Abstract
Contrast induced nephropathy (CIN) is a major cause of morbidity, and increased costs as well as an increased risk of death. This study was evaluated effects of exogenous sphingosylphosphorylcholine (SPC) administration on CIN in rats. Eight animals were included in each of the following eight groups: control, control phosphate-buffered solution (PBS), control SPC 2, control SPC 10, CIN, CIN PBS, CIN SPC 2 and CIN SPC 10. The induced nephropathy was created by injected with 4 g iodine/kg body weight. SPC was administered 3 d at a daily two different doses of 2 μm/mL and 10 μm/mL intraperitoneally. The severity of renal injury score was determined by the histological and immunohistochemical changes in the kidney. Malondialdehyde (MDA), nitric oxide (NO) and superoxide dismutase (SOD) were determined to evaluate the oxidative status in the renal tissue. Treatment with 2 and 10 μM SPC inhibited the increase in renal MDA, NO levels significantly and also attenuated the depletion of SOD in the renal injuryCIN. These data were supported by histopathological findings. The inducible nitric oxide synthase positive cells and apoptotic cells in the renal tissue were observed to be reduced with the 2 and 10 μM SPC treatment. These findings suggested that 2 and 10 μM doses can attenuate renal damage in contrast nephropathy by prevention of oxidative stress and apoptosis. The low and high dose SPC may be a promising new therapeutic agent for CIN.
Introduction
Contrast-induced nephropathy (CIN), the incidence varies from 3.3 to 76%, is now one of the three leading causes of acute kidney injury in the world.Citation1 CIN is a major cause of morbidity, end stage renal disease, prolonged hospitalization, and increased costs as well as an increased risk of death.Citation1,Citation2 CIN is defined as an absolute increase in serum creatinine (sCr) concentration ≥0.5 mg/dL or as a relative increase ≥25% above baseline within 3 d of contrast media exposure.Citation3
The pathophysiology of CIN is a complex interplay between vascular and tubular effects. Tubular toxicity, generation of reactive oxygen species (ROS) cause lipid peroxidation and cytotoxic damage,Citation4 and medullary blood flow reduction are suggested as being the risk factors of CIN.Citation5–7 And also free radicals react with nitric oxide (NO) to produce peroxynitrite, an oxidative and very reactive nitrosative species capable of reducing the bioavailability of NO, thereby increasing tissue damage.Citation4 This conditions may contribute to the increased apoptosis in the pathogenesis of renal injury at the time of CIN.Citation8,Citation9
The sphingolipid metabolites, sphingosine, sphingosine-1-phosphate, and sphingosylphosphorylcholine (SPC) are considered to be the most powerful stimulators for cell proliferation and DNA synthesis.Citation10,Citation11 Sphingolipids are novel second messengers mediating a variety of cellular responses. One of the sphingolipids, SPC, induces cell proliferation and apoptosis and is also known to inhibit the growth of various cell types, particularly tumor cells.Citation12,Citation13 It is known that sphingolipids have a wide spectrum of biological activities, such as affecting NO production,Citation14 regulating ROS,Citation15 and providing potent anti-inflammatory effects.Citation16,Citation17 The different biological effects of SPC are dependent on specific SPC concentrations.Citation18 The previously studies demonstrated that the dose-response of SPC in promoting wound healing indicated that not only was SPC efficacious but also that optimal efficacy was achieved with 2–3 μM but above 10 μM SPC concentration was cytotoxic.Citation10,Citation17 Therefore, we used 2 μM for optimal efficacy and 10 μM for above this threshold in this study.
The aims of this study were to investigate the role of oxidative injury and the effect of exogenous SPC administration on acute renal injury induced by contrast media and to evaluate the role of NO in oxidative injury. Renal tissue oxidative stress was evaluated by changes in the amount of lipid peroxide malondialdehyde (MDA) and NO and the activities of antioxidant enzymes (superoxide dismutase [SOD]). The neutrophil activation and other changes due to renal injury were evaluated by histological and immunohistochemical changes in the kidney.
Materials and methods
The Ethical Committee of Trakya University approved all animal procedures and the experimental protocol (TUHDYEK-2013/05).
Animals
Sixty-eight adult Wistar Albino rats, weighing 200–250 g, were used in this study. Rats were provided by the Experimental Research Center of the Medical Faculty of Trakya University. The rats were kept in a windowless animal quarter with automatically controlled temperature (22 ± 2 °C) and illumination (lights on at 7 am and off at 9 pm: 14 h light/10 h dark cycle). Humidity ranged from 50 to 55%. Rats were fed with standard rat chow and tap water ad libitum.
Eight animals were included in each of the following eight groups (n = 8): (1) control group; (2) control phosphate-buffered solution (PBS) group; 1 mL PBS was given intraperitoneally (i.p.) once a day 3 d; (3) control SPC 2 group: 2 μM SPC was given i.p. once a day 3 d; (4) control SPC 10 group: 10 μM SPC was given i.p. once a day 3 d; (5) CIN group; (6) CIN PBS group: 1 mL PBS was given i.p. once a day just after CIN and 2 d after nephropathy; (7) CIN SPC 2 group: 2 μM SPC was given i.p. once a day just after CIN and 2 d after nephropathy; (8) CIN SPC 10 group: 10 μM SPC was given i.p. once a day just after CIN and 2 d after nephropathy.
Experimental protocols
The rats were anesthetized with ketamine (90 mg/kg) and xylazine (10 mg/kg) intraperitoneally. The tail veins of the Wistar Albino rats were injected with iodixanol a dosage of 4 g iodine/kg body weight (BW) and with saline at equal volume as control.Citation19
The SPC was obtained from the Sigma Chemical Company (St. Louis, MO). It was initially dissolved in 100% ethanol and the resulting SPC/ethanol solution was subsequently dissolved in a PBS containing 2 mg/mL and 10 mg/mL bovine serum albumin to acquire a stock solution (2 and 10 mM), as described in Aksu et al.Citation17 The SPC stock solution was stored at −80 °C. SPC was administered to SPC 2 groups and SPC 10 groups (2 and 10 μm/mL, respectively) immediately after nephropathy for 3 d at a daily dose of i.p. PBS was administered to the PBS group’s rats immediately after CIN for 3 d at a daily dose of 1 mL i.p. The control group received equal amounts of saline i.p. for 3 d.
After 3 d of treatment, the animals were decapitated. After opening the abdominal cavity, the renal tissue samples were immediately placed in 10% neutral buffered formalin solution for histological evaluation, or were stored at −80 °C for subsequent biochemical assay.
Biochemical measures
The renal tissues were homogenized in a tenfold volume of physiological saline solution using a homogenizer (Ultra-Turrax T25, IKA; Werke 24,000 rpm; Germany). The homogenate was centrifuged at 10,000×g for 1 h to remove debris. Clear upper supernatant was taken, and tissue analyses were carried out. All procedures were performed at +4 °C throughout the experiments.
SOD activities were studied on hemolysates using commercial kits (Randox Laboratories, Crumlin, UK)Citation20 and were respectively expressed as U/mg protein and U/kg protein. NO has a half-life of only a few seconds because it is readily oxidized to nitrite (NO2−) and subsequently to nitrate (NO3−), which serve as index parameters of NO production. The method for plasma nitrite and nitrate levels was based on the Griess test.Citation21 Results are expressed as μmol/g wet tissue. Renal tissues MDA levels were determined by a method based on the reaction with thiobarbituric acid (TBA) at 90–100 °C.Citation22 The results are expressed as nmol/g wet tissue. After lysing samples and removing the precipitate, disodium hydrogen phosphate and DTNB solution were added, and the color formed was read at 412 nm.
Histopathological assessment of kidneys
The kidneys were removed, cut into sections, and fixed in 10% formaldehyde. After embedding in paraffin, 5 mm sections were stained with hematoxylin and eosin before assessment by light microscopy (original magnification 400×, Olympus CX31; Olympus, Tokyo, Japan). Tubular damage was scored as follows: Each section was examined in 10 fields, and the average percentage of the impaired renal tubules was then calculated.
These steps were then followed by semi-quantitative analysis of the kidney sections by a pathologist functioning in a blind manner. Tubular necrosis and proteinaceous casts were graded according to a previous methodology. The following scoring system was used for the histopathological evaluation of tissues under light microscopy; where no damage was assigned a 0 index; <25% damage was assigned 1; 25–50% damage was assigned 2; 50–75% damage was assigned 3 and >75% damage was assigned a 4 index.
Inducible nitric oxide synthase (iNOS) immunohistochemistry
The kidney tissues were fixed in 10% buffered formalin solution, and, following routine laboratory methods, embedded in paraffin. Five-micrometer paraffin tissue sections were mounted on poly-L-lysine slides. The slides were air-dried and the tissue deparaffinized. The avidin biotin complex method was used for immunohistochemical staining, as previously reported.Citation23 The sections were incubated with rabbit polyclonal anti-iNOS (Abcam, dilution 1:100) (Abcam, Cambridge, MA). Diaminobenzidine (DAB) was used as a chromogen, and the sections were counterstained with hematoxylin. In each section, the numbers of cells in 10 different areas selected at random were counted and the arithmetic mean calculated. For the ×20 magnification, the 100 μmCitation2 area was determined by means of a micrometer slide. Finally, all counts were converted to number of iNOS positive cells per unit area (mm2).
TUNEL assay
A terminal deoxynucleotidyltransferase dUTP nick-end labeling (TUNEL) assay was used to assess renal apoptosis with an apoptosis detection kit (Millipore ApopTag, Billerica, MA) according to the manufacturer’s instructions. For each slide, 10 fields were randomly chosen, with TUNEL-positive cells staining dark brown staining within the nucleus of apoptotic cells. TUNEL-positive cells were quantified under high-power magnification by a researcher in a blinded manner and the apoptotic index was calculated (the number of TUNEL-positive cells/total number of cells counted ×100).
Statistical analyses
Data were analyzed using a commercially available statistics software package (SPSS® for Windows). Distributions of the groups were analyzed with one sample Kolmogorov–Smirnov test. All groups showed normal distribution, so that parametric statistical methods were used to analyze the data. One-way ANOVA test was performed and post hoc multiple comparisons were done with LSD. Results were presented as means ± standard deviation (SD), and p-values <0.05 were regarded as statistically significant.
Results
Biochemical findings
A total of 3 d after the nephropathy, sCr and blood urea nitrogen (BUN) levels were found to be elevated significantly in the CIN and CIN PBS groups and CIN treated with SPC compared to the control groups (for sCr p < 0.001, for BUN p < 0.001, respectively). The CIN rats treated with SPC manifested marked reduction in BUN levels than CIN and CIN PBS groups (p < 0.01). The values of the sCr and BUN levels are shown in .
Table 1. Biochemical parameters of renal function evaluation.
In the CIN and CIN PBS groups, tissue SOD activity was significantly lower (p < 0.01), and MDA and NO levels were higher (p < 0.01) than in the control group. The 2 and 10 μM SPC treatment led to significant increases in mean tissue SOD activity (p < 0.05). The 2 and 10 μM SPC treatment significantly lowered MDA and NO levels (p < 0.05). Also, the 10 μM SPC treatment was very decreased NO levels than in the 2 μM SPC treatment (p < 0.01) ().
Table 2. Antioxidant enzyme and lipid peroxide levels.
Histopathological findings
To examine effects of CIN injury in rats, we observed the renal morphological changes using microscopy. After 3 d, CIN markedly increased widespread loss of brush border, a denudation of tubular cells, tubule dilatation, and intratubular obstruction by granular casts were detected in rat kidney compared with the control. As shown in . SPC administrated at doses of 2 and 10 μM after CIN significantly inhibited renal histological changes, as shown in ().
Figure 1. Renal tissue H & E staining images: (a) Control group, (b) PBS group, (c) SPC2 group, (d) SPC10 group, (e) Contrast group, (f) Contrast + PBS, (g) Contrast + SPC2 group, and (h) Contrast + SPC10 group. a, b, c, and d figures, the histology of renal tissues was normal in appearance. However, contrast group (e) have been necrotic cells, cytoplasmic vacuole, and tubule injuries were reasonably higher in the renal tissues. Contrast + SPC2 and SPC10 groups’ tubule injuries and necrotic cells were highly decreased in the renal tissues compared with contrast and contrast + PBS groups (Hematoxylin–Eosin stain, magnifications: ×200).

Table 3. Renal histologic evaluation in the various treatment groups.
Immunohistochemical and TUNEL findings
As shown in and , the number of iNOS-positive cells was significantly increased in the CIN and CIN PBS groups after nephropathy and decreased remarkably in the group treated with 2 and 10 μM SPC (p < 0.05). The TUNEL staining results showed that the TUNEL-positive cell level increased remarkably after nephropathy (CIN and CIN PBS groups compared to the control groups: p < 0.05) and decreased weakly in response to 2 and 10 μM SPC. As shown in and .
Figure 2. Immunohistochemical analyses of iNOS. (a) Control group, (b) PBS group, (c) SPC2 group, (d) SPC10 group, (e) Contrast group, (f) Contrast + PBS, (g) Contrast + SPC2 group, and (h) Contrast + SPC10 group. In the contrast group, the number of iNOS positive cells was a significantly increased in the renal tubes. In the Contrast + SPC2 and contrast + SPC10 groups, iNOS positive cells numbers were markedly reduced (iNOS immunohistochemical staining, magnifications: ×400).
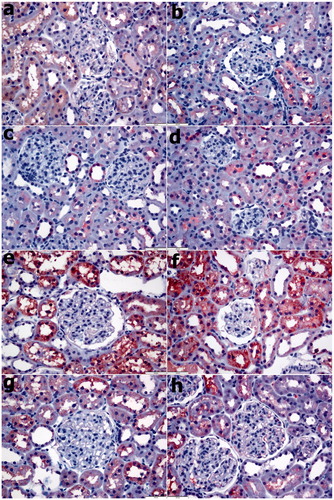
Figure 3. TUNEL staining. (a) Control group, (b) PBS group, (c) SPC2 group, (d) SPC10 group, (e) Contrast group, (f) Contrast + PBS, (g) Contrast + SPC2 group, and (h) Contrast + SPC10 group. Control, PBS, SPC2, and SPC10 groups a small number of TUNEL-positive cells were observed in the renal tissue. In the Contrast group that TUNEL-positive cells were significantly higher in the renal tissues. Contrast + SPC2 and Contrast + SPC10 groups, TUNEL-positive cells were significantly decreased in the renal compared with contrast group. (Arrows: TUNEL positive cells) (TUNEL staining, magnifications: ×400).
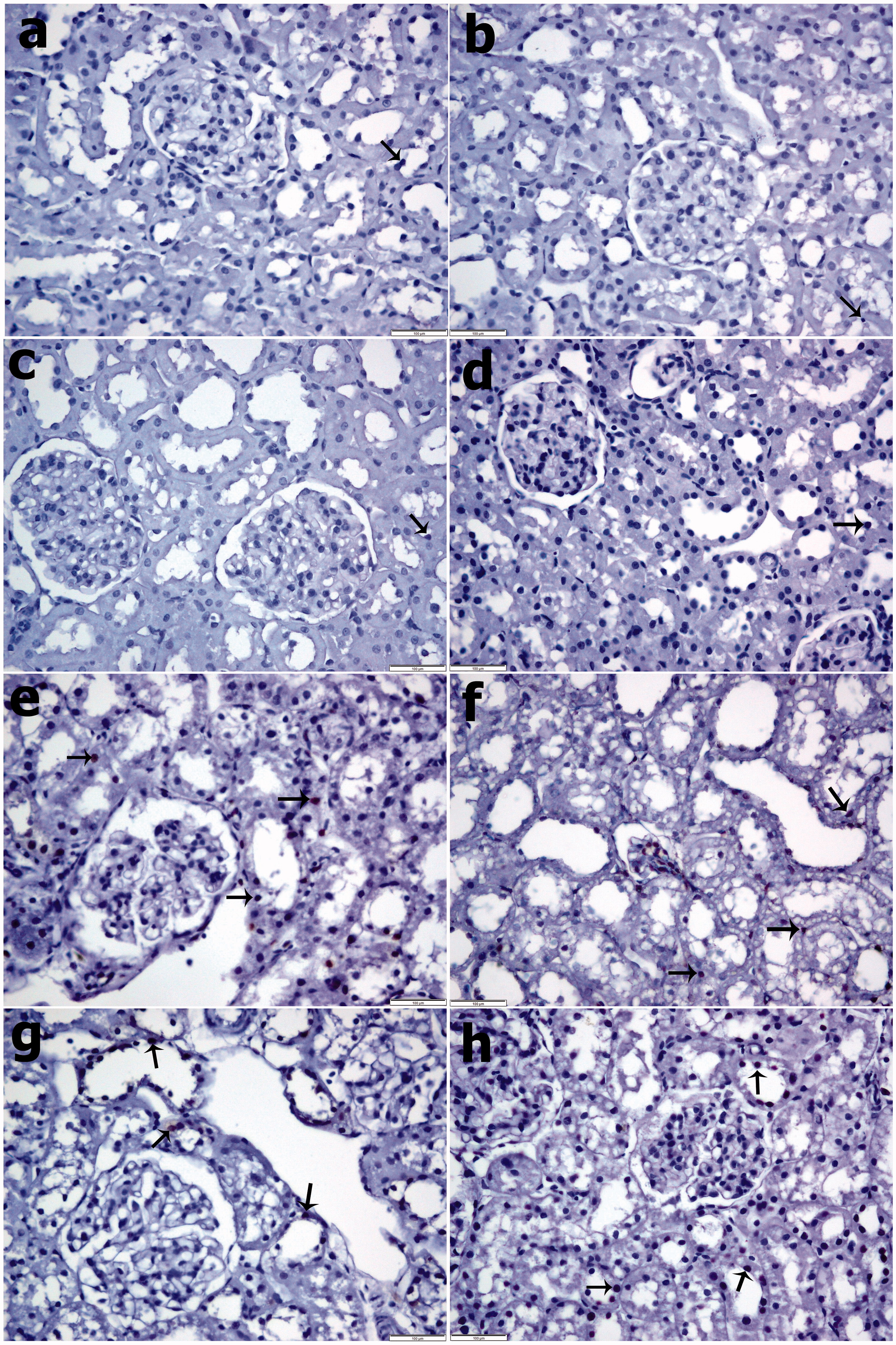
Table 4. Distribution of iNOS positive cells density in the different treatment groups (iNOS positive cells/mm2).
Table 5. The numbers of TUNEL positive cells in the different treatment groups.
Discussion
The true incidence of CIN in the elderly is not known, although in the United States and Europe, radiocontrast nephropathy is reported to be third most usual cause of hospital-acquired acute renal failure accounting for 10% of all causes of hospital-acquired renal failure.Citation24 The precise mechanisms of CIN have not been explained in details. As far as can be explained, in vitro studies and studies in animals suggest several factors have thought to be related to pathogenesis of CIN, for example hemodynamic changes of renal blood flow, which causes hypoxia in the renal medulla, direct toxic effect of the contrast media on renal cells, partly mediated by ROS.Citation25–27 Moreover, low blood flow in the medulla, which has a high demand for oxygen, might result from increased perivascular hydrostatic pressure, high viscosity, or changes in vasoactive substances such as endothelin, NO, and adenosine.Citation28,Citation29 Furthermore, contrast agents have direct toxic effects on kidney tubular cells, inducing vacuolization, change in mitochondrial function, and even apoptosis.Citation28,Citation30
In this study, CIN significantly increased denudation of tubular cells, tubule dilatation and intratubular obstruction by granular casts, but subsided following administration of 2 and 10 μM SPC. CIN increased MDA and total NO levels, and this significantly reduced MDA and NO levels with both doses SPC. Administration of 2 and 10 μM SPC for CIN resulted in increased SOD in the renal tissue. We have previously shown used SPC can reduce the hepatic damage in extrahepatic cholestasis and lung contusion by prevention of the oxidative stress and of the inflammatory process in liver bile duct ligation and pulmonary contusion.Citation17,Citation23 The contrary results suggest that the SPC-induced generation of ROS plays a crucial role in the cell death of endothelial cells through extracellular signal-regulated kinase-dependent pathways.Citation31 In this study, the anti-oxidant property of SPC re-expressed using biochemical methods.
A previous study demonstrated for the first time that SPC treatment stimulates the proliferation with an EC50 of 5 μM, whereas concentrations of SPC greater than 10 μM induced an apoptosis-like cell death of mesenchymal stem cells derived from adipose tissue.Citation32 However, at a concentration of greater than 30 μM, SPC attenuated proliferation due to cytotoxicity.Citation33 In previous study, SPC lost its antioxidant and anti-apoptotic properties in the group treated with 10 μM SPC. The effect of high-dose SPC may be dependent on the triphasic effects of the dose.Citation23 But in this study, we did not see the triphasic effects in 2 and 10 μM SPC dosages. These two separate doses gave the similar effects, the possible reasons for this similarity may be the insufficiency of renal tissue concentration for showing triphasic effects or the difference in answers between lung and kidneys to high concentrations of SPC. This is the black point and limitation of our study which may be lightened with studies that shows organ–tissue dosage interactions in near future.
Even though the pathogenesis is not fully clear, various vasoactive substances, such as adenosine, endothelin, and NO, oxidative injuries and apoptosis are factors believed to be involved.Citation34,Citation35 NO is synthesis from L-arginine. Although NO is a potent vasodilator molecule, excess production can lead to production-dependent apoptotic or necrotic injury in tubular epithelial cells.Citation36 It is now clear that SPC may increase NO production in endothelial cells in situ, which subsequently reduces the contraction of smooth muscle cells in the intact vascular strips.Citation37 SPC induced [Ca2.]i elevation and NO production in endothelial cells in situ.Citation37 In the smooth muscle of pig coronary arteries, SPC induced vessel contraction via Ca2 + sensitization and reduced NO production.Citation38 At low physiological doses (10–100 nM), transient sphingosine 1-phosphate induced vasodilation was observed in preconstructed mesenteric arteries.Citation39 In contrast, the majority of studies showing vasoconstriction have used at least 10-fold higher concentrations.Citation40 In vivo studies have demonstrated that SPC has a reducing effect on NO.Citation17 The activation of NO may be through direct action of SPC on the endothelial NO synthase (eNOS)/NO pathway, which results in NO release and vasodilation.Citation41 Recently, a Murch et al. Citation14 study demonstrated that SPC exerts potent anti-inflammatory effects in vivo in endotoxemic rats. SPC exerts most of its action by binding to and activating cell surface receptors, thereby triggering various cell responses such as vasoconstriction or dilation, angiogenesis, stress fiber formation, and cytoskeletal rearrangements.Citation42,Citation43 Another study showed that SPC activates the transforming growth factor β/Smad signaling cascade and, thereby, is able to reduce the promoter activity and expression of pro-inflammatory enzymes like secretory phospholipase A2 and iNOS in renal mesangial cells.Citation44 SPC is probably due to its activity in reducing iNOS to keep NO levels within physiological limits. Positive effects of SPC kept NO levels in physiologic limits so that vascular permeability is kept within normal limits. This results in decreased renal permeability, edema and perivascular hydrostatic pressure, and recovery of compliance in the CIN. All these effects of SPC eventually reduced apoptosis in this experimental study of CIN.
NO can give rise to lipid peroxidation causing the formation of reactive nitrogen products when produced in excessive quantities. It can directly lead to DNA damage, mitochondrial membrane damage, or apoptosis through the p53 tumor suppressor gene stabilization pathway and damage in intracellular proteins and enzymes.Citation45,Citation46 A rise in NO can lead to apoptotic or necrotic injury in tubular epithelial cells.Citation35,Citation47 While research suggests that a decrease in NO production is involved in the pathogenesis of CIN, clinical studies have not supported this finding.Citation34,Citation47 Sphingolipids are novel second messengers mediating a variety of cellular responses, including cell proliferation and apoptosis.Citation23 Previous studies have shown that high concentrations of SPC induce apoptosis via the mitochondrial pathway.Citation31,Citation32 The similar cell culture study using low-dose SPC concentration demonstrated that SPC was effective as an anti-apoptotic agent.Citation48 Keeping the NO levels in normal range with SPC treatment may prevents the apoptosis that is caused by direct DNA fragmentation induced by increased NO levels in CIN. In addition, decreased levels of MDA in SPC treatment group show that it also inhibits lipid peroxidation. The damage in cellular and mitochondrial membranes caused by lipid peroxidation may be prevented with SPC treatment which provides an effective decrease in levels of NO. Therefore, cellular damage in CIN may be prevented by the antiapoptotic effects of SPC. The results of this study suggest that SPC might be beneficial in preventing CIN.
In conclusion, these findings suggest that SPC can reduce the renal damage in CIN by preventing the oxidative stress, and apoptosis. All of these findings suggest that SPC may be a promising new therapeutic agent for CIN.
There are limitations in this study: the serum concentration of the SPC was not measured and exact SPC levels in the blood are unknown, concentration of the SPC of renal tissue levels were absent, so the spectrum changes in the level of NO are also unknown. Recently study; renal tubular cells undergoing apoptosis were characterized by condensation of the nuclear chromatin and wrinkling of nuclear membranes. Representative changes of ultrastructure were a judged by transmission electron microscope.Citation49 But we are not used this method. In future studies, these limitations are questions that need to be answered.
Disclosure statement
The authors report no conflicts of interest. The authors alone are responsible for the content and writing of the paper.
References
- James MT, Samuel SM, Manning MA, et al. Contrast-induced acute kidney injury and risk of adverse clinical outcomes after coronary angiography: A systematic review and meta-analysis. Circ Cardiovasc Interv. 2013;6:37–43.
- Okoye O, Ojogwu L, Unuigbe E, Oviasu E. Frequency and risk factors of contrast-induced nephropathy after contrast procedures in a Nigerian tertiary centre. West Afr J Med. 2013;32:19–25.
- Thomsen HS, Morcos SK. Contrast media and the kidney: European society of urogenital radiology (ESUR) guidelines. Br J Radiol. 2003;76:513–518.
- Detrenis S, Meschi M, Musini S, Savazzi G. Lights and shadows on the pathogenesis of contrast-induced nephropathy: State of the art. Nephrol Dial Transplant. 2005;20:1542–1550.
- Wang F, Li J, Huang B, et al. Clinical survey on contrast-induced nephropathy after coronary angiography. Ren Fail. 2013;35:1255–1259.
- Sanadgol H, Abdani S, Tabatabaiee P, Mohammadi M. Protective effect of high dose short term statin therapy with normal saline in prevention of contrast-induced nephropathy among iodixanol-receiving patients. J Ren Inj Prev. 2012;1:43–45.
- Kwok CS, Pang CL, Yeong JK, Loke YK. Measures used to treat contrast-induced nephropathy: Overview of reviews. Br J Radiol. 2013;86:20120272.
- Haeussler U, Riedel M, Keller F. Free reactive oxygen species and nephrotoxicity of contrast agents. Kidney Blood Press Res. 2004;27:167–171.
- Bakris GL, Lass N, Gaber AO. Radiocontrast mediuminduced declines in renal function: A role for oxygen free radicals. Am J Physiol. 1990;258:115–120.
- Yagmurlu A, Aksu B, Bingol-Kologlu M, et al. A novel approach for preventing esophageal stricture formation: Sphingosylphosphorylcholine-enhanced tissue remodeling. Pediatr Surg Int. 2004;20:778–782.
- Higuchi K, Kawashima M, Ichikawa Y, Imokawa G. Sphingosylphosphorylcholine is a melanogenic stimulator for human melanocytes. Pigment Cell Res. 2003;16:670–678.
- Mulders AC, Nau S, Li Y, Michel MC. Effects of sphingosine-1-phosphate and sphingosylphosphorylcholine on intracellular Ca2+ and cell death in prostate cancer cell lines. Auton Autacoid Pharmacol. 2007;27:173–179.
- Xu Y. Sphingosylphosphorylcholine and lysophosphatidylcholine: G protein-coupled receptors and receptor-mediated signal transduction. Biochim Biophys Acta. 2002;1582:81–88.
- Murch O, Abdelrahman M, Collino M, et al. Sphingosylphosphorylcholine reduces the organ injury/dysfunction and inflammation caused by endotoxemia in the rat. Crit Care Med. 2008;36:550–559.
- Jeon ES, Kang YJ, Song HY, et al. Sphingosylphos-phorylcholine generates reactive oxygen species through calcium-, protein kinase Cdelta- and phospholipase D-dependent pathways. Cell Signal. 2005;17: 777–787.
- Huwiler A, Pfeilschifter J. Lipids as targets for novel anti-inflammatory therapies. Pharmacol Ther. 2009;124:96–112.
- Aksu B, Umit H, Kanter M, et al. Effects of sphingosylphosphorylcholine against cholestatic oxidative stress and liver damage in the common bile duct ligated rats. J Pediatr Surg. 2009;44:702–710.
- Zhang H, Buckley NE, Gibson K, Spiegel S. Sphingosine stimulates cellular proliferation via a protein kinase C-independent pathway. J Biol Chem. 1990;265:76–81.
- Lenhard DC, Pietsch H, Sieber MA, et al. The osmolality of nonionic, iodinated contrast agents as an important factor for renal safety. Invest Radiol. 2012;47:503–510.
- Sun Y, Oberley LW, Li YA. A simple method for clinical assay of superoxide dismutase. Clin Chem. 1988;34:497–500.
- Green LC, Wagner DA, Glogowski J, Skipper PL, Wishnok JS, Tannenbaum SR. Analysis of nitrate, nitrite, and [15N]nitrate in biological fluids. Anal Biochem. 1982;126:131–138.
- Buege JA, Aust SD. Microsomal lipid peroxidation. Methods Enzymol. 1978;52:302–310.
- Aksu B, Ayvaz S, Aksu F, et al. Effects of sphingosylphosphorylcholine against oxidative stress and acute lung injury induced by pulmonary contusion in rats. J Pediatr Surg. 2015;50:591–597.
- Briguori C, Tavano D, Colombo A. Contrast agent-associated nephrotoxicity. Prog Cardiovasc Dis. 2003;45:493–503.
- Murphy SW, Barrett BJ, Parfrey PS. Contrast nephropathy. J Am Soc Nephrol. 2000;11:177–182.
- Heyman SN, Reichman J, Brezis M. Pathophysiology of radiocontrast nephropathy: A role for medullary hypoxia. Invest Radiol. 1999;34:685–691.
- Morcos SK, Thomsen HS, Exley CM, Members of Contrast Media Safety Committee of European Society of Urogenital Radiology (ESUR). Contrast media: Interactions with other drugs and clinical tests. Eur Radiol. 2005;15:1463–1468.
- Persson PB, Hansell P, Liss P. Pathophysiology of contrast medium-induced nephropathy. Kidney Intern. 2005;68:14–22.
- Kurtoglu T, Durmaz S, Akgullu C, et al. Ozone preconditioning attenuates contrast-induced nephropathy in rats. J Surg Res. 2015;195:604–611.
- Aurelio A, Durante A. Contrast-induced nephropathy in percutaneous coronary interventions: Pathogenesis, risk factors, outcome, prevention and treatment. Cardiology. 2014;128:62–72.
- Jeon ES, Lee MJ, Sung SM, Kim JH. Sphingosylphosphorylcholine induces apoptosis of endothelial cells through reactive oxygen species-mediated activation of ERK. J Cell Biochem. 2007;100:1536–1547.
- Jeon ES, Kang YJ, Song HY, et al. Role of MEK-ERK pathway in sphingosylphosphorylcholine-induced cell death in human adipose tissue-derived mesenchymal stem cells. Biochim Biophys Acta. 2005;1734:25–33.
- Desai NN, Carlson RO, Mattie ME, et al. Signaling pathways for sphingosylphosphorylcholine-mediated mitogenesis in Swiss 3T3 fibroblasts. J Cell Biol. 1993;121:1385–1395.
- Wong PC, Li Z, Guo J, Zhang A. Pathophysiology of contrast-induced nephropathy. Int J Cardiol. 2012;158:186–192.
- Quintavalle C, Brenca M, De Micco F, et al. In vivo and in vitro assessment of pathways involved in contrast media-induced renal cells apoptosis. Cell Death Dis. 2011;2:155.
- Romano G, Briguori C, Quintavalle C, et al. Contrast agents and renal cell apoptosis. Eur Heart J. 2008;29:2569–2576.
- Mogami K, Mizukami Y, Todoroki-Ikeda N, et al. Sphingosylphosphorylcholine induces cytosolic Ca2+ elevation in endothelial cells in situ and causes endothelium-dependent relaxation through nitric oxide production in bovine coronary artery. FEBS Lett. 1999;457:375–380.
- Todoroki-Ikeda N, Mizukami Y, Mogami K, et al. Sphingosylphosphorylcholine induces Ca(21)-sensitization of vascular smooth muscle contraction: Possible involvement of rho-kinase. FEBS Lett. 2000;482:85–90.
- Dantas AP, Igarashi J, Michel T. Sphingosine 1-phosphate and control of vascular tone. Am J Physiol Heart Circ Physiol. 2003;28:2045–2052.
- Watterson KR, Ratz PH, Spiegel S. The role of sphingosine-1-phosphate in smooth muscle contraction. Cell Signal. 2005;17:289–298.
- Nofer JR, van der Giet M, Tölle M, et al. HDL induces NO-dependent vasorelaxation via the lysophospholipid receptor S1P3. J Clin Invest. 2004;113:569–581.
- Meyer zu Heringdorf D, Himmel HM, Jakobs KH. Sphingosylphosphorylcholine-biological functions and mechanisms of action. Biochim Biophys Acta. 2002;1582:178–189.
- Nixon GF, Mathieson FA, Hunter I. The multi-functional role of sphingosylphosphorylcholine. Prog Lipid Res. 2008;47:62–75.
- Xin C, Ren S, Eberhardt W, Pfeilschifter J, Huwiler A. Sphingosylphosphorylcholine acts in an anti-inflammatory manner in renal mesangial cells by reducing interleukin-1beta-induced prostaglandin E2 formation. J Lipid Res. 2007;48:1985–1996.
- Thomas DD, Espey MG, Ridnour LA, et al. Hypoxic inducible factor 1alpha, extracellular signal-regulated kinase, and p53 are regulated by distinct threshold concentrations of nitric oxide. Proc Natl Acad Sci U S A. 2004;101:8894–8899.
- Hannibal L. Nitric oxide homeostasis in neurodegenerative diseases. Curr Alzheimer Res. 2016;13:135–149.
- Ulusoy S, Ozkan G, Mungan S, et al. GSPE is superior to NAC in the prevention of contrast-induced nephropathy: Might this superiority be related to caspase 1 and calpain 1? Life Sci. 2014;103:101–110.
- Nofer JR, Levkau B, Wolinska I, et al. Suppression of endothelial cell apoptosis by high density lipoproteins (HDL) and HDL-associated lysosphingolipids. J Biol Chem. 2001;276:34480–34485.
- Gong X, Wang Q, Tang X, et al. Tetramethylpyrazine prevents contrast-induced nephropathy by inhibiting p38 MAPK and FoxO1 signaling pathways. Am J Nephrol. 2013;37:199–207.