Abstract
Objective: To examine the effect of an aqueous extract of Radix Paeoniae Alba (RPA) on the formation of calcium oxalate (CaOx) stones and the potential mechanism underlying the effect.
Materials and methods: An in vitro assay was used to determine whether the RPA extract prevents the formation of CaOx or promotes CaOx dissolution. We also investigated the efficacy of the extract in vivo as a preventive and therapeutic agent for experimentally induced CaOx nephrolithiasis in rats. Various biochemical, molecular, and histological parameters were assessed in kidney tissue and urine at the end of the in vivo experiment.
Results: Significant dissolution of formed crystals (8.99 ± 1.43) and inhibition of crystal formation (2.55 ± 0.21) were observed in vitro after treatment with 64 mg/mL of the RPA extract compared with a control treatment (55.10 ± 4.98 and 54.57 ± 5.84, respectively) (p < .05). In preventive protocols, the RPA extract significantly reduced urinary and renal oxalate levels and increased urinary calcium and citrate levels compared to the control. In addition, the RPA preventive protocol significantly decreased osteopontin expression, renal crystallization, and pathological changes compared to the control. These changes were not observed in rats on the therapeutic protocol.
Conclusions: RPA is a useful agent that prevents the formation of CaOx kidney stones.
Introduction
Nephrolithiasis is the third most prevalent disorder affecting the urinary system, and it arises as a result of a cascade of events initiated by supersaturation and followed by crystal nucleation, growth, aggregation, retention, and migration to renal papillary surfaces.Citation1 Urinary tract stone formation affects people worldwide, sparing no geographical, cultural or racial groups. Almost 80% of all urinary tract stones are predominately composed of calcium oxalate (CaOx).Citation2 The high recurrence rate of CaOx stones is worrying, and long-term treatment strategies have been inadequate.Citation3 Although nephrolithiasis has been recognized since ancient times, the mechanism of renal stone formation remains unclear, and there are few available agents that effectively prevent this process. Minimally invasive management techniques, such as percutaneous nephrolithotomy, have been developed over the past two decades, but they can cause serious side effects, such as hemorrhage, infection, and renal fibrosis. Therefore, there is an urgent need to identify therapeutics that effectively prevents crystal deposition and development.
Medicinal plants hold an important role in various ancient and modern medication systems. Even today, the extraction of active agents from plants is economical, and generally the side effects associated with extracted agents are mild. Thus, reagents derived from medicinal plants offer treatment options that may be beneficial for the majority of the world’s population. Pharmacological investigations into the use of medicinal plants for traditional antinephrolithic therapy have revealed therapeutic potential in both in vitro and in vivo models.Citation4–6
Radix Paeoniae Alba (RPA), the dried root of Paeonia lactiflora Pall without bark, has been used as a medicinal herb in traditional Chinese medicine for centuries based on its wide range of pharmacological activities. In ancient pharmacology, RPA was used to calm liver wind, relieve pain, nourish blood, regulate menstrual functions, and suppress sweating.Citation7 In modern pharmacology, RPA decoctions are used to treat rheumatoid arthritis, systemic lupus erythematosus, hepatitis, dysmenorrhea, muscle cramping and spasms, and long-standing fever.Citation8,Citation9 However, no scientific data are available to establish the beneficial effect of this plant on nephrolithiasis. In this study, we investigated the antinephrolithic effect of an RPA extract on CaOx nephrolithiasis and the possible mechanisms underlying this effect using different models.
Materials and methods
Plant materials and extraction
Because traditional Chinese medicine reagents are typically used after a long decoction, our study used this method to obtain aqueous extracts for further assessments.Citation10 Methods for extracting active ingredients from plants have been previously described. For the herbs used in this study, 1 kg of raw material was immersed in 5 L of distilled water for 2 h and decocted for 30 min. The supernatant was collected by filtration. Subsequently, the residue was mixed with 3 L of distilled water and boiled again. Finally, two aliquots of aqueous extracts were combined, vacuum filtered, concentrated, and lyophilized. The freeze-dried powder (52 g) was stored in a freezer at −80 °C.
Determination of the extract’s effect on CaOx crystallization
Calcium oxalate monohydrate crystallization was promoted by mixing calcium chloride (8 mmol/L) and sodium oxalate (1 mmol/L); the final solution contained 200 mmol/L sodium chloride and 10 mmol/L sodium acetate. These concentrations were chosen because they are close to the physiological concentrations of these compounds in urine. The pH was adjusted to 5.7 to mimic the typical pH of first morning urine samples collected from people who tend to form calcium stones.Citation11 Two tests were performed. In the first test (crystal formation inhibition test), the RPA powder was mixed with crystallization reagents to obtain a RPA concentration of 0–64 mg/mL before crystallization (0 h). As a control, at time 0 h, an equivalent volume of distilled water was mixed with the crystallization reagents. All samples were incubated with agitation at 500 rpm at 37 °C for 24 h. In the second test (dissolution test), after crystal formation was induced for 24 h, the RPA extracts were mixed to obtain a concentration of 0–64 mg/mL. An equivalent volume of distilled water was mixed with the formed crystals as a control. An inverted microscope (400×; Nikon Corporation, Tokyo, Japan) was used to observe crystal morphology and numbers. At 24 h after the addition of the RPA extract or distilled water, five randomly selected fields were observed. For each concentration of extract examined, independent tests were performed in triplicate.
In vivo studies
The Animal Research Committee of Lanzhou University approved all animal experimental protocols, and the procedures were performed in accordance with ethical guidelines for animal care. Male Sprague–Dawley rats (n = 60; aged 7 weeks; weight 180–200 g) were acclimated to room temperature for more than 4 weeks; the rats were fed a standard commercial rat chow during the study. After the acclimation period, the rats were randomly divided into six equal groups. Group A (control group) received distilled water instead of tap water. Groups B-F received 1% v/v ethylene glycol in distilled water for 28 days. Group B served as the control nephrolithic group. Animals in groups C and D were treated orally for 28 days with 220 mg/kg or 660 mg/kg RPA extract in distilled water, respectively, and served as the prophylactic groups. Groups E and F did not receive the RPA extract for the first 14 days and were treated orally with 220 mg/kg or 660 mg/kg RPA extract in distilled water from day 15 to 28; these served as the treatment groups.
Analysis of urine samples
All the animals were housed in individual metabolic cages, and a 24-h urine sample was collected on day 28. The 24-h urine sample was either analyzed immediately or stored at -80 °C. Urinary calcium and magnesium levels were measured using an automated analyzer (model 705, SRL, Tokyo, Japan). Urinary oxalate was analyzed by direct precipitation followed by titration, as previously described.Citation12 Urine citrate was measured with a Citric Acid Enzyme Bio-analysis Kit (Megazyme, Wicklow, Ireland).
Kidney sample collection and kidney homogenate analysis
The animals were sacrificed under anesthesia; both kidneys were removed and washed with cold 0.9% NaCl. The left kidney was cut in half; one half was stored at −80 °C for Western blot analysis, and the other half was fixed in 10% formalin, embedded in paraffin, cut into 4-μm-thick sections, and stained for histopathology (hematoxylin and eosin) or immunohistochemistry. The right kidney was minced with scissors and then homogenizedin 0.9% NaCl using a glass homogenizer. The homogenate was centrifuged at 2000 rpm for 10 min in a refrigerated centrifuge to remove cell debris. Tissue calcium and oxalate levels were determined in the supernatant using commercially available kits (Biovision Ltd, Milpitas, CA and Instruchemie Ltd, Netherlands).
Renal crystal deposition
Renal crystal deposits were assessed as follows: no deposits = zero points; crystal deposits in the papillary tip = one point; crystal deposits in the cortico-medullary junction = two points; and crystal deposits in the cortex = three points. If the crystals were found in multiple regions, the points were added together for a total score.Citation13
Kidney pathological examination
Pathological changes were semi-quantified using the following scoring system: zero = invisible lesions; one = renal interstitium inflammatory infiltration with lesion area <20% and mild tubular dilation; two = renal interstitium inflammatory infiltration with lesion area <40% and obvious tubular dilation; and three = renal interstitium inflammatory infiltration with lesion area >40% and severe tubular dilation.Citation14
Microbiological studies
At the end of the experiment, urine aspirated from the bladder was used to assess microbiological variation. The kidneys were aseptically removed and transected. Half of one kidney was individually homogenized in sterile normal saline solution (5 mL). Before the tissue homogenates were cultured on plates, the homogenates were diluted (10−1, 10−3, and 10−5), and bacteria were enumerated after correcting for the dilution factor. Standard microbiological techniques were used. Urinary infection was defined as more than 105 colony-forming units per mL (cfu/mL) in urine. The presence of ≥105 cfu/g renal tissue indicated pyelonephritis.Citation15
Immunohistochemistry
Osteopontin (OPN) immunohistochemistry was performed using the streptavidin–biotin peroxidase complex technique and a goat polyclonal antibody against OPN (ab36125, Abcam Inc., Boston, MA). After deparaffinization and dehydration, formalin-fixed, paraffin-embedded sections were incubated in 3% H2O2 in distilled water for 30 min at room temperature, and antigen retrieval was performed by boiling the slides in 0.01 M citrate buffer for 20 min. The sections were washed in 50 mM Tris–HCl containing 0.05% Tween, pH 7.6, for 2 min. To block non-specific binding, all sections were treated with 5% skim milk for 30 min at room temperature. The slides were then incubated with the primary antibody (1 μg/mL for the OPN antibody) overnight at 4 °C. The reaction was stopped by rinsing the sections with 0.01 M phosphate-buffered saline (PBS). The slides were then incubated with biotinylated anti-mouse/rabbit IgG serum (secondary antibody), treated with a peroxidase-labeled streptavidin–biotin complex, washed with PBS and stained with DAB.
Western blotting analysis
Kidney tissues were homogenized in lysis buffer. After measuring protein concentration using a bicinchoninic acid (BCA) protein assay kit (Beyotime Institute of Biotechnology, Haimen, China), proteins in the homogenates were separated by sodium dodecyl sulfate (SDS)-polyacrylamide gelelectrophoresis (PAGE) and transferred onto a polyvinylidene fluoride (PVDF) membrane. After blocking in 5% non-fat milk, the membranes were incubated with anti-GAPDH and anti-OPN (1:1000; Wanleibio) antibodies at 4 °C overnight. After a washing step, the membranes were incubated with horseradish peroxidase-conjugated secondary antibody (1:5000; Beyotime) for 1 h at room temperature. Enhanced electrochemiluminescence reagent (Wanleibio) was added before the X-ray film was exposed. The protein levels were semi-quantified by gray-scale analysis using a Gel-Pro Analyzer (Media Cybernetics, Bethesda, MD). All target protein values were normalized to GAPDH values.
Statistical analysis
The data are presented as the mean ± standard error and were analyzed using one-way analysis of variance followed by Tukey’s test. A p value <.05 was considered to indicate significance.
Results
Effect of the extract on CaOx crystallization
The crystal dissolution test revealed abundant crystals, including claviform CaOx (black arrow), prismatic CaOx (red arrow) and quadrate CaOx (blue arrow) crystals, in the control group (0 mg/mL) (). The addition of 64.0 mg/mL RPA extract to the formed crystals for 24 h significantly decreased the number of crystals (), and the extract also caused the CaOx crystals to adopt a more claviform shape (). In addition, the extract had a dose-dependent effect on the number of crystals observed using an inverted microscope (400×) ().
Figure 1. CaOx crystal morphology and number (400×). (A) Crystal formation in the control group during the crystal dissolution test. (B) RPA extract (64.0 mg/mL) was added to the formed CaOx crystals for 24 h. (C) Number of crystals in the two tests.
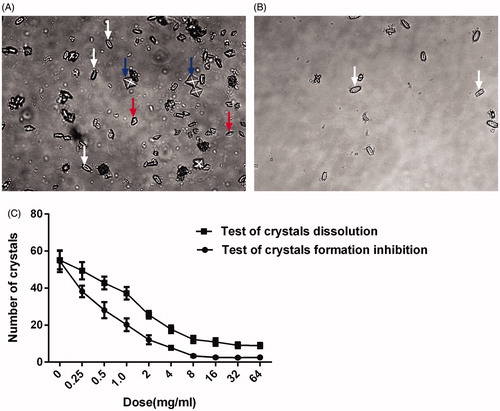
Crystal number and morphology were similar in the control groups in the two tests. Crystal formation was significantly reduced 24 h after the addition of 64.0 mg/mL RPA extract to the crystallization reagents at time 0 h ().
Biochemical changes in urine and kidney tissue
As shown in , urinary and renal oxalate levels were significantly increased (p < .05) in group B compared with group A. Urinary calcium was significantly lower in group B compared with group A (p < .05), and urinary citrate levels were also lower in group B compared with group A. In groups E and F, the RPA extract significantly reduced urinary and renal oxalate values (p < .05) and significantly increased urinary calcium and citrate. However, the above variables were not significantly improved in groups C and D compared with group B (p > .05).
Table 1. Biochemical variables in urine and kidney tissue (x ± s).
Renal crystal deposits
As shown in , massive CaOx crystals were observed in the renal tubular lumen of the animals in group B, especially in the proximal convoluted tubule (green arrows) (). The scores were not significantly lower in groups C and D compared with group B () (p > .05). There were fewer crystal deposits in groups E and F compared with group B () (p < .05). However, no significant differences were found between groups E and F () (p > .05).
Figure 2. Histopathology and crystal deposition in the rat kidney. (A) Gross anatomy of the kidney (left to right: groups A, B, D and F). (B) Micrograph of HE-stained renal tissue. (C) Pathological alterations and CaOx-deposition scores. Green arrows indicate renal crystals; red arrows indicate inflammatory infiltration. The data are presented as the mean ± SD. *p < .05 compared with group A; #p < .05 compared with group B; and $p < .05 compared with group E.
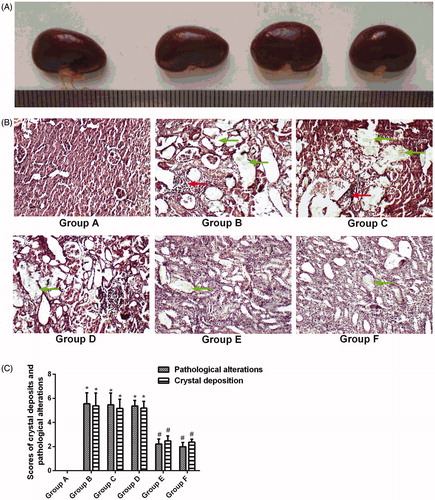
Histopathological examination
shows that pathological changes, such as renal swelling, were not found in the kidneys of the animals in group B, indicating that inducing stones with 1.0% ethylene glycol throughout the entire 4-week experimental period did not lead to gross renal alterations. The gross alterations observed in the kidneys were similar in all groups.
The renal tissue from the animals in group B was severely damaged. Indeed, glomerular degeneration, inflammatory infiltration (red arrows), and enlarged renal tubules with amorphous deposits in the lumen () were observed. Improvements were noted in the renal tissues from groups E and F; the pathological alterations in the animals in these groups included slight inflammatory infiltration and mild tubule dilation (), and the pathological scores were lower for these groups than for group B (p < .05). However, no significant differences were observed between groups E and F () (p > .05). The pathological scores were significantly higher for groups C and D than for group A (p < .05) ().
Microbiological studies
As shown in , no rats from group A (0%) developed a urinary tract infection, whereas the urinary tract infection rate was 100% (10 of 10) in group B. After preventive treatment with the RPA extract, the urinary tract infection rate was 20% (2 of 10) in group E and 10% (1of 10) in group F (p < .05 compared with group B). However, the urinary tract infection rates were 80% (8 of 10) and 90% (9 of 10) in groups C and D, respectively (p > .05 compared with group B). The pyelonephritis rates of groups A, B, E, F, C, and D (0%, 100%, 20%, 10%, 80%, and 90%, respectively) were consistent with the urinary tract infection rates.
Immunohistochemical analysis of the kidneys
Immunostaining was used to evaluate OPN production in renal cells in response to hyperoxaluria and CaOx crystal deposition. OPN expression was almost non-existent in kidneys from normal rats (), but occasional staining was clearly evident in the peritubular region (). In contrast, kidneys from the rats in group B showed marked staining (), with the most noticeable staining on expansive renal tubular epithelial cells (). The increased OPN staining in the renal tubules of groups C and D () was similar to that of group B. However, limited OPN staining was observed in groups E and F ().
Quantification of renal OPN protein expression by western blotting
OPN protein expression in the kidney was quantitatively analyzed by Western blotting, which revealed a specific band corresponding to a molecular weight of 41 kDa (. Densitometry of this band confirmed an increase in OPN protein expression in group B compared with group A (p < .05). This increase was significantly suppressed by preventive treatment with RPA (groups E and F) (p < .05 versus group B). However, OPN expression levels were similar between the therapeutic groups (C and D) and group B.
Figure 5. Western blots for OPN expression. (A) Renal OPN expression was analyzed by Western blotting. (B) Quantitative densitometric analysis of OPN expression. The data are presented as the mean ± SD of three experiments (*p < .05 compared with group A, #p < .05 compared with group B, and $p < .05 for group F compared with group E).
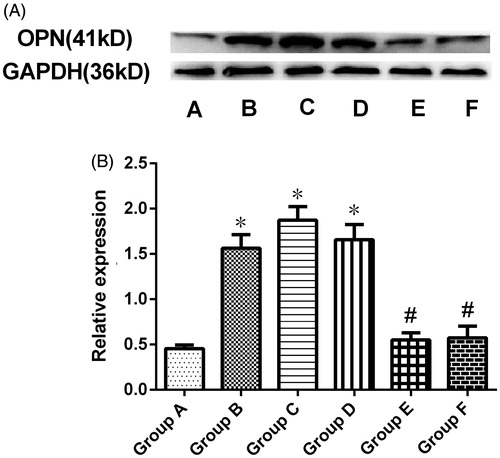
Discussion
In recent years, various herbal and synthetic medicines have been produced to reduce nephrolithiasis in preventative or treatment settings, but no medication is yet available that completely treats or prevents urinary stones; therefore, some patients require surgical intervention due to large stones that cannot pass. Medications can minimize stone size, break stones apart, or dilate the lumen to better enable stone passage. In this study, we investigated the protective and curative effects of RPA on ethylene glycol-induced nephrolithiasis in rats. We particularly focused on phytotherapy, which is common in traditional medicine and serves as an alternative to primary healthcare.
To investigate the medicinal value of RPA for treatment of nephrolithiasis, we evaluated the antiurolithic activity of a crude extract of RPA using different in vitro assays as well as in vivo using a rat model of nephrolithiasis.
In previous studies, Nishihata et al.Citation16 reported that the Kampo extracts Takusha and Sanshishi showed obvious inhibitory effects on CaOx aggregation (64.2 and 84.5%, respectively) and on crystal adhesion to Madin-Darby canine kidney cells (54.6 and 88.2%, respectively). However, in our in vitro assays, RPA had a dose-dependent effect on formed crystals. Our crystal dissolution data suggest that the potential activity of RPA as a nephrolithic inhibitor is related to its complexing of Ca2+ in CaOx embryos, leading to CaOx dissolution. We are unaware of any previous research indicating that components found in RPA extracts could dissolve CaOx. However, it must be recognized that the ionizing ability of RPA in buffer below pH 5.7 may be what enables RPA to interfere with the precipitation and growth of CaOx crystals. Among the various risk factors for kidney stone disease, crystalluria is important for determining the severity and likelihood of disease recurrence. If repeatedly observed in early morning urine samples, crystalluria can predict the risk of stone recurrence in people who tend to form calcium stones.Citation17 The RPA extract used here both decreased crystal size and caused CaOx crystals to adopt a more claviform shape; such crystals are less likely to adhere to renal epithelial cells than quadrate crystals.Citation18 Crystal polymorphism plays a significant role in CaOx kidney stones. Quadrate crystals are more stable and more frequently observed in kidney stones than claviform crystals. Macromolecules isolated from the urine of healthy persons inhibit quadrate crystal formation and promote the formation of claviform crystals, which do not readily attach to kidney epithelial cells.Citation19 This suggests that the formation of claviform crystals protects against kidney stones due to the weak capacity of these crystals to form stable aggregates and adhere to renal epithelial cells, thus potentially inhibiting a key step in stone formation.Citation20 Additionally, in our experiments, RPA not only dissolved formed crystals but also inhibited crystal formation, which is analogous to the inhibitory effect of Takusha and Sanshishi on CaOx crystal formation.Citation16
We used an animal nephrolithiasis model to confirm the in vivo efficacy of RPA against kidney stone formation. Animal nephrolithiasis models have been widely used to study the effects of reagents extracted from plants on crystal formation. Xiang et al.Citation21 found that an N-butanol extract of Urtica dentata hand prevented CaOx deposition and protected renal tissue in a rat nephrolithiasis model. These effects possibly resulted from a combination of the anti-inflammatory and analgesic effects of the extract in combination with its urination-promoting action. However, in the present study, we found that rats with nephrolithiasis showed low urinary calcium and citrate and high urinary and renal oxalate compared to control animals. Oxalate, a far more significant promoter of kidney stones, exhibits 15-fold greater efficacy in the urinary saturation of CaOx compared with calcium.Citation22 Notably, we did not observe elevated urinary calcium in our rat nephrolithiasis model; on the contrary, hypocalciuria was noted. One possible explanation for this finding is that oxalate combines with free calcium, thereby reducing urinary calcium. However, when urinary oxalate was decreased by preventative RPA treatment in groups E and F, the above process was suppressed, and urinary calcium levels partially recovered. However, no changes were observed in renal calcium. In the kidney, calcium mainly localizes within renal cells, and changing urinary calcium ion concentrations is not sufficient to significantly alter calcium ion levels in the kidney. It has been reported that the main risk factor for recurrent CaOx calculus is hypocitraturia.Citation23 Urinary citrate can prevent CaOx crystal formation by inhibiting crystal nucleation and growth.Citation24,Citation25 Our results showed that urinary citrate levels decreased in the rat nephrolithiasis model. The RPA extract increased the urinary citrate concentration and reduced crystal deposition in the preventive groups compared with group B. Therefore, hypercitraturic activity might represent a potential mechanism for the antinephrolithic action of RPA.
Furthermore, emerging evidence indicates that an interaction exists between bacteria and CaOx kidney stones. First, patients with kidney stones are more likely to present with a urinary tract infection than the general population.Citation26,Citation27 Second, in previous studies, bacteria could be cultured from 19 to 32% of CaOx stones, and non-urease-producing Escherichia coli was the most common type of bacteria found.Citation28,Citation29 E. coli, a major member of the Gram-negative bacterial family Enterobacteriaceae, contributes to a wide range of kidney pathologies, from pyelonephritis to kidney allograft rejection.Citation30,Citation31 Chutipongtanate et al.Citation32 indicated that bacteria can directly promote CaOx formation in vitro in a manner comparable to the stone formation promoted by the membranes of fragmented red blood cells.Citation33 Although preventive treatment with the studied RPA extract, which contains antimicrobial compounds, could significantly improve urinary tract infections, which may inhibit crystal formation, the RPA extract did not result in such improvements in the present work.
Kidney stone formation is a multi-faceted process that includes crystal nucleation, growth, and aggregation in the kidneys, in addition to crystal retention therein. These processes are controlled by various factors, including urinary proteins, which are also major constituents in the matrices of kidney stones. OPN, Tamm–Horsfall protein (THP), bikunin, prothrombin fragment-1, α1-microglobulin, inter-α-inhibitor, and matrix Gla protein are some of the major crystallization modulators.Citation34 It has been suggested that OPN expression and production play important roles in crystal deposition in the kidney.Citation35 Studies in rat model shave shown that OPN expression significantly increases with the development of hyperoxaluria, and further increases were observed after CaOx crystal deposition in the kidney,Citation36,Citation37 which is consistent with our results. Surface immobilization of OPN by collagen granules promotes the aggregation and adhesion of CaOx crystals in artificial urine.Citation38 Knocking out the OPN gene led to decreased CaOx crystal deposition in kidneys of hyperoxaluric mice, a model generated via intra-peritoneal administration of glyoxylate.Citation39 In addition, in hyperoxaluric rats, OPN coats epithelial cell surfaces, which may promote crystal attachment.Citation40 Crystal deposition could lead to changes in OPN protein expression, which in turn could promote the formation of urinary calculi, thus creating a vicious cycle. In the present study, we found that preventive treatment with RPA extract significantly decreased OPN expression.
In groups E and F, nephrolithiasis-related indicators were not significantly improved, potentially because the formation of crystals prior today 14 eliminated the targets of RPA. In addition, the number of crystals was not significantly reduced. The etiology of CaOx nephrolithiasis is complex and related to many factors. Improvements in certain indicators may not lead to the minimization or dissolution of formed crystals, as indicated by the observations made on day 14 for groups E and F. Indeed, crystal-related indices did not significantly improve in either of these groups. However, further studies are needed to confirm this hypothesis.
Conclusions
The results of the present study indicate that the use of an aqueous extract of RPA has the potential to prevent kidney stone formation. However, the exact mechanism underlying this protective effect is not completely understood. Further studies should be conducted to better characterize the mechanism of action of RPA in preventing kidney stone formation as well as to evaluate possible toxic effects associated with long-term oral administration of RPA.
Disclosure statement
The authors have no conflicts of interest with respect to this work.
Funding
This study was supported by the Youth science and technology fund projects in gansu province [Grant number 1506RJYA247].
References
- Moe OW. Kidney stones: Pathophysiology and medical management. Lancet. 2006;367:333–344.
- Khan SR. Animal models of kidney stone formation: An analysis. World J Urol. 1997;15:236–243.
- Butterweck V, Khan SR. Herbal medicines in the management of urolithiasis: Alternative or complementary? Planta Med. 2009;75:1095–1103.
- Orhan N, Onaran M, Sen I, Isik Gonul I, Aslan M. Preventive treatment of calcium oxalate crystal deposition with immortal flowers. J Ethnopharmacol. 2015;163:60–67.
- Saha S, Verma RJ. Antinephrolithiatic and antioxidative efficacy of Dolichos biflorus seeds in a lithiasic rat model. Pharm Biol. 2015;53:16–30.
- de Cogain MR, Linnes MP, Lee HJ, et al. Aqueous extract of Costus arabicus inhibits calcium oxalate crystal growth and adhesion to renal epithelial cells. Urolithiasis. 2015;43:119–124.
- Zhang X, Wang Y, Liang Q, et al. The correlation between chemical composition, as determined by UPLC-TOF-MS, and acute toxicity of Veratrum nigrum L. and Radix paeoniae alba. Evid Based Complement Alternat Med. 2014;2014:892797.
- He DY, Dai SM. Anti-inflammatory and immunomodulatory effects of Paeonia lactiflora pall., a traditional chinese herbal medicine. Front Pharmacol. 2011;2:10.
- Feng C, Liu M, Shi X, et al. Pharmacokinetic properties of paeoniflorin, albiflorin and oxypaeoniflorin after oral gavage of extracts of Radix Paeoniae Rubra and Radix Paeoniae Alba in rats. J Ethnopharmacol. 2010;130:407–413.
- Li HB, Jiang Y, Wong CC, Cheng KW, Chen F. Evaluation of two methods for the extraction of antioxidants from medicinal plants. Anal Bioanal Chem. 2007;388:483–488.
- Kulaksizoglu S, Sofikerim M, Cevik C. In vitro effect of lemon and orange juices on calcium oxalate crystallization. Int Urol Nephrol. 2008;40:589–594.
- Archer HE, Dormer AE, Scowen EF, Watts RW. Studies on the urinary excretion of oxalate by normal subjects. Clin Sci (Lond). 1957;16:405–411.
- Yamaguchi S, Wiessner JH, Hasegawa AT, Hung LY, Mandel GS, Mandel NS. Study of a rat model for calcium oxalate crystal formation without severe renal damage in selected conditions. Int J Urol. 2005;12:290–298.
- Li X, Liang Q, Sun Y, et al. Potential mechanisms responsible for the antinephrolithic effects of an aqueous extract of Fructus aurantii. Evid Based Complement Alternat Med. 2015;2015:491409.
- Barros M, Martinelli R, Rocha H. Experimental supratrigonal cystectomy: II-Evaluation of urinary calculi, infection, and bladder dysfunction in the pathogenesis of renal failure. Int Urol Nephrol. 2008;40:329–332.
- Nishihata M, Kohjimoto Y, Hara I. Effect of Kampo extracts on urinary stone formation: An experimental investigation. Int J Urol. 2013;20:1032–1036.
- Daudon M, Hennequin C, Boujelben G, Lacour B, Jungers P. Serial crystalluria determination and the risk of recurrence in calcium stone formers. Kidney Int. 2005;67:1934–1943.
- Wesson JA, Ward MD. Role of crystal surface adhesion in kidney stone disease. Curr Opin Nephrol Hypertens. 2006;15:386–393.
- Khan A, Khan SR, Gilani AH. Studies on the in vitro and in vivo antiurolithic activity of Holarrhena antidysenterica. Urol Res. 2012;40:671–681.
- Sheng X, Ward MD, Wesson JA. Crystal surface adhesion explains the pathological activity of calcium oxalate hydrates in kidney stone formation. J Am Soc Nephrol. 2005;16:1904–1908.
- Xiang M, Zhang S, Lu J, et al. Antilithic effects of extracts from Urtica dentata hand on calcium oxalate urinary stones in rats. J Huazhong Univ Sci Technol Med Sci. 2011;31:673–677.
- Borghi L, Meschi T, Amato F, Briganti A, Novarini A, Giannini A. Urinary volume, water and recurrences in idiopathic calcium nephrolithiasis: A 5-year randomized prospective study. J Urol. 1996;155:839–843.
- Menon M, Mahle CJ. Urinary citrate excretion in patients with renal calculi. J Urol. 1983;129:1158–1160.
- Xu H, Zisman AL, Coe FL, Worcester EM. Kidney stones: An update on current pharmacological management and future directions. Expert Opin Pharmacother. 2013;14:435–447.
- Goldberg H, Grass L, Vogl R, Rapoport A, Oreopoulos DG. Urine citrate and renal stone disease. CMAJ. 1989;141:217–221.
- Hugosson J, Grenabo L, Hedelin H, Lincolon K, Pettersson S. Chronic urinary tract infection and renal stones. Scand J Urol Nephrol. 1989;23:61–66.
- Holmgren K, Danielson BG, Fellstrom B, Ljunghall S, Niklasson F, Wikstrom B. The relation between urinary tract infections and stone composition in renal stone formers. Scand J Urol Nephrol. 1989;23:131–136.
- Wang X, Krambeck AE, Williams Jr JC, et al. Distinguishing characteristics of idiopathic calcium oxalate kidney stone formers with low amounts of Randall's plaque. Clin J Am Soc Nephrol. 2014;9:1757–1763.
- Tavichakorntrakool R, Prasongwattana V, Sungkeeree S, et al. Extensive characterizations of bacteria isolated from catheterized urine and stone matrices in patients with nephrolithiasis. Nephrol Dial Transplant. 2012;27:4125–4130.
- Grover RK, Cheng J, Peng Y, et al. The costimulatory immunogen LPS induces the B-Cell clones that infiltrate transplanted human kidneys. Proc Natl Acad Sci USA. 2012;109:6036–6041.
- Kaijser B, Hanson LA, Jodal U, Lidin-Janson G, Robbins JB. Frequency of E. coli K antigens in urinary-tract infections in children. Lancet. 1977;1:663–666.
- Chutipongtanate S, Sutthimethakorn S, Chiangjong W, Thongboonkerd V. Bacteria can promote calcium oxalate crystal growth and aggregation. J Biol Inorg Chem. 2013;18:299–308.
- Chutipongtanate S, Thongboonkerd V. Red blood cell membrane fragments but not intact red blood cells promote calcium oxalate monohydrate crystal growth and aggregation. J Urol. 2010;184:743–749.
- Khan SR, Kok DJ. Modulators of urinary stone formation. Front Biosci. 2004;9:1450–1482.
- Asselman M, Verhulst A, De Broe ME, Verkoelen CF. Calcium oxalate crystal adherence to hyaluronan-, osteopontin-, and CD44-expressing injured/regenerating tubular epithelial cells in rat kidneys. J Am Soc Nephrol. 2003;14:3155–3166.
- Alex M, Sauganth Paul MV, Abhilash M, Mathews VV, Anilkumar TV, Nair RH. Astaxanthin modulates osteopontin and transforming growth factor beta1 expression levels in a rat model of nephrolithiasis: A comparison with citrate administration. BJU Int. 2014;114:458–466.
- Katsuma S, Shiojima S, Hirasawa A, et al. Global analysis of differentially expressed genes during progression of calcium oxalate nephrolithiasis. Biochem Biophys Res Commun. 2002;296:544–552.
- Umekawa T, Iguchi M, Kurita T. The effect of osteopontin immobilized collagen granules in the seed crystal method. Urol Res. 2001;29:282–286.
- Hamamoto S, Nomura S, Yasui T, et al. Effects of impaired functional domains of osteopontin on renal crystal formation: Analyses of OPN transgenic and OPN knockout mice. J Bone Miner Res. 2010;25:2712–2723.
- Yamate T, Kohri K, Umekawa T, et al. Interaction between osteopontin on Madin Darby canine kidney cell membrane and calcium oxalate crystal. Urol Int. 1999;62:81–86.