Abstract
In various renal injuries, tissue damage occurs and platelet activation is observed. Recent studies suggest that some factors, such as serotonin, are released into microenvironment upon platelet activation following renal injury. In the present study, we aimed to investigate whether platelets and platelet-released serotonin are involved in the functional regulation of renal proximal tubular epithelial cells (PTECs). PTECs were obtained by primary cell culture and treated with platelet lysate (PL) (2 × 106/mL, 4 × 106/mL, 8 × 106/mL) or serotonin (1 μM or 5 μM) for 12 or 24 h. Phenotypic transdifferentiation of epithelial cells into myofibroblasts were demonstrated under light microscope and confirmed by the determination of α-smooth muscle actin gene expression. Serotonin and PL were shown to induce epithelial–mesenchymal transdifferentiation of PTECs. After stimulation of PTECs with serotonin or PL, matrix metalloproteinase-2, tissue inhibitor of metalloproteinase-1, and collagen-α1 gene expressions, which were reported to be elevated in renal injury, were determined by real-time PCR and found to be upregulated. Expressions of some inflammatory cytokines such as tumor necrosis factor-α, interleukin-6, and transforming growth factor-β1 were found to be increased in both protein and gene levels. Recently there is no published report on the effect of serotonin on renal PTECs. Results obtained in this study have lightened the role of serotonin and platelet-mediated effects of serotonin on fibrotic and inflammatory processes in PTECs.
Introduction
Renal diseases are among the most important causes of morbidity and mortality in many countries. In various renal injuries, tissue damage occurs and platelet activation is observed. Recent studies propose that activated platelets release some factors and they may participate in fibrosis and inflammation observed after renal injury. Platelets are the major serotonin storage site out of central nervous system (CNS)Citation1,Citation2 and like other contents, serotonin is also released upon platelet activation into local microenvironment of injured area.Citation3 Although effects of serotonin are primarily studied in CNS; serotonin synthesis was shown in renal proximal tubular epithelial cells (PTECs); serotonin receptors were identified in kidneyCitation4–12 and it was reported that serotonin has important effects on renal metabolism and glomerular function.Citation6 Although serotonin was shown to affect renal functions, responses to serotonin were proposed to be different.Citation13–15 Consequently serotonergic responses were reported to be dependent on species and type of vascular zone; besides even in the same vascular zone, serotonin-induced effects have been reported to be different according to the receptor subtype in the tissue.Citation15–17
In renal injury, levels of various factors increase and among these factors, transforming growth factor-β1 (TGF-β1) is the key fibrogenic factor in tubular epithelial–myofibroblast transdifferentiation (EMT) and it plays a critical role in fibrosis and macrophage accumulation.Citation18,Citation19 During the healing process of renal tissue, initially platelets aggregate, neutrophils migrate to injury site, and basal epithelial cells start to proliferate. Over time, macrophages replace neutrophils, granulation tissue is formed, neovascularization occurs, fibroblasts begin to proliferate and collagen accumulation in injured tissue starts. Appearance of myofibroblasts in granulation tissue contributes to wound closure and significantly leads to decreased size of the original injury.Citation20 Recent studies suggest that tubular EMT is an important and complex process in the tubulointerstitial fibrosis and this phenotypic transformation has recently been reported in progressing human glomerulonephritis. In many studies, PTECs are claimed to be transdifferentiate into fibroblasts/myofibroblasts during renal injury.Citation18
In this study, we aimed to investigate whether platelets or platelet-released serotonin regulate PTECs. Therefore, we stimulate obtained PTECs by primary cell culture and treated with platelet lysate (PL) (2 × 106/mL, 4 × 106/mL, 8 × 106/mL) or serotonin (1 μM or 5 μM) for 12 or 24 h. Phenotypic transdifferentiation of these cells into myofibroblasts was demonstrated with light microscope and α-smooth muscle actin (α-SMA) gene expression. We also determined TGF-β1 protein and gene expression levels. Furthermore, matrix metalloproteinase-2 (MMP-2), tissue inhibitor of metalloproteinase-1 (TIMP-1), and collagen-α1 (COL-α1) gene expressions which are reported to be elevated in renal injury and protein and gene expressions of some inflammatory cytokines such as tumor necrosis factor-α (TNF-α) and interleukin-6 (IL-6) were investigated. Achieved results demonstrated for the first time that platelet-derived serotonin regulates renal PTECs by increasing secretion of TGF-β1, MMP-2, and inflammatory cytokines and promoting their transdifferentiation into myofibroblasts.
Materials and methods
Materials
In primary cell culture, DMEM/F-12, HBSS, HEPES, insulin-transferrin-selenium, and newborn calf serum were purchased from Gibco/Life Technologies; Type I collagen rat-tail was purchased from Millipore and all other chemicals were purchased from Sigma-Aldrich (Steinheim, Germany). Immunocytochemistry staining was obtained with FloidTM Cell Imaging Station (Life Technologies, Carlsbad, CA).
Mouse PTEC culture
This study was approved by the Animal Ethics Committee of Hacettepe University (2014/32-4), and all animal experiments were carried out in accordance with the U.K. Animals (Scientific Procedures) Act, 1986 and associated guidelines. Primary culture of mouse PTECs were achieved by a modification of previously described method of Terryn et al.Citation21 Kidneys were isolated from male Swiss albino mice (6–8 weeks) and renal cortices were dissected in ice-cold dissection solution (HBSS within glucose, glycine, alanine, HEPES, pH 7.4) and sliced into small pieces. The pieces were transferred into collagenase solution (dissection solution with 0.1% (wt/vol) Type II collagenase and soybean trypsin inhibitor) at 37 °C and digested for 30 min. The homogenate was re-suspended into the appropriate amount of culture medium DMEM/F12 without phenol red (supplemented with newborn calf serum, HEPES, sodium pyruvate, L-glutamine, hydrocortisone, insulin-transferrin-selenium, nonessential amino acids, penicillin, and streptomycin). Plates were pre-coated with Type I collagen:phosphate-buffered saline (PBS) (1:5) combination and incubated overnight at 4 °C. The proximal tubule fragments were seeded onto collagen-coated plates and left unstirred for 48 h at 37 °C and 95% air-5% CO2 in a standard humidified incubator (MCO-18AC-PE, SANYO Electric Co.). The medium was then changed every 2 days.
Immunocytochemical characterization of PTECs
Cell nuclei were stained with NucBlueTM Live Cell Stain (Life Technologies, OR) to demonstrate cell viability. In order to prove the purity of PTECs, characterization was performed with positive and negative markers under light microscope. In PTECs, aquaporin-1 (AQP-1), aminopeptidase N (CD13), and megalin are expressed in apical/basolateral membranes, brush border membranes, and epithelial cell membranes respectively.Citation22–24 Aquaporine-2 (AQP-2) is expressed in apical cell membranes of connecting tubules and collecting ducts.Citation8 Considering these data, rabbit polyclonal anti-AQP-1 antibody (1:200; StressMarq Biosciences, Victoria, BC, Canada)Citation22, rabbit polyclonal anti-Lrp2/Megalin antibody (1:200; Bioss, Woburn, MA)Citation22, and rabbit polyclonal anti-CD13 (1:200; Bioss, Woburn, MA) were used as positive markers and rabbit polyclonal anti-AQP-2 antibody (1:200; StressMarq Biosciences, Victoria, BC, Canada)Citation25 was used as negative marker.
Platelet isolation
Peripheral blood was collected and centrifuged at 150× g for 10 min at room temperature. The platelet-rich plasma was aspirated and centrifuged at 1500 × g for 15 min to obtain platelet pellet. The pellet was re-suspended in PBS and re-centrifuged at 200 × g for 5 min to eliminate any remaining white and red blood cells. The platelets were counted, suspensions were adjusted to appropriate concentration, then aliquoted and immediately frozen at −80 °C. Platelet activation was obtained by freezing and thawing cycle which was previously reported and this last preparation is defined as PL.Citation26
Treatment of cells
In order to demonstrate the effects of serotonin or PL stimulation on PTECs, cells were starved with serum-free DMEM/F12 for 24 h and incubated with 1 or 5 μM of serotonin or 2 × 106/mL, 4 × 106/mL, 8 × 106/mL of PL for either 12 or 24 h. Following incubation period, media and cell lysates were collected for ELISA and RT-PCR assays, respectively.
ELISAs
Mouse IL-6, TGF-β1, and TNF-α ELISA kits (Novex/Life Sciences, Frederick, MD) were performed according to the manufacturer’s instructions to determine the levels of inflammatory cytokines in culture media of PTECs.
RNA isolation, cDNA synthesis, and quantitative RT-PCR analysis
Following the stimulation period, PTECs were scraped with lysis buffer and RNA isolation was performed according to the manufacturer’s instructions by using PureLinkTM RNA Mini Kit (Ambion/Life Technologies, Carlsbad, CA). The concentration of RNA was determined by measuring the absorbance at 260 nm in a spectrophotometer (Shimadzu UV-1700, Tokyo, Japan). First-strand cDNA was synthesized from up to 1 μg of total RNA by reverse transcription for 60 min at 42 °C in a final volume of 20 μL of RT buffer with 200 U of Superscript II (Invitrogen/Life Technologies, Carlsbad, CA), 3 μM random primers, 1 μL deoxyribonucleoside triphosphates (dNTPs), 0.1 M dithiothreitol (DTT), and 40 U RNaseOUT according to manufacturer’s instructions.
Real-time PCR analysis was performed in 96-well plates by using ViiATM 7 Software (Life Technologies/Applied Biosystems, Carlsbad, CA) system and by using SYBR Green PCR Master Mix. Amplification reactions (25 μL) were carried out in duplicate with 5 μL of 1:5 diluted template cDNA according to manufacturer’s protocol. Each assay was normalized with GAPDH (housekeeping gene) gene expression from the same cDNA sample. The parameters included a single cycle of 94 °C for 10 min followed by 40 cycles of 95 °C for 15 s, annealing, and 60 °C for 1 min. All primer set used () was previously assessed to check for amplification in PTECs.
Table 1. Primer sets used in RT-PCR experiments.
Statistical analysis
Statistical analyses were performed by GraphPad Prism 6.0 software (GraphPad Software, San Diego, CA) for Mac OSX. Results are expressed as mean ± SD. Experimental groups were compared using Student’s t-test or one-way ANOVA tests. A value of p < 0.05 was considered as significant.
Results
Primary PTEC culture and immunocytochemical characterization
Proximal tubules were observed on the second day of seeding under light microscope. Starting from the second day, PTECs migrate from proximal tubules to the plate surface pre-coated with collagen. PTECs were shown to grow slowly and became confluent in 10 days and showed the characteristic cobblestone appearance ().
Figure 1. Light microscopy images and characterization of PTECs. Proximal tubules on the first day of seeding at 10× magnification (A) and 40× magnification (B); PTECs on the 10th day of seeding at 10× magnification (C) and 40× magnification (D). Immunocytochemical staining of PTECs with NucBlueTM Live Cell Stain (E), megalin (F), AQP-1 and AQP-2 (G), and aminopeptidase N (H).
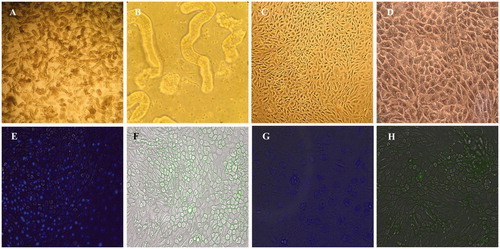
Detection of blue fluorescent confirmed the cell viability of PTECs () and immunocytochemical staining were performed in order to confirm the purity of primary cell culture. Megalin and aminopeptidase N that are considered as proximal tubule markers both showed positive green staining in cell membranes in accordance with the manufacturer’s guidelines ().Citation22–24 In order to demonstrate positive and negative staining, AQP-1 and AQP-2 antibodies were used together in the same wells. While AQP-1 antibody showed positive blue fluorescent in PTEC membranes, AQP-2 expression was not observed in PTECs as expected ().
Phenotypic transdifferentiation and α-SMA upregulation
After stimulation with 1 or 5 μM of serotonin or 2 × 106/mL, 4 × 106/mL, 8 × 106/mL of PL, PTECs were shown to lose their characteristic cobblestone appearance and differentiate into myofibroblast phenotype (). Phenotypic transdifferentiation was also confirmed with a significant dose-dependent increase in α-SMA gene expression levels after stimulation with serotonin (1 or 5 μM) or PL (2 × 106/mL, 4 × 106/mL, 8 × 106/mL) (p < 0.001) ().
Figure 2. Phenotypic transdifferentiation of PTECs under light microscope (10× magnification). Before stimulation (A); after stimulation with 1 μM serotonin for 12 h (B); 5 μM serotonin for 24 h (C); 4 × 106/mL of PL for 12 h (D); 4 × 106/mL of PL for 24 h (E); 8 × 106/mL of PL for 24 h (F); α-SMA gene expressions of PTECs after stimulation with serotonin or PL for 12 h or 24 h (G) (***p < 0.001, *p < 0.05 vs. control, n = 6).
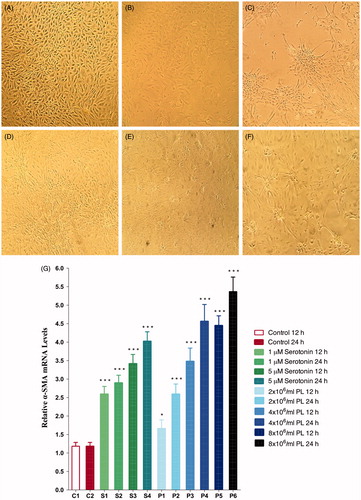
TGF-β1 is a critical mediator in activation before fibrosis and transdifferentiation of PTECs into myofibroblasts. Both serotonin and PL were shown to induce TGF-β1 gene expression and secretion dose dependently ().
TNF-α and IL-6 gene expressions and protein levels
To investigate the proinflammatory effects of platelet-derived serotonin on PTECs, IL-6, and TNF-α gene expressions and protein levels were determined. As presented in , stimulation with 1 or 5 μM serotonin or 2 × 106/mL, 4 × 106/mL, 8 × 106/mL of PL both increased IL-6 and TNF-α gene expressions and protein levels.
MMP-2, TIMP-1, and COL-α1 gene expressions
MMP-2, TIMP-1, and COL-α1 gene expressions were found to increase both dose- and time-dependently with serotonin (1 or 5 μM) or PL (2 × 106/mL, 4 × 106/mL, 8 × 106/mL) stimulation (p < 0.001) (). Upregulating effects of serotonin and PL on MMP-2, TIMP-1, and COL-α1 gene expressions suggests that platelets may play a role in the degradation of ECM. Stimulation with PL resulted in higher increase in gene expressions suggesting that other factors released from platelets may increase the effects of serotonin.
Discussion
Renal injuries are among the most important causes of morbidity and mortality in developed countries. In various acute and chronic renal diseases, tissue damage occurs and platelet activation is observed. Recent studies suggest that some factors released from activated platelets may participate in inflammation and fibrosis observed after renal injury.Citation27,Citation28 Serotonin is one of those factors and its effects on PTECs during renal injury has not been investigated yet. Investigation of these effects is important for slowing down or stopping the active pathway processes which are developed during renal injury and for synthesis of new drugs for treatment of renal injury. Renal PTECs are known to play a role in response to renal injury by phenotypic transdifferentiation. Because of this phenotypic transformation into myofibroblasts, these cells gain ability to secrete MMPs and TIMPs.
During normal healing process in damaged renal tissue, the phenotype of the PTECs is necessary to differentiate into myofibroblasts.Citation29,Citation30 Typical renal injury recovery consists of three phases: inflammation, proliferation, and remodeling. Myofibroblasts occur during the early phase of tissue formation in wound-healing processes; become more evident in the proliferation phase and disappear, probably with an apoptotic mechanism, during subsequent period of recovery.Citation31 Myofibroblast, which is characterized by α-SMA expression, is the main source of ECM and is the best prognostic marker of disease progression in experimental glomerulonephritis in humans. Therefore, it is important to understand the origin of myofibroblasts and mechanisms involved in the process.Citation18 Once myofibroblasts occur, their contraction abilities continue for a long time. This contraction is provided by tension caused by stress fibers in ECM-surrounding tissues. This contractile phenotype plays an important role in wound healing. This contraction is then stabilized by the deposition of ECM, in particular collagen, and thus become permanent.Citation32
In this study, PTECs were stimulated in vitro with serotonin (1 or 5 μM) or PL (2 × 106/mL, 4 × 106/mL, 8 × 106/mL) and cell responses were investigated. With all stimulations (serotonin or PL), PTEC transdifferentiation into myofibroblasts was detected microscopically and quantitatively by dose-dependent increase in α-SMA gene expressions.
TGF-β1 plays a crucial role in progression of renal fibrosis and is one of the most important regulators in transdifferentiation of PTECs into myofibroblasts. TGF-β1 and its downstream SMAD pathway are considered to be a key regulator in pathogenesis of renal fibrosis in experimental animal models and human renal diseases. It also regulates renal fibrosis by stimulating ECM synthesis and inhibiting ECM degradation.Citation33 In this study, following the stimulation of PTECs with serotonin or PL, both TGF-β1 protein levels and relative gene expressions showed a dose-dependent increase, suggesting that platelet-derived serotonin is among the important mediators of increase in TGF-β1 levels and confirmed that TGF-β1 regulates the transdifferentiation of PTECs into myofibroblasts.
Fibrosis is associated with both qualitative and quantitative changes in the ECM and most significant structural feature of fibrosis is the increase in ECM accumulation in damaged tissue. Most of the studies involving kidney are focused on MMP-2 and MMP-9 among MMP subtypes. Studies in various animal models have shown that MMP-2 and MMP-9 expressions and activities are upregulated in renal fibrosis.Citation34–38 A recent study, performed with MMP-2 null mice, reported that fibrogenesis is not accelerated and MMP-2 overexpression is essential to stimulate tubulointerstitial fibrosis in PTECs.Citation39 MMP-2 has also been shown to be necessary to induce in vitro tubular EMT.Citation40 In literature, renal injury models carried out in various human and rodent models have shown increased expression of TIMP-1 in both protein and gene levels, suggesting that TIMP-1 is involved in early periods of renal damage progression.Citation34 During renal damage, fibrosis is progressive and expansion of fibrotic tissue results in damage of healthy tissues and organs. Although molecular mechanism of fibrosis has not yet fully understood; uncontrolled synthesis and accumulation of collagen is shown to be responsible for fibrotic alterations in studies performed with humans and experimental study models.Citation41 In various types of experimental fibrotic renal injuries induced by different chemical compounds in rats; Type I, III, and IV collagen expressions were reported to be increased.Citation42–45 Besides it has been demonstrated that Type I collagen accumulation is increased in interstitial fibrotic areas of unilateral urethral obstruction modelCitation46 and tubulointerstitial scar tissue is mainly consisted of fibronectin, tenascin, and Type I and III collagen.Citation47 Tubulointerstitial fibroblasts and PTECs have been reported to synthesize in vitro Type I and III procollagens in addition to other ECM components.Citation48–50 Myofibroblasts have also been shown to maintain the ability of fibroblasts to synthesize interstitial Type I and III collagen.Citation48,Citation51 In this present study, MMP-2, TIMP-1, and COL-α1 gene expressions were found to be elevated following 12 and 24 h of serotonin or PL stimulation; suggesting that platelets participate in ECM matrix degradation. Increase in gene expressions was higher with PL stimulation compared to serotonin stimulation, proposing that other factors released from platelets may enhance the effects of serotonin.
This study is also focused on identifying potential proinflammatory effects of serotonin and PL on renal PTECs. Following renal injury, the first phase of the tissue repair process is the inflammatory response. During the inflammatory phase, agents such as vascular endothelial growth factor, thrombin, and histamine are secreted from mast cells, monocytes, macrophages, and circulating platelets that are activated in response to injury, to increase vascular permeability. Consequently, platelets and leucocytes accumulate at damaged area and platelets are activated by contacting with collagen and other ECM components. Following activation, platelets are activated and secrete the contents of the dense granules and α-granules, including serotonin, to microenvironment.Citation52
Previous studies revealed that IL-6 levels are elevated in patients with chronic renal injury.Citation53 However, the exact role of IL-6 in the pathogenesis of renal fibrosis is unknown. In this study, stimulation of PTECs with serotonin or PL was represented to dose dependently increase IL-6 protein levels and gene expression; suggesting that the regulatory effects of serotonin on IL-6 formation is in transcriptional level. Some previous studies have reported that increase in TNF-α and IL-6 levels in various renal injuries are related.Citation54–56 Therefore, we aimed to demonstrate the effects of serotonin or PL on TNF-α expression. Stimulation with serotonin or PL resulted in a dose-dependent increase in TNF-α expressions.
In this study, platelets and platelet-derived serotonin have been demonstrated to play an important role in regulation of renal PTECs. Recently no literature indicates the effects of serotonin on renal PTECs. After reaching the site of action, platelets are activated and therefore serotonin is released to the microenvironment and exposure of cells to serotonin is known to be increased. Therefore, it is important to determine the role of serotonin in damage or healing processes. Obtained results have lightened the role of serotonin and platelet-mediated effects of serotonin on PTECs. These results are ought to be supported with in vivo tubulointerstitial fibrosis model studies. Thus the role of serotonin in the process, resulting in inflammation and fibrosis during renal injury will be determined comprehensively.
Disclosure statement
The authors report no conflicts of interest. The authors alone are responsible for the content and writing of the paper.
References
- Mohammad-Zadeh LF, Moses L, Gwaltney-Brant SM. Serotonin: A review. J Vet Pharmacol Therapeut. 2008;31:187–199.
- Papadimas GK, Tzirogiannis KN, Mykoniatis MG, Grypioti AD, Manta GA, Panoutsopoulos GI. The emerging role of serotonin in liver regeneration. Swiss Med Weekly. 2012;142:w13548.
- Kroll MH, Afshar-Kharghan V. Platelets in pulmonary vascular physiology and pathology. Pulm Circul. 2012;2:291–308.
- Verbeke M, Van de Voorde J, de Ridder L, Lameire N. Beneficial effect of serotonin 5-HT2-receptor antagonism on renal blood flow autoregulation in cyclosporin-treated rats. J Am Soc Nephrol. 1999;10:28–34.
- Friedrich F, Paulmichl M, Kolb HA, Lang F. Inward rectifier K channels in renal epithelioid cells (MDCK) activated by serotonin. J Membr Biol. 1988;106:149–155.
- Sole MJ, Madapallimattam A, Baines AD. An active pathway for serotonin synthesis by renal proximal tubules. Kidney Int. 1986;29:689–694.
- Hayashi M, Yamaji Y, Kitajima W, Saruta T. Aromatic L-amino acid decarboxylase activity along the rat nephron. Am J Physiol. 1990;258:F28–F33.
- Takata K, Matsuzaki T, Tajika Y, Ablimit A, Hasegawa T. Localization and trafficking of aquaporin 2 in the kidney. Histochem Cell Biol. 2008;130:197–209.
- Hoyer D, Clarke DE, Fozard JR, et al. International Union of Pharmacology classification of receptors for 5-hydroxytryptamine (serotonin). Pharmacol Rev. 1994;46:157–203.
- Barnes NM, Sharp T. A review of central 5-HT receptors and their function. Neuropharmacology. 1999;38:1083–1152.
- Hannon J, Hoyer D. Molecular biology of 5-HT receptors. Behav Brain Res. 2008;195:198–213.
- Raymond JR, Mukhin YV, Gelasco A, et al. Multiplicity of mechanisms of serotonin receptor signal transduction. Pharmacol Therapeut. 2001;92:179–212.
- Shoji T, Tamaki T, Fukui K, Iwao H, Abe Y. Renal hemodynamic responses to 5-hydroxytryptamine (5-HT): Involvement of the 5-HT receptor subtypes in the canine kidney. Eur J Pharmacol. 1989;171:219–228.
- Morán A, Velasco C, Martín ML, San Román L. Renal vasoconstrictor response to 5-hydroxytryptamine in the in situ autoperfused rat kidney: Involvement of angiotensin II and the 5-HT2 receptor activation. Eur J Pharmacol. 1997;330:205–211.
- Tian RX, Kimura S, Kondou N, et al. DOI, a 5-HT2 receptor agonist, induces renal vasodilation via nitric oxide in anesthetized dogs. Eur J Pharmacol. 2002;437:79–84.
- Calama E, Garcia M, Jarque MJ, Moran A, Martin ML, San Roman L. 5-Hydroxytryptamine-induced vasodilator responses in the hindquarters of the anaesthetized rat, involve beta2-adrenoceptors. J Pharm Pharmacol. 2003;55:1371–1378.
- Calama E, Moran A, Ortiz de Urbina AV, Martin ML, San Roman L. Vasoconstrictor responses to 5-hydroxytryptamine in the autoperfused hindquarters of spontaneously hypertensive rats. Pharmacology. 2004;71:66–72.
- Lan HY. Tubular epithelial-myofibroblast transdifferentiation mechanisms in proximal tubule cells. Curr Opin Nephrol Hypertens. 2003;12:25–29.
- Kie JH, Kapturczak MH, Traylor A, Agarwal A, Hill-Kapturczak N. Heme oxygenase-1 deficiency promotes epithelial-mesenchymal transition and renal fibrosis. J Am Soc Nephrol. 2008;19:1681–1691.
- Liu B, Connolly MK. The pathogenesis of cutaneous fibrosis. Semin Cutan Med Surg. 1998;17:3–11.
- Terryn S, Jouret F, Vandenabeele F, et al. A primary culture of mouse proximal tubular cells, established on collagen-coated membranes. Am J Physiol Renal Physiol. 2007;293:F476–F485.
- Hara-Chikuma M, Verkman AS. Aquaporin-1 facilitates epithelial cell migration in kidney proximal tubule. J Am Soc Nephrol. 2006;17:39–45.
- Kotlo K, Shukla S, Tawar U, Skidgel RA, Danziger RS. Aminopeptidase N reduces basolateral Na+ -K+ -ATPase in proximal tubule cells. Am J Physiol Renal Physiol. 2007;293:F1047–F1053.
- Verroust PJ, Christensen EI. Megalin and cubilin–the story of two multipurpose receptors unfolds. Nephrol Dial Transpl. 2002;17:1867–1871.
- Kamiyama M, Garner MK, Farragut KM, Kobori H. The establishment of a primary culture system of proximal tubule segments using specific markers from normal mouse kidneys. Int J Mol Sci. 2012;13:5098–5111.
- Yabanoglu S, Akkiki M, Seguelas M-H, Mialet-Perez J, Parini A, Pizzinat N. Platelet derived serotonin drives the activation of rat cardiac fibroblasts by 5-HT2A receptors. J Mol Cell Cardiol. 2009;46:518–525.
- Hamasaki Y, Doi K, Maeda-Mamiya R, et al. A 5-hydroxytryptamine receptor antagonist, sarpogrelate, reduces renal tubulointerstitial fibrosis by suppressing PAI-1. Am J Physiol Renal Physiol. 2013;305:F1796–F1803.
- Tang WW, Ulich TR, Lacey DL, et al. Platelet-derived growth factor-BB induces renal tubulointerstitial myofibroblast formation and tubulointerstitial fibrosis. Am J Pathol. 1996;148:1169–1180.
- Meran S, Steadman R. Fibroblasts and myofibroblasts in renal fibrosis. Int J Exp Pathol. 2011;92:158–167.
- Darby IA, Hewitson TD. Fibroblast differentiation in wound healing and fibrosis. Int Rev Cytol. 2007;257:143–179.
- Li B, Wang JH. Fibroblasts and myofibroblasts in wound healing: Force generation and measurement. J Tissue Viabil. 2011;20:108–120.
- Gabbiani G. The myofibroblast in wound healing and fibrocontractive diseases. J Pathol. 2003;200:500–503.
- Yanagita M. Inhibitors/antagonists of TGF-beta system in kidney fibrosis. Nephrol Dial Transpl. 2012;27:3686–3691.
- Genovese F, Manresa AA, Leeming DJ, Karsdal MA, Boor P. The extracellular matrix in the kidney: A source of novel non-invasive biomarkers of kidney fibrosis? Fibrogen Tissue Rep. 2014;7:4.
- LeBleu VS, Teng Y, O’Connell JT, et al. Identification of human epididymis protein-4 as a fibroblast-derived mediator of fibrosis. Nat Med. 2013;19:227–231.
- Catania JM, Chen G, Parrish AR. Role of matrix metalloproteinases in renal pathophysiologies. Am J Physiol Renal Physiol. 2007;292:F905–F911.
- Gonzalez-Avila G, Iturria C, Vadillo-Ortega F, Ovalle C, Montano M. Changes in matrix metalloproteinases during the evolution of interstitial renal fibrosis in a rat experimental model. Pathobiology. 1998;66:196–204.
- Turck J, Pollock AS, Lee LK, Marti HP, Lovett DH. Matrix metalloproteinase 2 (gelatinase A) regulates glomerular mesangial cell proliferation and differentiation. J Biol Chem. 1996;271:15074–15083.
- Zeisberg M, Neilson EG. Mechanisms of tubulointerstitial fibrosis. J Am Soc Nephrol. 2010;21:1819–1834.
- Cheng S, Lovett DH. Gelatinase A (MMP-2) is necessary and sufficient for renal tubular cell epithelial-mesenchymal transformation. Am J Pathol. 2003;162:1937–1949.
- Razzaque MS, Taguchi T. Cellular and molecular events leading to renal tubulointerstitial fibrosis. Med Elect Microsc. 2002;35:68–80.
- Cheng M, Razzaque MS, Nazneen A, Taguchi T. Expression of the heat shock protein 47 in gentamicin-treated rat kidneys. Int J Exp Pathol. 1998;79:125–132.
- Razzaque MS, Taguchi T. Localization of HSP47 in cisplatin-treated rat kidney: A possible role in tubulointerstitial damage. Clin Exp Nephrol. 1999;3:222–228.
- Razzaque MS, Shimokawa I, Nazneen A, Higami Y, Taguchi T. Age-related nephropathy in the Fischer 344 rat is associated with overexpression of collagens and collagen-binding heat shock protein 47. Cell Tissue Res. 1998;293:471–478.
- Liu D, Razzaque MS, Cheng M, Taguchi T. The renal expression of heat shock protein 47 and collagens in acute and chronic experimental diabetes in rats. Histochem J. 2001;33:621–628.
- Yokoi H, Mukoyama M, Nagae T, et al. Reduction in connective tissue growth factor by antisense treatment ameliorates renal tubulointerstitial fibrosis. J Am Soc Nephrol. 2004;15:1430–1440.
- Moselhy S. Pathogenesis of primary tubulointerstitial nephritis. Egypt J Pediatr Allergy Immunol. 2008;6:35–37.
- Tang WW, Van GY, Qi M. Myofibroblast and [alpha]1(III) collagen expression in experimental tubulointerstitial nephritis. Kidney Int. 1997;51:926–931.
- Creely JJ, DiMari SJ, Howe AM, Hyde CP, Haralson MA. Effects of epidermal growth factor on collagen synthesis by an epithelioid cell line derived from normal rat kidney. Am J Pathol. 1990;136:1247–1257.
- Kuncio GS, Neilson EG, Haverty T. Mechanisms of tubulointerstitial fibrosis. Kidney Int. 1991;39:550–556.
- Hogemann B, Gillessen A, Bocker W, Rauterberg J, Domschke W. Myofibroblast-like cells produce mRNA for type I and III procollagens in chronic active hepatitis. Scand J Gastroenterol. 1993;28:591–594.
- Diegelmann RF, Evans MC. Wound healing: An overview of acute, fibrotic and delayed healing. Front Biosci. 2004;9:283–289.
- Yang J, Chen J, Yan J, et al. Effect of interleukin 6 deficiency on renal interstitial fibrosis. PLoS One. 2012;7:e52415.
- Girndt M, Kohler H, Schiedhelm-Weick E, Schlaak JF, Meyer zum Buschenfelde KH, Fleischer B. Production of interleukin-6, tumor necrosis factor alpha and interleukin-10 in vitro correlates with the clinical immune defect in chronic hemodialysis patients. Kidney Int. 1995;47:559–565.
- Linderholm M, Ahlm C, Settergren B, Waage A, Tarnvik A. Elevated plasma levels of tumor necrosis factor (TNF)-alpha, soluble TNF receptors, interleukin (IL)-6, and IL-10 in patients with hemorrhagic fever with renal syndrome. J Infect Dis. 1996;173:38–43.
- Malide D, Russo P, Bendayan M. Presence of tumor necrosis factor alpha and interleukin-6 in renal mesangial cells of lupus nephritis patients. Human Pathol. 1995;26:558–564.