Abstract
Curcumin has several biological functions particularly antioxidant and anti-inflammatory. The aims of this study are determination of the protective effects of curcumin on cisplatin-induced renal tubular cell apoptosis and related pathways in kidney. Eighteen male Wistar albino rats were randomly divided into three groups (n = 6): the control, cisplatin (CP), and cisplatin + curcumin (CP + CUR). Acute renal damage was induced by single dose of cisplatin (7.5 mg/kg) injected by intraperitoneally (i.p). The animals of curcumin-treated group were received daily 200 mg/kg curcumin per os (po), starting from 2 days before the injection of cisplatin to the day of sacrifice. Forty-eight hours after cisplatin injection, samples of cardiac blood and kidneys were harvested from the animals. In this study, the major finding is that curcumin treatment ameliorates the following conditions associated with cisplatin-induced nephrotoxicity: (1) the development of kidney injury (histopathology), (2) inflammatory responses [myeloperoxidase (MPO) and tumor necrosis factor-alpha (TNF-α), interleukin-1 beta (IL-1β), IL-6, IL-10 levels], (3) the degree of lipid peroxidation [malondialdehyde (MDA) level], (4) renal tubular cell apoptosis (active caspase-3) and expression of related proteins [p53, Fas, and Fas ligand (Fas-L)] by immunohistochemistry, (5) renal dysfunction (serum urea and creatinine). In a conclusion, this study suggests that curcumin has antiapoptotic effect against cisplatin nephrotoxicity, in addition to anti-inflammatory and antioxidant properties.
Introduction
Acute renal failure is frequently encountered during cisplatin chemotherapy, which is therefore an important consideration in restricting the use of the drug.Citation1 Although some strategies exist to prevent cisplatin nephrotoxicity, no recommended specific treatment is currently available.
The pathophysiology of cisplatin-induced acute renal damage is associated with proximal tubular injury and such fundamental mechanisms as oxidative stress, inflammation, and vascular damage. Previous studies have demonstrated the role of many interrelated factors involved in cisplatin-induced proximal tubular damage. These factors include a direct toxic effect on renal tubular cells,Citation2 DNA damage,Citation3 mitochondrial dysfunction,Citation4 apoptosis,Citation5 and autophagy.Citation6 Recent studies have focused on cisplatin-induced renal tubular cell apoptosis, and many apoptotic pathways, including tumor necrosis factor (TNF) receptors or the Fas-activated extrinsic pathway, the intrinsic mitochondrial pathway (Bax pathway) controlled by the Bcl-2 family, and the endoplasmic reticulum (ER) stress pathway, have been described as involved in renal tubular cell death.Citation7 Therefore, previous studies demonstrated that different agents exert the renoprotective effect by inhibiting cisplatin mediated tubular cell apoptosis.Citation8–10
Curcumin, a major component of turmeric, is known to have anti-inflammatory and antioxidant effects in various pathologies.Citation11–17 In addition, recent studies have revealed that the antiapoptotic properties of curcumin against different agent-mediated acute renal injury.Citation11,Citation12,Citation15 It has been reported that curcumin prevents and ameliorates the severity of cisplatin nephrotoxicity, by inhibiting various cytokines and chemokines such as TNF-α and IL-1β and scavenging free radicals.Citation4,Citation14,Citation17–19 However, there is no any study to support the antiapoptotic effect of curcumin in cisplatin-induced nephrotoxicity, yet. Therefore, in this study, we evaluated the effects of curcumin on renal tubular cell apoptosis (the immunoreactivities of active caspase-3, Fas, Fas-L, and phospho-p53), in addition to inflammation [the levels of IL-1β, TNF-α, IL-6, IL-10, and myeloperoxidase (MPO)], lipid peroxidation [malondialdehyde (MDA)], and renal function (urea and creatinine levels) in cisplatin-mediated acute renal damage.
Materials and methods
Animals
Eighteen 4-month-old male Wistar albino rats were obtained from the Experimental Animal Center of Trakya University. The animals were fed daily tap water and pellet foods, including 21% pure protein, under optimum laboratory conditions (temperature, 22 ± 2 °C; humidity, 50–55%; light/dark period: 12 h/12 h, optimum air conditioning system). All animals received humane care according to the criteria outlined in the Guide for the Care and Use of Laboratory Animals prepared by the National Academy of Sciences and published by the National Institutes of Health (Bethesda, MD). The design of the study and the experimental protocol were approved by the Ethical Committee of Trakya University (TÜHDYEK 2013/53).
Experimental protocol
Animals were randomized into three groups (n = 6) as follows: control, cisplatin (CP), and cisplatin + curcumin (CP + CUR). Acute renal damage was induced by a single dose of cisplatin (Sigma-Aldrich, Saint Louis, MO) (7.5 mg/kg, dissolved in saline), injected intraperitoneally (i.p). The animals of the curcumin-treated group received daily per os (po) 200 mg/kg curcumin (Sigma-Aldrich, Saint Louis, MO), dissolved in corn oil, starting from 2 days before the injection of cisplatin to the day of sacrifice. Control animals were treated with corn oil (a vehicle for curcumin) in the same way. The doses of curcuminCitation4 and cisplatinCitation20 were chosen on the basis of previously published studies.
Forty-eight hours after cisplatin injection, under xylazine/ketamine anesthesia, cardiac blood and the right and left kidneys of all the animals were harvested. For histopathological examination, the right kidneys were fixed in 10% formalin and the left kidneys were stored at −80 °C until biochemical analysis.
Histopathological examination
Kidney tissues were fixed in 10% formalin, and then standard dehydration and paraffin-embedding procedures were performed. 5 μm kidney sections were cut and stained with hematoxylin-eosin (H&E). Light microscopic analysis (×400 magnification) of the kidneys was performed in 20 (10 cortical, 10 outer medullae) randomly selected areas in each section by blinded observation. To evaluate acute kidney injury, tubulointerstitial damage involving tubular necrosis, tubular atrophy, tubular lumen dilation, and inflammatory cell infiltration were scored on a scale from 0 to 4: 0 = normal, 0.5 = small focal areas, 1 = involvement of <10% of the cortices and outer medullae, 2 = 10–25% involvement of the cortices and outer medullae, 3 = 25–75% involvement of the cortices and outer medullae, and 4 = extensive damage involving more than 75% of the cortices and outer medullae.Citation21 Their average score was used for comparison.
Immunohistochemistry
Sections were incubated at 56 °C overnight, and then deparaffinized in xylene and rehydrated. They were boiled in citrate buffer (10 mM; pH 6.0) for antigen retrieval. The sections were immersed in 3% H2O2 to inhibit endogenous peroxidase activity. They were then incubated with 5% normal goat serum (Vector Laboratories, Burlingame, CA) at room temperature, to block the nonspecific binding of antibodies, after which the sections were incubated with primary antibody polyclonal rabbit active caspase-3 in dilution 1/200 (AB3623, Millipore, Darmstadt, Germany), polyclonal rabbit Fas; 1/100 (NB120-13550 Novus Biologicals, Littleton CO), polyclonal rabbit Fas-L; 1/100 (ab-15285 abcam, Cambridge, UK), overnight at 4 °C; monoclonal rabbit anti-phospho-p53; 1/200 [(Ser392), klon EP155Y, Millipore, Darmstadt, Germany] for 1.5 h at room temperature. At room temperature, biotinylated anti-rabbit antibody (Vector Laboratories) in 1/400 dilution was added for 30 min. Negative controls included staining tissue sections with the omission of the primary antibody. The antigen–antibody complex was detected using a streptavidin-biotin-peroxidase kit (Vector Laboratories). Finally, sections were incubated with 3.3-diaminobenzidine (DAB, Vector Laboratories), so as to visualize immunolabeling, and counterstained with Mayer’s hematoxylin.
The active caspase-3, and phospho-p53 immunostaining cells were identified by intense brown nuclear staining, and 20 (10 cortical, 10 outer medullae) randomly selected areas (×400 magnification) were evaluated in each section. Data were expressed as the average of cell numbers per sample. The immunoreactivity of Fas and Fas-L was described as a histological score (H-SCORE) obtained by multiplying the number of activated cells (0–100% of cells) by the intensity of staining (1 = low, 2 = medium, 3 = high). The sections were observed under a microscope at high magnification (×400) in the 20 randomly selected areas using a double blind protocol. The average score of all groups was used for comparison.
Assessment of renal function
Blood samples were prepared for biochemical analysis, and then the serum urea and creatinine levels were measured using ADVIA 1800 Chemistry System (Siemens Healthcare diagnostics, Tokyo, Japan). Concentrations of urea and creatinine were given as mg/dL.
Measurement of MPO and cytokine levels
MPO activity as markers of inflammation, proinflammatory cytokines TNF-α, IL-1β, IL-6, and anti-inflammatory cytokine IL-10 concentrations were evaluated in kidney samples frozen at −80 °C. MPO (SEA601Ra), TNF-α (SEA133Ra), IL-1β (SEA563Ra), IL-6 (SEA079Ra), and IL-10 (SEA056Ra) were determined with RAT Platinum Enzyme-linked Immunosorbent Assay (ELISA) Kits (WuhanUSCN Business Co. Ltd., Houston, TX), according to the manufacturer’s instructions, using an absorbance reader (BioTek ELx800, BioTek, Winooski, VT) at 450 nm. Results were calculated by the four-parameter curve method and expressed as picograms (pg)/mg tissue.
Detection of lipid peroxidation
MDA, a marker of lipid peroxidation, was estimated to indicate peroxide damage to membranes as a result of inflammation. MDA levels in kidney tissues were measured according to Jain et al.’s method.Citation22 The results were expressed as nmol/mg.
Statistical analysis
Statistical analyses were performed using SPSS 20.0 software (Licence No: 10240642) provided by Trakya University Faculty of Medicine, Department of Biostatistics and Medical Computation. Differences in measurements of the parameters among the groups were analyzed using the Kruskal–Wallis test. The Mann–Whitney U test was also used when significant results were obtained. A p value of <.05 was considered statistically significant.
Results
Histopathological findings
Light microscopic examination of the kidney tissues of the control group represented a normal histological structure (). Forty-eight hours after exposure to a single dose of cisplatin, tubular necrosis, atrophy, luminal dilatation, and inflammatory cell infiltration were detected, especially in the corticomedullary region of the rat kidneys (). As a result, the tissue damage score was significantly higher than that of the control group (p < .01, ). However, the curcumin treatment significantly decreased the severity of damage (), and consequently the tissue damage score in this group was also lower than that of the cisplatin group (p < .01, ).
Figure 1. Photomicrographs illustrate morphological (H&E, ×200) (A–C) and apoptotic (active caspase-3, ×400) (D–F) changes in rat kidney. Control group (A, D), cisplatin group (B, E), cisplatin + curcumin group (C, F). Caspase-3 immunopositive cells (arrows).
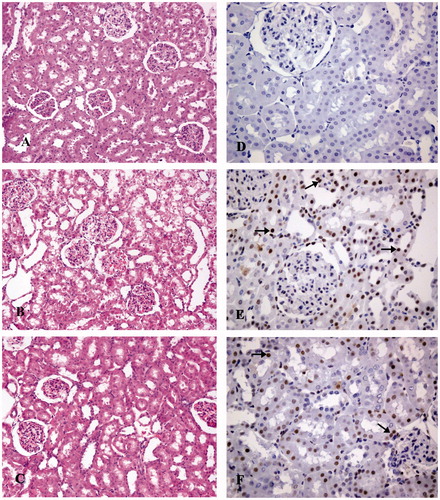
Table 1. Effect of curcumin on renal histopathology, tubular cell apoptosis, and related proteins in cisplatin-induced nephrotoxicity (mean ± SD, n = 6).
Renal tubular epithelial cell apoptosis
Renal tubular epithelial cell apoptosis was evaluated using active caspase-3 immunoreactivity in the study. The results are presented in and . In the kidneys of the control group, relatively few active caspase-3 positive tubular epithelial cells were detected (). Cisplatin treatment caused apoptosis in renal tubular cell of the rats and significantly increased the number of active caspase-3 positive cells () (p < .01). Oral curcumin treatment blocked renal epithelial cell apoptosis and the number of active caspase-3 positive cells were reduced () (p < .01).
Phospho-p53, Fas, and Fas-L immunoreactivity
Phospho-p53 expression was observed in the kidney tissues of all cisplatin-injected groups, excluding the control group, by immunohistochemistry. Renal tubular epithelial cells, especially their nuclei, were stained positively, while a lack of glomerular immunoreactivity was detected ()). Because of an increase in phospho-p53 expression in the rat kidneys 48 h after a single dose of cisplatin (), the number of phospho-p53 positive tubular cells in the renal cortex and outer medulla layer was relatively higher in this group (). Fas expression was observed in the renal specimens of all groups ()). In the renal tubules of the control group (), lightly stained (mostly plasmalemma and apical cytoplasm of tubular cell) and scarce numbers of immunopositive cells were observed, and the mean H-SCORE of the group was 8.00 ± 2.73. In the cisplatin group, moderate degrees of staining intensity were observed (), the mean H-SCORE of that group being significantly higher than that of the control group (p < .01, ). Similarly, exposure to cisplatin also induced an increase in Fas-L expression, observed especially in the apical plasma membrane of the renal tubular cells () and also a 3-fold higher H-SCORE was detected relative to the control group (p < .01, ). On the other hand, curcumin treatment markedly decreased expression of phospho-p53 (), Fas (), and Fas-L () (p < .01, ).
Renal function parameters
As a marker of impaired renal function, serum urea and creatinine values of the rats that were given only cisplatin significantly increased when compared with the control subjects (p < .01).This increase was significantly lower when curcumin was added to the cisplatin ().
Table 2. Effect of curcumin on renal function, MPO activitiy, MDA, and cytokines levels in cisplatin-induced nephrotoxicity (mean ± SD, n = 6).
Myeloperoxidase and cytokine levels
As a consequence of the inflammatory reaction developed in the rat kidneys following application of a single dose of cisplatin, we detected significantly increased tissue MPO activity in rats relative to the control subjects (p < .01), whereas curcumin with anti-inflammatory properties decreased MPO levels (p < .01, ).
Renal TNF-α, pro-, and anti-inflammatory cytokine levels measured using the ELISA method is shown in . Tissue TNF-α and IL-1β levels of the rats in the cisplatin group marked relative to those of the control group. However, curcumin treatment decreased their levels remarkably, without any statistically significant intergroup differences, because of higher standard deviations of the values obtained. As a result of exposure to cisplatin, the levels of the proinflammatory cytokine IL-6 increased significantly (p < .01), while those of the anti-inflammatory cytokine IL-10 decreased (p < .05). However, curcumin treatment significantly ameliorated cytokine levels (p < .05, ).
Lipid peroxidation level
Analysis of the MDA levels of the renal tissues in the experimental groups revealed significantly higher levels of MDA in cisplatin-treated subjects when compared with the controls (p < .01). However, MDA levels significantly decreased with oral curcumin treatment (p < .01, ).
Discussion
Although the mechanisms of cisplatin-induced nephrotoxicity are very complex, in recent years the importance of apoptosis and related pathways, in addition to oxidative stress and inflammation, has been stressed.Citation23 In this study, the major finding is that curcumin treatment ameliorates the following conditions associated with cisplatin-induced nephrotoxicity: (1) development of acute renal injury (histological improvement), (2) inflammation (levels of MPO and cytokines), (3) lipid peroxidation (MDA level), (4) renal tubular cell apoptosis (active caspase-3) and expression of related proteins (p53, Fas, and Fas-L) and (5) renal dysfunction (serum urea and creatinine). To the best of our knowledge, this is the first study to evaluate the effect of curcumin on renal epithelial cell apoptosis in cisplatin-induced nephrotoxicity.
The fundamental mechanisms associated with cisplatin nephrotoxicity are the production of reactive oxygen species (ROS) and depletion of the antioxidant system, as well as accumulation of the products of lipid peroxidation in the renal tissue.Citation2 Cisplatin induces increased production of ROS (particularly hydroxyl radicals), because of a deranged respiratory chain secondary to mitochondrial dysfunction. It has been reported that increased ROS production, along with DNA damage due to the activation of signal pathways involving various protein kinases, phosphorylation, and activation of p53-led cell death, can be responsible for the renal damage and renal failure observed in cisplatin nephrotoxicity.Citation24,Citation25 Therefore, in many experimental models, the renoprotective effects of different antioxidants or oxidant stress inhibitors against cisplatin-nephrotoxicity have been reported.Citation14,Citation24,Citation25 Jiang et al. revealed that dimethylurea (DMTU), which is the pharmacological scavenger of hydroxyl radical and a general antioxidant N-acetylcysteine, suppresses cisplatin-induced tubular cell apoptosis and renal histopathological and functional changes, by decreasing accumulation of hydroxyl radicals and activation of p53.Citation24 Curcumin has been regarded as a bifunctional antioxidant, in that it exerts direct scavenging activity on superoxide, hydroxyl, hydrogen peroxide, and peroxyl radicals, and also promotes the upregulation of various cytoprotective and antioxidant proteins (superoxide dismutase, catalase, and glutathione peroxidase).Citation4,Citation26 In this study, the MDA levels, as an indicator of oxidative stress was elevated in kidney of rats exposure to cisplatin and curcumin treatment caused remarkable reduction in MDA levels, confirming the antioxidant effect of curcumin. Our findings are well matched with previous reports demonstrating similar effects of curcumin on renal damage induced by oxidative stress.Citation4,Citation14,Citation18,Citation19
Inflammatory mechanisms are closely related to the pathogenesis of cisplatin-induced nephrotoxicity. In this study, we determined the increased renal MPO levels, a marker of inflammation, as a result of cisplatin exposure. It is a known fact that cisplatin increases TNF-α expression in renal tubular cells by activating nuclear factor kappa β (NF-Kβ). TNF-α can trigger tubular cell death and tissue damage mediated by TNF receptors 1 and 2. Besides, it can activate proinflammatory cytokines such as interleukins (IL-1β, IL-4, IL-6) and transforming growth factor-beta 1 (TGF-β1).Citation7 In cisplatin nephrotoxicity, increases in the levels of various cytokines such as TNF-α, IL-1β, IL-6, and TGF-β1 have been demonstrated.Citation27,Citation28 Following cisplatin treatment, an increase in TNF-α levels is mostly related to accumulation of ROS and activation of NF-Kβ and p38 MAPK. This relationship has been demonstrated by the renoprotective effect provided by the hydroxyl radical scavenger DMTU, which inhibits TNF-α and p38 MAPK pathways in cisplatin-treated mice.Citation24 In this study, increases in renal concentrations of TNF-α and proinflammatory cytokines IL-1β and IL-6 were observed after exposure to cisplatin, however curcumin treatment partially or significantly decreased the levels of cytokines. Moreover, the decrease in the tissue levels of an anti-inflammatory cytokine IL-10 after the injection of cisplatin was prevented with curcumin treatment. We think that these effects caused from the strong hydroxyl radical scavenging and NF-Kβ inhibiting activities of curcumin. Numerous studies have reported the usefulness of curcumin in various inflammatory conditions, thanks to its regulation of NF-Kβ activity, which inhibits the release of proinflammatory cytokines.Citation13,Citation14,Citation16,Citation17
It has been demonstrated that cisplatin accumulates in the mitochondria of renal cells and consequently promotes production of ROS, decreases calcium absorption in the mitochondria, and finally leads to the release of proapoptotic factors, which induce renal tubular cell death.Citation25 The basic pathways described for cisplatin-induced renal tubular cell apoptosis include the extrinsic pathway, the intrinsic pathway related to mitochondria, and the ER stress pathway. In the extrinsic pathway, the binding of membrane ligands (Fas-L and TNF-α) to cell death receptors (Fas and TNF) induces activation of caspases (caspase-8 and caspase-3), which triggers apoptosis.Citation7 Death receptor-mediated apoptosis in human proximal tubular cellsCitation29 and experimental animalsCitation8,Citation30 has been associated with an increase in Fas and Fas-L expression in renal tissue. Until recently, Fas-L-associated apoptotic stimulus has been known to be mediated by activated T lymphocytes; however, Linkermann et al. demonstrated that Fas-L expressing tubular cells rather than inflammatory cells may play important roles in cisplatin-induced acute renal injury.Citation31 In this study, significant increases in the number of active caspase-3 positive renal tubular cells and expression of Fas and Fas-L in renal tubules were detected immunohistochemically, indicating the role of the apoptosis in cisplatin nephrotoxicity.
Activation of protein kinases and transcription factors (NF-Kβ and p53) are two important apoptotic mechanisms defined in cisplatin-induced renal injury, in addition to caspase and proapoptotic pathways.Citation32 Tumor suppressor protein p53 is considered as an important regulator of cisplatin-mediated cell death. p53 regulates proapoptotic genes, which lead to an increase in the permeabilization of mitochondrial membrane and expression of apoptogenic genes.Citation33 Cisplatin damages DNA directly by binding to DNA and/or indirectly by way of oxidative stress, which occurs as a consequence of the accumulation of free radicals, especially hydroxyl radicals. Thus, the activation of two important protein kinases, ATM (ataxia telangiectasia-mutated) and ATR (ataxia telangiectasia and Rad3-related protein), are responsible for DNA damage and directly induce phosphorylation and activation of p53.Citation24,Citation34 Cisplatin treatment is reported to activate p53 in both in vivo kidneysCitation5 and renal epithelial cell cultures.Citation24 Similarly, we also detected relatively higher phospho-p53 expressions in renal tubular cells after exposure to cisplatin. DMTU, a hydroxyl radical scavenger, has been found to suppress cisplatin nephrotoxicity by inhibiting TNF-α, Fas, Fas-L, and activation of p53.Citation24,Citation30 In addition, it has been reported that epigallocatechin, which has anti-inflammatory and antioxidant properties, blocks cisplatin-mediated apoptosis by inhibiting the Fas/Fas-L pathway and activation of p53.Citation8 It is known that the important effect of the Sirtuin (SIRT) family is regulation of gene expression. SIRT1 reduces the transcriptional efficiency of p53 against oxidative stress and DNA damage and suppresses programmed cell death.Citation35 Additionally, it regulates the DNA repair capacities of cells and plays a key role in inflammation by regulating NF-Kβ.Citation36,Citation37 A recent study by Ugur et al. demonstrated that increased MDA and decreased SIRT1 levels in cisplatin-mediated nephrotoxicity were improved with curcumin treatment.Citation18 In this study, we used curcumin in order to prevent cisplatin nephrotoxicity, because of its antioxidant and strong anti-inflammatory activity by inhibiting NF-Kβ and proinflammatory cytokines. For the first time, we determined that curcumin prevented the development of renal tubular cell apoptosis (active caspase-3) by reducing the activation of proteins (Fas, Fas-L, and p53) involved in cisplatin-induced cell death. On the other hand, recent studies have revealed that the antiapoptotic properties of curcumin against acute renal injury caused by ischemia/reperfusionCitation11,Citation15 and gentamicin.Citation12
In this study, significant increases in serum urea and creatinine levels after exposure to cisplatin were accepted as an indicator of nephrotoxicity. A marked improvement was detected in renal histopathology and function parameters supporting the protective effect of curcumin against oxidative stress, inflammation, and tubular cell death caused by cisplatin-induced nephrotoxicity. Similar effects of curcumin on renal failure caused by cisplatin nephrotoxicity have been revealed in other studies.Citation17–19
In conclusion, the results of our study suggest that curcumin attenuates cisplatin-induced nephrotoxicity by preventing oxidative stress, inflammation, and apoptosis. Thus, we think that the results revealed in this study, for the first time, provides a basis for further research to discover the antiapoptotic effects of curcumin and contribute to the development of preventative treatment against cisplatin-induced nephrotoxicity.
Disclosure statement
The authors report no conflicts of interest. The authors alone are responsible for the content and writing of the paper.
Funding
This work was supported by Scientific and Technological Research Council of Turkey (TUBİTAK-ARDEB-SBAG-113S831).
References
- Hartmann JT, Fels LM, Knop S, Stolt H, Kanz L, Bokemeyer C. A randomized trial comparing the nephrotoxicity of cisplatin/ifosfamide-based combination chemotherapy with or without amifostine in patients with solid tumors. Invest New Drugs. 2000;18:281–289.
- Kuhlmann MK, Burkhardt G, Köhler H. Insights into potential cellular mechanisms of cisplatin nephrotoxicity and their clinical application. Nephrol Dial Transplant. 1997;12:2478–2480.
- Wang D, Lippard SJ. Cellular processing of platinum anticancer drugs. Nat Rev Drug Discov. 2005;4:307–320.
- Waseem M, Kaushik P, Parvez S. Mitochondria-mediated mitigatory role of curcumin in cisplatin-induced nephrotoxicity. Cell Biochem Funct. 2013;31:678–684.
- Wei Q, Dong G, Yang T, Megyesi J, Price PMÇ, Dong Z. Activation and involvement of p53 in cisplatin-induced nephrotoxicity. Am J Physiol Renal Physiol. 2007;293:F1282–F1291.
- Yang C, Kaushal V, Shah SV, Kaushal GP. Autophagy is associated with apoptosis in cisplatin injury to renal tubular epithelial cells. Am J Physiol Renal Physiol. 2008;294:777–787.
- Miller RP, Tadagavadi RK, Ramesh G, Reeves WB. Mechanisms of cisplatin nephrotoxicity. Toxins (Basel). 2010;2:2490–2518.
- Zou P, Song J, Jiang B, et al. Epigallocatechin-3-gallate protects against cisplatin nephrotoxicity by inhibiting the apoptosis in mouse. Int J Clin Exp Pathol. 2014;7:4607–4616.
- Lv Q, Yao Y, Wang W, Xiong W, Liao WH. Biliverdin protects against cisplatin-induced apoptosis of renal tubular epithelial cells. J Huazhong Univ Sci Technolog Med Sci. 2016;36:48–52.
- Li J, Gui Y, Ren J, et al. Metformin protects against cisplatin-induced tubular cell apoptosis and acute kidney injury via AMPKα-regulated autophagy induction. Sci Rep. 2016;6:23975.
- Awad AS, El-Sharif AA. Curcumin immune-mediated and anti-apoptotic mechanisms protect against renal ischemia/reperfusion and distant organ induced injuries. Int Immunopharmacol. 2011;11:992–996.
- He L, Peng X, Zhu J, et al. Protective effects of curcumin on acute gentamicin-induced nephrotoxicity in rats. Can J Physiol Pharmacol. 2015;93:275–282.
- İlbey YO, Ozbek E, Cekmen M, Simsek A, Otunctemur A, Somay A. Protective effect of curcumin in cisplatin-induced oxidative injury in rat testis: Mitogen-activated protein kinase and nuclear factor-kappa B signaling pathways. Hum Reprod. 2009;24:1717–1725.
- Kuhad A, Pilkhwal S, Sharma S, Tirkey N, Chopra K. Effect of curcumin on inflammation and oxidative stress in cisplatin-induced experimental nephrotoxicity. J Agric Food Chem. 2007;55:10150–10155.
- Rogers NM, Stephenson MD, Kitching AR, Horowitz JD, Coates PT. Amelioration of renal ischemia-reperfusion injury by liposomal delivery of curcumin to renal tubular epithelial and antigen-presenting cells. Br J Pharmacol. 2012;166:194–209.
- Soetikno V, Sari FR, Veeraveedu PT, et al. Curcumin ameliorates macrophage infiltration by inhibiting NF-κB activation and proinflammatory cytokines in streptozotocin induced-diabetic nephropathy. Nutr Metab (Lond). 2011;8:35.
- Ueki M, Ueno M, Morishita J, Maekawa N. Curcumin ameliorates cisplatin-induced nephrotoxicity by inhibiting renal inflammation in mice. J Biosci Bioeng. 2013;115:547–551.
- Ugur S, Ulu R, Dogukan A, et al. The renoprotective effect of curcumin in cisplatin-induced nephrotoxicity. Ren Fail. 2015;37:332–336.
- Sahin K, Orhan C, Tuzcu M, et al. Comparative in vivo evaluations of curcumin and its analog difluorinated curcumin against cisplatin-induced nephrotoxicity. Biol Trace Elem Res. 2014;157:156–163.
- Sahu BD, Rentam KK, Putcha UK, Kuncha M, Vegi GM, Sistla R. Carnosic acid attenuates renal injury in an experimental model of rat cisplatin-induced nephrotoxicity. Food Chem Toxicol. 2011;49:3090–3097.
- Francescato HD, Costa RS, Júnior FB, Coimbra TM. Effect of JNK inhibition on cisplatin-induced renal damage. Nephrol Dial Transplant. 2007;22:2138–2148.
- Jain SK, McVie R, Duett J, Herbst JJ. Erythrocyte membrane lipid peroxidation and glycosylated hemoglobin in diabetes. Diabetes. 1989;38:1539–1543.
- Hartmann JT, Lipp HP. Toxicity of platinum compounds. Expert Opin Pharmacother. 2003;4:889–901.
- Jiang M, Wei Q, Pabla N, et al. Effects of hydroxyl radical scavenging on cisplatin-induced p53 activation, tubular cell apoptosis and nephrotoxicity. Biochem Pharmacol. 2007;73:1499–1510.
- Santos NA, Bezerra CS, Martins NM, Curti C, Bianchi ML, Santos AC. Hydroxyl radical scavenger ameliorates cisplatin-induced nephrotoxicity by preventing oxidative stress, redox state unbalance, impairment of energetic metabolism and apoptosis in rat kidney mitochondria. Cancer Chemother Pharmacol. 2008;61:145–155.
- Barzegar A, Moosavi-Movahedi AA. Intracellular ROS protection efficiency and free radical-scavenging activity of curcumin. PLoS One. 2011;6:e26012.
- Zhang B, Ramesh G, Norbury C, Reeves WB. Cisplatin-induced nephrotoxicity is mediated by tumor necrosis factor-alpha produced by renal parenchymal cells. Kidney Int. 2007;72:37–44.
- Faubel S, Lewis EC, Reznikov L, et al. Cisplatin-induced acute renal failure is associated with an increase in the cytokines interleukin (IL)-1beta, IL-18, IL-6, and neutrophil infiltration in the kidney. J Pharmacol Exp Ther. 2007;322:8–15.
- Razzaque MS, Koji T, Kumatori A, Taguchi T. Cisplatin-induced apoptosis in human proximal tubular epithelial cells is associated with the activation of the Fas/Fas ligand system. Histochem Cell Biol. 1999;111:359–365.
- Tsuruya K, Tokumoto M, Ninomiya T, et al. Antioxidant ameliorates cisplatin-induced renal tubular cell death through inhibition of death receptor-mediated pathways. Am J Physiol Renal Physiol. 2003;285:F208–F218.
- Linkermann A, Himmerkus N, Rölver L, et al. Renal tubular Fas ligand mediates fratricide in cisplatin-induced acute kidney failure. Kidney Int. 2011;79:169–178.
- Ozkok A, Edelstein CL. Pathophysiology of cisplatin-induced acute kidney injury. Biomed Res. 2014;2014:967826.
- Seth R, Yang C, Kaushal V, Shah SV, Kaushal GP. p53-dependent caspase-2 activation in mitochondrial release of apoptosis-inducing factor and its role in renal tubular epithelial cell injury. J Biol Chem. 2005; 280:31230–31239.
- Shiloh Y. ATM and related protein kinases: Safeguarding genome integrity. Nat Rev Cancer. 2003;3:155–168.
- Vaziri H, Dessain SK, Ng Eaton E, et al. hSIR2 (SIRT1) functions as an NAD-dependent p53 deacetylase. Cell. 2001;107:149–159.
- Jeong J, Juhn K, Lee H, et al. SIRT1 promotes DNA repair activity and deacetylation of Ku70. Exp Mol Med. 2007;39:8–13.
- Yeung F, Hoberg JE, Ramsey CS, et al. Modulation of NF-kappaB-dependent transcription and cell survival by the SIRT1 deacetylase. EMBO J. 2004;23:2369–2380.