Abstract
During times of war and natural disasters, rhabdomyolysis-induced myoglobinuric acute renal failure (ARF) can assume epidemic proportions. Free radicals play an important role in the pathogenesis of myoglobinuric ARF. Vitamin C is a major antioxidant, scavenging free radicals. We have not found any studies on the effect of vitamin C on myoglobinuric ARF. Thus, we aimed to investigate the effects of vitamin C on the myoglobinuric ARF formed by glycerol in rats. Three groups of rats were employed in this study. Group 1 served as control, group 2 was given 50% glycerol (10 mL/kg, i.m.), and group 3 was given glycerol plus vitamin C (20 mg/kg, i.p. for four days). Ninety-six hours after glycerol injections, blood samples and kidney tissues were taken from the anesthetized rats. Urea and creatinine levels in plasma; N-acetyl-beta-D-glucosaminidase activity in urine; malondialdehyde levels, superoxide dismutase and catalase enzyme activity in kidney tissue were determined. Histopathological changes and iron accumulation in the kidney tissue were evaluated. In this study, glycerol administration led to marked renal oxidative stress and severe renal functional and morphological deterioration. The treatment of animals with vitamin C partially corrected the renal dysfunction and morphological impairment. In this respect, vitamin C appears to be a promising candidate for the prevention of rhabdomyolysis-induced ARF. Higher dosages of vitamin C than in 20 mg/kg may be beneficial for better functional and morphological recovery in this model ARF.
INTRODUCTION
During times of war and natural disasters, rhabdomyolysis-induced myoglobinuric ARF can assume epidemic proportions.Citation[1] The main pathophysiologic mechanisms are renal vasoconstriction, intraluminal cast formation, and direct heme protein-induced cytotoxicity.Citation[2],Citation[3] Several studies have revealed that free radicals play an important role in the pathogenesis of myoglobinuric ARF.Citation[4–7]
Myoglobin, the major heme-containing protein, is bound to plasma proteins, and only small amounts of it reach urine. When massive amounts of myoglobin are released, the binding capacity of the plasma protein is exceeded. Then, myoglobin (∼19 kD) is easily filtered by the glomeruli and reaches the tubules. The porphyrin ring is rapidly catabolized, releasing its iron content.Citation[2],Citation[8–10] Any released free ferrous (Fe2+) iron could participate in the Fenton and Haber-Weiss chemistry, where catalytic amounts of iron are sufficient to yield hydroxyl radicals (OH°) from superoxide anyon (O2−) and hydrogen peroxide (H2O2).Citation[8–10] Hydrogen peroxide is capable of abstracting a hydrogen atom from polyunsatured fatty acids to initiate lipid peroxidation.Citation[8] It has been shown that without invoking the release of free iron, the heme group in myoglobin itself can redox cycle between different oxidation states and promote lipid peroxidation reactions as a consequence of its ability to decompose lipid hydroperoxides to peroxyl and alkoxyl radicals.Citation[11–13] Peroxidation of lipid structures in the kidney results in extensive cellular damage.Citation[14]
Vitamin C acts as a potent water-soluble antioxidant in biological fluids by scavenging physiologically relevant reactive oxygen species (i.e., O2−, H2O2, singlet oxygen) and reactive nitrogen species.Citation[15–18] Vitamin C has been shown to protect against various types of injuries involving oxidative stress.Citation[19–23] Thus far, there appear to be no studies on the effect of vitamin C on myoglobinuric ARF. We aimed to investigate the effects of vitamin C on the development of acute myoglobinuric renal failure formed by glycerol in rats.
MATERIALS AND METHODS
Animals
Male Spraque Dawley rats, bred in the Experimental Research Center of Trakya University (Edirne, Turkey), were used in this study. The rats were housed in an air-conditioned room with 12 h light-dark cycles, where the temperature (21 ± 2°C) and relative humidity (60–65%) were kept constant. The study protocol was approved by the Ethics Committee of Trakya University Faculty of Medicine.
Drugs
Glycerol was purchased from Merck KGaA, vitamin C was purchased from Roche Chemicals and Pharmaceutics, and all other chemicals and reagents used were of analytical grade.
Study Design
Rats were divided into three groups, each comprising 10 animals. The animals were allowed free access to food but deprived of drinking water for 24 h before glycerol injection. Group 1 comprised the control group and received the equivalent volume of saline solution for glycerol. Rhabdomyolysis was induced by injecting glycerol (50% vol/vol in sterile saline), 10 mL/kg body weight; half of the dose was administered in each hind limb muscle in groups 2 and 3. Group 2 animals (ARF group) received saline solution intraperitoneally in an equal dose to the vitamin C in Group 3, immediately after glycerol injection. Group 3 animals (ARF-VC Group) received vitamin C intraperitoneally (20 mg/kg), immediately after glycerol injection. The intraperitoneal injections of saline or vitamin C were repeated every 24 h for four days in the ARF and ARF-VC groups. The animals were placed in individual metabolic cages after the glycerol injection for 24 h urine collections while allowing free access to food and water. Three rats died during the follow-up period: two rats in the ARF group and one rat in the ARF-VC group. The dying rats were substituted. At the end of the 96 h, rats were anesthetized with 10 mg/kg xylazine + 50 mg/kg ketamine, a midline abdominal incision was performed, and then the animals were neutralized by collecting their blood via intracardiac punction. Kidneys were harvested: the left kidney was deep frozen for further enzymatic analysis, whereas the right kidney was stored in 10% formalin for the histological sectioning. Urine and blood samples were centrifuged immediately (3000 g for 5 min at 4°C), and samples were stored at −84°C until assay.
Renal Function, Electrolyte Assays
Biochemical analyses of serum and urine were made using the specific Abbott kits in Abbott C8000 Architect auto analyzer. Creatinine clearance was calculated as mL/min/100 g body weight. Urinary N-acetyl-beta-D-glucosaminidase (NAG) activity, a reliable marker of the tubular cell damage, was determined using a Bohringer Mannheim BiochemicaR (No. 875406) kit in a Shimadzu UV 160 A spectrophotometer. Urinary NAG activity to urinary creatinine excretion ratio was calculated as U/mg.
Malondialdehyde Assay
Kidney tissue samples were homogenized with ice-cold 150 mmol/L KCl for the determination of malondialdehyde (MDA) levels. Homogenates were centrifuged at 2600 g for 10 min at 4°C. The MDA concentrations of renal tissue, a measure of lipid peroxidation, were assayed in the form of thiobarbituric acid-reactive substances.Citation[24] Supernatant (200 μL) was added to 0.2 mL of 8.1% sodium dodecyl sulphate, 1.5 mL of 20% acetic acid (pH 3.5), 1.5 mL of 0.8% thiobarbituric acid, and 0.6 mL distilled water. This mixture was heated to 95°C for 60 min. After cooling with tap water, 1.0 mL distilled water and 5.0 mL n-butanol-pyridine mixture (15 : 1, v/v) were added, and samples were shaken vigorously and centrifuged at 2600 g for 10 min at 25°C. The absorbance of the organic layer was read at 532 nm. MDA was quantified using an extinction coefficient of 1.56 × 105 L/mol per cm and kidney levels expressed as nmol MDA/mg tissue.
Enzyme Assays
Enzyme activities were determined after renal tissue homogenization with phosphate-buffered saline at pH 7.4. SOD activity was estimated in the homogenate by cytochrome c reduction in a xanthine-xanthine oxidase-generating system.Citation[25] The SOD activity was determined from a standard curve of percentage of inhibition of cytochrome c reduction with known SOD activity. The specific activity is expressed in units per milligram protein.
Catalase activity was measured according to the Aebi's method.Citation[26] The principle of the catalase activity measurement is based on the determination of the rate constant (k; /s) of the decomposition of hydrogen peroxide at 240 nm. Results are expressed as the rate constant per gram tissue protein.
Kidney Morphological Studies
Right kidneys were cut longitudinally and fixed in 10% formalin solution. Tissues were embedded in paraffin wax, and 4 μm sections were stained with hematoxylin and eosin. The extent of histological renal tubular necrosis and tubular cast formation for each animal were evaluated semiquantitatively by a pathologist analyzing the kidney sections without knowledge of the treatment groups. The extent of tubular injury was evaluated in terms of the percentage area of sections showing a given level of histological damage. In addition, 4 μm sections of paraffin-embedded tissues stained were with iron stain (procedure no. HT 20; Sigma Diagnostics, St. Louis, Missouri, USA). The percentage of iron accumulation for each group was calculated after semiquantitative evaluation of the granular blue-coloured areas. In the present study, a special ocular (Nikon, Tokyo, Japan), which has 400 cells, was used to detect the percentage of casts, necrosis, and iron accumulation.
Statistical Analysis
Data were expressed as mean ± SD. All statistical analyses were conducted with the SPSS/PC 10.0 statistical software package. The Kruskal-Wallis test was used to compare the three groups. In two-group comparisons, the Mann-Whitney U test was used. A Pearson correlation test was performed to evaluate the relationship between the parameters in the groups. A value of p <0.05 was accepted as statistically significant.
RESULTS
As shown in , glycerol administration resulted in a significant increase in urea and creatinine levels, as well as in urinary NAG activity; it also decreased creatinine clearance significantly. Treatment with vitamin C partially improved renal dysfunction when compared to the ARF group.
Table 1 Biochemical results of study groups
The levels of kidney tissue MDA significantly increased in rats treated with glycerol as compared to the control rats. The administration of vitamin C to glycerol-treated rats significantly decreased the levels of MDA (see ).
A significant decrease in the activities of enzymatic antioxidants was observed in glycerol-treated rats. Treatment with vitamin C significantly increased the activity of catalase and increased the activity of SOD in glycerol-treated rats (see ).
A Pearson correlation test showed some relationship between parameters in glycerol-treated rats (ARF and ARF-VC groups). Kidney tissue MDA levels were positively correlated with tubular cell necrosis (r = 0.480, p = 0.032; see ) and tubular iron accumulation (r = 0.482, p = 0.031, see ). Tubular cell necrosis was positively correlated with tubular iron accumulation (r = 0.514, p = 0.020, see ).
Figure 1. Scattergram showing the relation between kidney tissue MDA levels (in nmol/mg tissue) and tubular cell necrosis (in %) in patient groups (ARF and ARF-VC groups).
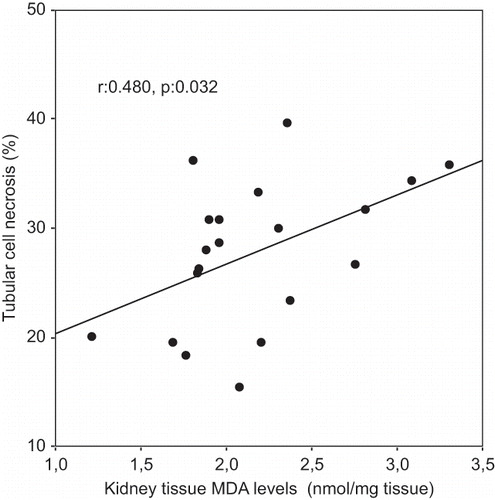
Figure 2. Scattergram showing the relation between kidney tissue iron accumulations (in %) and kidney tissue MDA levels (in nmol/mg tissue) in patient groups (ARF and ARF-VC groups).
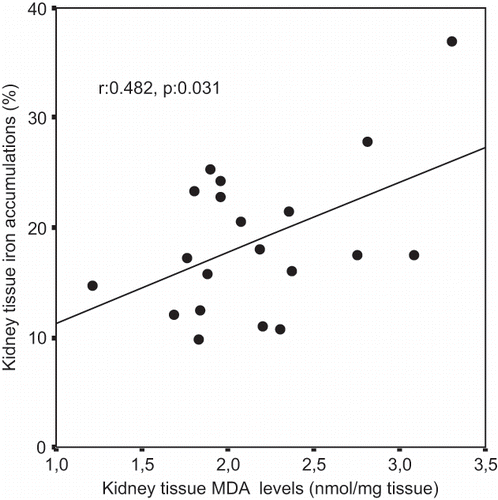
Figure 3. Scattergram showing the relation between kidney tissue iron accumulations (in %) and tubular cell necrosis (in %) in patient groups (ARF and ARF-VC groups).
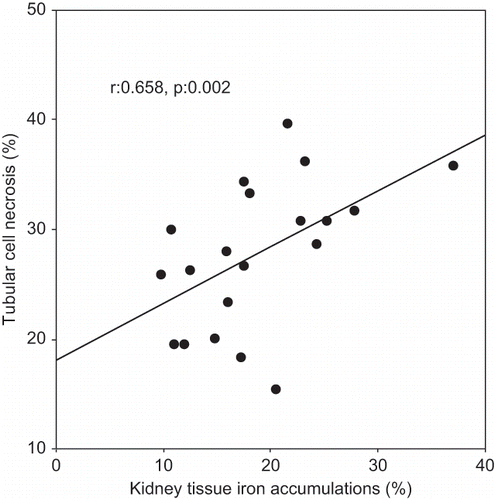
The histological changes in all groups were examined, and the results are given in . The control group did not show any histopathological changes (see and ). In contrast, in the ARF group, the basic histological abnormalities were tubular necrosis and cast formation. Necrosis was most severe in the cortical segments of the proximal tubules, and less extensive changes were observed in the medullar segment of the kidney (see ). Blue granular deposits showed iron accumulation in the tubular system (see ). Tubular cell necrosis, cast formation, and iron accumulation partially decreased with vitamin C treatment (see and ).
Table 2 Histological findings in study groups
Figure 5. Kidney section of a glycerol+saline-treated rat (ARF group) showing severe tubular necrosis and cast formation (H&E, × 100).
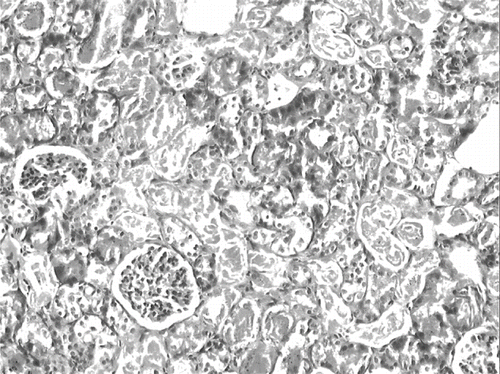
Figure 6. Kidney section of a glycerol + vitamin C-treated rat (ARF-VC group). The level of tubuler necrosis and cast formation is decreased compared with the ARF group (H&E, × 100).
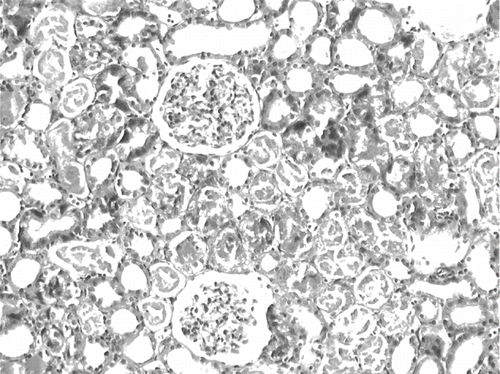
Figure 7. Kidney section of a control rat. There are no granular deposits in iron-stained section (iron stain, × 100).
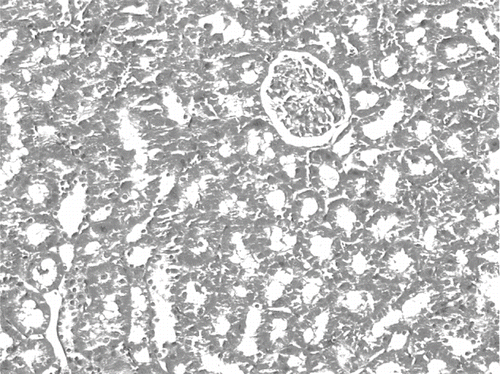
DISCUSSION
In the present study, glycerol-induced renal damage was accompanied by significant depletion of antioxidant enzymes in the kidney, as well as in enhanced lipid peroxidation. These results are in agreement with those reported earlier by other researchers. In several studies, it has been demonstrated that free radicals play an important role in the pathogenesis of myoglobinuric ARF based on the findings that in rats with rhabdomyolysis, levels of MDA and F2 isoprostanes in the kidney are increased.Citation[4–7],Citation[27–29]
The protective effect of vitamin C against oxidative injury has been shown in various models, such as paracetamol-induced nephrotoxicity in rats,Citation[19] gentamicin-induced nephrotoxicity in Guinea pigs,Citation[20] arsenic-induced liver and kidney damage in rats,Citation[21] cisplatin-induced nephrotoxicity in adult rats,Citation[22] and vancomycin-induced nephrotoxicity in rats.Citation[23] In the present study, vitamin C treatment produced a significant reduction in renal tissue MDA levels, which reflects lipid peroxidation; a significant increase in renal tissue catalase, which catalyzes the decomposition of H2O2; a slight increase in renal tissue SOD, which catalyzes the dismutation of O2− to H2O2 and dioxygen, providing a first line of defense against O2−-induced damage. Also, renal functional and morphologic impairment, observed with glycerol injection, was partially corrected by vitamin C treatment.
The protective effect of vitamin C appears to be mainly due to its free radical scavenger effects. Vitamin C has been shown to react directly with physiologically relevant reactive oxygen species and reactive nitrogen species. These include free radicals such as OH−, O2−, singlet oxygen, aqueous peroxyl radicals, and nitrogen dioxide, as well as nonradical species such as hypochlorous acid, ozone, nitroxide, and peroxynitrite.Citation[15–18] In this way, vitamin C breaks free radical chain reactions, decreases consumption of endogen antioxidant enzymes, and prevents important biological macromolecules such as lipids, proteins, and DNA from oxidative damage. Superoxide anyon, given its great affinity for nitric oxide (NO), is able to rapidly remove it by its conversion into toxic peroxynitrite.Citation[30] Reduced bioavailable nitric oxide (NO), a potent renal vasodilatator, can contribute to renal hypoperfusion and tissue injury in the setting of myoglobinuric ARF.Citation[31] It has been shown that vitamin C improves NO bioavailability and NO-mediated resistance vessel dilatation.Citation[32] This molecular mechanism provides potential renoprotective capacity through the inhibition of renal vasoconstriction in myoglobinuric ARF. In addition to scavenging reactive oxygen species and reactive nitrogen species, vitamin C can regenerate other small molecule antioxidants, such as α-tocopherol and glutathione, from their respective radical species.Citation[14],Citation[33] It has been shown that vitamin C enhances carnitine biosynthesis.Citation[34] Carnitine is an antioxidant molecule that prevents lipid peroxidation.Citation[35] In addition, vitamin C has been shown to effectively inhibit the formation of ferryl myoglobin.Citation[12] This oxide form of myoglobin represents a toxic molecule that triggers oxidation reactions such as lipid peroxidation.Citation[11–13]
On the other hand, theoretically, the reduction of transition metal ions by ascorbate could also have deleterious effects via the production of hydroxyl radicals or lipid alkoxyl radicals by reaction of the reduced metal ions with hydrogen peroxide or lipid hydroperoxides.Citation[36] Although this Fenton chemistry occurs readily in vitro, its relevance in vivo has been a matter of some controversy, the main point of contention being the availability of catalytic metal ions in vivo. The levels of “free” metal ions are thought to be very low due to their sequestration by various metal binding proteins such as ferritin, transferrin, and ceruloplasmin.Citation[37] A trial in which non-smokers were supplemented with 6,000 mg/day of vitamin C showed a nonsignificant trend toward reduced plasma levels of MDA.Citation[38] However, during tissue injury, metal ions may be released from their stores and could subsequently interact with vitamin C.Citation[36] Contrary to its putative prooxidant role in the presence of redox-active transition metal ions, it has been suggested that vitamin C provides antioxidant protection of lipids in biological fluids, animals, and humans, both with and without iron cosupplementation.Citation[18] Berger et al.Citation[39] showed that when sufficient exogenous iron (as ferrous ammonium sulfate) is added to plasma to saturate transferrin in order to generate nonprotein-bound, bleomycin-detectable iron, endogenous and exogenous vitamin C inhibits rather than promotes lipid peroxidation. Two other studies carried out with plasma, lymph, and synovial fluid have shown that vitamin C can catalyze the formation of hydroxyl radicals, but only when iron-EDTA, is added,Citation[40],Citation[41] not ferrous ammonium sulfate.Citation[41] Retsky et al.Citation[42] reported that vitamin C effectively protects low density lipoproteins from metal ion-dependent oxidation in iron-overloaded human plasma in vitro. Chen et al.Citation[43] reported that plasma and liver F2-isoprostane levels were increased in vitamin C-deficient Guinea pigs supplemented with iron, and were reduced by vitamin C cosupplementation. Healthy volunteers who were supplemented with both iron and vitamin C (60 or 260 mg/day) for 12 weeks showed a modest reduction of ex vivo low-density lipoprotein oxidizability and beneficial effects in platelet function; no evidence of a pro-oxidant effect of vitamin C and iron cosupplementation was observed.Citation[44] The results from the present in vivo study, in agreement with previous in vitro and in vivo studies, demonstrate that vitamin C acts as an antioxidant toward lipids in glycerol-induced ARF.
Because vitamin C, a strong antioxidant, prevents the formation of ferrly myoglobin, it has a strong theoretical advantage over other antioxidants in treating myoglobinuric ARF, as the above phenomena contribute substantially to muscle and tubular damage.Citation[12] Therefore, in our study, vitamin C is preferred as an antioxidant. On the other hand, theoretically, vitamin C can have a pro-oxidant effect in the presence of in vitro redox-active transion ions. Citation[36] Consequently, in this study, the effect of vitamin C in the progress of myoglobinuric ARF is examined for the first time, and a low dose of vitamin C (20 mg/kg) is used. Antunes et al.Citation[22] observed that vitamin C showed a protective effect against cisplatin-induced oxidative damage on rat kidneys on a dose-dependent basis. In this study, vitamin C, applied at 100–200 mg/kg, prevented the increase of the serum creatinine and decrease of creatinine clearance better than the vitamin C applied at 50 mg/kg doses. Recently, Bulduk et al.Citation[45] reported that vitamin C, with 20 mg/kg dosages, partially corrected the plasma vitamin C levels and oxidative events in streptozotocin-induced diabetic rats. In the present study, vitamin C, in 20 mg/kg dosages, significantly reduced MDA levels when compared with saline-treated rats, whereas MDA levels in the vitamin C group were found to be significantly higher than in the control group. In the light of our study, it is concluded that higher doses of vitamin C than 20 mg/kg—for example, 50–200 mg/kg doses—may be beneficial for the better functional and morphological recovery in this model ARF.
In summary, a novel finding in the present study is that vitamin C helps in the prevention of renal damage induced by glycerol in rats. In this respect, vitamin C appears to be a promising candidate for the prevention of rhabdomyolysis-induced ARF. More animal experiments with higher doses of vitamin C should be conducted. Clinical trials need to be carried out to determine the usefulness and dose of vitamin C in rhabdomyolysis-induced myoglobinuric acute renal failure. Higher doses of vitamin C may be required to prevent rhabdomyolysis-induced renal damage in humans as compared to rats because humans cannot synthesize vitamin C.
DECLARATION OF INTEREST
The authors report no conflicts of interest. The authors alone are responsible for the content and writing of the paper.
ACKNOWLEDGMENTS
This work was supported by Trakya University, Scientific Researchs Committee (project TUBAB-501).
REFERENCES
- Sever MS, Erek E, Vanholder R, et al. Lessons learned from the catastrophic Marmara earthquake: Factors influencing the final outcome of renal victims. Clin Nephrol. 2004; 61: 413–421
- Zager RA. Rhabdomyolysis and myohemoglobinuric acute renal failure. Kidney Int. 1996; 49: 314–326
- Abassi ZA, Hoffman A, Better OS. Acute renal failure complicating muscle crush injury. Semin Nephrol. 1998; 18: 558–565
- Paller MS. Hemoglobin- and myoglobin-induced acute renal failure in rats: Role of iron in nephrotoxicity. Am J Physiol. 1988; 255: F539–F544
- Shah SV, Walker PD. Evidence suggesting a role for hydroxyl radical in glycerol-induced acute renal failure. Am J Physiol. 1988; 255: F438–F443
- Zager RA. Combined mannitol and deferoxamine therapy for myohemoglobinuric renal injury and oxidant tubular stress. Mechanistic and therapeutic implications. J Clin Invest. 1992; 90: 711–719
- Nath KA, Balla G, Vercellotti GM, et al. Induction of heme oxygenase is a rapid, protective response in rhabdomyolysis in the rat. J Clin Invest. 1992; 90: 267–270
- Emerit J, Beaumont C, Trivin F. Iron metabolism, free radicals, and oxidative injury. Biomed Pharmacother. 2001; 55: 333–339
- Paller MS, Hedlund BE. Role of iron in postischemic renal injury in the rat. Kidney Int. 1988; 34: 474–480
- Baliga R, Ueda N, Walker PD, Shah SV. Oxidant mechanisms in toxic acute renal failure. Am J Kidney Dis. 1997; 29: 465–477
- Kanner J, Harel S. Initiation of membranal lipid peroxidation by activated metmyoglobin and methemoglobin. Arch Biochem Biophys. 1985; 237: 314–321
- Galaris D, Korantzopoulos P. On the molecular mechanism of metmyoglobin-catalyzed reduction of hydrogen peroxide by ascorbate. Free Radic Biol Med. 1997; 22: 657–667
- Patel RP, Diczfalusy U, Dzeletovic S, Wilson MT, Darley-Usmar VM. Formation of oxysterols during oxidation of low density lipoprotein by peroxynitrite, myoglobin, and copper. J Lipid Res. 1996; 37: 2361–2371
- Freeman BA, Crapo JD. Biology of disease: Free radicals and tissue injury. Lab Invest. 1982; 47: 412–426
- Nishikimi M. Oxidation of ascorbic acid with superoxide anion generated by the xanthine-xanthine oxidase system. Biochem Biophys Res Commun. 1975; 63: 463–468
- Bielski BH. Chemistry of ascorbic acid radicals. Ascorbic acid: Chemistry, metabolism, and uses. Adv Chem Ser. 1982; 200: 81–100
- Bodannes RS, Chan PC. Ascorbic acid as a scavenger of singlet oxygen. FEBS Lett. 1979; 105: 195–196
- Frei B, Stocker R, England L, Ames BN. Ascorbate: The most effective antioxidant in human blood plasma. Adv Exp Med Biol. 1990; 264: 155–163
- Abraham P. Vitamin C may be beneficial in the prevention of paracetamol-induced renal damage. Clin Exp Nephrol. 2005; 9: 24–30
- Kavutcu M, Canbolat O, Oztürk S, et al. Reduced enzymatic antioxidant defense mechanism in kidney tissues from gentamicin-treated guinea pigs: Effects of vitamins E and C. Nephron. 1996; 72: 269–274
- Singh S, Rana SV. Amelioration of arsenic toxicity by L-Ascorbic acid in laboratory rat. J Environ Biol. 2007; 28(Suppl. 2)377–384
- Antunes LMG, Darin JD, Bianchi MD. Protective effects of vitamin c against cisplatin-induced nephrotoxicity and lipid peroxidation in adult rats: A dose-dependent study. Pharmacol Res. 2000; 41: 405–411
- Ocak S, Gorur S, Hakverdi S, Celik S, Erdogan S. Protective effects of caffeic acid phenethyl ester, vitamin C, vitamin E and N-acetylcysteine on vancomycin-induced nephrotoxicity in rats. Basic Clin Pharmacol Toxicol. 2007; 100: 328–333
- Ohkawa H, Ohishi N, Yagi K. Assay for lipid peroxides in animal tissues by thiobarbituric acid reaction. Anal Biochem. 1979; 95: 351–358
- Flohé L, Otting F. Superoxide dismutase assays. Methods Enzymol. 1984; 105: 93–104
- Aebi H. Catalase in vitro. Methods Enzymol. 1984; 105: 121–126
- Chander V, Singh D, Chopra K. Catechin, a natural antioxidant protects against rhabdomyolysis-induced myoglobinuric acute renal failure. Pharmacol Res. 2003; 48: 503–509
- Stefanovic V, Savic V, Vlahovic P, Cvetkovic T, Najman S, Mitic-Zlatkovic M. Reversal of experimental myoglobinuric acute renal failure with bioflavonoids from seeds of grape. Ren Fail. 2000; 22: 255–266
- Chander V, Chopra K. Molsidomine, a nitric oxide donor and L-arginine protects against rhabdomyolysis-induced myoglobinuric acute renal failure. Biochim Biophys Acta. 2005; 1723: 208–214
- Rubanyi GM, Vanhoutte PM. Superoxide anions and hyperoxia inactivate endothelium-derived relaxing factor. Am J Physiol. 1986; 250: H822–H827
- Valdivielso JM, López-Novoa JM, Eleno N, Pérez Barriocanal F. Role of glomerular nitric oxide in glycerol-induced acute renal failure. Can J Physiol Pharmacol. 2000; 8: 476–482
- Cross JM, Donald AE, Nuttall SL, Deanfield JE, Woolfson RG, Macallister RJ. Vitamin C improves resistance but not conduit artery endothelial function in patients with chronic renal failure. Kidney Int. 2003; 63: 1433–1442
- Meister A. Glutathione-ascorbic acid antioxidant system in animals. J Biol Chem. 1994; 269: 9397–9400
- Rebouche CJ. Ascorbic acid and carnitine biosynthesis. Am J Clin Nutr. 1991; 54(Suppl. 6)1147S–1152S
- Loots DT, Mienie LJ, Bergh JJ, Van der Schyf CJ. Acetyl-L-carnitine prevents total body hydroxyl free radical and uric acid production induced by 1-methyl-4-phenyl-1,2,3,6-tetrahydropyridine (MPTP) in the rat. Life Sci. 2004; 75: 1243–1253
- Halliwell B. Vitamin C: antioxidant or pro-oxidant in vivo?. Free Rad Res 1996; 25: 439–454
- Halliwell B, Gutteridge JMC. Oxygen free radicals and iron in relation to biology and medicine: some problems and concepts. Arch Biochem Biophys. 1996; 246: 501–514
- Anderson D, Phillips BJ, Yu T, Edwards AJ, Ayesh R, Butterworth KR. The effects of vitamin C supplementation on biomarkers of oxygen radical generated damage in human volunteers with ‘low’ or ‘high’ cholesterol levels. Environ Mol Mutagen. 1997; 30: 161–174
- Berger TM, Polidori MC, Dabbagh A, et al. Antioxidant activity of vitamin C in iron-overloaded human plasma. J Biol Chem. 1997; 272: 15656–15660
- Winterbourn CC. Hydroxyl radical production in body fluids: Roles of metal ions, ascorbate and superoxide. Biochem J. 1981; 198: 125–131
- Minetti M, Forte T, Soriani M, Quarisima V, Menditto A, Ferrari M. Iron-induced ascorbate oxidation in plasma as monitored by ascorbate free radical formation: No spin-trapping evidence for the hydroxyl radical in iron-overload plasma. Biochem J. 1992; 282: 459–465
- Retsky KL, Freeman MW, Frei B. Ascorbic acid oxidation product(s) protect human low density lipoprotein against atherogenic modification. Anti- rather than prooxidant activity of vitamin C in the presence of transition metal ions. J Biol Chem. 1993; 268: 1304–1309
- Chen K, Suh J, Carr AC, Morrow JD, Zeind J, Frei B. Vitamin C suppresses oxidative lipid damage in vivo, even in the presence of iron overload. Am J Physiol Endocrinol Metab. 2000; 279: E1406–1412
- Yang M, Collis CS, Kelly M, Diplock AT, Rice-Evans C. Do iron and vitamin C co-supplementation influence platelet function or LDL oxidizability in healthy volunteers?. Eur J Clin Nutr. 1999; 53: 367–374
- Bulduk E, Gönül B, Ozer C. Effects of vitamin C on muscle glycogen and oxidative events in experimental diabetes. Mol Cell Biochem. 2006; 292: 131–137