Abstract
Objectives
Acute kidney injury (AKI) is a common complication after lung transplantation (LTx) which is closely related to the poor prognosis of patients. We aimed to explore potential risk factors and outcomes associated with early post-operative AKI after LTx.
Methods
A retrospective study was conducted in 136 patients who underwent LTx at our institution from 2017 to 2019. AKI was defined according to the Kidney Disease: Improving Global Outcomes (KDIGO) guideline. Univariate and multivariate analyses were conducted to identify risk factors related to AKI. The primary outcome was the incidence of AKI after LTx. Secondary outcomes were associations between AKI and short-term clinical outcomes and mortality.
Results
Of the 136 patients analyzed, 110 developed AKI (80.9%). AKI was associated with higher baseline eGFR (odds ratio (OR) 1.01 (95% confidence interval (CI): 1.00–1.03)) and median tacrolimus (TAC) concentration (OR 1.15 (95% CI: 1.02–1.30)). Patients with AKI suffered longer mechanical ventilation days (p = .015) and ICU stay days (p = .011). AKI stage 2–3 patients had higher risk of 1-year mortality (HR 16.98 (95% CI: 2.25–128.45)) compared with no-AKI and stage 1 patients.
Conclusions
Our results suggested early post-operative AKI may be associated with higher baseline eGFR and TAC concentrations. AKI stage 1 may have no influence on survival rate, whereas AKI stage 2–3 may be associated with increased mortality at 1-year.
Introduction
Lung transplantation (LTx) remains the only choice for patients with end-stage lung disease who wish to improve life quality and prolong life span. However, despite progresses made in surgical techniques and immunosuppressive regimens, patients’ survival rates are still hampered by serious post-operative complications [Citation1]. Acute kidney injury (AKI) is one of the most common complications with high incidence rates ranging from 39% to 69% [Citation2]. It may be linked to other complications, including infection, bronchial dehiscence [Citation3], sepsis [Citation4], fluid overload [Citation5], and possibly lead to increased short-term and long-term mortality [Citation6].
Current knowledge on AKI is limited and sometimes contradictory, especially in the LTx population, regarding epidemiology, risk factors, and its relation with mortality [Citation7]. Researchers have employed different definitions of AKI since 2005 [Citation8], from risk, injury, failure, loss of kidney function and end-stage renal failure (RIFLE) criteria, acute kidney injury network (AKIN ) classification to KDIGO guideline, leading to a wide span of conclusions [Citation9–11]. Potential risk factors ever reported included baseline glomerular filtration rate [Citation12], transplant type [Citation13], male sex [Citation14], cyclosporine use [Citation3], supratherapeutic tacrolimus (TAC) trough, ≥3 nephrotoxic drugs other than TAC and others [Citation7,Citation10]. Among these studies, study population concentrated in patients in the United States and Canada [Citation3,Citation15]. Only one study in Chinese population has been reported in 2014, and its population size was relatively small [Citation16], leaving a major void in this area.
Therefore, our objectives in this retrospective study were: (1) analyzing the incidence and potential risk factors affecting the occurrence of AKI in a single Chinese center and (2) elucidating the relation between AKI and clinical outcomes.
Materials and methods
Study design and population
In this single center, observational, retrospective study, we included all patients who underwent LTx in China-Japan Friendship Hospital during the period between April 2017 and July 2019. The inclusion criteria were: (1) LTx for the first time and (2) age ≥18. The exclusion criteria were: (1) multiple organ transplantation; (2) survived less than seven days after transplantation; and (3) missing data.
The study was approved by the Ethics Committee of China-Japan Friendship Hospital (no. 2019-65-K45). All patients signed informed consent when undergoing LTx, in which they gave permission for use of anonymized medical data by scientists.
Immunosuppressive regimens
Per protocols in our center, for induction therapy, patients received methylprednisolone or in combination with basiliximab. Methylprednisolone was given intraoperatively at a dose of 500–1000 mg. Basiliximab was given both before transplantation and on post-operative day (POD) 4 at a dose of 20 mg.
Maintenance immunosuppressive regimen consists of TAC, mycophenolate mofetil (MMF) and prednisone. TAC was given on POD1. Starting dose was 2 mg, twice daily, except those with exceptionally high or low body weight were given a personalized dose per attending physician’s judgment. Dose adjustments were based on whole-blood TAC trough concentration measurements. The target therapeutic range is 8–12 ng/mL in the first week. The initial dose of MMF was 1000 mg, twice daily and was adjusted according to area under the curve (AUC) measurements. Prednisone was given intravenously at 1 mg/(kg·d) during POD 1–3, and then switched to oral administration at 0.5 mg/(kg·d), and gradually tapered to 5–10 mg daily for long-term maintenance.
AKI definition
AKI was determined and staged according to the ‘Kidney Disease: Improving Global Outcomes’ (KDIGO) Clinical Practice Guideline, based on serum creatinine (SCr) obtained from the transplantation date until seven days after transplantation [Citation17]. According to the guideline, AKI was defined as any of the followings: (1) increase in SCr by ≥26.5 μmol/L within 48 h; (2) increase in SCr to ≥1.5 times baseline in the first seven PODs. AKI stages were determined as: (1) stage 1, SCr increase to 1.5–1.9 times baseline; (2) stage 2, SCr increase to 2.0–2.9 times baseline; (3) stage 3, SCr increase to ≥3.0 times baseline or to ≥353.6 μmol/L or initiation of renal replacement therapy (RRT). Baseline SCr was defined as SCr obtained prior to LTx. Recovery from an AKI episode was defined by return to no-AKI KDIGO class (<1.5 times baseline creatinine) at any time during the initial hospital stay after transplantation.
Data collection
Patients’ electronic medical records were reviewed and data pertinent to AKI were collected. Baseline demographics (age, sex, height, and weight), pre-operative (pulmonary diagnosis, comorbidities, smoking history, baseline ALT, AST, RBC, hemoglobin, hematocrit, glucose, and SCr), intra-operative (transplant type, operation duration, ECMO support, and blood loss volume), and post-operative (ECMO support, TAC concentration, nephrotoxic drugs, mechanical ventilation duration, ICU stay, and hospital stay) data were obtained. eGFR were calculated using the modification of diet in renal disease (MDRD) equation [Citation18].
Potential nephrotoxic drugs involved in patients’ regimen included: vancomycin, piperacillin tazobactam sodium, amikacin, trimethoprim, amphotericin B (inhalation), ganciclovir, acyclovir, rifampicin, and ethambutol.
Outcome measures
The primary outcome was the incidence of AKI during POD1–POD7 after LTx. Secondary outcomes were short-term outcomes, including mechanical ventilation days, ICU stay days, and hospital stay days; and mortality, including 30-day and 1-year mortality. Hospital stay days were counted from transplantation day to first discharge from hospital.
Statistical analysis
Distribution of data was assessed using the Shapiro–Wilk test. Descriptive statistics were expressed as mean ± standard deviation (SD) for normally distributed continuous variables or median (interquartile range, IQR) for non-normally distributed continuous variables. Categorical variables were presented as count (percentage). Differences between groups were analyzed by Student’s t-test or ANOVA for normally distributed continuous variables; Mann–Whitney’s U or the Kruskal–Wallis tests for non-normally distributed continuous variables, as appropriate. Pearson’s chi-square test or Fisher’s exact test was used to compare differences between groups in categorical variables. Potential risk factors with a p< .20 in univariate analysis were incorporated into multivariate logistic regression in a forward: LR stepwise fashion. Collinearity was assessed and model fit was assessed by the Hosmer–Lemeshow goodness-of-fit test. 30-day and 1-year survival rates stratified by AKI stages were analyzed by the Kaplan–Meier analysis and compared using the log-rank test. Associations of AKI stages and 30-day and 1-year mortality were assessed using the Cox proportional hazard models after adjusting for possible confounders, including baseline demographics, intra-operative and post-operative risk factors for AKI. Risk factors with p< .05 were considered statistically significant. Data were processed using Statistical Package for Social Science (SPSS) 19.0 (SPSS Inc., Chicago, IL).
Results
Incidence of AKI
During April 2017 and July 2019, 150 patients received LTx. From this study population, 11 were excluded due to missing data, two were excluded for death within 24 h, and one was excluded due to less than 18 years old, leaving 136 for final analysis. The median (IQR) age was 60 (54–64) years old, and 86.0% were male. The frequency of hypertension, diabetes mellitus, and hyperlipidemia before surgery was 20.6, 31.6, and 7.4%, respectively. The most common pulmonary diagnosis was interstitial lung disease (ILD; 71.3%), followed by chronic obstructive pulmonary disease (COPD; 16.9%), pulmonary hypertension (PH; 7.4%), others including bronchiolitis obliterans and bronchiectasis (3.7%) and cystic fibrosis (CF; 0.7%). The baseline eGFR was 137 ± 40 mL/min/1.73 m2.
Of the 136 patients analyzed, 110 experienced AKI (80.9%) during the first post-operative week, with the highest incidence on POD 1 and POD 2 (33.1%) (). The incidence of AKI on POD 3–POD 7 was 8.8, 2.2, 0.7, 2.2, and 0.7%, respectively. AKI stage 1 occurred in 38 (27.9%), stage 2 in 32 (23.5%), and stage 3 in 40 (29.4%) of patients. Nineteen patients (14.0%) required RRT.
Risk factors of AKI
Baseline demographic characteristics of the patients with AKI were similar to patients without AKI (). Pre-operative eGFR and SCr exhibited slight difference between no-AKI and AKI group; however, the difference was not statistically significant. In univariate analysis, only median TAC concentration was significantly associated with AKI occurrence (). In AKI group, median TAC concentration was 7.6 (4.7–11.0) ng/mL, while in no-AKI group it was 5.0 (2.4–7.8) ng/mL. Median TAC concentrations also showed ascending tendency across AKI stages.
Table 1. Baseline demographic characteristics of LTx patients by AKI status.
Table 2. Intra-operative and post-operative parameters of LTx patients by AKI status.
Five variables, including baseline SCr, eGFR, hemoglobin, glucose, and median Tac concentration were further assessed in multivariate model (). Higher baseline eGFR (odds ratio (OR) 1.01 (95% confidence interval (CI): 1.00–1.03)) and median TAC concentrations (OR 1.15 (95% CI: 1.02–1.30)) post-transplantation were associated with associated with increased risk of AKI.
Table 3. Multivariate analysis for risk factors of AKI.
Short-term outcomes
Of our study population, the median (IQR) mechanical ventilation days was 2 (1–3), median (IQR) ICU stay days was 4 (3–5) and median (IQR) hospital stay days was 44 (30–60). shows the post-operative short-term outcomes in no-AKI patients and AKI patients. AKI patients had longer mechanical ventilation days and ICU stay days compared with no-AKI group. No statistically significant difference was observed in hospital stay days ().
Table 4. Post-operative outcomes by AKI status.
When comparing mechanical ventilation days between different stages, we found AKI stage 3 patients suffered longer duration than no-AKI patients and AKI stage 1 (p = .002 and p = .034, respectively; Bonferroni’s correction). Also, stage 3 patients spent more days in ICU than no-AKI patients (p = .006; Bonferroni’s correction).
Survival outcomes
Thirty-day and 1-year survival rates of the study population after LTx were 87.5 and 82.4%, respectively. The Kaplan–Meier analysis showed 30-day survival rate was 100.0, 100.0, 87.5, and 67.5% in no-AKI, AKI stages 1, 2, and 3, respectively (no-AKI versus AKI stage 2, p = .065; no-AKI versus AKI stage 3, p = .002); and 1-year survival rate was 100.0, 97.4, 84.4, and 55.0%, respectively (no-AKI versus AKI stage 1, p = .408; no-AKI versus AKI stage 2, p = .037; no-AKI versus AKI stage 3, p = 9.7 × 10−5) ().
Figure 2. Kaplan–Meier’s survival curves stratified by AKI stages and no-AKI at (A) 30-day and (B) 1-year.
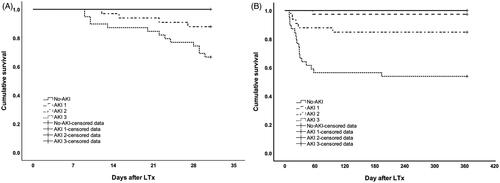
According to Kaplan–Meier’s analysis, no statistically significance in survival rates between no-AKI and AKI stage 1 was observed. Hence, we combined no-AKI and AKI stage 1 into one group for further analysis. From the Cox proportional hazard model (adjusting for potential confounders, including baseline demographics, intra-operative and post-operative risk factors for AKI), severe AKI (stages 2 and 3) in the first post-operative week was found to be associated to increased risk of 1-year mortality (HR 16.98 (95% CI: 2.25–128.45)), but not 30-day mortality (Tables S1 and S2).
Discussion
We conducted a retrospective study in Chinese LTx patients to elucidate the incidence, risk factors of AKI and its association with clinical outcomes. We drew three major conclusions from this study: (1) AKI has a high occurrence in the first post-operative week, (2) it relates to baseline eGFR and TAC concentration, and (3) it is associated with increased 30-day and 1-year mortality.
The overall incidence of AKI in our study population was 80.9%, which was high in comparison with previous studies [Citation10,Citation14,Citation19]. It may be due to the following reasons: (1) different AKI definitions and (2) population demographics. A meta-analysis in LTx patients summarized data published between 2005 and 2019: the incidence rate of AKI was estimated to be 49% (95% CI: 38.3–59.8%), 55.5% (95% CI: 45.2–65.4%), and 53% (95% CI: 38.2–67.3%) by RIFLE, AKIN, and KDIGO criteria, respectively. KDIGO criteria combines the concept of relative baseline SCr change within seven days in RIFLE criteria and a small increase in SCr within 48 h in AKIN criteria, therefore, it has higher sensitivity and could theoretically recognize more AKI patients [Citation17,Citation20]. The highest incidence rate classified by KDIGO criteria was 68.8%, reported by Fidalgo et al. [Citation3], which was still lower than ours. We analyzed our population demographics, and found the majority of LTx patients were elderly male patients (Figure S1). Bennett et al. reported male gender was related to higher risk of AKI [Citation14], and attributed it partly to the data reported by the International Society for Heart and Lung Transplantation (ISHLT) registry claiming that male gender was associated with worse lung transplant outcomes [Citation21]. Also, he mentioned AKI patients were slightly older than no-AKI patients, which was in accordance with conclusion drew by George et al. [Citation13] and hence the high incidence of AKI may be partly attributed to the characteristic of our population demographics.
One major finding in our study was higher baseline eGFR contributed to AKI after LTx, which was counterintuitive, and contradicted by many previous studies [Citation8,Citation12,Citation22]. Similar results reported were explained by a higher proportion of cystic fibrosis patients [Citation8,Citation23]; however, since only one case of cystic fibrosis was found in our study, this explanation does not seem to work for us. Study by Navis et al. also showed an inverse relationship between baseline GFR and post-operative renal function at 1 month [Citation24]. He concluded patients with renal impairment before LTx would have less pronounced decline in renal function after surgery. However, most patients in our study (91.2%) had eGFR values over 90 mL/min/1.73 m2, which indicates a normal renal function and hence is not consistent with the reported population setting in Navis’ study.
One possible mechanism behind the link between higher baseline eGFR and AKI is glomerular hyperfiltration (GH). Glomerular hyperfiltration, described as an absolute increase in GFR, occurs in various clinical conditions including diabetes, obesity, pregnancy, and critically ill patients [Citation25,Citation26]. Hyperfiltration state may indicate kidneys have little reserve capacity, thus intolerable to nephrotoxic insults and prone to incidence of AKI. Sandery et al. also demonstrated in their study that the elevated baseline eGFR was a significant risk factor of AKI in children receiving nephrotoxic drugs, and attributed the pathogenesis to GH [Citation27].
TAC concentration was associated with post-operative AKI in our study, and a gradient increase with worsening AKI severity was observed (). The acute nephrotoxicity of TAC may be due to vasoconstriction of glomerular afferent arterioles when concentration is high [Citation28,Citation29]. Till now, only two studies elucidated the relationship between TAC concentration and post-operative AKI in LTx patients [Citation10,Citation30]. Similar to Sikma et al.’s study, our study also found a small proportion of AKI patients were supratherapeutic (7.9, 18.7, and 30.0% for AKI stages 1, 2, and 3, respectively). This conclusion suggests even routine whole-blood concentration measurements of TAC were not supratherapeutic, patients may still face the risk of developing AKI. Sikma et al. proposed using unbound plasma concentration in lieu of whole-blood concentration, which may be a more sensitive marker of nephrotoxicity. However, currently, most centers routinely test whole-blood TAC concentration, either by immunoassays or by LC–MS/MS, to guide dosing [Citation31]. New therapeutic drug monitoring (TDM) approaches focusing on intracellular or tissue TAC concentration maybe a valuable option in predicting patients’ outcomes in the future [Citation31,Citation32].
AKI patients suffered longer postoperative mechanical ventilation duration and longer ICU stay but did not alter hospital stay days in our study. Correlation between mechanical ventilation and AKI has been reported in previous studies [Citation3,Citation16,Citation33]. Since this is a retrospective study, we could not determine the causal relationship between AKI and mechanical ventilation. Koyner and Murray described a ‘deleterious bidirectional relationship’ between AKI and acute lung injury [Citation34]. This pathological lung–kidney crosstalk, including perioperative lung ischemia/reperfusion and ventilator-induced lung injury, could possibly precipitate the onset of AKI after LTx [Citation35]. Also, AKI may aggravate patients’ conditions and lead to weaning failure [Citation36].
Regardless of staging severity, the association between AKI and mortality has been addressed in many studies [Citation8,Citation14,Citation33]. We investigated 30-day and 1-year mortality and found the survival rates were significantly different between AKI and no-AKI patients (Figure S2). But when further stratified by stages, no significant difference was found between AKI stage 1 and no-AKI patients at both 30-day and 1-year (). The 2017 consensus report on acute kidney disease and renal recovery of the Acute Disease Quality Initiative (ADQI) 16 Workgroup summarized that recovery from AKI within 48–72 h after onset is associated with better outcomes than longer durations of AKI [Citation6]. In our study, 52.6% of stage 1 patients (n = 20) recovered from AKI within 72 h (Table S3), whereas 6.2% of stage 2 (n = 2) and no patients from stage 3 were recovered. Hence, this result could partly explain the finding in . Similar to our study, Xue et al. also reported only severe AKI group (AKIN stages 2 and 3) was associated with higher long-term mortality rates [Citation16]. Moreover, Calabrese et al. directly used KDIGO stages 2 and 3 as the definition of AKI in their study [Citation37]. These findings were consistent with our conclusion that AKI stages 2 and 3 were related increased 1-year mortality.
The characteristic of our population demographics made this study unique: (1) we had less cystic fibrosis patients compared with patients from previous studies; (2) our patients were mainly elderly male patients. Meanwhile, several notable limitations exist in our study. First, this is a retrospective study and not all potential risk factors for AKI were collected due to missing data on electronic medical records, including ischemic time, blood transfusions, vasopressors requirement, genotypes, and other factors ever reported. Second, we did not include urine output data in AKI definition or fluid volume data in statistical analysis because we were not able to acquire the data. Fluid status is a significant factor to consider when interpreting AKI incidence and clinical outcomes. To protect allograft function, patients are maintained on negative fluid balance early after LTx, possibly leading to decreased renal perfusion [Citation38]. Meanwhile, fluid overload and oliguria have been reported to be associated with worse clinical outcomes, including longer mechanical ventilation days, ICU stay days, and increased mortality in both no-AKI and AKI patients [Citation5,Citation39]. Hence, the influence of fluid balance following LTx on clinical outcomes needs to be clarified in further investigation. Third, as we mentioned above, our study population were concentrated in male, elderly patients, which may not reflect the reality in female and younger patients. Future studies, especially multi-center, prospective study on large-population are needed to elucidate risk factors pertain to AKI after LTx.
In conclusion, our single-center experience shows that LTx patients have a high incidence of AKI after surgery. Factors including baseline eGFR and TAC concentrations are associated with AKI onset. AKI patients suffered longer duration of mechanical ventilation and ICU stay. Approximately, half patients of AKI stage 1 could recover from AKI and AKI stage 1 did not significantly affect survival rate, whereas AKI stages 2 and 3 are associated with increased mortality. This finding may imply that severe AKI (stages 2 and 3) at early post-operative period identifies higher mortality risk and patients may deserve closer attention.
Abbreviations | ||
ADQI | = | acute disease quality initiative |
AKI | = | acute kidney injury |
AKIN | = | acute kidney injury network |
ALT | = | anine aminotransferase |
AST | = | aspartate aminotransferase |
AUC | = | area under curve |
BMI | = | body mass index |
BSA | = | body surface area |
CF | = | cystic fibrosis |
CI | = | confidence interval |
COPD | = | chronic obstructive disease |
ECMO | = | extracorporeal membrane oxygenation |
eGFR | = | estimated glomerular filtration rate |
GH | = | glomerular hyperfiltration |
ICU | = | intensive care unit |
ILD | = | interstitial lung disease |
IMV | = | invasive mechanical ventilation |
IQR | = | interquartile range |
ISHLT | = | international society for heart and lung transplantation |
KDIGO | = | kidney disease = improving global outcomes |
LTx | = | lung transplantation |
MDRD | = | modification of diet in renal disease |
MMF | = | mycophenolate mofetil |
OR | = | odds ratio |
PH | = | pulmonary hypertension |
POD | = | post-operative day |
RBC | = | red blood cells |
RIFLE | = | risk, injury, failure, loss of kidney function and end-stage renal failure |
RRT | = | renal replacement therapy |
SCr | = | serum creatinine |
SD | = | standard deviation |
TAC | = | tacrolimus |
TDM | = | therapeutic drug monitoring |
Supplemental Material
Download PDF (421.8 KB)Disclosure statement
No potential conflict of interest was reported by the author(s).
References
- Lyu DM, Zamora MR. Medical complications of lung transplantation. Proc Am Thorac Soc. 2009;6(1):101–107.
- Atchade E, Barour S, Tran-Dinh A, et al. Acute kidney injury after lung transplantation: perioperative risk factors and outcome. Transplant Proc. 2020;52(3):967–976.
- Fidalgo P, Ahmed M, Meyer SR, et al. Incidence and outcomes of acute kidney injury following orthotopic lung transplantation: a population-based cohort study. Nephrol Dial Transplant. 2014;29(9):1702–1709.
- Bagshaw SM, George C, Bellomo R, et al. Early acute kidney injury and sepsis: a multicentre evaluation. Crit Care. 2008;12(2):R47.
- Teixeira C, Garzotto F, Piccinni P, et al. Fluid balance and urine volume are independent predictors of mortality in acute kidney injury. Crit Care. 2013;17(1):R14.
- Chawla LS, Bellomo R, Bihorac A, et al. Acute kidney disease and renal recovery: consensus report of the Acute Disease Quality Initiative (ADQI) 16 Workgroup. Nat Rev Nephrol. 2017;13(4):241–257.
- Lertjitbanjong P, Thongprayoon C, Cheungpasitporn W, et al. Acute kidney injury after lung transplantation: a systematic review and meta-analysis. J Clin Med. 2019;8(10):1713–1731.
- Rocha PN, Rocha AT, Palmer SM, et al. Acute renal failure after lung transplantation: incidence, predictors and impact on perioperative morbidity and mortality. Am J Transplant. 2005;5(6):1469–1476.
- Jacques F, El-Hamamsy I, Fortier A, et al. Acute renal failure following lung transplantation: risk factors, mortality, and long-term consequences. Eur J Cardiothorac Surg. 2012;41(1):193–199.
- Sikma MA, Hunault CC, van de Graaf EA, et al. High tacrolimus blood concentrations early after lung transplantation and the risk of kidney injury. Eur J Clin Pharmacol. 2017;73(5):573–580.
- Ri HS, Son HJ, Oh HB, et al. Inhaled nitric oxide therapy was not associated with postoperative acute kidney injury in patients undergoing lung transplantation: a retrospective pilot study. Medicine (Baltimore). 2018;97(22):e10915.
- Grimm JC, Lui C, Kilic A, et al. A risk score to predict acute renal failure in adult patients after lung transplantation. Ann Thorac Surg. 2015;99(1):251–257.
- George TJ, Arnaoutakis GJ, Beaty CA, et al. Acute kidney injury increases mortality after lung transplantation. Ann Thorac Surg. 2012;94(1):185–192.
- Bennett D, Fossi A, Marchetti L, et al. Postoperative acute kidney injury in lung transplant recipients. Interact Cardiovasc Thorac Surg. 2019;28(6):929–935.
- Nguyen AP, Gabriel RA, Golts E, et al. Severity of acute kidney injury in the post-lung transplant patient is associated with higher healthcare resources and cost. J Cardiothorac Vasc Anesth. 2017;31(4):1361–1369.
- Xue J, Wang L, Chen CM, et al. Acute kidney injury influences mortality in lung transplantation. Ren Fail. 2014;36(4):541–545.
- Khwaja A. KDIGO clinical practice guidelines for acute kidney injury. Nephron Clin Pract. 2012;120(4):c179–c184.
- Stevens LA, Coresh J, Greene T, et al. Assessing kidney function-measured and estimated glomerular filtration rate. N Engl J Med. 2006;354(23):2473–2783.
- Balci MK, Vayvada M, Salturk C, et al. Incidence of early acute kidney injury in lung transplant patients: a single-center experience. Transplant Proc. 2017;49(3):593–598.
- Kim JH, Kim YS, Choi MS, et al. Prediction of clinical outcomes after kidney transplantation from deceased donors with acute kidney injury: a comparison of the KDIGO and AKIN criteria. BMC Nephrol. 2017;18(1):39–51.
- Lund LH, Khush KK, Cherikh WS, et al. The registry of the International Society for Heart and Lung Transplantation: thirty-fourth adult heart transplantation report-2017; focus theme: allograft ischemic time. J Heart Lung Transplant. 2017;36(10):1037–1046.
- Banga A, Mohanka M, Mullins J, et al. Characteristics and outcomes among patients with need for early dialysis after lung transplantation surgery. Clin Transplant. 2017;31(11):e13106.
- Ishikawa S, Griesdale DE, Lohser J. Acute kidney injury within 72 hours after lung transplantation: incidence and perioperative risk factors. J Cardiothorac Vasc Anesth. 2014;28(4):931–935.
- Navis G, Broekroelofs J, Mannes GP, et al. Renal hemodynamics after lung transplantation. A prospective study. Transplantation. 1996;61(11):1600–1605.
- Sasson AN, Cherney DZ. Renal hyperfiltration related to diabetes mellitus and obesity in human disease. World J Diabetes. 2012;3(1):1–6.
- De Waele JJ, Dumoulin A, Janssen A, et al. Epidemiology of augmented renal clearance in mixed ICU patients. Minerva Anestesiol. 2015;81:1079–1085.
- Sandery BJ, Erlich JH, Kennedy SE. Acute kidney injury following intravenous acyclovir in children. Arch Dis Child. 2020;105(12):1215–1219.
- Naesens M, Kuypers DRJ, Sarwal M. Calcineurin inhibitor nephrotoxicity. Clin J Am Soc Nephrol. 2009;4(2):481–508.
- Miano TA, Flesch JD, Feng R, et al. Early tacrolimus concentrations after lung transplant are predicted by combined clinical and genetic factors and associated with acute kidney injury. Clin Pharmacol Ther. 2020;107(2):462–470.
- Brunet M, van Gelder T, Åsberg A, et al. Therapeutic drug monitoring of tacrolimus-personalized therapy: second consensus report. Ther Drug Monit. 2019;41(3):261–307.
- Capron A, Lerut J, Latinne D, et al. Correlation of tacrolimus levels in peripheral blood mononuclear cells with histological staging of rejection after liver transplantation: preliminary results of a prospective study. Transpl Int. 2012;25(1):41–47.
- Pensi D, De Nicolò A, Pinon M, et al. An UPLC–MS/MS method coupled with automated on-line SPE for quantification of tacrolimus in peripheral blood mononuclear cells. J Pharm Biomed Anal. 2015;107:512–517.
- Wehbe E, Duncan AE, Dar G, et al. Recovery from AKI and short- and long-term outcomes after lung transplantation. Clin J Am Soc Nephrol. 2013;8(1):19–25.
- Koyner JL, Murray PT. Mechanical ventilation and the kidney. Blood Purif. 2010;29(1):52–68.
- Imai Y, Parodo J, Kajikawa O, et al. Injurious mechanical ventilation and end-organ epithelial cell apoptosis and organ dysfunction in an experimental model of acute respiratory distress syndrome. JAMA. 2003;289(16):2104–2112.
- Fidalgo P, Ahmed M, Meyer SR, et al. Association between transient acute kidney injury and morbidity and mortality after lung transplantation: a retrospective cohort study. J Crit Care. 2014;29(6):1028–1034.
- Calabrese DR, Florez R, Dewey K, et al. Genotypes associated with tacrolimus pharmacokinetics impact clinical outcomes in lung transplant recipients. Clin Transplant. 2018;32(8):e13332.
- Arnaoutakis GJ, George TJ, Robinson CW, et al. Severe acute kidney injury according to the RIFLE (risk, injury, failure, loss, end stage) criteria affects mortality in lung transplantation. J Heart Lung Transplant. 2011;30(10):1161–1168.
- Wiedemann HP, Wheeler AP, Bernard GR, et al. Comparison of two fluid-management strategies in acute lung injury. N Engl J Med. 2006;354:2564–2575.