Abstract
Long non-coding RNAs (LncRNAs) participate in the regulation of chronic kidney disease (CKD), and acute kidney injury (AKI) is identified as an important risk factor for CKD. This study investigated the involvement of a novel LncRNA MALAT1 in regulating lipopolysaccharide (LPS)-induced cell pyroptosis and inflammation in the human renal tubular epithelial HK-2 cells. Here, the HK-2 cells were subjected to LPS (2 μg/mL) treatment to establish cellular AKI models in vitro, and we validated that LPS triggered NLRP3-mediated pyroptotic cell death, promoted cell apoptosis and inflammation-associated cytokines secretion to induce HK-2 cell injury. Then, a novel LncRNA MALAT1/miRNA (miRNA)-135b-5p axis was verified to rescue cell viability in LPS treated HK-2 cells by targeting NLRP3. Mechanistically, miRNA-135b-5p bound to LncRNA MALAT1, and LncRNA MALAT1 positively regulated NLRP3 through acting as RNA sponger for miRNA-135b-5p. Further gain- and loss-of-function experiments evidenced that both LncRNA MALAT1 ablation and miRNA-135b-5p overexpression reversed LPS-induced cell pyroptosis, apoptosis, and inflammation in the HK-2 cells, and the protective effects of LncRNA MALAT1 knock-down on LPS-treated HK-2 cells were abrogated by silencing miRNA-135b-5p. In general, our study firstly investigated the role of the LncRNA MALAT1/ miRNA-135b-5p/NLRP3 signaling cascade in regulating LPS-induced inflammatory death in HK-2 cells.
Introduction
Chronic kidney disease (CKD) damages normal kidney functions and brings huge health burden for human beings worldwide, and about 8–16% patients are annually diagnosed with CKD [Citation1]. Of note, as the results of its complicated pathogenesis, the therapeutic efficacy of the current strategies for CKD treatment is seriously limited [Citation2,Citation3]. In addition, previous literatures identify that acute kidney injury (AKI) is the main risk factor for CKD [Citation4], and transition from AKI to CKD seriously degrades the life quality of human beings [Citation5]. In recent publications, researchers agree that cell pyroptosis-mediated super-inflammation is pivotal for the pathogenesis of AKI-associated CKD [Citation6,Citation7]. Therefore, it is worthwhile to investigate whether elimination of cell pyroptosis and inflammation is effective to cure AKI-associated CKD, and more diagnostic and therapeutic biomarkers need to be verified. Thus, to explore this issue, the human renal tubular epithelial HK-2 cells were subjected to lipopolysaccharide (LPS) treatment to induce cellular AKI models in vitro as previously described [Citation8,Citation9].
Long non-coding RNAs (LncRNAs) are recently identified as key regulators for various diseases, such as cancers [Citation10–12] and liver fibrosis [Citation13,Citation14]. Interestingly, data from Li et al. [Citation15] and Santer et al. [Citation16] evidence that LncRNAs are validated as potential prognostic biomarkers for CKD, but the information regarding to this field is still scarce, which makes it urgent and necessary to explore the relationship between LncRNAs and CKD progression. Interestingly, LPS alters the expression patterns of multiple LncRNAs to facilitate the development of Alzheimer’s disease [Citation17], chondrocytes injury [Citation18], and sepsis [Citation19], and pyroptotic pathways mediated cell death can be regulated by LncRNAs [Citation20–22]. Based on the above information, our preliminary work screens out a novel LncRNA MALAT1 that can be positively regulated by LPS treatment in the HK-2 cells. As previously described, LncRNA MALAT1 positively regulates inflammation [Citation23–25] and previous work suggests that upregulation of LncRNA MALAT1 aggravates NLRP3-mediated pyroptotic cell death in multiple diseases, including diabetic atherosclerosis [Citation26] and diabetic nephropathy [Citation27]. Of note, Song et al. [Citation21] and Liu et al. [Citation28] evidence that silencing of LncRNA MALAT1 is effective to protect against high-glucose-induced endothelial and epithelial cell pyroptosis.
MicroRNAs (miRNAs) function as ‘bridge’ to combine LncRNAs and mRNA [Citation29,Citation30], and various LncRNAs-miRNAs-mRNA competing for endogenous RNA (ceRNA) networks are identified to be closely associated with CKD pathogenesis [Citation8,Citation31]. In addition, LncRNA MALAT1 exerts its inflammation-promoting effects through modulating miR-181c-5p [Citation32], miR-26b [Citation33], and miR-590 [Citation34], and Dai et al. notice that knock-down of LncRNA MALAT1 releases miR-146a to ameliorate LPS-induced acute lung injury [Citation35], and LncRNA MALAT1 regulates LPS-induced cytokines secretions in human gingival fibroblasts by sponging miR-20a [Citation36]. Among all the miRNAs, miRNA-135b-5p targets both LncRNA MALAT1 [Citation37] and 3′ untranslated regions of NLRP3 mRNA [Citation38,Citation39], and Li et al. evidence that miRNA-135b-5p silences AMPK phosphatase Ppm1e to restrain LPS-mediated production of pro-inflammatory cytokines [Citation40]. In addition, miRNA-135b-5p is reported to be relevant to renal fibrosis [Citation41].
Herein, this study showed that LPS triggered pyroptotic cell death and inflammatory injuries in HK-2 cells in vitro. Moreover, it was revealed that the LncRNA MALAT1/miRNA-135b-5p/NLRP3 signaling cascade could be modulated by LPS treatment, and targeting this pathway was effective to rescue cell viability in the HK-2 cells treated with LPS. Our preliminary data provided new biomarkers for CKD diagnosis and therapy, which also broadened our knowledge in this field.
Materials and methods
Cell culture, treatment, and vectors transfection
Human renal tubular epithelial HK-2 cells were bought from American Type Culture Collection (ATCC, Manassas, VA) and were maintained in the DMEM/F12 medium (Gibco, Waltham, MA) containing 10% FBS (Gibco, Waltham, MA) under the standard culture conditions with 5% CO2 at 37 °C. Based on our preliminary work (data not shown), the HK-2 cells at passage 3–6 were subjected to LPS (2 μg/mL) treatment for 0, 24, and 48 h, respectively. In addition, the gene manipulating vectors for LncRNA MALAT1, and miRNA-135b-5p mimic and inhibitor were constructed by Sangon Biotech (Shanghai, China) according to the previous publications [Citation37–39].
Real-time qPCR
Following the protocols provided by the previous studies [Citation37–39], the real-time qPCR was performed to examine genes expression levels at transcriptional levels. Briefly, total RNA was obtained by TRIzol (Beyotime, Shanghai, China) and analyzed by the following agarose electrophoresis. Next, the total RNA was reversely transcribed, and the quantification of the target genes were measured by using the SYBR Green PCR kit (Qiagen, Hilden, Germany) . The primer sequences could be found in the previous publications [Citation6,Citation7,Citation37–39].
Western blot
RIPA lysis buffer (Beyotime, Shanghai, China) was bought to extract total proteins from the cells, which were further separated by using the 10% SDS-PAGE. Then, according to the molecular weight of the proteins, the target proteins were transferred onto the polyvinylidene fluoride (PVDF, Solarbio, Beijing, China) membranes. The PVDF membranes with transferred proteins were subsequently blocked by 5% blocking buffer and were probed with the primary antibodies against NLRP3 (1:1500, Abcam, Cambridge), ASC (1:2000, Abcam, Cambridge), IL-1β (1:2000, Takara, Berkeley, CA), IL-18 (1:1500, Takara, Berkeley, CA) and GAPDH (1:2000, Abcam, Cambridge) overnight at 4 °C and were incubated with the secondary antibodies for 1 h at room temperature. Finally, the protein bands were visualized by the ChemiDocTM XRS system (Bio-Rad, Hercules, CA) and GAPDH was used as internal control. The Image J software was employed to analyze the gray values of the protein bands.
Cell proliferation and viability
The HK-2 cells were seeded onto the 96-well plates at the density of 5 × 104 cells per well and were subjected to LPS treatment for 0, 24, and 48 h, respectively. The cells were then incubated with the MTT solution (20 μL/well) for 1 h at 37 °C, which were vortexed and a microplate reader (Bio-Rad, Hercules, CA) was purchased to examine the OD values to reflect relative cell proliferation. Moreover, the LPS treated HK-2 cells were stained with trypan blue staining buffer for 20 min at 37 °C, and a microscope (ThermoFisher Scientific, Waltham, MA) was used to observe and count the dead blue cell numbers, which were used for calculating cell viability.
Enzyme-linked immunosorbent assay (ELISA)
The HK-2 cells were subjected to LPS treatment for 48 h, and the supernatants were collected and analyzed by using the commercial ELISA kit (Solarbio, USA) in keeping with their protocols. The relative expressions of the inflammatory cytokines (IL-1β, IL-18, IL-6, and TGF-β) were measured by using a microplate reader (Bio-Rad, Hercules, CA) at the wavelength of 450 nm.
Examination of cell apoptosis
The HK-2 cells were treated with LPS for 48 h, and the cells were stained with the Annexin V-FITC and PI dyes for 40 min at room temperature without light exposure according to the protocols of Cell Apoptosis Detection Kit (Invitrogen, Waltham, MA). Then, a flow cytometer (Becton Dickinson, Franklin Lakes, NJ) was used to examine cell apoptosis ratio, the cells stained with either Annexin V-FITC or PI were regarded as dead cells.
Dual-luciferase reporter gene system assay
The binding sites in LncRNA MALAT1 and miRNA-135b-5p were predicted by using the online starBase software (http://starbase.sysu.edu.cn/), which were validated by the following dual-luciferase reporter gene system assay in keeping with the previous work [Citation8,Citation31]. The wild-type and mutant LncRNA MALAT1 were cloned into luciferase reporters by Sangon Biotech (Shanghai, China), and were co-transfected with the miRNA-135b-5p mimic into the HK-2 cells.
Data analysis
The data in this study were collected and were shown as means ± standard deviation (SD), which were further analyzed by SPSS version 18.0 software (SPSS Inc., Chicago, IL). Specifically, the Student’s t-test was used to compare the means from two groups, and the comparisons among multiple groups were conducted by using the one-way ANOVA analysis. Individual experiment had three times of biological repetitions, and each biological replicate contained three times of technical replicates. *p < .05 was regarded as statistical significance.
Results
LPS treatment significantly influenced cellular functions and promoted inflammatory injury in HK-2 cells
Based on the experimental protocols provided by the previous work [Citation8] and our preliminary experiments (data not shown), the HK-2 cells were exposed to 2 μg/mL of LPS for 0, 24, and 48 h to establish the nephritic injury cell models. As shown in , the MTT assay results showed that LPS suppressed cell proliferation abilities in a time-dependent manner, and consistent with this, the trypan blue staining assay results in supported that HK-2 cell viability were also significantly inhibited by LPS treatment. Next, the HK-2 cells with or without LPS stimulation were stained with Annexin V-FITC and PI, and the following flow cytometer results showed that LPS significantly increased both apoptotic and necroptotic cell ratio in the HK-2 cells (). In addition, by performing Western Blot analysis, we noticed that the protein levels of cell pyroptosis associated biomarkers, including NLRP3, ASC, IL-1β, and IL-18 were apparently upregulated by LPS (), indicating that LPS triggered pyroptotic cell death in HK-2 cells. Moreover, the supernatants of the HK-2 cells were collected, and the Real-Time qPCR results in and the ELISA results in evidenced that LPS promoted pro-inflammatory cytokines (IL-1β, IL-18, IL-6, and TGF-β) generation and secretion in HK-2 cells and its supernatants.
Figure 1. LPS triggered pyroptotic cell death and inflammatory responses in HK-2 cells. (A) Cell proliferation and (B) viability were examined by MTT assay and trypan blue staining assay. (C,D) The HK-2 cells were stained with Annexin V-FITC and PI, and the apoptotic cell ratio was determined by flow cytometer. (E,F) The pyroptosis-associated biomarkers in HK-2 cells were measured by Western Blot analysis. The uncropped WB images are shown in Figure S1. (G) Real-time qPCR and (H) ELISA were performed to evaluate inflammatory cytokines generation and secretion in HK-2 cells and its supernatants. Individual experiment had three times of biological repetitions, and each biological replicate contained three times of technical replicates, and *p < .05.
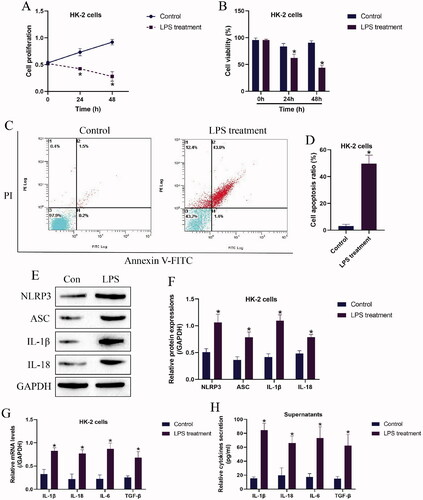
The regulating mechanisms of LncRNA MALAT1, microRNA-135b-5p, and NLRP3 in HK-2 cells
Given that LncRNAs/ miRNAs ceRNA networks are closely associated with CKD pathogenesis and LPS-induced inflammatory injury, which encouraged us to investigate this issue, and here we identified that the expression levels of LncRNA MALAT1 and miRNA-135b-5p could be regulated by LPS treatment (). Specifically, LPS significantly upregulated LncRNA MALAT1 (), while downregulated miRNA-135b-5p in HK-2 cells (). Interestingly, through conducting bioinformatics analysis, we noticed that miRNA-135b-5p potentially bound to LncRNA MALAT1 (), which were supported by the following dual-luciferase reporter gene system assay (). As shown in , miRNA-135b-5p mimic significantly decreased relative luciferase activities in the HEK293T cells co-transfecting with wild-type LncRNA MALAT1. In addition, LncRNA MALAT1 () and miRNA-135b-5p () were respective overexpressed and silenced in HK-2 cells, and we found that knock-down of LncRNA MALAT1 decreased NLRP3 mRNA () and protein () levels in the LPS treated HK-2 cells, which were reversed by silencing miRNA-135b-5p, suggesting that LncRNA MALAT1 exerted its regulating effects on NLRP3 by releasing miRNA-135b-5p.
Figure 2. The regulating effects of LPS treatment on the LncRNA MALAT1/microRNA-135b-5p axis. The expression levels of (A) LncRNA MALAT1 and (B) microRNA-135b-5p were detected by Real-Time qPCR. (C) Prediction and (D) validation of the binding sites in LncRNA MALAT1 and microRNA-135b-5p. (E,F) The LncRNA MALAT1/microRNA-135b-5p axis was manipulated in HK-2 cells, and the vectors transfection efficacy was examined by Real-Time qPCR. (G–I) LPS promoted NLRP3 expressions in HK-2 cells by regulating the LncRNA MALAT1/microRNA-135b-5p axis. The uncropped WB images are shown in Figure S2. Individual experiment had three times of biological repetitions, and each biological replicate contained three times of technical replicates, and *p < .05.
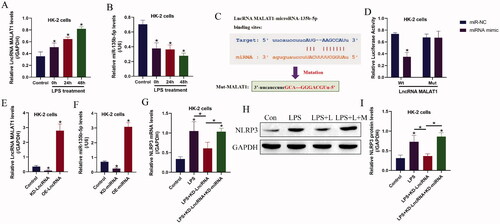
Both LncRNA MALAT1 and microRNA-135b-5p involved in regulating LPS-induced HK-2 cell pyroptosis
Since both LncRNA MALAT1 and miRNA-135b-5p can be modulated by LPS treatment, we conjectured that the above two genes might be closely associated with LPS-induced cell death and inflammation in HK-2 cells. As shown in ,B), LncRNA MALAT1 ablation and miRNA-135b-5p overexpression rescued cell proliferation and viability in HK-2 cells treated with LPS. Then, the following data in ) supported that LPS-induced cell apoptosis in HK-2 cells was also suppressed by silencing LncRNA MALAT1 and upregulating miRNA-135b-5p. In addition, the pyroptosis-associated biomarkers were examined by Western Blot analysis, which showed that the promoting effects of LPS treatment on NLRP3, ASC, IL-1β, and IL-18 were abrogated by both LncRNA MALAT1 ablation and miRNA-135b-5p overexpression (). Consistently, the real-time qPCR results in and the ELISA in suggested that LPS-induced cytokines generation and secretions, including IL-1β, IL-18, IL-6, and TGF-β, were also hampered by silencing LncRNA MALAT1 and upregulating miRNA-135b-5p.
Figure 3. LPS triggered inflammatory cell death in HK-2 cells by modulating LncRNA MALAT1 and microRNA-135b-5p. Examination of (A) cell proliferation and (B) viability by MTT assay and trypan blue staining assay. (C,D) Annexin V-FITC and PI double staining assay was utilized to determine cell apoptosis ratio in HK-2 cells. (E,F) Western Blot analysis was performed to measure the expression levels of NLRP3, ASC, IL-1β, and IL-18 in HK-2 cells. The uncropped WB images are shown in Figure S3. (G) Real-Time qPCR and (H) ELISA were respectively performed to examine production and secretion of the inflammation-associated cytokines in HK-2 cells and its supernatants. Individual experiment had three times of biological repetitions, and each biological replicate contained three times of technical replicates, and *p < .05.
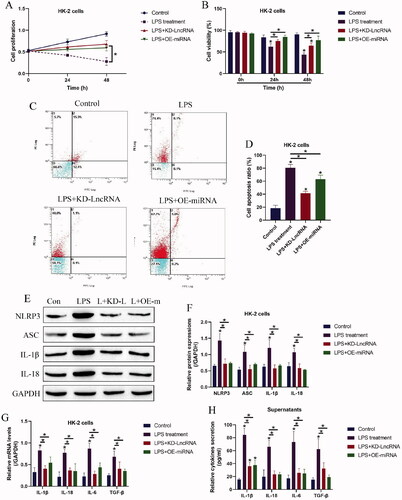
Knock-down of LncRNA MALAT1 reversed LPS-induced cell death in HK-2 cells by releasing microRNA-135b-5p
We next investigated whether targeting LncRNA MALAT1 ameliorated LPS-induced pyroptotic cell death in HK-2 cells by releasing miRNA-135b-5p, and to achieve this, the LncRNA MALAT1 downregulation vectors and miRNA-135b-5p inhibitor were co-transfected into the HK-2 cells (), which were subsequently treated with LPS stimulation. The HK-2 cells were divided into three groups, including control, LncRNA MALAT1 silencing (KD-LncRNA MALAT1), LncRNA MALAT1 silencing plus miRNA-135b-5p knock-down (KD-LncRNA MALAT1 + KD-miRNA), and the functional experiments were performed. As expected, silencing of LncRNA MALAT1 rescued cell proliferation () and viability () in LPS treated HK-2 cells, which were reversed by miRNA-135b-5p inhibitor. Similarly, the Annexin V-FITC- and PI-positive apoptotic cell ratio in the LPS-treated HK-2 cells was decreased by LncRNA MALAT1 ablation, which were also abrogated by miRNA-135b-5p silence (). In addition, the LncRNA MALAT1/ miRNA-135b-5p axis regulated cell pyroptosis and inflammation in a similar manner (). Specifically, the inhibiting effects of LncRNA MALAT1 ablation on NLRP3, ASC, IL-1β, and IL-18 expressions in the LPS-treated HK-2 cells () and the levels of IL-1β, IL-18, IL-6, and TGF-β in HK-2 cells () and its supernatants () were abolished by co-transfecting cells with miRNA-135b-5p inhibitor.
Figure 4. Knock-down of LncRNA MALAT1 attenuated LPS-induced cell pyroptosis in HK-2 cells by releasing microRNA-135b-5p. (A) Cell proliferation and (B) viability were determined by MTT assay and trypan blue staining assay. (C,D) Cell apoptosis ratio in HK-2 cells was examined. (E,F) Western Blot analysis was performed to examine the levels of pyroptosis-associated proteins. The uncropped WB images are shown in Figure S4. (G,H) The levels of inflammation-associated cytokines were determined in HK-2 cells and its supernatants. Individual experiment had three times of biological repetitions, and each biological replicate contained three times of technical replicates, and *p < .05.
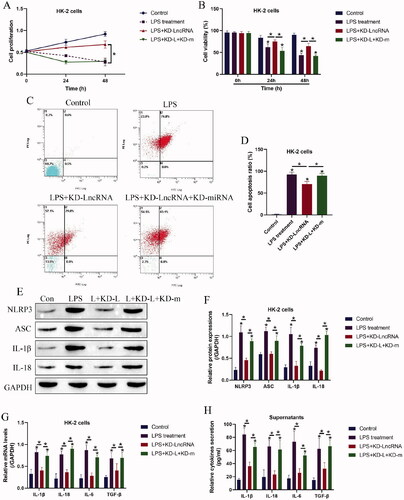
Discussion
Recent publications report that AKI is an important risk factor for CKD [Citation42,Citation43], which makes investigations on AKI-associated CKD necessary and meaningful. However, up until now, the therapeutic efficacy of current treatment strategies for AKI-associated CKD is limited as the results of its unclear pathogenesis mechanisms. Hence, it is urgent to uncover the underlying mechanisms that regulate AKI-associated CKD progression. To achieve this, we established LPS-induced AKI models in the human renal tubular epithelial HK-2 cells according to the previous work [Citation8,Citation9]. As expected, LPS suppressed cell proliferation and viability, while promoted cell apoptosis and pro-inflammation cytokines secretion in HK-2 cells, which were supported by the previous literatures [Citation8,Citation9], implying that LPS brought detrimental effects on HK-2 cells . Moreover, consistent with the existed data that cell pyroptosis occurs during the pathogenesis of CKD [Citation44,Citation45] and LPS treatment [Citation46], we evidenced that LPS also triggered NLRP3-mediated pyroptotic cell death in HK-2 cells, suggesting that cell pyroptosis was closely associated with LPS-induced AKI development.
Based on the existed information that LncRNAs play critical roles in regulating AKI and CKD progression [Citation15,Citation16], and LPS-induced cell pyroptosis can be modulated by various LncRNAs, such as LncRNA CTD-2574D22.4 [Citation18] and lncRNA UCA1 [Citation19], we conjectured that there might exist novel LncRNAs that regulated the progression of AKI associated CKD. Among all the LncRNAs, LncRNA MALAT1 involves in regulating inflammation [Citation23–25] and NLRP3-mediated pyroptotic cell death in multiple diseases, including diabetic atherosclerosis [Citation26], and diabetic nephropathy [Citation27]. Interestingly, data from different teams suggest that targeting LncRNA MALAT1 is a novel strategy to attenuate LPS-induced inflammatory responses and AKI [Citation35], LncRNA MALAT1 regulates high-glucose induced cell pyroptosis in endothelial and epithelial cells [Citation21,Citation28], and LncRNA MALAT1 is closely associated with renal ischemia-reperfusion injury and inflammation [Citation47]. In this study, we verified that LncRNA MALAT1 involved in regulating LPS-induced HK-2 cell pyroptosis. Specifically, the expression levels of LncRNA MALAT1 were upregulated in LPS treated HK-2 cells, and we evidenced that the promoting effects of LPS on cell apoptosis, pyroptosis, and inflammation were reversed by silencing LncRNA MALAT1, which were supported by the previous work [Citation21,Citation28,Citation35], indicating that knock-down of LncRNA MALAT1 attenuated LPS-induced HK-2 injury.
According to the principles of LncRNAs-miRNAs-mRNA ceRNA networks [Citation29,Citation30], LncRNAs exert their biological functions through acting as RNA spongers to absorb and restrain the downstream target miRNAs, causing the alterations of expression patterns of the diseases-associated genes. Given that LncRNA MALAT1 positively regulates NLRP3-mediated cell pyroptosis [Citation21,Citation28], we hypothesized that some miRNAs might bridge LncRNA MALAT1 and NLRP3 mRNA. As expected, we screened out a novel miRNA-135b-5p that potentially targeted both LncRNA MALAT1 [Citation37] and exerted its inhibiting effects on NLRP3 [Citation38,Citation39], and further results evidenced that LncRNA MALAT1 positively regulated NLRP3 expressions by sponging miRNA-135b-5p. Interestingly, miRNA-135b-5p reversed LPS induced inflammatory responses [Citation40], and our data supported that LPS downregulated miRNA-135b-5p, and upregulation of miRNA-135b inhibited NLRP3-mediated cell pyroptosis to recover cellular functions in LPS-treated HK-2 cells. In addition, we evidenced that the protective effects of LncRNA MALAT1 ablation on LPS-treated HK-2 cells were abrogated by silencing miRNA-135b-5p, suggesting that LncRNA MALAT1 regulated LPS-induced CKD progression in a miRNA-135b-5p-dependent manner. However, we only conducted in vitro experiments to investigate the role of the LncRNA MALAT1/ miRNA-135b-5p axis in regulating LPS-induced AKI, which needed to be further validated by the in vivo experiments in our future work.
Conclusions
Collectively, we concluded that knock-down of LncRNA MALAT1 suppressed NLRP3-mediated pyroptotic cell death via releasing miRNA-135b-5p, resulting in the survival of HK-2 cells and targeting the LncRNA MALAT1/ miRNA-135b-5p axis could be used as potential strategy for AKI associated CKD diagnosis and treatment.
Abbreviations | ||
AKI | = | Acute kidney injury |
ceRNA | = | Competing for endogenous RNA |
CKD | = | Chronic kidney disease |
ELISA | = | Enzyme-linked immunosorbent assay |
LncRNAs | = | Long non-coding RNAs |
LPS | = | Lipopolysaccharide |
miRNAs | = | microRNAs |
Supplemental Material
Download JPEG Image (1.6 MB)Supplemental Material
Download JPEG Image (1 MB)Supplemental Material
Download JPEG Image (1.7 MB)Supplemental Material
Download JPEG Image (1.7 MB)Disclosure statement
No potential conflict of interest was reported by the author(s).
Additional information
Funding
References
- Jha V, Garcia-Garcia G, Iseki K, et al. Chronic kidney disease: global dimension and perspectives. Lancet. 2013;382(9888):260–272.
- Shroff GR, Chang TI. Risk stratification and treatment of coronary disease in chronic kidney disease and End-Stage kidney disease. Semin Nephrol. 2018;38(6):582–599.
- Siener R. Dietary treatment of metabolic acidosis in chronic kidney disease. Nutrients. 2018;10(4):512.
- Fiorentino M, Grandaliano G, Gesualdo L, et al. Acute kidney injury to chronic kidney disease transition. Contrib Nephrol. 2018;193:45–54.
- Matsushita K, Saritas T, Eiwaz MB, et al. The acute kidney injury to chronic kidney disease transition in a mouse model of acute cardiorenal syndrome emphasizes the role of inflammation. Kidney Int. 2020;97(1):95–105.
- Chung SD, Lai TY, Chien CT, Yu HJ. Activating nrf-2 signaling depresses unilateral ureteral obstruction-evoked mitochondrial stress-related autophagy, apoptosis and pyroptosis in kidney. PLoS One. 2012;7(10):e47299.
- Xu G, Yue F, Huang H, et al. Defects in MAP1S-mediated autophagy turnover of fibronectin cause renal fibrosis. Aging (Albany NY). 2016;8(5):977–985.
- Deng W, Chen K, Liu S, et al. Silencing circular ANRIL protects HK-2 cells from lipopolysaccharide-induced inflammatory injury through up-regulating microRNA-9. Artif Cells Nanomed Biotechnol. 2019;47(1):3478–3484.
- Liu Y, Bi X, Xiong J, et al. MicroRNA-34a promotes renal fibrosis by downregulation of klotho in tubular epithelial cells. Mol Ther. 2019;27(5):1051–1065.
- Dastmalchi N, Safaralizadeh R, Nargesi MM. LncRNAs: potential novel prognostic and diagnostic biomarkers in colorectal cancer. Curr Med Chem. 2020;27(30):5067–5077.
- Li Y, Jiang T, Zhou W, et al. Pan-cancer characterization of immune-related lncRNAs identifies potential oncogenic biomarkers. Nat Commun. 2020;11(1):1000.
- Tu C, Yang K, Wan L, et al. The crosstalk between lncRNAs and the hippo signalling pathway in cancer progression. Cell Prolif. 2020;53(9):e12887.
- He Z, Yang D, Fan X, et al. The roles and mechanisms of lncRNAs in liver fibrosis. Int J Mol Sci. 2020;21(0 ):1482.
- Unfried JP, Fortes P. LncRNAs in HCV infection and HCV-related liver disease. Int J Mol Sci. 2020;21(6):2255.
- Li N, Cui Y, Yin M, et al. Screening potential prognostic biomarkers of long non-coding RNAs for predicting the risk of chronic kidney disease. Braz J Med Biol Res. 2019;52(11):e8333.
- Santer L, López B, Ravassa S, et al. Circulating long noncoding RNA LIPCAR predicts heart failure outcomes in patients without chronic kidney disease. Hypertension. 2019;73(4):820–828.
- Tang L, Liu L, Li G, et al. Expression profiles of long noncoding RNAs in intranasal LPS-Mediated Alzheimer’s disease model in mice. BioMed Res Int. 2019;2019:1–14.
- Li L, Zhang L, Zhang Y, et al. Inhibition of long non-coding RNA CTD-2574D22.4 alleviates LPS-induced apoptosis and inflammatory injury of chondrocytes. Curr Pharm Des. 2019;25(27):2969–2974.
- Chen Y, Fu Y, Song YF, Li N. Increased expression of lncRNA UCA1 and HULC is required for pro-inflammatory response during LPS induced sepsis in endothelial cells. Front Physiol. 2019;10:608.
- Mao Q, Liang XL, Zhang CL, et al. LncRNA KLF3-AS1 in human mesenchymal stem cell-derived exosomes ameliorates pyroptosis of cardiomyocytes and myocardial infarction through miR-138-5p/Sirt1 axis. Stem Cell Res Ther. 2019;10(1):393.
- Song Y, Yang L, Guo R, et al. Long noncoding RNA MALAT1 promotes high glucose-induced human endothelial cells pyroptosis by affecting NLRP3 expression through competitively binding miR-22. Biochem Biophys Res Commun. 2019;509(2):359–366.
- Zhang Y, Liu X, Bai X, et al. Melatonin prevents endothelial cell pyroptosis via regulation of long noncoding RNA MEG3/miR-223/NLRP3 axis. J Pineal Res. 2018;64(2):e12449.
- Chen H, Wang X, Yan X, et al. RETRACTED: LncRNA MALAT1 regulates sepsis-induced cardiac inflammation and dysfunction via interaction with miR-125b and p38 MAPK/NFκB. Int Immunopharmacol. 2018;55:69–76.
- Huang M, Wang H, Hu X, et al. lncRNA MALAT1 binds chromatin remodeling subunit BRG1 to epigenetically promote inflammation-related hepatocellular carcinoma progression. Oncoimmunology. 2019;8(1):e1518628.
- Wang L, Qi Y, Wang Y, et al. LncRNA MALAT1 suppression protects endothelium against oxLDL-Induced inflammation via inhibiting expression of MiR-181b target gene TOX. Oxid Med Cell Longev. 2019;2019:8245810.
- Han Y, Qiu H, Pei X, et al. Low-dose sinapic acid abates the pyroptosis of macrophages by downregulation of lncRNA-MALAT1 in rats with diabetic atherosclerosis. J Cardiovasc Pharmacol. 2018;71(2):104–112.
- Li X, Zeng L, Cao C, et al. Long noncoding RNA MALAT1 regulates renal tubular epithelial pyroptosis by modulated miR-23c targeting of ELAVL1 in diabetic nephropathy. Exp Cell Res. 2017;350(2):327–335.
- Liu C, Zhuo H, Ye MY, et al. LncRNA MALAT1 promoted high glucose-induced pyroptosis of renal tubular epithelial cell by sponging miR-30c targeting for NLRP3. Kaohsiung J Med Sci. 2020;36(9):682–691.
- Huang Y. The novel regulatory role of lncRNA-miRNA-mRNA axis in cardiovascular diseases. J Cell Mol Med. 2018;22(12):5768–5775.
- Li DS, Ainiwaer JL, Sheyhiding I, et al. Identification of key long non-coding RNAs as competing endogenous RNAs for miRNA-mRNA in lung adenocarcinoma. Eur Rev Med Pharmacol Sci. 2016;20(11):2285–2295.
- Liu P, Zhang B, Chen Z, et al. m6A-induced lncRNA MALAT1 aggravates renal fibrogenesis in obstructive nephropathy through the miR-145/FAK pathway. Aging (Albany NY). 2020;12(6):5280–5299.
- Cao DW, Liu MM, Duan R, et al. The lncRNA Malat1 functions as a ceRNA to contribute to berberine-mediated inhibition of HMGB1 by sponging miR-181c-5p in poststroke inflammation. Acta Pharmacol Sin. 2020;41(1):22–33.
- Ruan Z, Wang S, Yu W, et al. LncRNA MALAT1 aggravates inflammation response through regulating PTGS2 by targeting miR-26b in myocardial ischemia-reperfusion injury. Int J Cardiol. 2019;288:122.
- Zhou Q, Run Q, Li CY, et al. LncRNA MALAT1 promotes STAT3-Mediated endothelial inflammation by counteracting the function of miR-590. Cytogenet Genome Res. 2020;160(10):565–578.
- Dai L, Zhang G, Cheng Z, et al. Knockdown of LncRNA MALAT1 contributes to the suppression of inflammatory responses by up-regulating miR-146a in LPS-induced acute lung injury. Connect Tissue Res. 2018;59(6):581–592.
- Li J, Wang M, Song L, et al. LncRNA MALAT1 regulates inflammatory cytokine production in lipopolysaccharide-stimulated human gingival fibroblasts through sponging miR-20a and activating TLR4 pathway. J Periodontal Res. 2020;55(2):182–190.
- Luo L, Wang Y, Hu P, et al. Long Non-Coding RNA metastasis associated lung adenocarcinoma transcript 1 (MALAT1) promotes hypertension by modulating the hsa-miR-124-3p/nuclear receptor subfamily 3, group C, member 2 (NR3C2) and hsa-miR-135a-5p/NR3C2 axis. Med Sci Monit. 2020;26:e920478.
- Li A, Yu Y, Ding X, et al. MiR-135b protects cardiomyocytes from infarction through restraining the NLRP3/caspase-1/IL-1β pathway. Int J Cardiol. 2020;307:137–145.
- Zeng R, Luo DX, Li HP, et al. MicroRNA-135b alleviates MPP+-mediated Parkinson's disease in in vitro model through suppressing FoxO1-induced NLRP3 inflammasome and pyroptosis . J Clin Neurosci. 2019;65:125–133.
- Li P, Fan JB, Gao Y, et al. miR-135b-5p inhibits LPS-induced TNFα production via silencing AMPK phosphatase Ppm1e. Oncotarget. 2016;7(47):77978–77986.
- Garcia-Vives E, Solé C, Moliné T, et al. The urinary exosomal miRNA expression profile is predictive of clinical response in lupus nephritis. Int J Mol Sci. 2020;21(0 ):1372.
- Abdalrahim MS, Khalil AA, Alramly M, et al. Pre-existing chronic kidney disease and acute kidney injury among critically ill patients. Heart Lung. 2020;49(5):626–629.
- Hsu CY, Chinchilli VM, Coca S, et al. Post-Acute kidney injury proteinuria and subsequent kidney disease progression: the assessment, serial evaluation, and subsequent sequelae in acute kidney injury (ASSESS-AKI) study. JAMA Intern Med. 2020;180(3):402–410.
- Wang M, Yang L, Yang J, et al. Shen Shuai IIRecipe attenuates renal injury and fibrosis in chronic kidney disease by regulating NLRP3 inflammasome and Sirt1/Smad3 deacetylation pathway. BMC Complement Altern Med. 2019;19(1):107.
- Zhang K, Fan C, Cai D, et al. Contribution of TGF-Beta-Mediated NLRP3-HMGB1 activation to tubulointerstitial fibrosis in rat with angiotensin II-Induced chronic kidney disease. Front Cell Dev Biol. 2020;8:1.
- Yang H, Lv H, Li H, et al. Oridonin protects LPS-induced acute lung injury by modulating Nrf2-mediated oxidative stress and Nrf2-independent NLRP3 and NF-κB pathways. Cell Commun Signal. 2019;17(1):62.
- Tian H, Wu M, Zhou P, et al. The long non-coding RNA MALAT1 is increased in renal ischemia-reperfusion injury and inhibits hypoxia-induced inflammation. Ren Fail. 2018;40(1):527–533.