Abstract
Oxygen metabolites play an important role in the pathogenesis of myoglobinuric acute renal failure (ARF). Previously, we have reported a down regulation of peroxisome proliferator activated receptor γ (PPARγ) in glycerol-induced ARF, and the induction of PPARγ has been shown to provide renal protection. In this study, we determined the protective influence of U74389G, a hydroxyl radical scavenger in myoglobinuric ARF, and its association with PPARγ-mediated renal protection in the rat. Vascular responses to AII were determined in renal pre-glomerular vessels following the induction of ARF with glycerol (50%, v/v, i.m.). The extent of renal damage and function were assessed with or without pre-treatment with U74389G (10 mg/kg × 21 days). In ARF, AII vasoconstriction was enhanced (97%; p < 0.05), and AII production was doubled. U74389G reduced AII vasoconstriction and production by 42% (p < 0.05) and 40% (p < 0.05), respectively. U74389G reduced proteinuria (85%; p < 0.05), which was four times higher in ARF. Similarly, U74389G enhanced Na+ excretion twofold while reducing plasma creatinine (24%; p < 0.05) and BUN (31%; p < 0.05). U74389G attenuated free radical generation in ARF while nitrite excretion was unchanged. In renal pre-glomerular vessel, PPARγ expression, activity, and mRNA were significantly lower in ARF rats; this was unchanged with U74389G treatment. On the other hand, U74389G significantly reduced NFκB protein expression, which was elevated in ARF by 25% (p < 0.05). We suggest that antioxidant U74389G blunted renal injury and improved renal function in glycerol-induced ARF through the reduction of free radical production and/or inhibition of NFκB without affecting PPARγ.
INTRODUCTION
Glycerol-induced acute renal failure (ARF) is characterized by myoglobinuria, tubular necrosis,Citation[1] and enhanced renal vasoconstriction.Citation[2] Although the mechanism by which glycerol induces ARF is not clear, this phenomenon is a widely recognized experimental model of myoglobinuric nephropathy, and the renal vasoconstriction seen in the initial phase of the injury is considered to be an important pathogenic event. Other than tubular necrosis, endothelial damage and vasoconstriction may be important contributors to the ensuing ischemia. Moreover, increased renal production of AII in ARF has been reported.Citation[3] Based on these observations, a potential role exists for AII as mediators of the vasoconstriction and the decrease in renal function seen after glycerol administration. It was reported earlier that the burst of oxygen free radicals in glycerol-induced ARF leads to the altered activity of AII in renal vessels.Citation[4] It has been further pointed out that the hydroxy radical is the species involved,Citation[5] as scavenging of this species offered protection in glycerol-induced ARF.Citation[6] Although the involvement of this oxygen specie is obvious in glycerol-induced ARF, it is not clear how this radical modifies the expression of endogenous vasoconstrictors or their receptors.
Peroxisome proliferator-activated receptors (PPARs) belongs to the nuclear receptor super family and consists of three types of receptors: PPARα, PPARδ (or β), and PPARγ, which are ligand activated transcription factors. PPARs contain a central DNA binding domain that recognizes peroxisome proliferator response elements (PPRE) in the promoters of their target genes. Once bound to the response elements, PPARs activate transcription through a conserved C-terminal ligand binding domain.Citation[7] The binding of the ligand to the ligand-binding domain results in a conformational change in the receptor, which allows transactivation of the appropriate genes.
PPARγ has been found in endothelial and vascular smooth muscle cellsCitation[8] and is known to modulate gene expression in vascular cells.Citation[9] In vascular smooth muscle cells (VSMC), PPARγ activation inhibits proliferation and migration, the release of matrix-degrading enzyme, matrix-metalloproteinase-9 expression, and oxidative stress, suggesting its role in vascular function.Citation[10] The activation of PPARγ suppresses the transcription of angiotensin type-1 receptor (AT1),Citation[11] decreases blood pressure,Citation[12] and ameliorated micro-albuminuria.Citation[13] These observations indicate that a role exists for PPARγ in the regulation of vascular function and systemic hemodynamics.
It is not clear how PPARγ may be involved in the vascular changes observed in glycerol-induced ARF. Glycerol also generates hydrogen peroxide, a potent oxygen free radical in the proximal tubular cells.Citation[14] This iron-dependent generation of hydrogen peroxide is a major contributor to renal failureCitation[4] and also down regulates PPARγ expression.Citation[15] These observations demonstrate that down regulation of PPARγ is associated with free radical-induced impairment of vascular function. Accordingly, in our recent study using the isolated perfused kidney, we demonstrated a reduced PPARγ gene expression and activity in glycerol-induced ARF that was associated with increased free radical activity.Citation[16] These observations led us to hypothesize that a role exists for PPARγ via AII in a free radical-mediated pathology and enhanced vascular reactivity of glycerol-induced ARF. In this study, we explored renal vascular reactivity in glycerol-induced ARF and examined the role of U74389G, a potent hydroxyl radical scavenger, in ARF and its effect on PPARγ in modulating renal function in glycerol-induced ARF.
MATERIALS AND METHODS
All chemicals used in this study were of the highest grade commercially available. Phenylephrine, glycerol, and angiotensin II were obtained from Sigma-Aldrich (St. Louis, Missouri, USA). U74389G was purchased from Cayman Chemical Company (Ann Arbor, Michigan, USA). Antibodies were purchased from Santa Cruz (Santa Cruz, California, USA).
This study was approved by the Texas Southern University Animal Care and Use Committee and was performed according to NIH guidelines for the care and use of laboratory animals.
Animals
Male Sprague-Dawley rats (250–300 g, Harlan Sprague Dawley, Houston, Texas, USA) were used for this study. The animals were housed under standard conditions of light and dark cycle with free access to food and water.
Rats were randomly divided in two groups (i.e., control and ARF) and received either vehicle or treatment. Treatment groups received U74389G (UG), a potent hydroxyl radical scavenger (10 mg/kg/day; orally, 21 days) while the vehicle groups received equal volume of distilled water.
Induction of Acute Renal Failure
ARF was induced using the standard method involving administration of 50% glycerol (v/v, 8 mL/kg, i.m.). After 21 days of study period, in UG or vehicle treated rats, the required amount of glycerol was administered as a deep intramuscular injection equally distributed to both hind legs. Rats were deprived of food and water for 24 hours after glycerol administration.
After injecting glycerol, rats were placed in metabolic cages, and 24 hr urine was collected. Blood was collected via cardiac puncture under pentobarbital anesthesia (Na pentobarbital 50 mg/kg; i.p). Kidneys were processed for isolation of renal microvessels and subsequent biochemical and molecular biology analysis.
Preparation of Isolated Renal Microvessels
A standard in vitro pressurized arteriole preparation was used to study the responses of the renal preglomerular (interlobular) vessels. Preglomerular vessels (intraluminal diameter [ID], 90–120 μm; length 1 mm) were carefully removed, cleaned, and placed in an organ chamber. The ends of the microvessel were cannulated, and the vessels were pressurized to 80 mm Hg and equilibrated for 45–60 min. The tissue was continuously bathed with oxygenated (95% O2/5% CO2) Krebs-Henseleit buffer (118.3 NaCl, 4.7 KCl, 1.2 CaCl2, 1.2 MgSO4, 25.0 NaHCO3, 1.2 KH2PO4, and 5.5 glucose) and maintained at 37°C, pH 7.4. Cumulative concentration-response relationships were established for AII (2.5–25 ng). Angiotensin II (2.5, 5, 10, and 25 ng; in 25 μl volume) was administered randomly, and changes in internal diameter (ID) of renal arterioles were recorded. The doses were given 5–10 min apart, and a drug-tissue contact time of 3 min was allowed to achieve maximum effect. The drugs were washed off with Kreb's solution before adding another. The organ chamber was placed on the stage of an inverted microscope (Olympus BX40; Olympus, Tokyo, Japan) attached to a video camera, a video monitor, and a calibrated video caliper (video micrometer, model JV6000T, Javelin Systems).
Biochemical Analysis
Urinary protein was measured colorimetrically using a kit from Sigma. Urinary excretion of Na (UNaV) was determined by flame photometry (Genway FP7, Jenway Ltd, Essex, UK). Urinary excretion of nitrite (UNOXV) was determined colorimetrically using the Griess assay as described earlier.Citation[16] A commercially available EIA kit (Cayman Chemicals, Ann Arbor, Michigan, USA) was used to determine plasma AII. Plasma level of 8-isoprostane, an indicator of free radical activity, was also measured by an EIA kit (Cayman Chemicals, Ann Arbor, Michigan, USA).
Preparation of Renal Microvascular Extract
After mid-ventral laparotomy, both kidneys were flushed with 20 ml of ice cold (4°C) Kreb's buffer through the left and right renal arteries. Kidneys were removed and cleaned of fats, and a 1–2 mm thick slice was made along the longitudinal axis in each kidney. Each of these slices was examined under dissecting microscope, and renal preglomerular vessels (∼90–120 μM) were isolated. All subsequent procedures were performed at 4°C. Vascular tissues were placed in homogenizing buffer (10 mM potassium phosphate, pH 7.0) and homogenized using a polytron homogenizer. Cytoplasmic and nuclear fractions were separated by standard differential centrifugation and used for Western blot analysis.
Western Blot Analysis of PPARγ and NFκB Protein Expression
Equal amounts (40 μg) of cytosolic (for PPARγ) or nuclear (for NFκB) protein was loaded onto 12% SDS-polyacrylamide gel and subjected to electrophoresis. Separated proteins were blotted onto PVDF membranes (Amersham Biosciences, Piscataway, New Jersey, USA). Membranes were then blocked with blocking buffer (5% milk in Tris-buffered saline containing 0.1% tween [TBST]). After blocking, the membranes were probed with polyclonal anti-PPARγ and NFκB antibody (1:400, dilution, Santa Cruz, California, USA) in blocking buffer overnight at 4°C. The membranes were washed extensively with TBST and then incubated with anti-rabbit IgG conjugated to horseradish peroxidase (1:8000 dilution, Amersham Biosciences) for 1 hr. After extensive washing with TBST, membranes were subjected to enhanced chemiluminescence detection using ECL-Plus from Amersham Biosciences (Piscataway, New Jersey, USA). Signals were captured on x-ray films and quantitation measurements were performed by using a Personal Densitometer SI scanner (PDSI) and Image Quant V 5.0 software from Molecular Dynamics.
PPARγ Activity Assay
PPARγ activity was determined in the nuclear fraction by TransAM PPARγ kit from active motif (Carlsbad, California, USA) following the manufacturer's protocol. Briefly, 10 μg of proteins from nuclear fractions of renal vascular homogenates were added to an oligonucleotide-coated plate and incubated for 1 hr. PPARγ antibody was added and incubated for another hour. Anti-IgG HRP was added at this time and incubated for one more hour. At the end of this period, the plate was washed and developing solution added followed by stop solution. Absorbance was measured within five minutes at 450 nm with a reference wavelength of 655 nm using plate reader (EL808, BIO-TEK Instruments, Inc).
PPARγ Gene Expression by RT-PCR
Renal microvessels were collected under microscope and processed to isolate total RNA using the RNeasy Mini kit (Qiagen, Valencia, California) according to the manufacturer's instructions. Total RNA was quantified, and 100 ng of the RNA was reverse transcribed using TaqMan reverse transcription reagents at 48°C for 30 min. The cDNA was then amplified using TaqMan Universal polymerase chain reaction (PCR) master mix and Assay on Demand primer probe sets for PPARγ, as well as internal control 18S RNA in a real-time PCR system 7300 (Applied Biosystems, Foster City, California, USA). Each PCR amplification was performed in triplicates wells, using the following conditions: 3 min at 95°C, 30 s at 95°C, and 30 s at 60°C through 40 cycles, followed by two cycles of 30 s at 72°C and 1 min at 60°C. The final two cycles were included to perform melt curve analysis to assure that there were no primer dimers. Negative PCR controls including omission of reverse transcriptase or omission of cDNA or primers were used to validate each batch of template before use. Data were collected at the end of 40 cycles and analyzed using the RQ Study software v1.3 (Applied Biosystems). PPARγ gene expression was normalized with 18sRNA and expressed as compared to untreated control.
Statistical Analysis
Response to agonists was expressed as peak changes in ID of vessels from their basal values. Data were presented as mean ± SEM and comparisons were made within each group and between groups using ANOVA and student t-test for significant differences. In all cases, p ≤ 0.05 was considered significant.
RESULTS
Effect of U74389G on Renal Injury and Renal Function
The administration of glycerol resulted in massive proteinuria and deteriorated renal function. illustrates that in ARF rats, proteinuria was about four times higher than the control rats. This was accompanied by a reduction in 24 hr urinary Na+ excretion by 80 ± 25% (p < 0.05). Pretreatment with U74389G blunted proteinuria by 85 ± 12% (p < 0.05) and enhanced reduction in Na+ excretion twofold. Deteriorating renal function was also evident as plasma creatinine (75 ± 5%; p < 0.05) and BUN levels (69 ± 4%; p < 0.05) were higher in ARF rats compared to the control (see ). U74389G prevented this deterioration of renal function and reduced plasma creatinine and BUN by 24 ± 2% (p < 0.05) and 31 ± 2% (p < 0.05), respectively.
Figure 1. Effect of U74389G on renal damage and function. Urinary excretion of protein (, left) and Na+ (right) or plasma creatinine (, left) and BUN (right) in different groups of control or ARF rats treated with vehicle (Veh) or U74389G (UG: 10 mg/kg/day; orally) for 21 days. Values are mean ± SEM. *p < 0.05 versus control; #p < 0.05 versus ARF; n = 6 rats/group. Abbreviations: control = untreated rats; Cont+UG = control rats treated with U74389G; ARF = glycerol-induced acute renal failure; ARF+Veh = ARF rats treated with vehicle; ARF+UG = ARF rats treated with U74389G.
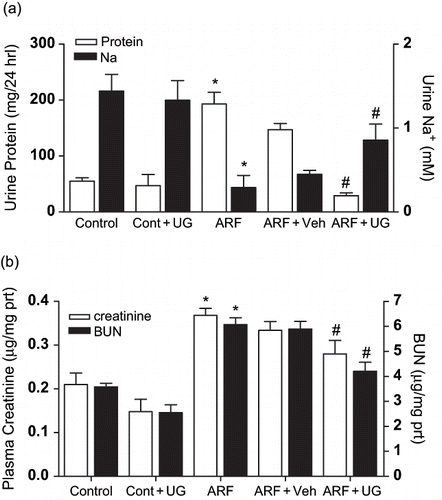
Effect of U74389G on Free Radical Activity and NO Production
Glycerol increased 8-isoprostane level in plasma, an indicator of free radical generation, by three-fold, suggesting an increased free radical generation (see ). Pre-treatment with U74389G blunted free radical generation in ARF rats plasmas, as evident by a reduction in 8-isoprostane by 30 ± 3% (p < 0.05). Urinary excretion of nitrite (UNOxV) was determined to establish NO production in response to glycerol administration (see ). UNOxV was significantly attenuated in ARF rats compared to the control (from 0.99 ± 0.1 to 0.49 ± 0.08 μmol/24 hr), which was unchanged after U74389G treatment.
Figure 2. Effect of U74389G on free radical activity and NO production. Plasma 8-Isoprostane () and urinary nitrite excretion () in different groups of control or ARF rats treated with vehicle (Veh) or U74389G (UG: 10 mg/kg/day; orally) for 21 days. Values are mean ± SEM. *p < 0.05 versus control; #p < 0.05 versus ARF; n = 6 rats/group. Abbreviations: control = untreated rats; Cont+UG = control rats treated with U74389G; ARF = glycerol-induced acute renal failure; ARF+Veh = ARF rats treated with vehicle; ARF+UG = ARF rats treated with U74389G.
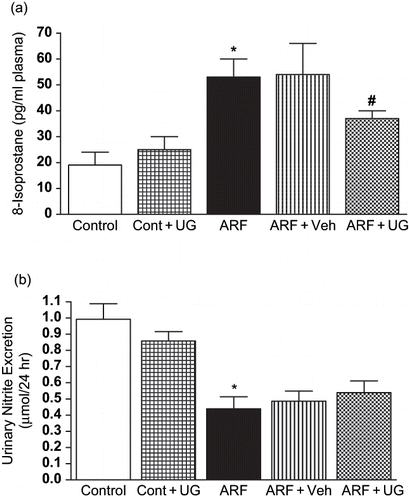
Effect of U74389G on Renal Pre-Glomerular Vascular Reactivity to AII and AII Production
The induction of ARF by glycerol increased renal pre-glomerular vascular response to AII by 97 ± 26% (p < 0.05). This was accompanied by an increased production of AII in ARF rats (4.47 ± 0.7 vs. 2.05 ± 0.3 pg/mg protein in control rats). Treatment with U74389G blunted the increase in AII vasoconstriction by 42 ± 6% (p < 0.05; see ). Similarly, U74389G treatment in ARF attenuated the increase in AII production by 40 ± 9% (p < 0.05; see ).
Figure 3. Effect of U74389G on renal micro vascular reactivity and plasma. AII vasoconstrictor response to angiotensin II in renal microvessel () and plasma angiotensin II level () in different groups of control or ARF rats treated with vehicle (Veh) or U74389G (UG: 10 mg/kg/day; orally) for 21 days. Values are mean ± SEM. *p < 0.05 versus control; #p < 0.05 versus ARF; n = 6 rats/group. Abbreviations: control = untreated rats; Cont+UG = control rats treated with U74389G; ARF = glycerol-induced acute renal failure; ARF+Veh = ARF rats treated with vehicle; ARF+UG = ARF rats treated with U74389G).
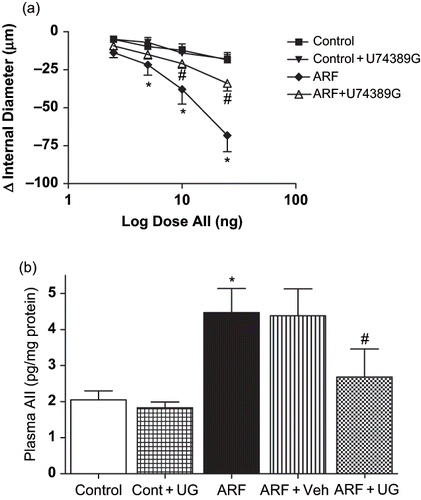
Effect of UG on PPARγ Protein Expression or Activity and mRNA Expression
When compared to the control rats, glycerol-induced ARF rats exhibits a reduced (23 ± 2%; p < 0.05) PPARγ protein expression (see ). This was also associated with a reduced PPARγ activity (36 ± 3%; p < 0.05) determined in the nuclear fraction from the vascular homogenate (see ). Although vehicle-treated ARF rats showed an increase in PPARγ protein expression, PPARγ protein expression and activity were unchanged in ARF rats after treatment with U74389G. Similarly, qRT-PCR results (see ) reveals that U74389G did not evoke any changes in PPARγ mRNA levels in ARF rats, which were reduced after the administration of glycerol by 24 ± 1% (p < 0.05).
Figure 4. Effect of U74389G on PPARγ protein expression or activity and mRNA in renal microvessel. PPARγ protein expression (), activity (), and mRNA expression () in the renal microvascular homogenates from control and ARF rats treated with U74389G (UG: 10 mg/kg/day; orally) or vehicle (Veh) for 21 days. Values are mean ± SEM. *p < 0.05 versus control; n = 6 rats/group. Abbreviations: control = untreated rats; Cont+UG = control rats treated with U74389G; ARF = glycerol-induced acute renal failure; ARF+Veh = ARF rats treated with vehicle; ARF+UG = ARF rats treated with U74389G.
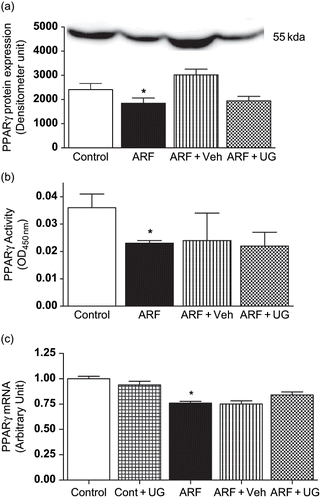
Effect of U74389G on NFκB Protein Expression
shows that in ARF rats, NFκB protein expression in nuclear fraction from homogenates of renal pre-glomerular vessel was increased by 25 ± 4% (p < 0.05). In ARF rats pre-treated with U74389G, NFκB expression was reduced by 23 ± 2% (p < 0.05).
Figure 5. Effect of U74389G on NFκB protein expression. Transcription factor NFκB protein expression in the microvascular homogenate (nuclear fraction) from kidneys of control and ARF rats treated with U74389G (UG: 10 mg/kg/day; orally) or vehicle (Veh) for 21 days. Values are mean ± SEM. *p < 0.05 versus control; #p < 0.05 versus ARF; n = 6 rats/ group. Abbreviations: control = untreated rats; Cont+UG = control rats treated with U74389G; ARF = glycerol-induced acute renal failure; ARF+Veh = ARF rats treated with vehicle; ARF+UG = ARF rats treated with U74389G.
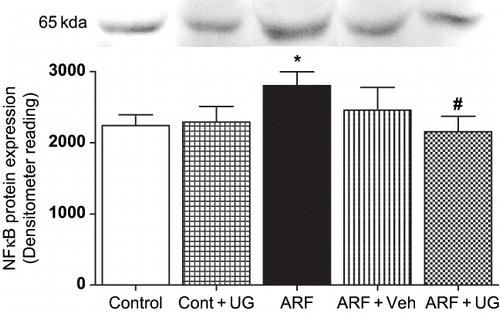
DISCUSSION
In the most widely used model of experimental myoglobinuric ARF, a single intramuscular injection of hypertonic solution of glycerol to the hind legs of rodents induces ARF with the similar symptoms observed in human rhabdomyolysis. This model was used in the present study to produce severe renal injury as evidenced by proteinuria, increased plasma creatinine, BUN, and a decrease in Na+ excretion. Treatment with antioxidant, U74389G (UG), protected against a glycerol-induced effect by reducing generation of free radicals, increasing Na+ excretion, reducing expression of NFκB, and improving vascular reactivity to AII.
Free radical generation has been implicated as one of the mechanisms involved in glycerol-induced ARF.Citation[4] It is known that at a high level, oxidative stress causes damage to cellular macromolecules such as membrane lipids, DNA and proteins. There are reports that high levels of free radicals causes tissue injury,Citation[17] and the use of free radical scavengers provides protection.Citation[18] In a previous study, we reported an increase in free radical activity in glycerol-induced ARF.Citation[16] As a follow up, glycerol treatment in this study resulted in a marked renal oxidative stress, as evident by high levels of 8-isoprostane, and significantly deranged renal function, as evidenced by increased plasma creatinine, BUN, and a decrease in Na+ excretion. In this study, treatment with UG, an antioxidant, reduced the generation of free radicals and improved kidney function consistent with the involvement of free radicals in deteriorating renal function in this model of ARF.
In our previous studies and those from other laboratories, the induction of ARF by glycerol caused a decrease in nitric oxide production.Citation[16],Citation[19] Enhanced renal vasoconstriction has also been reported in ARF,Citation[3],Citation[20] and our recent study also demonstrates an increased in response to AII.Citation[16] In many studies, the activation of PPARγ was shown to improve vascular function and reduce vasoconstrictor response to agonists.Citation[10] Because PPARγ transcriptionally regulates the production and activity of major endogenous vasoconstrictors like AII,Citation[11] it is possible that the alteration of vascular reactivity to AII observed in glycerol-induced ARF was mediated through an impaired transcriptional regulation of AII production by PPARγ that may be preceded by a free radical-mediated down regulation of PPARγ or its downstream effector genes. In our previous studies using the isolated kidney preparation, we reported an exaggerated renal vascular response to AII in ARF,Citation[20] which could be attributed to an increased free radical activity.Citation[16] In the present study, we evaluated the alteration in renal microvascular reactivity with increased generation of free radicals and alteration in PPARγ expression and activity. Treatment with UG although did not affect NO production but normalized the enhanced vasoconstrictor response to AII. As such, the generation of free radicals and alteration of the redox state of the cells can cause formation of endogenous vasoconstrictorsCitation[21] that may directly affect renal function by altering renal responses to vasoconstrictors. In support of this notion, an increased renal production of AII was observed in this study, an observation in agreement with earlier reports.Citation[3] AII is an important regulator of blood pressure and vascular resistance,Citation[22] and in glycerol-induced ARF, the burst of oxygen free radicals has been reported to lead altered activity of AII in renal vessels.Citation[4] We therefore interpret the finding in this study that UG, an antioxidant, provided beneficial effect in ARF by scavenging free radicals, thereby reducing vasoconstrictor response to AII.
The involvement of PPARs in cardiovascular diseases has been reported by several investigators.Citation[12],Citation[13] High levels of free radicals can affect the expression and activity of many proteins including PPARγ.Citation[4] PPARγ has been found in endothelial cells and vascular smooth muscle cellsCitation[8] and is involved in the regulation of vascular tone. Previously, using isolated perfused kidney, we have shown a reduced PPARγ protein expression and activity in glycerol-induced ARF.Citation[16] Similarly, this study also demonstrates a reduced PPARγ expression and activity in renal micro vessels from glycerol-induced ARF rats. This alteration can be the result of the degradation of PPARγ protein by high levels of free radicals. In this study, we expected to see an improvement in PPARγ protein expression. On the contrary, UG did not affect the expression or activity of this protein. These findings suggest that though free radicals affect PPARγ expression and activity, the beneficial effects provided by UG in ARF rats resulted from a direct antioxidant effect. These observations led us to investigate further expression of mRNA or protein in order to evaluate whether there is any post-transcriptional or post-translational modification of PPARγ. The observation showing unchanged PPARγ mRNA expression despite UG treatment further confirms our earlier observation that UG do not affect PPARγ gene expression; hence, the down regulation of PPARγ gene expression observed in ARF was unaffected by antioxidant UG.
The generation of oxygen free radicals can also activate other transcription factors such as NFκB,Citation[21] which has been reported to be involved in multiple organ dysfunction involving the liver, heart, lungs, and kidney.Citation[23],Citation[24] The speculation that the beneficial effect of UG observed in glycerol-induced ARF might be through a mechanism not involving PPARγ led us to NFκB as a possible mediator of UG in protecting against glycerol-induced ARF. Antioxidants such as UG can protect not only against direct free radical toxicity but also through their ability to inhibit the activation of NFκB and its subsequent damage.Citation[25] We verified this possibility and provided evidence that UG attenuated the increased expression of NFκB in glycerol-treated animals. Furthermore, a recent study by de Jesus Soares and colleagues shows that the inhibition of NFκB activation reduces glycerol-induced renal injury.Citation[26]
In conclusion, data from this study suggest that antioxidant UG blunted free radical-mediated renal injury, improved renal function, and diminished the enhanced vascular reactivity to AII in glycerol-induced ARF through a reduction in free radical production and/or inhibition of NFκB without affecting PPARγ.
ACKNOWLEDGMENT
This study was supported by American Heart Association, Texas Affiliate grant 0365001Y to Dr. Mohammad Newaz, and National Institutes of Health grant HL03674 to Dr. Adebayo Oyekan. We acknowledge the use of Research Centers in Minority Institutions (RCMI) facilities of Texas Southern University for this study.
REFERENCES
- Karam H, Bruneval P, Clozel JP, Loffler BM, Bariety J, Clozel M. Role of endothelin in acute renal failure due to rhabdomyolysis in rats. J Pharmacol Exp Ther. 1995; 274(1)481–486
- Venkatachalam MA, Rennke HG, Sandstrom DJ. The vascular basis for acute renal failure in the rat. Preglomerular and postglomerular vasoconstriction. Circ Res. 1976; 38(4)267–279
- Yanagisawa H, Nodera M, Umemori Y, Shimoguchi Y, Wada O. Role of angiotensin II, endothelin-1, and nitric oxide in HgCl2-induced acute renal failure. Toxicol Appl Pharmacol. 1998; 152(2)315–326
- Baliga R, Ueda N, Walker PD, Shah SV. Oxidant mechanism in toxic acute renal failure. Drug Metab Rev. 1999; 31(4)971–997
- Shah SV, Walker PD. Reactive oxygen metabolites in toxic acute renal failure. Ren Fail. 1992; 14(3)363–370
- Zager RA. Rhabdomyolysis and myohemoglobinuric acute renal failure. Kidney Int. 1996; 49: 314–326
- Forman BM, Chen J, Evans RM. Hypolipidemic drugs, polyunsaturated fatty acids, and eicosanoids are ligands for peroxisome proliferator-activated receptors alpha and delta. Proc Nat Acad Sci 1997; 94: 4312–4317
- Law RE, Goetze S, Xi XP, Jackson S, Kawano Y, Demer L, Fishbein MC, Meehan WP, Hsuch WA. Expression and function of PPARγ in rat and human vascular smooth muscle cells. Circulation. 2000; 101: 1311–1318
- Marx N, Duez H, Fruchart JC, Staels B. Peroxisome proliferator-activated receptors and atherogenesis: Regulator of gene expression in vascular cells. Circ Res. 2004; 94: 1168–1178
- Touyz RM, Schiffrin EL. Peroxisome proliferator activated receptors in vascular biology-molecular mechanism and clinical implications. Vascul Pharmacol. 2006; 45(1)19–28
- Sugawara A, Takeuchi K, Uruno A, Ikeda Y, Arima S, Kudo M, Sato K, Taniyama Y, Ito S. Transcriptional suppression of type-1 angiotensin II receptor gene expression by peroxisome proliferator activated receptor gamma in vascular smooth muscle cells. Endocrinology. 2001; 142(7)3125–3134
- Ogihara T, Rakugi H, Ikegami H, Mikami H, Masuo K. Enhancement of insulin sensitivity by troglitazone lowers blood pressure in diabetic hypertensives. Am J Hypertens. 1995; 8: 316–320
- Imano E, Kanda T, Nakatani Y, Nishida TM, Arai K, Motomura M, Kajimoto Y, Yamasaki Y, Hori M. Effect of troglitazone on microalbuminuria in patients with incipient diabetic nephropathy. Diabetes Care. 1998; 21: 2135–2139
- Zager RA. Combined mannitol and deferoxamine therapy for myohemoglobinuric renal injury and oxidant tubular stress. Mechanistic and therapeutic implications. J Clin Invest. 1992; 90(3)711–719
- Itoh H, Doi K, Tanaka T, Fukunaga Y, Hosoda K, Inoue G, Nishimura H, Yoshimasa Y, Yamori Y, Nakao K. Hypertension and insulin resistance: role of peroxisome proliferator activates receptor gamma. Clin Exp Pharmacol Physiol. 1999; 26(7)558–560
- Newaz MA, Yousefipour Z, Oyekan AO. Role of PPARγ on the pathogenesis and vascular changes in glycerol-induced acute renal failure. J Pharmacological Research. 2006; 54(3)234–240
- Juranek I, Bezek S. Controversy of free radical hypothesis: reactive oxygen species—cause or consequence of tissue injury?. Gen Physiol Biophys. 2005 Sep; 24(3)263–278
- Khan SR. Hyperoxaluria-induced oxidative stress and antioxidants for renal protection. Urol Res. Nov, 2005; 33(5)349–357, E-pub 2005 Nov 15.
- Chander V, Chopra K. Protective effect of resveratrol, a polyphenolic phytoalexin on glycerol-induced acute renal failure in rat kidney. Ren Fail. 2006; 28(2)161–169
- Newaz MA, Oyekan AO. Vascular responses to endothelin-1, angiotensin-II, and U46619 in glycerol-induced renal failure. J Cardiovasc Pharmacol. 2001; 38(4)569–577
- Martindale JL, Holbrook NJ. Cellular response to oxidative stress: Signaling for suicide and survival. J Cell Physiol. 2002; 192(1)1–15
- Arakawa K, Urata H. Hypothesis regarding the pathophysiological role of alternative pathways of angiotensin II formation in atherosclerosis. Hypertension. 2000; 36(4)638–641
- Tu B, Gong JP, Feng HY, Wu CX, Shi YJ, Li XH, Peng Y, Liu CA, Li SW. Role of NF-kB in multiple organ dysfunction during acute obstructive cholangitis. World J Gastroenterol. 2003; 9(1)179–183
- Cao CC, Ding XQ, Ou ZL, Liu CF, Li P, Wang L, Zhu CF. [Transfection of nuclear factor-kappaB decoy oligodeoxynucleotides prevents ischemic acute renal failure in rats]. Zhonghua Yi Xue Za Zhi. 2003; 83(18)1597–1602
- Suzuki YJ, Forman HJ, Sevanian A. Oxidants as stimulators of signal transduction. Free Rad Biol Med. 1997; 22(1–2)269–285
- Soares T, de J, Costa RS, Balbi APC, Coimbra TM. Inhibition of nuclear factor-kappa B activation reduces glycerol-induced renal injury. J Nephrol 2006; 19: 439–448