Abstract
Hyperlipidemia is an associated complication of diabetes mellitus. In the present study, we investigated the effect of tetrahydrocurcumin (THC), one of the active metabolites of curcumin on lipid profile in streptozotocin (STZ)-nicotinamide-induced diabetic rats. THC 80mg/kg body weight was orally administered to diabetic rats for 45 days, resulting in a significant reduction in blood glucose and a significant increase in plasma insulin in diabetic rats, which proved that THC possess an antidiabetic effect. THC also caused a significant reduction serum and liver cholesterol, triglycerides, free fatty acids, phospholipids, HMG CoA reductase activity, very low-density lipoprotein (VLDL) and low-density lipoprotein (LDL) cholesterol levels. The decreased serum high-density lipoprotein (HDL) cholesterol in diabetic rats was also reversed toward normalization after the treatment. These biochemical observations were supplemented by histopathological examination of liver section. The effect was compared with curcumin (80 mg/kg body weight). The results showed that THC had antihyperlipidemic action in control and experimental diabetic rats. The antidiabetic and antihyperlipidemic effects of THC are more potent than those of curcumin at the same dose.
INTRODUCTION
Diabetes mellitus is the most common human metabolic disease, affecting about 200 million people in the world. Changes in the concentration of lipids including cholesterol and triglycerides are complications observed frequently in the case of diabetes mellitus and certainly contribute to the development of vascular disease.Citation[1],Citation[2]
The relationship between diabetes mellitus and hyperlipidemia is a well-recognized phenomenon.Citation[3] Liver is an insulin-dependent tissue that plays a pivotal role in glucose and lipid homeostasis and is severely affected during diabetes. Liver participates in the uptake, oxidation, and metabolic conversion of free fatty acids, as well as the synthesis of cholesterol, phospholipids, and triglylcerides.Citation[4] Decreased glycolysis, impeded glycogenesis, and increased gluconeogenesis are some of the changes of glucose metabolism in the diabetic liver.Citation[5] There are many compositional abnormalities of lipoproteins: very low density lipoprotein (VLDL), low density lipoprotein (LDL), and high-density lipoprotein (HDL) have been found in diabetic patients. These alterations may be relevant in explaining, at least in part, the increased predisposition of diabetes to atherosclerosis.
Curcumin (diferuloylmethane) is the substance that gives the spice turmeric, which is extensively used in Indian cuisine as a component of curry powder, its yellow color. It is believed that curcumin is a potent antioxidant and anti-inflammatory agent. Practitioners of traditional Indian medicine believe that curcumin powder is beneficial against many diseases, including biliary disorders, anorexia, coughs, diabetes, hepatic disorders, rheumatism, sinusitis, cancer, and Alzheimer's disease.Citation[6] Curcumin has been shown to reduce hyperlipidemia,Citation[7] delay the development of cataracts,Citation[8] ameliorate renal lesions,Citation[9] and reduce the cross-linking of collagenCitation[10] in a streptozotocin (STZ)-treated diabetic animal model. Curcumin has also been shown to lower blood glucose levels in type 2 diabetic KK-Ay miceCitation[11]and STZ-treated rats.Citation[12] Curcumin supplementation promotes wound healing in STZ-treated diabetic rats and genetically diabetic miceCitation[13] and attenuates the phenylephrine-induced increase in vascular reactivity of the aorta in STZ-treated diabetic rats.
Tetrahydrocurcumin (THC) is one of the major metabolites of curcumin with potential bioactivity. This metabolite was identified in intestinal and hepatic cytosol from humans and rats.Citation[14],Citation[15] Recently, attention has focused on THC as one of the major metabolites of curcumin, because this compound appears to exert greater antioxidant activity in both in vitro and in vivo systems.Citation[16],Citation[17] Structurally (see ), THC and curcumin have identical β-diketone structures and phenolic groups, but differ in that THC lacks the double bonds.Citation[16] Sugiyama et al.Citation[18] demonstrated that THC exhibited similar physiological and pharmacological properties as the active form of curcumin in vivo. Naito et al.Citation[15] showed clear involvement of THC in biochemical and molecular actions at the cellular level in ameliorating oxidative stress in cholesterol-fed rabbits. Some researchers also have focused on the neuroprotective role of curcumin in amyloid neurotoxicity and amyloid fibril formation in Alzheimer's disease models and other possible neurodegenerative diseases.Citation[19] In our previous study, we have demonstrated the antidiabetic effect of THC in STZ-induced diabetic rats.Citation[20] The present investigation was carried out to study the effect of curcumin and THC on serum and liver lipids and lipid-metabolizing enzyme in rats with STZ and nicotinamide-induced diabetes.
MATERIALS AND METHODS
Animals
Adult (eight weeks) male albino Wistar rats, weighing 180–220 g and bred in the Central Animal House, Rajah Muthiah Medical College, Annamalai University, were used. All animal experiments were approved by the ethical committee (Vide. No: 284, 2005) of Annamalai University and were in accordance with the guidelines of the National Institute of Nutrition, Indian Council of Medical Research, Hyderabad, India. The animals were housed in polycarbonate cages in a room with a 12 h day-night cycle, temperature of 24 ± 2°C, and humidity of 45–64%. During the whole experimental period, animals were fed with a balanced commercial diet (Hindustan Lever Ltd., Mumbai, India) and water ad libitum.
Drugs and Chemicals
THC was a gift provided by Sabinsa Corporation, USA. Curcumin was purchased from Sigma Chemicals Company (St. Louis, Missouri, USA). All other chemicals and biochemicals were of analytical grade.
Induction of Diabetes
Type 2 diabetes mellitus was inducedCitation[21] in overnight fasted rats by a single intraperitoneal injection (i.p) of 65 mg/kg body weight STZ, 15 min after the i.p administration of 110 mg/kg body weight of nicotinamide. STZ was dissolved in 0.1 M citrate buffer (pH 4.5), and nicotinamide was dissolved in normal saline. Hyperglycemia was confirmed by the elevated glucose levels in plasma, determined at 72 h and then on day 7 after injection. The animals with blood glucose concentration more than 200 mg/dL will be used for the study.
Experimental Design
A total of 24 rats (18 diabetic surviving rats, 6 control rats) were used. The rats were divided into four groups of six each, after the induction of STZ diabetes, as follows:
Group I: Control rats.
Group II: Diabetic control rats.
Group III: Diabetic rats given THC (80 mg/kg body weight) in aqueous suspension daily using an intragastric tube for 45 days.
Group IV: Diabetic rats given curcumin (80 mg/kg body weight) in aqueous suspension daily using an intragastric tube for 45 days.Citation[22]
At the end of 45 days, the animals were deprived of food overnight and sacrificed by decapitation. Blood was collected in tubes containing potassium oxalate and sodium fluoride mixture for the estimation of blood glucose. Plasma was separated for the estimation of insulin and other biochemical parameters. Livers were immediately dissected out and washed in ice-cold saline to remove the blood.
Preparation of Liver Extract
Lipids were extracted from tissues by the method of Folch et al.Citation[23] using a chloroform-methanol mixture (CHCl3:MeOH)(2:1 v/v). A known weight of liver was homogenized in 7 ml of methanol using potter Elvehjam homogenizer. The contents were filtered into a previously weighed side arm flask, and residue on the filter paper was scrapped off and homogenized with 14 ml of CHCl3:MeOH mixture. This was again filtered into the side arm flask, and the residue was successively homogenized in CHCl3:MeOH (2:1 v/v), and each time this extract was filtered. The pooled filtrate in the flask was adjusted to a final volume ratio using CHCl3:MeOH and evaporated to dryness to a constant weight.
Thus, the obtained residue of lipids was dissolved in 5 ml of CHCl3:MeOH mixture and transferred into a centrifuge tube; 2 ml of 0.1 M potassium chloride was then added, shaken well, and centrifuged. The upper aqueous layer containing gangliosides was discarded. The chloroform layer was mixed with 1 ml of chloroform-methanol-potassium chloride mixture (1:10:10 v/v) and then centrifuged. This washing was repeated thrice, and each time, the upper layer was discarded. The lower layer was made up to 5 ml and used for the analysis of total cholesterol, triglycerides, free fatty acids, and phospholipids. The serum was also treated similarly for the estimation of lipids.
Biochemical Determination
Blood glucose was estimated colorimetrically using commercial diagnostic kits (Sigma Diagnostics (I) Pvt Ltd, Baroda, India).Citation[24] Plasma insulin was assayed by ELISA using a Boehringer-Mannheim kit with an ES300 Boehringer analyzer (Mannheim, Germany).
The total cholesterol was estimated by the method of Zlatkis et al.Citation[25] Then, 0.1 ml of the serum and lipid extract and 9.9 ml of ferric chloride-acetic acid reagent were added and allowed to stand for 15 min and then centrifuged. After centrifugation, 5 ml of the supernatant was added to 3 ml of conc. H2SO4. The color developed was read after 20 min at 560nm against a reagent blank. Values were expressed as mg/g (tissue) and mg/dL (serum).
HDL cholesterol fraction was separated by the precipitation techniques of Burnstein et al.,Citation[26] and the cholesterol content was determined by method of Zlatkis et al.Citation[25]
VLDL cholesterol was calculated using the following equationCitation[27]:
The values were expressed as mg/dL.
LDL – C was calculated using the following equation:
The values were expressed as mg/dL.
Triglycerides were estimated by the method of Foster and Dunn.Citation[28] Triglycerides are extracted by isopropanol, which upon saponification with potassium hydroxide yields glycerol and soap. The glycerol liberated is treated with metaperiodate, which releases formaldehyde, formic acid, and iodide. The formaldehyde released reacts with acetyl acetone and ammonia, forming a yellow compound, the intensity of which is measured at 420 nm.
Free fatty acids were estimated by the method of Falholt et al.Citation[29] In the presence of phosphate buffer, the lipid extract was shaken with a higher copper reagent (pH 8.1). Free fatty acids in the copper solution were determined colorimetrically with diphenyl carbazide.
Phospholipid content was estimated by the method of Zilversmit and Davis,Citation[30] which involves the conversion of organic phosphorus to inorganic phosphorus that then reacts with ammonium molybdate to form phosphomolybdic acid. This treatment with amino naphthol sulphonic acid forms a stable blue color, which is read at 680 nm.
The ratio between HMG-CoA and mevalonate in hepatic tissue was taken as an index of the activity of HMG-CoA reductase as described by Philipp and Shapiro.Citation[31] The ratio of HMG-CoA to mevalonate was calculated. Lower ratio indicates higher enzyme activity and vice-versa.
Histopathological Study
The liver samples fixed for 48 h in 10% formal-saline were dehydrated by passing successfully in different mixture of ethyl alcohol-water, cleaned in xylene, and embedded in paraffin. Sections of liver (4–5 μm thick) were prepared and then stained with hematoxylin and eosin dye, which mounted in a neutral deparaffinated xylene (DPX) medium for microscopic observations.
Statistical Analysis
The data for various biochemical parameters were analyzed using analysis of variance (ANOVA), and the group means were compared by Duncan's multiple range test (DMRT). Values were considered statistically significant if p < 0.05.Citation[32]
RESULTS
shows the level of blood glucose and plasma insulin of different experimental groups. The diabetic control rats showed a significant increase in the level of blood glucose with a significant decrease in the level of plasma insulin. Oral administration of THC to diabetic rats significantly reversed these biochemical changes. In agreement with these results, the present study also showed that the administration of THC and curcumin significantly improved blood glucose and plasma insulin levels. The THC administration showed to be more effective than curcumin.
Figure 2. Influence of curcumin and THC on the levels of blood glucose and plasma insulin in control and experimental rats. Values are given as mean ± S.D for six rats in each group. Values not sharing a common superscript letter differ significantly at p < 0.05 (DMRT).
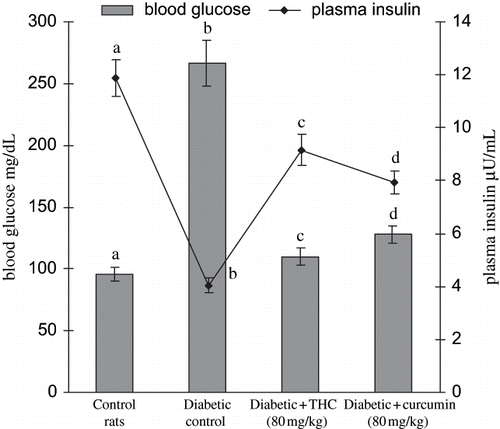
shows the level of serum total lipids, and LDL cholesterol (see ) and VLDL cholesterol (see ) were significantly increased whereas the level of HDL cholesterol (see ) significantly decreased in diabetic rats. The administration of THC and curcumin to diabetic rats reversed all of these changes back toward normal level. The effect of THC was more potent than curcumin.
Figure 3. Influence of THC and curcumin on serum cholesterol, triglycerides, free fatty acids, and phospholipids in control and experimental rats. Values are given as mean ± S.D for six rats in each group. Values not sharing a common superscript letter differ significantly at p < 0.05 (DMRT).
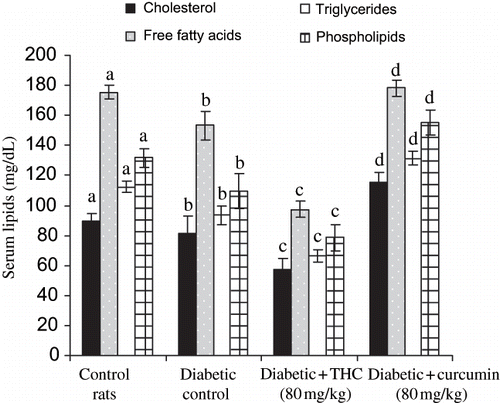
Figure 4. Influence of THC and curcumin on the levels of LDL cholesterol in control and experimental rats. Values are given as mean ± S.D for six rats in each group. Values not sharing a common superscript letter differ significantly at p < 0.05 (DMRT).
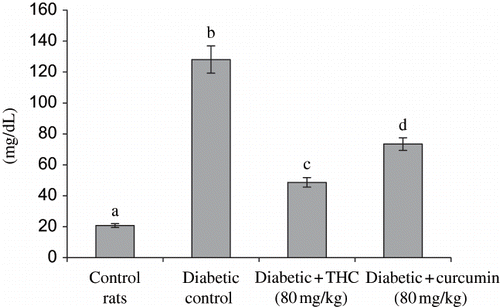
Figure 5. Influence of THC and curcumin on the levels of VLDL cholesterol in control and experimental rats. Values are given as mean ± S.D for six rats in each group. Values not sharing a common superscript letter differ significantly at p < 0.05 (DMRT).
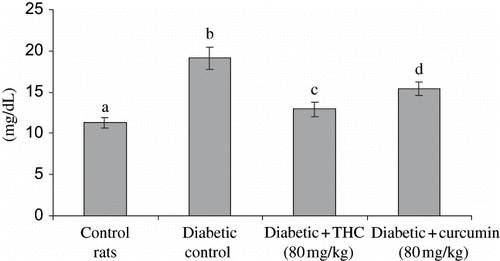
Figure 6. Influence of THC and curcumin on HDL cholesterol levels of control and experimental rats. Values are given as mean ± S.D for six rats in each group. Values not sharing a common superscript letter differ significantly at p < 0.05 (DMRT).
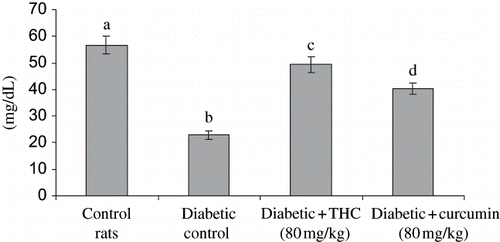
shows the level of liver HMG CoA reductase, cholesterol, triglycerides, free fatty acids, and phospholipids in control and experimental groups. The levels of all these lipids and HMG CoA reductase were significantly increased in diabetic rats, whereas the administration of THC and curcumin to diabetic rats significantly decreased all of these lipids and HMG CoA reductase to near normal level. The effect of THC was more prominent compared with curcumin.
Figure 7. Influence of THC and curcumin on hepatic HMG COA reductase levels of control and experimental rats. A: HMG CoA / Mevalonate ratio. Values are given as mean ± S.D for six rats in each group. Values not sharing a common superscript letter differ significantly at p < 0.05 (DMRT).
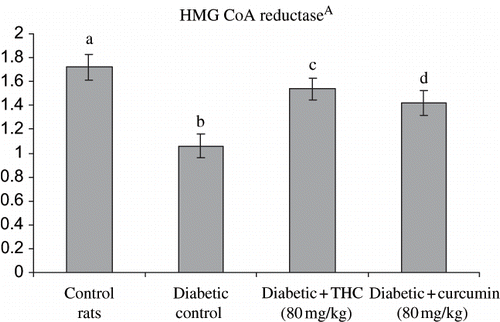
Figure 8. Influence of THC and curcumin on liver cholesterol, free fatty acids, and triglycerides levels of control and experimental rats. Values are given as mean ± S.D for six rats in each group.
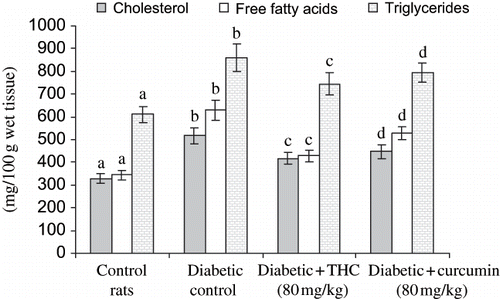
Figure 9. Influence of THC and curcumin liver phospholipids levels of control and experimental rats. Values are given as mean ± S.D for six rats in each group. Values not sharing a common superscript letter differ significantly at p < 0.05 (DMRT).
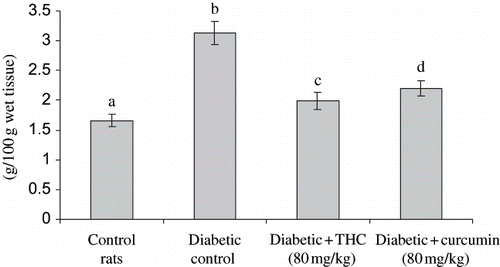
Pathological changes of the liver (see ) include inflammatory infiltration filling over the sinusoidal vacuolation of hepatocytic nuclei (see ) and portal triad with mild inflammation and cell infiltration (see ) in diabetic control rats. The above pathological changes were reduced in rats treated with THC (see ). Curcumin treated rats showed the normal appearance of hepatocytes with mild changes (see ).
Figure 10. Figure A: Control rat liver: H&E × 20; normal architecture of liver. Figure B: Diabetic treated rat liver: H&E × 20; inflammatory infiltration filling over the sinusoidal vacuolation of hepatocytic nuclei. Figure C: Diabetic treated rat liver: H&E × 20; portal triad with mild inflammation and cell infiltration. Figure D: Diabetic + THC treated rat liver: H&E × 20; near normal appearance of hepatocytes. Figure E: Diabetic + curcumin treated rat liver: H&E × 20; near normal appearance with mild changes in hepatocytes.
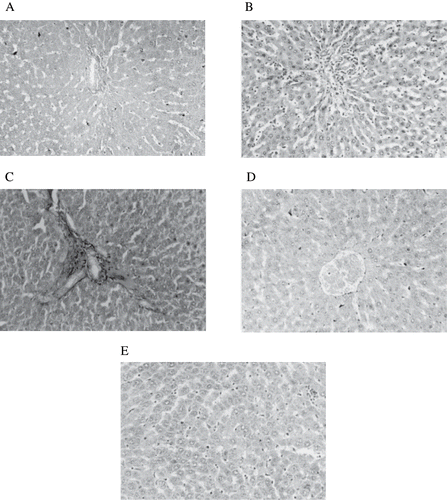
DISCUSSION
Type 2 diabetes is a heterogeneous disorder caused by beta cell dysfunction and reduced insulin sensitivity.Citation[33] Several monogenic forms of disease have been identifiedCitation[34],Citation[35]; however, the most common form of type 2 diabetes is polygenic and multifactorial.Citation[36] Lowering of serum lipid levels through dietary or drug therapy seems to be associated with a decrease in the risk of vascular disease and related complications.Citation[37]
Previously,Citation[20] we reported that THC at 80 mg/kg body weight had a better result than 20 and 40 mg/kg body weight; therefore, the 80 mg/kg body weight was used in this study. We found that THC decreases blood glucose in STZ diabetic rats. The possible mechanism by which THC mediates its antidiabetic action may be the potentiation of pancreatic secretion of insulin from existing β-cell or due to enhanced transport of blood glucose to peripheral tissue. It is evidenced by the significant increase in the level of insulin by THC in diabetic rats.
In this experiment, STZ-induced diabetes mellitus characterized by hyperglycemia caused a significant rise of the serum and liver lipids. These findings indicate that diabetes mellitus is accompanied by complications of atherosclerosis. Lowering of serum lipid levels through dietary or drug therapy seems to be associated with a decrease in the risk of vascular disease.Citation[38] Low-density lipoprotein oxidation is a potential atherogenic agent, and protecting LDL from oxidation prevents atherogenesis.Citation[38] It has been shown that nutrients decrease lipid peroxidation after reoxygenation by means of the initiation of the cardiopulmonary bypass circuit (CPB) when supplemented to the CPB prime, and so they may protect against atherogenesis.Citation[39]
Hyperlipidemia is a recognized complication of diabetes mellitus, characterized by elevated levels of cholesterol, triglycerides, and phospholipids, as well as changes in lipoprotein composition.Citation[40] The results of this study indicate that lipid and lipoprotein abnormalities developed in the diabetic condition were significantly counteracted by administration of THC. Excess fatty acid in serum produced by the STZ-induced diabetes promotes the conversion of some liver fatty acids into phospholipids and cholesterol. These two substances along with excess triglycerides formed at the same time in the liver may be discharged into the blood in the form of lipoproteins.Citation[41] It was observed by Krauss-Friedman that the plasma lipoproteins increase as much as threefold in STZ and nicotinamide-induced diabetes, giving a total concentration of serum lipids of several percent higher than normal. Thus, high lipid concentration may lead to the rapid development of atherosclerosis in diabetic patients.Citation[42] Besides serum cholesterol, the elevated levels of triglycerides and phospholipids are also significantly counteracted by THC.
Increased triglyceride and reduced HDL cholesterol levels are the key characteristics of dyslipidemia in type 2 diabetes.Citation[43] Hypertriglyceridemia in type 2 diabetes can result from an increased hepatic VLDL over production and impaired catabolism of triglyceride-rich particles. The function of lipoprotein lipase (Lpl), a key enzyme in the removal and degradation of triglycerides from circulation, is attenuated by both insulin deprivation and insulin resistance. Dysfunction of Lpl contributes to hypertriglyceridemia in the fasting and postprandial state. It has been postulated that a high plasma triglyceride level influences LDL size and density through a cycle of lipid exchange.Citation[44] The altered composition of lipoproteins in diabetes is facilitated by the action of cholesterol ester transfer protein.Citation[45] A decrease in serum total cholesterol level with an increase in the HDL-C level in diabetic rats treated with THC and curcumin can be ascribed to the reduction of LDL-C and VLDL-C.
β-hydroxy-β- methylglutamyl coenzyme A (HMG-CoA) reductase catalyzes the rate-limiting step in cholesterol biosynthesis, and its activity correlates closely with the rate of tissue cholesterol synthesis. As reported earlier, the activity of HMG-CoA reductase was found to be increased significantly in diabetic rats.Citation[43] The increase in the liver cholesterol in diabetic rats observed in our study could be due to increased cholesterogenesis.Citation[46] The significant increase in the level of extrahepatic cholesterol could be due to the decreased removal of cholesterol from extrahepatic tissues by HDL cholesterol. The increased concentration of free fatty acids in liver may be due to lipid breakdown, and this may cause increased generation of NADPH, which results in the activation of NADPH-dependent microsomal lipid peroxidation.
Accumulation of triglycerides is one of the risk factors in coronary heart disease. The significant increase in the level of triglycerides in the liver of diabetic rats may be due to the lack of insulin. Because insulin activates the enzyme Lpl and hydrolysis triglycerides under normal conditions,Citation[47] THC increases HDL-C. This would be useful in diseases like diabetes mellitus and Coronary Heart Disease (CHD) because of their inverse relationship. THC reduces triglycerides in STZ-induced diabetic rats and may prevent the progression of CHD. Metabolic aberrations in diabetic rats suggest a high turnover of triglycerides and phospholipids. THC may antagonize the metabolic aberration and thereby restore the normal metabolism by tilting the balance from high lipids to high carbohydrate turnover.
In our study, histopathological observation in diabetic control saw inflammatory infiltration filling over the sinusoidal vacuolation of hepatocytic nuclei and portal triad with mild inflammation and cell infiltration in the liver. The reaction is provoked by the increased production of highly reactive intermediates of STZ, which are normally detoxified by endogenous GSH but when present in excess, can deplete GSH stores, allowing the reactive intermediate to react with and destroy hepatic cells.Citation[48] The above pathological changes were reduced in diabetic rats treated with THC and curcumin. The histological evidence of diabetic control rats suggest that structural alterations at the end of 45 days are due to STZ-induced free radical generation quite early in diabetes. Thus, in addition to a blood glucose-lowering effect, histopathological observations also support the notion that THC and curcumin at 80 mg/kg body weight produced significant antihyperglycemic activity by protecting the tissues against STZ action. The protective effect of THC was more prominent compared with curcumin.
Thus our data conclude that THC and curcumin significantly reduce the levels of serum and liver lipids, which are actively raised in STZ and nicotinamide diabetes rats. The mode of action of our compound has additional lipid-lowering properties, and it is considered to have a possible therapeutic value. THC suggests the possibility of biochemical mechanisms that regulate glucose and lipid homeostasis.
REFERENCES
- Howard BV, Savage PJ, Bennion LJ, Bennett PH. Lipoprotein composition in diabetes mellitus. Atheroscler. 1978; 30: 153–162
- Nikkila EA, Kekki M. Plasma triglyceride transport in diabetes mellitus. Metabolism. 1973; 22: 1–22
- Satyanarayana K. Chemical examination of Scoparia dulcis (Linn): Part I. J. Indian Chem. Soc. 1969; 46: 765–766
- Sochor M, Baquer NZ, McLean P. Glucose over and under utilization in diabetes: Comparative studies on the changes in the activities of enzymes of glucose metabolism in rat kidney and liver. Mol. Physiol. 1985; 7: 51–68
- Gupta BL, Baquer NZ. Hexokinase, glucose-6-phosphate dehydrogenase and antioxidant enzymes in diabetic reticulocytes: Effects of insulin and vanadate. Biochem. Mol. Biol. Int. 1998; 46: 1145–1152
- Aggarwal B, Kumar A, Bharti A. Anticancer potential of curcumin: Preclinical and clinical studies. Anticancer Res. 2003; 23: 363–398
- Babu P, Srinivasan K. Hypolipidemic action of curcumin, the active principle of turmeric Curcuma longa in streptozotocin induced diabetic rats. Mol. Cell. Biochem. 1997; 166: 169–175
- Suryanarayana P, Saraswat M, Mrudula T, Krishna P, Krishnaswamy K, Reddy G. Curcumin and turmeric delay streptozotocin-induced diabetic cataract in rats. Invest. Ophthalmol. Vis. Sci. 2005; 46: 2092–2099
- Babu P, Srinivasan K. Amelioration of renal lesions associated with diabetes by dietary curcumin in streptozotocin diabetic rats. Mol. Cell. Biochem. 1998; 181: 87–96
- Sajithlal G, Chithra P, Chandrakasan G. Effect of curcumin on the advanced glycation and cross-linking of collagen in diabetic rats. Biochem. Pharmacol. 1998; 56: 1607–1614
- Nishiyama T, Mae T, Kishida H, Tsukagawa M, Mimaki Y, Kuroda M. Curcuminoids and sesquiterpenoids in turmeric (Curcuma longa L) suppress and increase in blood glucose level in type 2 diabetic KK-Ay mice. J. Agric. Food. Chem. 2005; 53: 959–963
- Mahesh T, Balasubashini M, Menon V. Effect of photo-irradiated curcumin treatment against oxidative stress in streptozotocin-induced diabetic rats. J. Med. Food. 2005; 8: 251–255
- Sidhu G, Mani H, Gaddipati J, Singh A, Seth P, Banaudha K. Curcumin enhances wound healing in streptozotocin induced diabetic rats and genetically diabetic mice. Wound. Rep. Regen. 1999; 7: 362–374
- Holder GM, Plummer JL, Ryan AJ. The metabolism and excretion of curcumin (1,7,-bis(4-hydroxy-3-methoxyphenyl)-1,6-hepadiene-3,5-dione) in rat. Xenobiotica. 1978; 8: 761–768
- Naito M, Wu X, Normura H, Kodama M, Kato Y, Osaswa T. The protective effect of tetrahydrocurcumin on oxidative stress in cholesterol-fed rabbits. J. Atheroscler. Thromb. 2002; 9: 243–250
- Okada K, Wangpoengtrakul C, Tanaka T, Toyokuni S, Uchida K, Osawa T. Curcumin and especially tetrahydrocurcumin ameliorate oxidative stress-induced renal injury in mice. J. Nutr. 2001; 31: 2090–2095
- Pari L, Murugan P. Protective role of tetrahydrocurcumin against erythromycin estolate induced hepatotoxicity. Pharmacol. Res. 2004; 49: 481–486
- Sugiyama Y, Kawakishi S, Osawa T. Involvement of the β-diketone moiety in the antioxidant mechanism of tetrahydrocurcuminoids. Biochem. Pharmacol. 1996; 52: 519–525
- Lin JK, Lin–Shiau SY. Mechanisms of cancer chemoprevention by curcumin. Pro. Natl. Sci. Counc. Repub. China. 2001; 25: 59–66
- Pari L, Murugan P. Effect of tetrahydrocurcumin on blood glucose, plasma insulin and hepatic key enzymes in streptozotocin induced diabetic rats. J. Basic Clin. Physiol. Pharmacol. 2005; 16: 257–274
- Masiello P, Broca C, Gross R, Roye M, Manteghetti M, Hillaire-Buys D. Experimental NIDDM: Development of a new model in adult rats administered streptozotocin and nicotinamide. Diabetes. 1998; 47: 224–229
- Murugan P, Pari L. Antioxidant effect of tetrahydrocurcumin in streptozotocin—nicotinamide induced diabetic rats. Life Sci. 2006; 79: 1720–1728
- Folch J, Lees M, Solane SGH. A simple method for isolation and purification of total lipids from animal tissues. J. Biol. Chem. 1957; 226: 497–509
- Lott JA, Turner K. Evaluation of trinder's glucose oxidase method for measuring glucose in serum and urine. Clin. Chem. 1975; 21/12: 1754–1760
- Zlatkis A, Zak B, Boyle GJ. A method for the determination of serum cholesterol. J. Clin. Med. 1953; 41: 486–492
- Burnstein M, Scholnic MR, Mortin R. Rapid method of isolation of lipoprotein from human serum by precipitation of polyanion. J. Lipid Res. 1970; 11: 583–587
- Friedwerd WT, Levy RI, Fredrickson DS. Estimation of concentration of low-density lipoprotein cholesterol in plasma, without the use of the preparative ultracentrifuge. Clin. Chem. 1972; 18: 499–502
- Foster JB, Dunn RT. Stable reagents for determination of serum triglyceride by colorimetric condensation method. Clin. Chim. Acta. 1973; 19: 338–340
- Falholt K, Falholt W, Lund B. An assay colorimetric method for routine determination of free fatty acids in plasma. Clin. Chim. Acta. 1973; 46: 105–111
- Zilversmit BB, Davis AK. Micro determination of plasma phospholipids by TCA precipitation. J. Lab. Clin. Med. 1950; 35: 155–160
- Philipp B, Shapiro DJ. Improved methods for the assay and activation of 3-hydroxy-3-methylglutaryl-co-enzyme A reductase. J. Food Res. 1979; 20: 588–593
- Duncan BD. Multiple range test for correlated and heteroscedastic means. Biometrics. 1957; 13: 359–364
- Scheen AJ. Pathophysiology of type 2 diabetes. Acta. Clin. Belg. 2003; 58: 335–341
- Maassen JA, Van den Ouweland JM, Losekoot M, Lemkes HH. From gene to disease: Mutation in mitochondrial DNA and maternally inherited diabetes mellitus with deafness (MIDD). Ned. Tijdschr. Geneeskd. 2001; 145: 1153–1154
- Shih DQ, Stoffel M. Molecular etiologies of MODY and other early onset forms of diabetes. Curr. Diab. Rep. 2002; 2: 125–134
- Froguel P, Velho G. Genetic determinants of type 2 diabetes. Recent Prog. Horm. Res. 2001; 56: 91–105
- Brown GB, Xue-Qiao Z, Sacco DE, Alberts JJ. Lipid lowering and plaque regression. New insights into prevention of plaque disruption and clinical events in coronary disease. Circulation. 1993; 87: 1781–1791
- Rhoads GG, Gulbrandse CL, Kagan A. Serum lipoproteins and coronary artery disease in a population study of Hawaiian Japanese men. New. Engl. J. Med. 1976; 294: 293–298
- Yanni AE, Yatzidis HA, Kavantzas NG, Agapitos EV, Perrea DN, Karayannacos PE. Dietary L-aspartate and L-glutamate inhibit fatty streak initiation in cholesterol-fed rabbit. Nutr. Metab. Cardiovasc. Dis. 2003; 13: 80–86
- Segal P, Bachorik PS, Rifkind BM, Levy RI. Lipids and dyslipoproteinemia. Clinical Diagnosis and Management by Laboratory Methods17th, JH Bernard, DA Nelson, RH Tomar, JA Washington, WB Saunders. W.B. Saunders, Philadelphia 1984; 180–203
- Bopanna KN, Kannan J, Sushma G, Balaraman R, Rathod SP. Antidiabetic and antihyperlipidemic effects of Neem seed kernel powder on alloxan diabetic rabbits. Indian J. Pharmacol. 1997; 29: 162–167
- Pushparaj P, Tan CH, Tan BK. Effects of Averrhoa bilimbi leaf extract on blood glucose and lipids in streptozotocin-diabetic rats. J. Ethnopharmacol. 2000; 72: 69–76
- Lehto S, Haffner SM, Pyorala K, Kallio V, Laakso M. Dyslipidemia and hyperglycemia predict coronary heart disease events in middle-aged patients with NIDDM. Diabetes. 1997; 46: 1354–1359
- Taskinen MR, Lahdenpera S, Syvanne M. New insights into lipid metabolism in non-insulin-dependent diabetes mellitus. Ann. Med. 1996; 28: 335–340
- Eisenberg S. Preferential enrichment of large sized very low-density lipoprotein populations with transferred cholesterylesters. J. Lipid Res. 1985; 26: 487–494
- Frayn KN. Insulin resistance and lipid metabolism. Curr. Opin. Lipidol. 1993; 4: 197–204
- Ashokumar N, Pari L, Manimakalai A, Selvarau. Effect of N-benzoyl-D-phenylalanine on streptozotocin induced changes in the lipid and lipoprotein profile in rats. J. Pharm. Pharmacol. 2005; 57: 359–366
- Blum J, Fridovich I. Inactivation of glutathione peroxidase by superoxide radical. Arch. Biochem. Biophys. 1985; 240: 500–508