Abstract
Objective
The aim of present report was to elucidate the effect of cell division cycle associated 4 (CDCA4) on the proliferation and apoptosis of Wilm’s tumor cells, and to further evaluate its underlying mechanism.
Methods
The expression profiles of CDCA4 and clinical information of Wilm’s tumor patients were obtained from public Therapeutically Applicable Research to Generate Effective Treatments (TARGET) database portal. Real-time qPCR and western blot analyses were utilized to determine the expression levels of CDCA4. Gain- and loss-of-function of CDCA4 assays were conducted with transfection technology to investigate the biological role of CDCA4 in Wilm’s tumor cells. Cell counting kit 8 and flow cytometer assays were employed to examine the effect of CDCA4 on the cells proliferation and apoptosis. Protein expression levels of indicated markers in each group of Wilm’s tumor cells were measured by western blot.
Results
The transcriptional expression of CDCA4 was drastically upregulated in Wilm’s tumor tissues according to the public TARGET database and in Wilm’s tumor cells. The cells viability was remarkably reduced whereas the cells apoptosis was increased in CDCA4-knockdown group compared with negative control group. However, CDCA4-overexpression group promoted the cells proliferation and suppressed the cells apoptosis. Furthermore, the protein expression levels of p-AKT, p-mTOR, and Cyclin D1 were significantly reduced after depletion of CDCA4, whereas overexpression of CDCA4 dramatically elevated these markers’ expression levels.
Conclusions
CDCA4 is highly expressed in Wilm’s tumor and promoted the proliferation whereas inhibited the apoptosis of Wilm’s tumor cells through activating the AKT/mTOR signaling pathway.
Introduction
Nephroblastoma, also known as Wilm’s tumor, is the most common malignant embryonic tumor of pediatric urinary system derived from renal embryonic cells [Citation1]. It accounts for 95% of kidney tumors in children, with an incidence of about one in 10,000 aged 15 years or younger [Citation2]. Currently, the overall survival rate for patients with localized Wilm’s tumor exceeds 90% at 5 years and 70% for metastatic disease [Citation3]. This is largely due to the significant advances in multidisciplinary approaches to treatment [Citation4]. Particularly, an increasing understanding of Wilm’s tumor biology has lead to the development of biomarkers that have been used to improve risk stratification and increase survival in groups with elevated risk [Citation5]. However, there is still a possibility of malignant metastasis in the late stage of Wilm’s tumor, leading to an unsatisfactory prognosis. In biology, the emergence of new biomarkers can be integrated into the treatment decision-making process [Citation6]. Thus, identifying new biomarkers and introducing new potential molecular targeted therapies may have a positive impact on the outcomes of Wilm’s tumor patients.
Cell division plays a vital role in the life process. Numerous studies have revealed that any malfunction in the process of cell division could lead to malignant tumors [Citation7–9]. Cell division cycle associated protein 4 (CDCA4) is a member of the CDCA family, which is co-expressed with known cell cycle genes including Cyclin, CDC2, CDC7, and others [Citation10]. It has been proven to play a unique role in regulating cell cycle and is associated with transition of G1/S phase [Citation11]. Furthermore, it has been reported that CDCA4 regulates the p53-dependent transcriptional activity and plays a vital role in transcriptional regulation and cell fate determination through JUN oncogene [Citation12]. Therefore, further study of the functions of the members of CDCA family, including CDCA4, and understanding their role in tumorigenesis may provide new ideas for preventing tumor progression. In addition, CDCA4 was reported to enhance proliferation and reduce apoptosis in human breast carcinoma cells [Citation13]. Besides, the expression of CDCA4 was observed to be over-expressed in human ovarian carcinoma tissues by comparison with the non-tumor counterparts [Citation14]. However, its role in Wilm’s tumor is still not fully elucidated.
Based upon the aforementioned information, the current report focused on the investigation of the effects of CDCA4 on the Wilm’s tumor cells viability and apoptosis, as well as on the exploration of the potential mechanism. We found that CDCA4 was over-expressed in Wilm’s tumor and resulted in a poor prognosis. Further functional experiments and mechanistic analysis demonstrated that CDCA4 could promote the Wilm’s tumor cells proliferation and reduced cells apoptosis partly by activating the serine/threonine protein kinase B (AKT)/rapamycin (mTOR) signaling pathway.
Materials and methods
Public data acquisition and analysis
In this study, the RNA sequencing and clinical information of samples were retrieved from the public Therapeutically Applicable Research to Generate Effective Treatments (TARGET) database portal (https://ocg.cancer.gov/programs/target/projects/kidney-tumors). Expression profiles in 126 Wilm’s tumor samples and 6 non-tumor samples were used to analyze CDCA4 expression. CDCA4 expression was divided into high and low expression groups using the median expression value as the boundary. Moreover, using the 121 Wilm’s tumor samples extracted from TARGET database with complete survival status, the relationship between CDCA4 expression and prognosis was analyzed by Kaplan-Meier survival method, following with a log-rank test to compare difference. Gene set enrichment analysis (GSEA) was performed to identify correlated signaling pathways of high and low expression groups.
Cell culture
Human Wilm’s tumor cell line WiT49, was provided by Bluefbio Life Science (Shanghai, China) and normal renal tubular epithelial cell line HK-2 was acquired from the Shanghai Cell Bank of Chinese Academy of Sciences. Human Wilm’s tumor cell line HCM-BROD-0051-C64 (C64) was obtained from ATCC (Manassas, VA, USA). Wilm’s tumor cells were grown in Dulbecco’s modified eagle medium (DMEM), supplied with 15% fetal bovine serum (Procell) and 1% penicillin/streptomycin solution (Procell). HK-2 cells were incubated in Dulbecco’s modified eagle medium (DMEM) containing 10% FBS and 1% penicillin/streptomycin solution. All cells were maintained under a 5% CO2 atmosphere at 37 °C.
Transfection
Cells were incubated in 6-well plates until they reached 80% confluence. Transfection was completed using Lipofectamine 2000 (Invitrogen, Carlsbad, CA, USA) following the manufacturer’s instruction. The sequences of small interfering RNAs (siRNAs) for CDCA4 (si-CDCA4-1, 5′-TAGACCTAAGAGTAAATTA-3′; si-CDCA4-2, 5′-GCUUUCACAAGUCACUUGA-3′) were used to silence CDCA4 and the scrambled sequence (5′-TTCTCCGAACGTGTCACGT-3′) was utilized as negative control (NC). The plasmids pcDNA3.1-CDCA4 and the empty pcDNA3.1 were designed to perform gain-of-function of CDCA4 assays. All the siRNAs and plasmids were designed by GenePharma (Shanghai, China).
Quantitative real-time PCR (qRT-PCR)
Total RNAs from Wilm’s tumor and HK-2 cells were extracted using TRIzol reagent (Invitrogen) following the manufacturer’s protocol. The reverse transcription was carried out by applying TaKaRa reverse transcription kit (TaKaRa, Tokyo, Japan). Real-time PCR was conducted in triplicate using TransStart® Top Green qPCR SuperMix (Transgen, Beijing, China). GAPDH was applied as an internal reference. Relative expression of CDCA4 was normalized to control and calculated using the 2−ΔΔCq method. The primer sequences are listed below:
CDCA4-forward: 5′-ATTTGAAACGCTGGAGACT-3′,
CDCA4-reverse: 5′-CCCATCATGCCTGTCAGTA-3′;
GAPDH-forward: 5′-TGACTTCAACAGCGACACCCA-3′,
GAPDH-reverse:5′-CACCCTGTTGCTGTAGCCAAA-3′.
Western blot assay
Cell lysates were acquired using the radio immunoprecipitation assay lysis buffer (Beyotime, Nantong, China) containing protease inhibitors. The bicinchoninic acid protein assay kit (Beyotime) was utilized to evaluate the protein concentrations. Next, 20 μg per well in the vertical electrophoresis tank was separated using the 10% SDS-PAGE and moved onto the PVDF membrane. Upon being blocked by 5% nonfat dried milk for an hour, these membranes were co-incubated with primary antibodies for 24 h. The primary antibodies include anti-CDCA4 (PA5-43601), anti-AKT (44-621 G), anti-phospho (p)-AKT (OMA1-03061), anti-mTOR (PA5-34663), anti-p-mTOR (44-1125 G), anti-Cyclin D1 (MA5-16356), and anti-GAPDH (TAB1001) which were all obtained from Thermo Fisher Scientific (Shanghai, China). After being washed with Tris buffered saline Tween thrice each 5 min, the membranes were hatched with an anti-rabbit horse radish peroxidase-linked antibody for an hour at room temperature. GAPDH served as an internal reference. The signals were visualized by an enhanced chemiluminescence detection system (Thermo Fisher Scientific) and quantified using the Image J software.
Cell proliferation assay
Cell viability was determined using the cell counting kit 8 (CCK8, Beyotime). After transfection, cells were seeded into a 96-well plate at a density of 1000 cells/100 μl/well and then cultured for 0, 24, 48, and 72 h in a 5% CO2 atmosphere at 37 °C. At the indicated time points, 10 μl CCK8 regent was supplied in each well and incubated for another 90 min. Next, the absorbance values were examined using a microplate reader at 450 nm. Statistics were analyzed to plot the cell proliferation curve.
Cell apoptosis assay
Annexin V/propidium iodide (PI) staining was conducted to examine the cells apoptotic rate using the Annexin V-FITC Apoptosis Detection Kit (Dojindo, Kumamoto, Japan). After transfected with siRNAs or plasmids for 24 h, cells were collected, digested with trypsinization, and rinsed with cold PBS, and then resuspended in 1 × binding buffer to achieve cell density of 1-5 × 106/ml. Next, 100 μl cell suspension was added with 5 μl Annexin V-FITC followed by 10 μl PI solution in dark and incubated for 15 min. Soon 400 μl PBS was added to each sample which was then examined by a FACScan flow cytometer (Becton-Dickinson, San Jose, CA) within 1 h. Data was analyzed using FlowJo software (Ashland, OR, USA).
Statistical analysis
Each assay was conducted in triplicate. The normality of data was verified by Shapiro-Wilk’s test. The data were analyzed using GraphPad Prism 6.0 software (San Diegl, CA, USA) or SPSS 22.0 software (IBM SPSS, Armonk, NY, USA) and presented as mean ± standard deviation. Student t test was utilized to compare the difference between two groups, and comparisons between multiple groups were conducted using one-way analysis of variance with a Tukey’s post-hoc test. p < 0.05 was considered to be statistically significant.
Results
Upregulated CDCA4 is found in Wilm’s tumor and leads to a poor prognosis
To illuminate the expression of CDCA4 in Wilm’s tumor, the transcription levels of CDCA4 were evaluated in 126 Wilm’s tumor tissues and 6 adjacent non-tumor tissues according to the TARGET database. Results shown in revealed that CDCA4 expression was higher in Wilm’s tumor tissues than that in adjacent non-tumor tissues (p < 0.001, ). Then we assessed the relative expression of CDCA4 in Wilm’s tumor WiT49 cells and normal renal tubular epithelial HK-2 cells. Consistently, the expression of CDCA4 was upregulated in Wilm’s tumor cells in comparison with HK-2 cells (p < 0.01, ). Furthermore, the survival curve indicated that high expression of CDCA4 led to a poor prognosis compared with low CDCA4 expression. Together, these findings demonstrated that CDCA4 may play a key role in the progression of Wilm’s tumor.
Figure 1. The expression of CDCA4 in Wilm’s tumor and the relationship with overall survival of patients. (a) CDCA4 transcriptional levels in Wilm’s tumor tissues (n = 6) and adjacent non-tumor tissues (n = 126) were analyzed according to the TARGET database. (b) CDCA4 mRNA level was measured in WiT49 cells and HK-2 cells by qRT-PCR analysis. p < 0.01. (c) Survival curve was plotted in high and low CDCA4 expression groups using Kaplan-Meier method (n = 121). **p < 0.01 vs. HK-2.
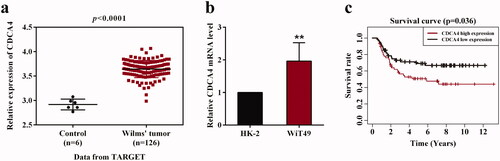
CDCA4 promotes WiT49 cells viability in vitro
To further probe the biological function of CDCA4 in WiT49 cells, its influence on the cell viability was detected using the gain- and loss-of-function of CDCA4 methods. Before detecting, the transfection status was assessed. The results revealed that si-CDCA4s notably suppressed the mRNA and protein expression levels of CDCA4 compared with control and si-NC group (p < 0.01, ). The si-CDCA4-2 was chosen to perform the following functional experiments due to its group presented a lower CDCA4 expression compared with si-CDCA4-1 group. Moreover, the expression levels of CDCA4 in WiT49 cells transfected with pcDNA3.1-CDCA4 were higher than that of control and empty vector group (p < 0.01, ). These detections indicated that we can perform the knockdown and overexpression functional experiments. The proliferation assays showed that knockdown of CDCA4 notably decreased the optical density (OD) values after transfection for 24, 48, and 72 h compared with si-NC-transfected cells (p < 0.05, ), suggesting that depletion of CDCA4 restrained the cells proliferation. On the other hand, the OD values were significantly elevated after CDCA4-overexpression compared with vector group (p < 0.05, ). All these results illustrated that CDCA4 may promote the proliferation of WiT49 cells.
Figure 2. The influence of CDCA4-knockdown and -overexpression on the CDCA4 expression and WiT49 cells proliferation. (a–b) mRNA expression (a) and protein expression levels (b) of CDCA4 were measured by qRT-PCR (a) and western blot (b) analyses after transfected with control, si-NC, si-CDCA4-1, and si-CDCA4-2 in WiT49 cells. (c) Quantification of b. **p < 0.01 vs. control or si-NC group. (d–e) mRNA expression (d) and protein expression levels (e) of CDCA4 were examined by qRT-PCR (d) and western blot (e) analyses after transfected with control, pcDNA3.1-vector, and pcDNA3.1-CDCA4 in WiT49 cells. (f) Quantification of e. **p < 0.01 vs. control or vector group. (g–h) The influences of CDCA4-knockdown (g) and -overexpression (h) on the WiT49 cells proliferation were determined by CCK8 assay. **p < 0.01 vs. si-NC or vector group.
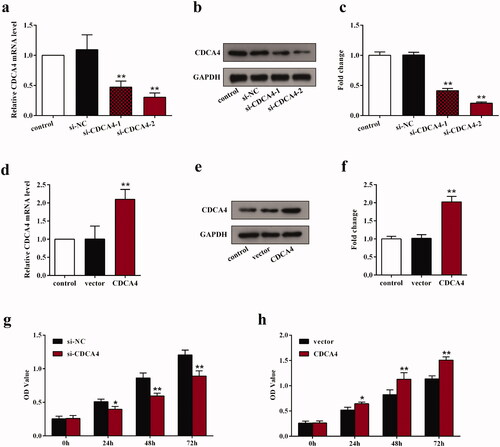
CDCA4 inhibits WiT49 cells apoptosis in vitro
To assess the apoptosis affected by CDCA4 in WiT49 cells, Annexin V-FITC/PI staining was performed. Results shown in demonstrated that depletion of CDCA4 promoted the cells apoptosis, as calculated that the percentage of cell apoptosis in si-CDCA4 group was 27.04 ± 3.47 while in the si-NC group was 14.31 ± 1.24 (p < 0.01, ). Inversely, the apoptotic rate in pcDNA3.1-CDCA4-transfected WiT49 cells (6.00 ± 1.68) was significantly lower than in vector group (15.42 ± 1.90, p < 0.01, ), indicating that CDCA4 may inhibit the WiT49 cells apoptosis.
CDCA4 activates the AKT/mTOR signaling pathway
To further understand the mechanism of CDCA4 affecting cell viability, we performed GSEA to identify signaling pathways associated with high and low CDCA4 expression groups. The results showed that cell cycle was closely related to high CDCA4 expression (). Moreover, as an important pathway in the progression of carcinomas, the mTOR signaling pathway was also included in the results of high CDCA4 expression group (). Thereupon, western blot analysis was utilized to assess the effects of CDCA4 on the protein levels of mTOR-related markers AKT and mTOR as well as cell cycle-related marker Cyclin D1. As expected, downregulation of CDCA4 significantly suppressed the level of Cyclin D1, and inhibited the AKT and mTOR phosphorylation levels (p < 0.01, ). On the contrary, the protein levels of p-AKT, p-mTOR, and Cyclin D1 were drastically enhanced after upregulation of CDCA4 compared with control (p < 0.01, ). However, no change was observed on the expression of AKT and mTOR in different groups (). All these results hinted that CDCA4 facilitated the cells viability in WiT49 cells partly through regulating the Cyclin D1 expression and activating the AKT/mTOR pathway. Additional Wilm’s tumor cell line, C64, was used to validate the key findings of the study (Supplementary figure 1–3).
Figure 4. CDCA4 affected cell cycle and AKT/mTOR signaling pathway. (a-b) GSEA was utilized to identify the relative signaling pathway associated with high CDCA4 expression. (c-f) The effects of CDCA4-knockdown (c–d) and -overexpression (e–f) on the AKT/mTOR relative markers and Cyclin D1 protein were evaluated using western blot analysis. (d) Quantification of c. (f) Quantification of e. **p < 0.01 vs. si-NC or vector group.
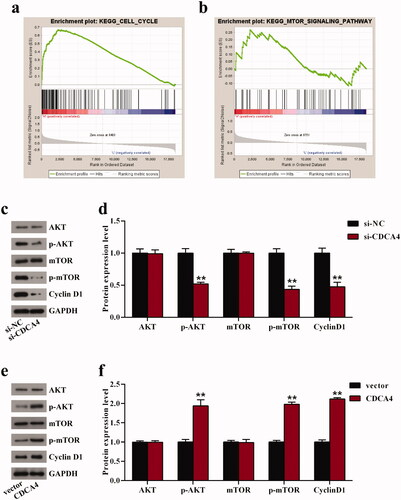
Discussion
Previous investigations have showed that acceleration of cell proliferation and downregulation of apoptosis are the most significant features of tumor tissues [Citation15]. Abnormal function of oncogenes or suppressive genes can result in uncontrolled cell growth characteristics such as increased proliferation and decreased apoptosis [Citation16,Citation17]. Although the survival rate of Wilm’s tumor patients was significantly improved by comprehensive treatments, nearly 10% of childhood patients still have poor survival due to the recurrence, metastasis, drug resistance and other reasons [Citation4,Citation18,Citation19]. Herein, this study focused on the exploration of the influences of CDCA4 on the Wilm’s tumor cells proliferation and apoptosis, as well as the potential mechanism, in the hope of providing a new insight for the treatment of Wilm’s tumor.
Accumulating evidences have revealed that abnormally expressed CDCAs are involved in the tumorigenesis [Citation14,Citation20–22]. In Wilm’s tumor, CDCA8 was found to be highly expressed and closely related to poor prognosis in patients overall survival [Citation23]. However, the role of other members of CDCA family in Wilm’s tumor remains to be fully elucidated. In our work, using the public data from the TARGET database, high expression of CDCA4 was initially uncovered and closely associated with the unsatisfactory prognosis in patients with Wilm’s tumor. Importantly, CDCA4 exhibited pro-proliferative and anti-apoptotic effects in Wilm’s tumor cells through gain- and loss-of-function methods. These are consistent with the current reports of CDCA4 in other cancers. Pang et al. clarified that CDCA4-knockdown effectively suppressed the growth of triple negative breast cancer MDA-MB-231 cells by promoting apoptosis in vitro [Citation24]. Moreover, CDCA4 was uncovered to be related to the breast cancer cell fate, as observed that downregulation of CDCA4 restrained MCF-7/ADM cells proliferation and induced apoptosis [Citation13]. Furthermore, CDCA4 was revealed to be a target of miR-15a, which displayed a strong inhibitory effect on the proliferation of melanoma cells [Citation25]. In addition, it has been reported that CDCA4 expression was markedly increased in ovarian cancer patients derived from multiple public database [Citation14]. Taken together, all these findings support that CDCA4 is associated with the Wilm’s tumor WiT49 cells fate, and may serve as an appreciable biomarker for the diagnosis and treatment of Wilm’s tumor in the future.
To in-depth explore the mechanism involved in CDCA4 affecting cell proliferation, we conduced GSEA and found that high expression of CDCA4 was linked to cell cycle and mTOR signaling pathway. Previous study also showed that genes of CDCA1 is co-expressed with known cell cycle genes including cyclin [Citation10]. Previous study demonstrated that CDCA4 participated in phosphatidylinositol 3 kinase (PI3K)-AKT signaling pathway [Citation26]. The mTOR is a central regulatory pathway that controls cell growth and homeostosis through complex molecular feedback that integrates various environmental signals [Citation27]. Abnormal mTOR signaling caused by different levels of genetic alterations in the signaling cascade is commonly observed in various types of cancer [Citation28]. The AKT/mTOR signaling cascade is the main signal transduction pathway in eukaryotic cells, regulating a variety of cellular processes including cell survival, proliferation and angiogenesis [Citation29], which means that inactivation of AKT/mTOR signaling pathway may be a potential strategy for treatment of Wilm’s tumor. Recent studies have also illustrated that AKT/mTOR pathway is involved in the pathogenesis and progression of Wilm’s tumor. Luo et al. indicated that miR-155-5p functions as a tumor inhibitor in Wilm’s tumor via inactivating the AKT/mTOR signaling pathway [Citation26]. Moreover, Li et al found that Salidroside suppresses Wilm’s tumor cells growth, leading to the deactivation of AKT/mTOR signaling pathway [Citation30]. The present study demonstrated that CDCA4-knockdown inhibited the AKT/mTOR signaling pathway in Wilm’s tumor cells whereas upregulation of CDCA4 activated the AKT/mTOR pathway. Thus, we concluded that CDCA4 exhibited anti-proliferation effects on the Wilm’s tumor cells via activating the AKT/mTOR signaling pathway.
The treatment strategies for patients with advanced renal cell carcinoma is rapidly evolving, and combination paradigm and novel first-line drugs will likely be introduced [Citation31]. The mechanistic target of mTOR, through its two distinct multiprotein complexes, mTORC1, and mTORC2, serves a key role in the modulation of cell survival [Citation32]. Aberrant mTORC1 and mTORC2 signaling is contributed to progression of cancers by modulating mTOR and its downstream targets. Additionally, a recent review reported that the target of mTOR seemed to be the most qualified target in several mammalian tumors, such as renal cell carcinoma, and mechanisms of resistance on targeting mTOR are rapidly developed due to this kinase has a critical role in cellular growth [Citation33]. Therefore, the role of PI3K/AKT/mTOR inhibitors in combinations with CDCA4 inhibition seems to be interesting and promising for control of Wilm’s tumor. The combination therapies as well as the CDCA4 biomarker that predict outcomes with treatments may be contributed to improvements in survival and other clinical outcomes for patients with Wilm’s tumor. However, the lack of in vivo experiments was a potential limitation to the present study, which should be further investigated in the future. In short, the present report revealed the high expression of CDCA4 in Wilm’s tumor, which was closely related to poor prognosis for childhood with Wilm’s tumor. Furthermore, we demonstrated that CDCA4 promoted the proliferation and induced the apoptosis via activation of the AKT/mTOR signaling pathway. All these findings provide a new insight into the pathogenic mechanism of Wilm’s tumor and suggest that CDCA4 may be a potential therapeutic target for treatment of Wilm’s tumor.
Author contributions
SL and JM designed this study. SL, CQ, YC, and DW performed the experiments and collected and analyzed the data with the help of ZT. ZT performed the biostatistical evaluation of the data. SL and JM contributed to drafting the manuscript. All authors have read and approved the final submitted manuscript.
Supplementary Figures
Download PDF (624.1 KB)Disclosure statement
The authors report no proprietary or commercial interest in any product mentioned or concept discussed in this article.
References
- Friedman AD. Wilms tumor. Pediatr Rev. 2013;34(7):328–330. discussion 330.
- Hohenstein P, Pritchard-Jones K, Charlton J. The yin and yang of kidney development and Wilms’ tumors. Genes Dev. 2015;29(5):467–482.
- Pritchard-Jones K. Controversies and advances in the management of Wilms’ tumour. Arch Dis Child. 2002;87(3):241–244.
- Cone EB, Dalton SS, Van Noord M, et al. Biomarkers for Wilms tumor: a systematic review. J Urol. 2016;196(5):1530–1535.
- Phelps HM, Kaviany S, Borinstein SC, et al. Iological drivers of Wilms tumor prognosis and treatment. Children (Basel, Switzerland). 2018;5(11):145.
- Irtan S, Ehrlich PF, Pritchard-Jones K. Wilms tumor: "State-of-the-art" update, 2016. Semin Pediatr Surg. 2016;25(5):250–256.
- Vader G, Lens SM. The Aurora kinase family in cell division and cancer. Biochim Biophys Acta. 2008;1786(1):60–72.
- Collins I, Garrett MD. Targeting the cell division cycle in cancer: CDK and cell cycle checkpoint kinase inhibitors. Curr Opin Pharmacol. 2005;5(4):366–373.
- Altieri DC. Survivin, versatile modulation of cell division and apoptosis in cancer. Oncogene. 2003;22(53):8581–8589.
- Walker MG. Drug target discovery by gene expression analysis: cell cycle genes. Curr Cancer Drug Targets. 2001;1(1):73–83.
- Hayashi R, Goto Y, Ikeda R, et al. CDCA4 is an E2F transcription factor family-induced nuclear factor that regulates E2F-dependent transcriptional activation and cell proliferation. J Biol Chem. 2006;281(47):35633–35648.
- Tategu M, Nakagawa H, Hayashi R, et al. Transcriptional co-factor CDCA4 participates in the regulation of JUN oncogene expression. Biochimie. 2008;90(10):1515–1522.
- Xu Y, Wu X, Li F, et al. CDCA4, a downstream gene of the Nrf2 signaling pathway, regulates cell proliferation and apoptosis in the MCF‑7/ADM human breast cancer cell line. Mol Med Rep. 2018;17(1):1507–1512.
- Zhang Y, Cheng Y, Zhang Z, et al. CDCA2 inhibits apoptosis and promotes cell proliferation in prostate cancer and is directly regulated by HIF-1α pathway. Front Oncol. 2020;10:725.
- Zhao XS, Han B, Zhao JX, et al. MiR-155-5p affects Wilms’ tumor cell proliferation and apoptosis via targeting CREB1. Eur Rev Med Pharmacol Sci. 2019;23(3):1030–1037.
- Compagni A, Christofori G. Recent advances in research on multistage tumorigenesis. Br J Cancer. 2000;83(1):1–5.
- Sulić S, Panić L, Dikić I, et al. Deregulation of cell growth and malignant transformation. Croat Med J. 2005;46(4):622–638.
- Qi C, Hu Y, Yang F, et al. Preliminary observations regarding the expression of collagen triple helix repeat-containing 1 is an independent prognostic factor for Wilms’ tumor. J Pediatr Surg. 2016;51(9):1501–1506.
- Brok J, Pritchard-Jones K, Geller JI, et al. Review of phase I and II trials for Wilms’ tumour - can we optimise the search for novel agents? Eur J Cancer. 2017;79:205–213.
- Phan NN, Wang CY, Li KL, et al. Distinct expression of CDCA3, CDCA5, and CDCA8 leads to shorter relapse free survival in breast cancer patient. Oncotarget. 2018;9(6):6977–6992.
- Chen C, Chen S, Luo M, et al. The role of the CDCA gene family in ovarian cancer. Ann Transl Med. 2020;8(5):190–190.
- Zhang Z, Shen M, Zhou G. Upregulation of CDCA5 promotes gastric cancer malignant progression via influencing cyclin E1. Biochem Biophys Res Commun. 2018;496(2):482–489.
- Wang X, Song P, Huang C, et al. Weighted gene co‑expression network analysis for identifying hub genes in association with prognosis in Wilms tumor. Mol Med Rep. 2019;19(3):2041–2050.
- Pang S, Xu Y, Chen J, et al. Knockdown of cell division cycle-associated protein 4 expression inhibits proliferation of triple negative breast cancer MDA-MB-231 cells in vitro and in vivo. Oncol Lett. 2019;17(5):4393–4400.
- Alderman C, Yang Y. The anti-melanoma activity and oncogenic targets of hsa-miR-15a-5p. RNA & Dis. 2016;3(4):e1450.
- Wu B, Huang Y, Luo Y, et al. The diagnostic and prognostic value of cell division cycle associated gene family in hepatocellular carcinoma. J Cancer. 2020;11(19):5727–5737.
- Sulaimanov N, Klose M, Busch H, et al. Understanding the mTOR signaling pathway via mathematical modeling. Wiley Interdiscip Rev Syst Biol Med. 2017;9(4):e1379.
- Tian T, Li X, Zhang J. mTOR signaling in cancer and mTOR inhibitors in solid tumor targeting therapy. IJMS. 2019;20(3):755.
- Mo C, Shetti D, Wei K. Erianin inhibits proliferation and induces apoptosis of HaCaT cells via ROS-Mediated JNK/c-Jun and AKT/mTOR signaling pathways. Molecules. 2019;24(15):2727.
- Xue H, Li P, Luo Y, et al. Salidroside stimulates the Sirt1/PGC-1α axis and ameliorates diabetic nephropathy in mice. Phytomedicine. 2019;54:240–247.
- Calvo E, Porta C, Grünwald V, et al. The current and evolving landscape of first-line treatments for advanced renal cell carcinoma. Oncologist. 2019;24(3):338–348.
- Ong PS, Wang LZ, Dai X, et al. Judicious toggling of mTOR activity to combat insulin resistance and cancer: current evidence and perspectives. Front Pharmacol. 2016;7:395.
- Formisano L, Napolitano F, Rosa R, et al. Mechanisms of resistance to mTOR inhibitors. Crit Rev Oncol Hematol. 2020;147:102886.