indicate SE of means (n = 6 in each groups). *p < 0.01 by comparison with control animals.
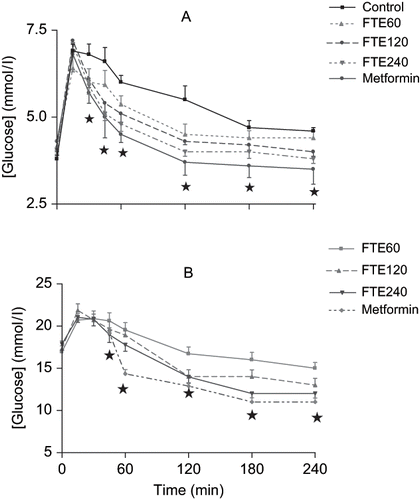
Similarly, oral administration of various doses of FTE (60, 120, and 240 mg.kg−1) decreased blood glucose concentrations of STZ-treated diabetic rats in a dose-dependent manner by 45 min until the end of the 4 h experimental period (see ). The glucose concentrations of STZ-treated diabetic control animals orally loaded with glucose did not significantly decline by the end of the 4-h experimental period. Metformin induced marked reductions in blood glucose concentrations by 45 min until the end of the 4-h experimental period.
In those animals in which FTE was chronically administered (120 mg.kg−1, p.o.) daily for five weeks at 09h00, the mean plasma concentration of glucose was significantly decreased in non-diabetic and STZ-induced diabetic rats by the end of the experimental period in comparison with respective control animals at the corresponding time (see ).
Table 1 Plasma glucose, Na+, K+, urea and creatinine concentrations and GFR in non-diabetic and STZ-diabetic control and rats administered FTE every third consecutive day for five weeks (n = 6 in all groups)
Renal Function Tests
Urine flow and Na+ excretion rates ranged from 9 to 10 ml.h−1and 619 to 660 μmol.h−1, respectively, in vehicle-infused control animals during the 4 h post-equilibration period, values that compared with the infusion rate (9 mL.h−1 and 693 μmol.h−1, respectively). K+ excretion rate was also stable throughout the post-equilibration period, ranging from 226 to 256 μmol.h−1. No significant changes in renal fluid flow and electrolyte excretion rates were observed in animals that were acutely treated with FTE. Compared with the control rats, the FTE-treated animals attained stable Na+ excretion and urine flow rate, which approximated the infusion rates throughout the experimental period. Similarly, the mean weekly urine volume and the urinary Na+ and K+ outputs were not significantly different between animals chronically treated with FTE (120 mg.kg−1, p.o.), and untreated rats. Urinary creatinine and urea outputs were not significantly different between the control and treated rats throughout the five-week experimental period. However, FTE treatment significantly reduced (p < 0.01) plasma creatinine concentration, unlike plasma urea concentration that was not altered (see ). By the end of the five-week period, FTE administration significantly elevated (p < 0.01) the GFR value in STZ-treated diabetic rats, but the increase in non-diabetic rats did not achieve statistical significance.
Hemodynamic Studies
Acute infusion of hypotonic saline to control animals did not show any significant variations in the mean arterial blood pressure throughout the 4-h post-equilibration period. However, acute intravenous infusion of FTE at 120 μg.h−1 for 1.5 h reduced the mean arterial blood pressure from a mean pre-treatment value of 119 ± 1 mmHg to 98 ± 4 mmHg (n = 6) by the end of treatment. The hypotensive effect of FTE persisted during the post-treatment period to a mean value of 110 ± 4 mmHg at the end of the experiment (see ). The mean arterial blood pressure (MAP) changes due long-term (chronic) FTE treatments are shown in . Chronic treatment of the rats with FTE (120 mg.kg−1 daily for five weeks, p.o.), caused significant decreases in MAP in non-diabetic and STZ-treated diabetic rats throughout the study period in comparison with control rats at the corresponding period ().
CELL CULTURE STUDIES
The MTT assay is a quantitative colorimetric method based on the reduced cleavage of the water soluble monotetrazolium salt MTT to a purple formazan in metabolically active cells. The MTT salt is actively transported into metabolically viable cells. Both and show the viability of LLC-PK1 and MDBK cells treated with various concentrations of FTE after 24, 48, and 72 hours. There was no toxicity noted in both cell lines after treatment with FTE. In , LLC-PK1 cell metabolism was increased with increased doses of FTE (600–1000 μg.mL−1) for all incubation time periods. In contrast, the MDBK cells () show increased metabolism for all concentrations of FTE. There was a significant increase in metabolism and cell viability after 72-h incubation, with significance being more pronounced at the highest concentrations (800 and 1000 μg.mL−1).
Figure 3. Viability of (A) LLC-PK1 and (B) MDBK cell lines treated with FTE. The cells were treated with 100, 200, 400, 600, 800, or 1000 μg FTE. Cell viability was determined by the Tryphan blue exclusion assay. Values for untreated control were taken as 100%. Each dose represents the mean of six treatments, while the vertical bars denote standard errors of the means.
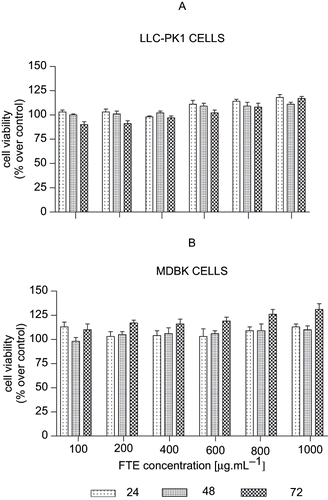
Rat Isolated Atrial Muscle Strips
The cardiac effects of FTE are shown in . This figure shows that FTE (5–80 mg.mL−1) produced concentration-related, negative chronotropic () and inotropic () effects on rat isolated spontaneously beating right- and electrically driven left- atrial muscle strips, respectively. The cardio-depressant effects of FTE were not modified by bath-applied atropine (10−8–10−6 M), suggesting that the cardio-inhibitory effects of the plant's extract are unlikely to be mediated through cholinergic mechanisms.
Figure 4. Effects of sequentially applied graded concen- trations of FTE (5–80 mg.mL−1) on the (A) rate and (B) force of contractions of rat isolated spontaneously beating right- and electrically driven left-atrial muscle strips, respectively. Each point represents the mean of 8–10 observations, while the vertical bars denote standard errors of the means. *p < 0.05, **p < 0.01, ***p < 0.001 versus control.
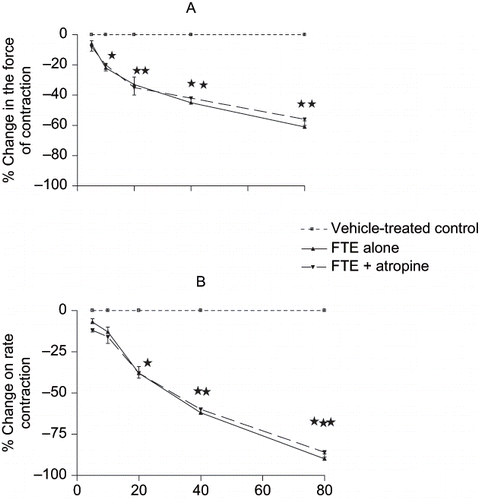
DISCUSSION
In the present study, we have evaluated the effects of short-term (acute) and long-term (chronic) oral treatments of Ficus thonningii stem-bark ethanolic extract (FTE) on cardiovascular systems and kidney functions of rats. The major findings of this study, apart from confirming our previous observations of the hypoglycaemic effects of oral administrations of the plant's extract,Citation[1] show that FTE decreases blood pressure without significant influences on renal function in normotensive rats. The management of diabetes mellitus without side effects is a global challenge, thus increasing the demand for natural products with antidiabetic activity.Citation[15] Cardiovascular and renal complications are the major causes of mortality in diabetes mellitus.Citation[16] We suggest that the use of FTE in the control of diabetes mellitus may be beneficial when hypertension and compromised renal function co-exist. This is significant considering the fact that diabetes mellitus is associated with cardiovascular complications and deterioration of kidney dysfunction in diabetic patientsCitation[17] and experimental animals.Citation[18–20] Therefore, the management of diabetes with FTE has the potential to address both renal and cardiovascular protection. Conventionally, renoprotection is achieved through a reduction in blood pressure with antihypertensive regimens.Citation[17], Citation[21–23] Of note in the present study is the hypotensive effect of FTE without altering kidney function, in contrast to previous reports of impaired renal function following the administration of some hypoglycaemic plant extracts.Citation[4],Citation[24] A significant increase in GFR as assessed by creatinine clearance and a concomitant decrease in plasma creatinine concentration was observed for the STZ-induced diabetic group treated with FTE over the five-week period. This finding is significant, given the fact that some antihypertensive agents (e.g., thiazide diuretics) and ß-blockers influence glycaemic control in a deleterious manner.Citation[25] Evidence from biomedical literature suggests that some herbal extracts have protective effects against cardiovascular disease in diabetes.Citation[26] We and several other authors have previously used creatinine clearance in rats to monitor GFR. Citation[9],Citation[10],Citation[27],Citation[28] It is likely that chronic FTE treatment increased creatinine secretion as evidenced by increased MDBK cell metabolic activity. However, further studies are required to establish the mechanism(s) through which FTE reduces plasma creatinine levels. The cell culture studies provide an experimental model to assess cytotoxicity and metabolic activity of FTE in the two renal cell lines. Initially, the MTT assay was used as a measure of cell viability and proliferation, with the mitochondrial reduction by succinate reductase system being the major contributor to MTT reduction.Citation[29] However, recent evidence shows that most MTT reduction occurs extra-mitochondrially with MTT salt crossing the intact plasma membranes to be reduced intracellularly.Citation[30] Other investigators have shown that most of the cellular reductions of MTT are dependent on microsomal enzymes and not only on succinate dehydrogenase.Citation[30–32] This microsomal reduction requires NADH and NADPH and is not affected by respiratory inhibitors.Citation[31] This clearly indicates that cellular reduction of MTT is related more to the glycolytic rate, and thus NADH production, than to respiration, and is therefore primarily a measure of the rate of glycolytic NADH production.Citation[32] Our cell culture data clearly support this finding, as MTT reduction is increased in the presence of FTE, especially at the higher concentrations. Our data also confirm the lack of toxicity of FTE on renal cells derived from two species, viz., pig and bovine. The findings of the present study suggest that FTE is a useful agent in increasing glucose uptake by renal cells and glucose uptake studies are needed to verify this phenomenon. Our data indicate that FTE has the potential to reduce plasma levels of creatinine in patients with diminished renal function and reduce cardiovascular and renal complications as well. The elevation of plasma creatinine is a risk factor for the development of cardiovascularCitation[33] and end stage renalCitation[34] diseases. FTE reduced mean arterial pressure in normotensive rats without significant effects on renal fluid and electrolyte handling, suggesting that the cardiovascular effects of FTE are mediated through influences on components of the cardiovascular system. We suggest that the cardio-inhibitory effects of FTE may contribute, in part at least, to the hypotensive of the plant's extract. This hypothesis is supported by our findings from experiments on isolated guinea-pig atrial muscle strips which demonstrated significant negative chronotropic and inotropic, cardiodepressant effects of FTE. In conclusion, our experimental findings suggest that FTE possesses reno- and cardio-protective effects in diabetes mellitus.
ACKNOWLEDGMENT
The authors thank Ms. K. Moodley for technical support.
REFERENCES
- Bwititi P, Musabayane CT. The effect of plant extracts on plasma glucose levels in rats. Acta Medica et Biologica (Japan). 1997; 45(4)167–169
- Bevevino LH, Aires MM. Effect of crude extract of roots of Bredemeyera floribundaWilld. II. Effect on glomerular filtration rate and renal tubular function of rats. J. Ethnopharmacol. 1994; 43(3)203–207
- Kreydiyyeh SI, Usta J. Diuretic effect and mechanism of action of parsley. J. Ethnopharmacol. 2002; 79(3)353–357
- Musabayane CT, Xozwa K, Ojewole JAO. Effects of Hypoxis hemerocallidea (Fisch. & C.A. Mey.) [Hypoxidaceae] corm (African Potato) aqueous extract on renal electrolyte and fluid handling in the rat Renal Failure (USA). 2005; 27(5)763–770
- Bucci TJ, Howard PC. Effect of fumosin mycotoxins in animals. J Toxicol. 1996; 15: 293–302
- Rumora L, Kovačič S, Rozgaj R, Čepelak I, Pepeljnjak S, Grubišić TZ. Cytotoxic and genotoxic effects of fumosin B1on rabbit kidney RK cell line. Arch Toxicol. 2002; 76: 55–61
- Li W, Choy DF, Lam MS, Morgan T, Sullivan ME, Post JM. Use of cultured cells of kidney origin to assess specific cytotoxic effects of nephrotoxins. Toxicology in vitro. 2003; 17(1)107–113
- Cummings BS, Zangar RC, Novak RF, Lash LH. Cytotoxicity of trichloroethylene and S-(1,2-dichlorovinyl)-L-cysteine in primary cultures of rat renal proximal tubular and distal tubular cells. Toxicology. 2000; 150: 83–98
- Bursztyn M, Ben-Ishay D, Mekler J, Raz I. Insulin-induced renal dysfunction in regular Sabra rats. Clin. Exp. Pharmacol. 1995; 22(1)S32–S33
- Travlos GS, Morris RW, Elwell MR, Duke A, Rosenblum S, Thompson MB. Frequency and relationships of clinical chemistry and liver and kidney histopathology findings in 13-week toxicity studies in rats. Toxicology. 1996; 107(1)17–29
- Narita T, Kakei M, Ito S. Aggressive antihypertensive treatment and serum lipid lowering therapy are necessary to prevent deterioration of the renal function even in elderly type 2 diabetic patients with persistent albuminuria. Gerentology. 2002; 48(5)302–308
- Twaij H, Kery A, Al-Khazraji NK. Some pharmacological, toxicological and phytochemical investigations on Centaurea phyllocephala. J. Ethnopharmacol 1983; 9(2–3)299–314
- Al-Khazraji SM, Al-Shamaony LA, Twaij HA. Hypoglycaemic effect of Artemisia alba. Effect of different parts and influence of the solvent on hypoglycaemic activity. J Ethnopharmacol. 1993; 40(3)163–166
- Ojewole JAO. Studies on the pharmacology of some antimalarial drugs. University of Strathclyde, GlasgowScotland, UK 1977, Ph.D. thesis
- Can A, Akev N, Ozsoy N, Bolkent S, Arda BP, Yanardag R, Okyar A. Effect of aloe vera leaf gel and pulp extracts on the liver in type II diabetic rat models. Biol Pharmaceut Bull. 2004; 27(5)694–698
- Krolewski AS, Warram JH, Rand LI, Kahn CR. Epidemiologic approach to the etiology of type I diabetes mellitus and its complications. N Engl J Med. 1987; 317(22)1390–1398
- UK Prospective Diabetes Study Group. Tight blood pressure control and risk of macrovascular and microvascular complications in type 2 diabetes. BMJ. 1998; 317: 703–713
- Musabayane CT, Ndhlovu CE, Balment RJ. Renal fluid and electrolyte handling in streptozotocin (STZ) diabetic rats. Renal Failure (USA) 1995; 17(2)107–116
- Umrani DN, Goyal RK. Beneficial effects of fenoldopam treatment on renal function in streptozotocin-induced diabetic rats. Clin Exp Hypertens. 2002; 24(3)207–219
- González-Albarrán O, Gómez O, Ruiz E, Vieitez P, García-Robles R. Role of systolic blood pressure on the progression of kidney damage in an experimental model of type 2 diabetes mellitus, obesity, and hypertension (Zucker rats). Am J Hypertens. 2003; 16(11 Pt 1)979–985
- Maschio G, Alberti D, Janin G, Locatelli F, Mann JF, Motolese M, Ponticelli C, Ritz E, Zuchelli P. The angiotensin-converting-enzyme inhibitor benazepril on the progression of chronic renal insufficiency. N Eng J Med. 1996; 334: 939–945
- Tanaka R, Kon V, Yoshioka T, Ichikawa I, Fogo A. Angiotensin-converting enzyme inhibitor modulates glomerular function and structure by distinct mechanisms. Kidney Int. 1994; 45: 537–543
- Bidani AK, Griffin KA, Bakris G, Picken MM. Lack of evidence of blood pressure-independent protection by renin-angiotensin system blockade after renal ablation. Kidney Int. 2000; 57: 1651–1661
- Bwititi P, Musabayane CT, Nhachi CFB. The effects of Opuntia megacantha (Prickly pear) on blood glucose and kidney function in streptozotocin (STZ) diabetic rats. Journal of Ethnopharmacol. 2000; 69: 247–252
- Ravid M, Savin H, Jutrin I, Bental T, Lishner M. Long-term stabilizing effect of angiotensin-converting enzyme inhibition on plasma creatinine and on proteinuria in normotensive type II diabetic patients. Ann Intern Med. 1993; 118(8)577–581
- Baluchnejadmojarad T, Roghani M. Endothelium-dependent and -independent effect of aqueous extract of garlic on vascular reactivity on diabetic rats. Fitoterapia. 2003; 74(7–8)630–637
- Girchev R, Markova P, Mikhov D, Natcheff N. Renal excretory function in conscious Long Evans and vasopressin deficient (Brattleboro) rats after endothelin-A receptor inhibition. Acta Physiol. Pharmacol. Bulg. 1998; 23(3–4)73–77
- Bertuzzi ML, Bensi N, Mayer N, Niebylski A, Armario A, Gauna HF. Renal mechanisms involved in stress-induced antinatriuresis and antidiuresis in rats. Arch. Physiol. Biochem. 2003; 111(3)259–264
- Slater TF, Sawyer B, Straeuli U. Studies on succinate-tetrazolium reductase systems. Points of coupling four different tetrazolium salts. Biochim Biophysics Acta. 1963; 77: 383–393
- Bernas T, Dobrucki JW. Mitochondrial and non-mitochondrial reduction of MTT: interaction of MTT and TMRE, JC-1, and NAO mitochondrial fluorescent probes. Cytometry. 2002; 47: 236–242
- Berridge MV, Tan AS. Characterization of the cellular reduction of MTT: subcellular localization, substrate dependence, and involvement of mitochondrial electron transport in MTT reduction. Arch Biochem Biophys. 1993; 303: 474–482
- Berridge MV, Tan AS, McCoy KD, Wang R. The biochemical and cellular basis of cell proliferation assays that use tetrazolium salts. Biochemica. 1996; 4: 15–20
- Mann JF, Gerstein HC, Pogue J, Bosch J, Yusuf S. Renal insufficiency as a predictor of cardiovascular outcomes and the impact of ramipril: The HOPE randomized trial. Ann Intern Med. 2001; 134: 629–636
- Iseki K, Ikemiya Y, Fukiyama K. Risk factors of end-stage renal failure disease and serum creatinine in a community-based mass screening. Kidney Int. 1997; 51: 850–854