Abstract
Background
Early recognition of persistent acute kidney injury (AKI) could optimize management and prevent deterioration of kidney function. The Doppler-based renal resistive index (RI) has shown promising results for predicting persistent AKI in preliminary studies. Here, we aimed to evaluate the performance of renal RI, clinical indicators, and their combinations to predict short-term kidney prognosis in septic shock patients.
Method
We performed a retrospective study based on data from a prospective study in a single-center general ICU between November 2017 and October 2018. Patients with septic shock were included. Clinical indicators were evaluated immediately at inclusion, and renal RI was measured within the first 12 h of ICU admission after hemodynamic stabilization. Persistent AKI was defined as AKI without recovery within 72 h. A multivariable logistic regression was used to select significant variables associated with persistent AKI. The discriminative power was evaluated by a receiver operating characteristic curve analysis.
Result
Overall, 102 patients were included, 39 of whom had persistent AKI. Renal RI was higher in the persistent AKI patients than in those without persistent AKI: 0.70 ± 0.05 vs. 0.66 ± 0.05; p = 0.001. The performance of RI to predict persistent AKI was poor, with an area under the receiver operating characteristic curve (AUROC) of 0.699 [95% confidence interval (CI) 0.600–0.786]. A clinical prediction model combining serum creatinine at inclusion and the nonrenal SOFA score showed a better prediction ability for nonrecovery, with an AUROC of 0.877 (95% CI 0.797–0.933, p = 0.0012). The addition of renal RI to this model did not improve the predictive performance.
Conclusion
The Doppler-based renal resistive index performed poorly in predicting persistent AKI and did not improve the clinical prediction provided by a combination of serum creatinine at inclusion and the nonrenal SOFA score in patients with septic shock.
Introduction
Acute kidney injury (AKI) is a common and severe complication in critically ill patients, especially in patients with sepsis, who account for up to 50% of all patients [Citation1,Citation2]. The development of AKI is associated with increased morbidity and short-term mortality [Citation3]. Early recognition of AKI without short-term recovery is challenging but crucial in ICU clinical practice, as the distinction between transient and persistent AKI might help to optimize treatment, such as promptly restoring kidney perfusion, limiting fluids, and avoiding nephrotoxic agents and might help with patients facing kidney replacement therapy (KRT) [Citation4], which then can improve the outcome [Citation5,Citation6].
Usual markers of kidney dysfunction, namely, insensitive serum creatinine (sCr) or nonspecific oliguria, limit the early diagnosis, differential diagnosis, or prognostic evaluation of AKI [Citation7]. Some urinary indexes and biomarkers, such as fractional excretion of urea, tissue inhibitor of metalloproteinases-2 and insulin-like growth factor-binding protein 7 ([TIMP-2]*[IGFBP7]), have been assessed for the early prediction of persistent AKI. However, these indicators perform poorly and cannot be recommended for widespread use in clinical practice [Citation8–10].
Vascular dysfunction may play a vital role in the pathophysiological mechanisms of septic AKI [Citation11]. Renal Doppler ultrasound can measure the renal resistive index (RI), which is a sonographic index that reflects alterations in the blood flow profile of the intrarenal arcuate or interlobar arteries, allowing us to explore kidney hemodynamics noninvasively. RI has been used to assess kidney perfusion [Citation12,Citation13] and to predict kidney dysfunction [Citation14–16] and recovery from AKI in the ICU [Citation16,Citation17]. Several studies have shown its efficiency in predicting persistent AKI [Citation15,Citation16,Citation18]; however, large heterogeneity between these studies was noted [Citation17]. In addition, AKI is a complex condition, and it is unlikely that any single marker of kidney injury will be able to fully predict the changes in kidney function. Some clinical variables, such as age or comorbid diabetes, contributing to hardening of the arteries have been associated with AKI and its short-term prognosis [Citation19]. Similarly, fluid accumulation has been demonstrated to be a negative predictor for the recovery of kidney function because it may cause hypoxemic injury to the kidney [Citation20]. Furthermore, as a systemic perfusion indicator, lactate, can reflect kidney hypoperfusion to some extent [Citation21], which may have an impact on the reversibility of AKI. Some studies have shown that the severity of illness may also help to predict kidney recovery from AKI [Citation1,Citation22]. The value of combining renal RI with clinical indicators has not been studied in the early prediction of persistent AKI. Therefore, the main objective of this study was to evaluate the ability of renal RI, clinical indicators, and especially the combination of these parameters to predict persistent AKI in patients with septic shock.
Methods
Data source and study population
For a retrospective study, we used the data of the patients with septic shock from a previous prospective study, which was conducted to evaluate the ability of renal RI and central venous pressure (CVP) to predict AKI in a single-center general ICU between November 2017 and October 2018 [Citation23]. The study protocol was approved by Hebei General Hospital Institutional Review Board (No. 2017–126), which waived the need for signed informed consent, given the noninterventional study design. In that previous study, all consecutive patients with septic shock according to the Third International Consensus Criteria [Citation24] were included immediately after admission to the ICU in Hebei General Hospital. The exclusion criteria were age <18 years, pregnancy, hospital stay <24 h, having already recovered from AKI according to our definition, chronic kidney disease stage IV–V, history of kidney transplantation, and no central venous catheterization of the internal jugular vein or subclavian vein. Patients with confirmed renal artery stenosis or renal vein thrombosis and cardiac arrhythmia were also excluded because these conditions might preclude renal Doppler measurement. The primary end-point of the previous study was the onset of AKI. Although the study was open, the investigators were blinded to the primary objective of this study, and patient management was at the discretion of the physician in charge, who was blinded to the renal RI.
For the current study, all the patients who were enrolled in the previous study were included. This study excluded the patients who in imminent need of KRT after ICU admission and those in whom the nature of AKI recovery was nonevaluable at 72 h after inclusion (i.e. no available sCr could be used to classify AKI because of discharge from ICU).
Definitions
Sepsis was defined according to the Third International Consensus Criteria as the presence of an infection (with a likelihood of at least possible) and organ dysfunction(s) represented by two or more Sequential Organ Failure Assessment (SOFA) points. Patients with septic shock could be identified with a clinical construct of sepsis with persisting hypotension requiring vasopressors to maintain a mean arterial pressure (MAP) of ≥65 mmHg and having a serum lactate level >2 mmol/L (18 mg/dL) despite adequate volume resuscitation [Citation24].
AKI was defined at study inclusion according to the Kidney Disease Improving Global Outcomes (KDIGO) classification using both the creatinine and urine output criteria. AKI was diagnosed by either an increase in sCr greater than or equal to 26.4 µmol/L or an sCr greater than 1.5 times from baseline or a urine output less than 0.5 mL/kg/h for 6 h. AKI severity was determined separately and was classified as stage 1, stage 2, or stage 3 based on the changes in the creatinine concentration or urine output according to the KDIGO guidelines [Citation25]. For each patient, baseline sCr was defined according to the sCr measured the year prior to admission to the ICU. When the baseline sCr level was unknown, this variable was estimated according to the Modification of Diet Renal Disease (MDRD) formula back-calculation [Citation26].
Transient AKI was defined as AKI with recovery occurring within 72 h after inclusion [Citation5,Citation16]. Recovery from AKI was defined as a decrease of at least one stage in AKI severity according to the KDIGO criteria (i.e. decrease of the sCr, reversal of oliguria in the absence of diuretic therapy, and absence of KRT). Persistent AKI was defined as persistent oliguria and/or as a steady or a higher AKI KDIGO stage [Citation16]. When no available sCr could be used to classify AKI because of death, AKI was classified as persistent if the patient met one of the KDIGO criteria for their last measurement. The duration of AKI was measured from its occurrence, which was generally considered to be the time that it was first diagnosed. For the patients who had been diagnosed with AKI at ICU admission, the duration of AKI was calculated from their ICU admission.
Hemodynamic stabilization was considered when the MAP was at least at 65 mmHg without the need for a bolus of fluids and/or the initiation of a vasopressor or an increase in the patient’s vasopressor dose within the last 6 h [Citation15,Citation16].
Diuretic use was defined as the use of diuretics at any time during the first 24 h in the ICU.
Study protocol and data collection
All eligible patients were treated according to the 2016 Surviving Sepsis campaign guidelines [Citation27]. Serum creatinine was measured at inclusion and then at least once daily, and urine output was recorded hourly for patients with an indwelling urinary catheter based on our standard procedures. Assessments of kidney function were performed at study inclusion and during the next 72 h according to the serum creatinine and/or urine output. AKI was only determined by creatinine elevation in patients who did not have their hourly urine output measured via an unlocated urinary catheter.
The patients were divided into persistent AKI (P-AKI) and nonpersistent AKI (no P-AKI) groups based on our definition for persistent AKI. Because no difference was observed between the renal RI values in the patients without AKI and those in the patients with transient AKI in a previous study [Citation16], these patients were assigned to one group, namely, the no P-AKI group.
Demographic data, such as age and sex, were recorded, together with any history of hypertension, diabetes, or chronic kidney disease. The baseline serum creatinine and its means of estimation, mode for ICU admission, time from vasopressor initiation to inclusion, and sepsis sources were collected. The following clinical indicators measured at inclusion were also noted: heart rate, MAP, sCr, hemoglobin, platelet count, total bilirubin, ratio of arterial oxygen partial pressure to fractional inspired oxygen, partial pressure of carbon dioxide, lactate, procalcitonin, CVP, norepinephrine dose and the need for mechanical ventilation. During patient management, the time from inclusion to hemodynamic stabilization, the amount of fluid therapy received within the first 24 h, and the use of diuretics were collected. Patients who were given nephrotoxic agents, including angiotensin II converting enzyme inhibitors or angiotensin receptor blockers, iodinated contrast agents, aminoglycosides, and glycopeptides, were also recorded. The severity of illness was determined using the Acute Physiology and Chronic Health Evaluation (APACHE II) and SOFA score at ICU admission. The SOFA score without a renal component was calculated simultaneously. Two investigators blinded to the primary objective of this study were responsible for collecting the data.
In each patient, renal sonography for RI measurement was performed within the first 12 h of ICU admission and after hemodynamic stabilization. The RI was calculated using a 2–5 MHz curved array transducer (Compact Xtreme CX50, Philips Medical Systems, Bothell, WA, USA) by two investigators (all certified physicians who had received training on the Doppler evaluation of kidney perfusion from the Chinese Critical Ultrasound Study Group). The B mode allowed kidney localization and the detection of signs of chronic kidney disease. A longitudinal view of the kidney was obtained in B-mode US in the posterolateral approach, and color Doppler allowed for vessel localization. An interlobar or arcuate artery was identified and then selected. Pulse-wave Doppler was used to measure blood velocities. The Doppler spectrum was considered optimal when at least three similar consecutive waveforms were visualized. The peak systolic velocity (Vmax) and the minimal diastolic velocity (Vmin) were recorded, and the RI was calculated with the following formula: (Vmax–Vmin)/Vmax. Three measurements were averaged to obtain the mean RI values used for the study[Citation13]. The right kidney was generally chosen for measurement. If the RI of the right kidney was not obtained, the left side could be selected.
Statistical analysis
Continuous variables were described as the mean ± standard deviation (SD) or median and interquartile range (IQR) based on their distribution. Categorical variables were expressed as frequencies and proportions. Comparisons between groups were assessed by unpaired t test or Mann–Whitney test. Categorical variables were analyzed using the chi-square test or Fisher’s exact test.
Based on previous findings [Citation16,Citation28], the sample size was calculated as follows. Assuming an RRI of 0.80 in patients with persistent AKI with a standard deviation of 0.06, using a two-sided test with an α-risk (type I error) of 5% and a statistical power of 80%, we needed 26 patients to detect a 10% absolute difference in RI between patients with persistent AKI and those with transient AKI or without AKI. According to the requirements of a reliable logistic model, we assumed that three or four variables would be included in a prediction model: “nonrenal SOFA score”, “norepinephrine dose”, “fluid balance” or “comorbid diabetes”. Ten events per variable means we needed 30–40 events (patients with persistent AKI) [Citation29]. Assuming a 40% incidence rate based on previous studies [Citation10,Citation16], 75–100 patients needed to be enrolled.
Logistic regression was performed to identify variables significantly associated with persistent AKI. The selected variables were “serum creatinine at inclusion”, “nonrenal SOFA score”, “lactate”, “fluid balance at 24 h” and “norepinephrine dose”, and they were selected according to their clinical relevance and statistical significance in the univariate analysis. A multivariable logistic regression analysis was then performed, and the variables were forced into the model (enter mode). The Hosmer Lemeshow test was used to check the goodness of fit of the logistic regression.
Discriminative power was evaluated in a receiver operating characteristic (ROC) curve analysis. The area under the receiver-operating characteristic curve (AUROC) was recorded, together with its 95% confidence interval (CI). AUROCs were compared in DeLong’s test [Citation30]. We used the Youden index to determine the optimal cutoff for calculations of specificity, sensitivity, and positive and negative predictive performances.
All statistical analyses were performed using SPSS 21 (IBM, Armonk, NY, USA) and MedCalc version 16.8 (MedCalc Software, Ostend, Belgium), with a two-sided p-value <0.05 considered statistically significant.
Result
Population characteristics
We identified 102 patients who were enrolled for the final analysis (). The characteristics of the 102 analyzed patients are shown in . The average age was 70 years, and approximately two-thirds were male. A total of 46 (45.1%) patients were admitted to the ICU after the operation, and 25 (24.5%) patients were from the emergency room, which is similar amount compared to that from the ward (28.4%). The presumed or confirmed sepsis sources were as follows: respiratory tract (40, 39.4%), abdominal (36, 35.3%), urinary tract (11, 10.8%), and other sites (15, 12.7%). The mean APACHE II and SOFA scores were 16.3 ± 4.8 and 8.5 ± 3.5, respectively. Nine patients received KRT in the ICU. The median ICU stay was 4.3 days, and the ICU mortality rate was 26.0%.
Table 1. Patient characteristics.
According to our definitions, the P-AKI group was comprised of 39 patients who developed persistent AKI, the no P-AKI group was comprised of 31 patients who had transient AKI and there were 32 patients who did not develop AKI. There were no differences in age or sex between the two groups. The P-AKI group had a higher proportion of patients with diabetes mellitus (41.0% vs. 22.2%, p = 0.043) but did not have a higher proportion of patients with hypertension and chronic kidney disease. The mode of ICU admission, primary source of sepsis and time from vasopressor initiation to inclusion were similar between the groups. Some physiological indexes and laboratory measurements, such as CVP, sCr at inclusion, platelets, total bilirubin, lactate concentration, and procalcitonin, were significantly different between the groups (). The patients with persistent AKI had higher SOFA scores (11.0 ± 3.6 vs. 6.9 ± 2.4; p < 0.001) and nonrenal SOFA scores (9.7 ± 3.5 vs. 6.6 ± 2.4; p < 0.001) at inclusion. In the patients with persistent AKI, a higher norepinephrine dose (0.48 [0.14–0.90] vs. 0.20 [0.10–0.35]); p = 0.006) and more fluid (45.6 ± 44.8 vs. 22.8 ± 22.6); p = 0.029) were needed; KRT was initiated in 23.1% of the patients; and their ICU mortality was significantly increased (46.2% vs. 25.5%; p < 0.001). However, their ICU stay duration did not differ (4.3 [2.7–7.2] vs. 4.0 [2.2–7.8]; p = 0.608).
Significant clinical indicators associated with persistent AKI
According to the clinical relevance and statistical significance of the variables in the univariate analysis, “serum creatinine at inclusion”, “nonrenal SOFA score”, “lactate” and “norepinephrine dose” were forced into a model for the prediction of persistent AKI. Based on logistic regression analysis, “serum creatinine at inclusion” and “nonrenal SOFA score” had a significant association with persistent AKI (). When “lactate” and “fluid balance at 24 h” were forced to combine with “serum creatinine at inclusion” and “nonrenal SOFA score”, they were not independently associated with persistent AKI, and neither were “fluid balance at 24 h” and “norepinephrine dose”. Therefore, the final model included serum creatinine at inclusion (OR, 1.03; 95% CI 1.01–1.04) and nonrenal SOFA score (OR, 1.48; 95% CI 1.21–1.81).
Table 2. Logistic regression models for the prediction of persistent AKI.
Performance of renal resistive index, clinical indicators and their combination for predicting persistent AKI
The renal RI was significantly higher in the patients with persistent AKI (0.70 ± 0.05 vs. 0.66 ± 0.05; p = 0.001). The performance of RI to predict persistent AKI was poor, with an AUROC (95% CI) of 0.699 (0.600–0.786). The sensitivity and specificity were 56.4% and 73.0%, respectively, for an optimal cutoff equal to 0.68. The clinical model combining serum creatinine at inclusion and the nonrenal SOFA score had a good performance for predicting persistent AKI, with an AUROC of 0.877 (0.797–0.933), which was significantly better than renal RI (z statistic = 3.076, p = 0.0021). When incorporated into these parameters, renal RI was not independently associated with persistent AKI (OR 1.09 per 0.01 unit; 95% CI 0.98–1.21, p = 0.116) and did not improve the performance of the clinical model for predicting persistent AKI (AUROC 0.877; 95% CI 0.797–0.934, p = 0.9749) ( and ).
Figure 2. ROC curve reflecting performance of renal resistive index, the clinical model and their combination for predicting persistent AKI Clinical model includes serum creatinine at inclusion and nonrenal Sequential Organ Failure Score (SOFA). RI: resistive index.
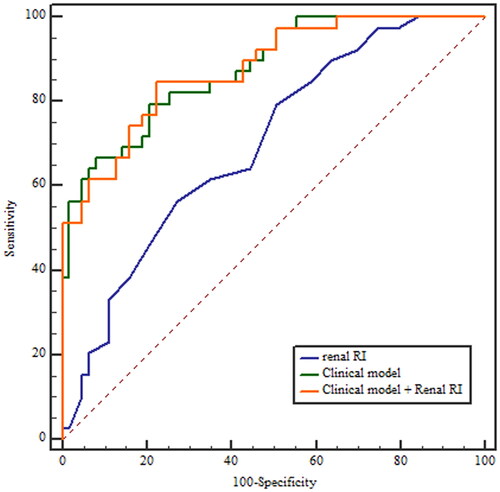
Table 3. Performance of renal RI, the clinical model and their combination for predicting persistent AKI.
The discriminative performance of renal RI was also assessed in the subgroup of patients presenting with AKI at inclusion, and it remained similarly limited in this subgroup, as well as in the patients whose baseline serum creatinine was calculated using different methods (). The percentage of patients aged 70 years or older was as high as 56.9% (58/102) in our study population. We also evaluated the predictive ability of renal RI in this subgroup, and the performance of RI was also not good, with an AUROC of 0.716 (95% CI 0.583–0.827) ().
Table 4. Performance of renal RI for predicting persistent AKI in different subgroups.
Discussion
In this study, we evaluated renal RI and clinical indicators to predict persistent AKI in patients with septic shock. Renal RI was unable to predict persistent AKI and did not improve the prediction provided by the usual clinical variables. A combination of serum creatinine at inclusion and the nonrenal SOFA score showed good performance in prognosticating AKI.
Several studies suggested that the Doppler-based renal resistive index might help to separate transient from persistent AKI in critically ill patients [Citation15,Citation16]. A recent study including 100 patients with AKI showed a very good performance of RI in predicting short-term reversibility of AKI in patients in a medical ICU [Citation31], and similar results were reported in a meta-analysis [Citation17]. In our cohort, the results were different. Several points can explain the differences.
First, renal RI has been considered a valuable and repeatable marker of renal vascular resistance (RVR) and blood flow [Citation13,Citation32] and was proposed as a biomarker to monitor kidney perfusion in ICU patients [Citation33]. However, experimental studies have shown that the association between Doppler-based RI and RVR was weak and that supraphysiological variations of resistance only translated into small RI variations clinically [Citation34]. In addition, numerous confounding factors could influence RI values, including vascular compliance, MAP, heart rate, hypoxemia and hypercapnia [Citation14,Citation28,Citation35–37]. In several studies, vascular compliance was a major determinant of RI [Citation34,Citation38,Citation39]. Decreased vascular compliance may result not only from preexisting subclinical vascular stiffness (e.g. aging [Citation40], hypertension [Citation41], diabetes mellitus [Citation42]) but also from acute changes in the renal central vasculature [Citation28], renal interstitial pressures or intra-abdominal pressure [Citation39,Citation43]. The impact of intra-abdominal pressure on renal RI also comes from the possible contribution of the reduction in the renal perfusion due to the increase of intra-abdominal pressure [Citation13,Citation44]. Furthermore, the relationship between RI and RVR seemed to be linear only when the vascular compliance was normal, but this relationship progressively disappeared when the arterial stiffness increased [Citation34,Citation38]. Given the numerous factors influencing RI and its weak correlation to renal blood flow, the potential ability of RI to predict the reversibility of AKI seems questionable.
Second, AKI is a complex and heterogeneous clinical syndrome. Kidney damage varies according to the type of primary insult, secondary effects and mitigating responses and leads to distinct molecular, cellular and functional changes. Different subtypes of AKI with varying recovery patterns have been identified [Citation45]. We focused on septic shock patients who were considered to be exposed to multiple kidney insults. The exact mechanism for sepsis-associated AKI is still under investigation. Macrovascular and microvascular dysfunction, immunological and autonomic dysregulation, and abnormal cellular response to injury have been proposed [Citation11]. Along with these findings, previous studies suggested that a longer duration of AKI may reflect a more serious form of AKI rather than specific pathophysiological mechanisms (e.g. septic, nephrotoxic, inflammatory) [Citation46,Citation47]. Based on the above complex mechanisms and their interaction, renal RI, as a preliminary assessment of microvascular changes in the kidney, may lack the ability to predict kidney recovery. Saade et al. [Citation48] performed a multicenter, prospective cohort study of 371 unselected ICU patients to evaluate the performance of RI to separate patients with transient AKI from patients with persistent AKI. Similar to our results, RI performed poorly in predicting persistent AKI, and it was not recommended that RI be used to assess short-term kidney prognosis in the ICU setting. In addition, in terms of assessing AKI severity, it was found that RI also may not be a good predictor of stage 3 AKI in the study conducted by Zhi et al. [Citation49].
Our hypothesis was that clinical indicators could improve the ability of RI in predicting kidney recovery; however, RI performed poorly. Finally, the clinical model combining sCr at inclusion and SOFA score without a renal component was more effective for predicting short-term changes in kidney function. Considering the complexity of AKI, researchers have attempted to utilize different clinical variables combined with or without AKI biomarkers to construct clinical prediction models for AKI recovery. In a single center cohort of 57 patients with AKI, Dewitte [Citation50] showed that when the nonrenal SOFA score, sCr at inclusion and fluid balance were selected to establish a clinical model, combinations of AKI markers in addition to this clinical model improved the discrimination of patients who would recover from AKI. Notably, this clinical model showed good predictive value for nonrecovery, with an AUROC of 0.87 (95% CI 0.78–0.9). Similarly, Jia et al. [Citation51] found that the predictive accuracy of nonrecovery can be improved when [TIMP-2]*[IGFBP7] was combined with the clinical factors of AKI diagnosed by the urine output criteria, AKI stage 2–3 and nonrenal SOFA score. Additionally, Titeca-Beauport et al. [Citation9] reported that a predictive model including sCr, nonrenal SOFA and two other clinical indicators had better discrimination in persistent AKI than [TIMP-2]*[IGFBP7] in the early phase of septic shock. In keeping with these findings, our study suggested that the nonrenal SOFA score and sCr at inclusion were independent predictors for persistent AKI and that their combination had good performance in prognosticating AKI.
The renal angina index (RAI), which is based on small changes in serum creatinine and patient conditions, is another remarkable predictive model. Analogous to myocardial angina, the concept of renal angina combines risk stratification and clinical signs to increase the pretest probability of AKI biomarkers [Citation52]. In fact, RAI was suggested to be efficient in discriminating pediatric patients who will develop persistent AKI [Citation53], and a similar score has been developed and validated in an adult setting and performed well in predicting the low risk of persistent AKI [Citation54]. Additionally, incorporation of AKI biomarkers into RAI may potentially improve prediction [Citation55]. However, several limitations, such as low event rate and unreported or poor calibration, make RAI controversial and difficult to be utilized clinically [Citation56]. Because renal RI measurement is a noninvasive, bedside, rapid and repeatable tool, we still want to use it to assess kidney recovery, despite its poor performance in our study. Similar to the process of constructing RAI, future studies could aim to utilize RI to risk stratify patients or to preliminarily select the potential population of patients at high risk of persistent AKI, among whom AKI biomarkers or other predictive tools may be more economical and powerful.
Our study had a high proportion of elderly patients, and the subgroup analyses did not find a predictive advantage of RI. However, elderly patients might have a higher baseline SOFA score because of comorbidities, and Ferreira et al.[Citation57] reported that compared with the admission SOFA score, the delta-SOFA score (differences between subsequent scores) was a better indicator of prognosis, especially in patients with chronic organ dysfunction. Therefore, dynamic changes in the SOFA score may be a better choice to develop a multivariable model instead of using a static value for this population. However, using a static value did not have an impact on the significance of our current prediction model because there was no difference in age between the two groups.
Our study has several limitations. First, our definition of persistent AKI differs from the recently suggested definition of the Acute Disease Quality Initiative group [Citation7]. However, the design of our study was initiated before the publication of this definition. Second, eighteen patients no longer required norepinephrine when they arrived at the ICU because of previous infection control and fluid resuscitation in the emergency room or operating room. Sixteen of them did not develop persistent AKI, which may lead to bias in the statistical results. Third, we did not obtain RI prior to resuscitation because the change in RI before and after hemodynamic resuscitation may be a better indicator for predicting persistent AKI. However, the value of RI was unstable due to the impact of dynamic changes in MAP prior to hemodynamic resuscitation [Citation58]. Fourth, we did not consider the effect of positive end-expiratory pressure and peak airway pressure on RI, which would lead to a high RI value [Citation43,Citation59]. Last, this was a single-center observational study focusing on septic shock patients, and the findings may not have sufficient external validation.
Conclusion
In summary, we found that the Doppler-based renal resistive index performed poorly in predicting persistent AKI and did not improve the clinical prediction provided by a combination of serum creatinine at inclusion and nonrenal SOFA score in patients with septic shock. To take better advantage of renal RI, further studies are needed to risk stratify patients or deem RI as a preliminary screening tool.
Ethical approval
This study complied with the guidelines of the Declaration of Helsinki and was approved by Hebei General Hospital Institutional Review Board (No. 2017–126), which waived the need for signed informed consent, given the noninterventional study design.
Acknowledgments
The authors thank all the participants of this study.
Disclosure statement
No potential conflict of interest was reported by the author(s).
Data availability statement
The datasets used and/or analyzed during the current study are available from the corresponding author on reasonable request.
Additional information
Funding
References
- Uchino S, Kellum JA, Bellomo R, Beginning and Ending Supportive Therapy for the Kidney (BEST Kidney) Investigators, et al. Acute renal failure in critically ill patients: a multinational, multicenter study. JAMA. 2005;294(7):813–818.
- Hoste EA, Bagshaw SM, Bellomo R, et al. Epidemiology of acute kidney injury in critically ill patients: the multinational AKI-EPI study. Intensive Care Med. 2015;41(8):1411–1423.
- Bagshaw SM. Short- and long-term survival after acute kidney injury. Nephrol Dial Transplant. 2008;23(7):2126–2128.
- Bouchard J, Soroko SB, Chertow GM, Program to Improve Care in Acute Renal Disease (PICARD) Study Group, et al. Fluid accumulation, survival and recovery of kidney function in critically ill patients with acute kidney injury. Kidney Int. 2009;76(4):422–427.
- Perinel S, Vincent F, Lautrette A, et al. Transient and persistent acute kidney injury and the risk of hospital mortality in critically ill patients: results of a multicenter cohort study. Crit Care Med. 2015;43(8):e269-75–e275.
- Truche AS, Ragey SP, Souweine B, et al. ICU survival and need of renal replacement therapy with respect to AKI duration in critically ill patients. Ann Intensive Care. 2018;8(1):127.
- Chawla LS, Bellomo R, Bihorac A, Acute Disease Quality Initiative Workgroup 16., et al. Acute kidney disease and renal recovery: consensus report of the acute disease quality initiative (ADQI) 16 workgroup. Nat Rev Nephrol. 2017;13(4):241–257.
- Wlodzimirow KA, Abu-Hanna A, Royakkers AA, et al. Transient versus persistent acute kidney injury and the diagnostic performance of fractional excretion of urea in critically ill patients. Nephron Clin Pract. 2014;126(1):8–13.
- Titeca-Beauport D, Daubin D, Van Vong L, et al. Urine cell cycle arrest biomarkers distinguish poorly between transient and persistent AKI in early septic shock: a prospective, multicenter study. Crit Care. 2020;24(1):280. Jun 1
- Pons B, Lautrette A, Oziel J, et al. Diagnostic accuracy of early urinary index changes in differentiating transient from persistent acute kidney injury in critically ill patients: multicenter cohort study. Crit Care. 2013;17(2):R56.
- Ronco C, Bellomo R, Kellum JA. Acute kidney injury. Lancet. 2019;394(10212):1949–1964.
- Schnell D, Darmon M. Bedside doppler ultrasound for the assessment of renal perfusion in the ICU: advantages and limitations of the available techniques. Crit Ultrasound J. 2015;7(1):24.
- Schnell D, Darmon M. Renal doppler to assess renal perfusion in the critically ill: a reappraisal. Intensive Care Med. 2012;38(11):1751–1760.
- Lerolle N, Guerot E, Faisy C, et al. Renal failure in septic shock: predictive value of doppler-based renal arterial resistive index. Intensive Care Med. 2006;32(10):1553–1559.
- Schnell D, Deruddre S, Harrois A, et al. Renal resistive index better predicts the occurrence of acute kidney injury than cystatin C. Shock. 2012;38(6):592–597.
- Darmon M, Schortgen F, Vargas F, et al. Diagnostic accuracy of doppler renal resistive index for reversibility of acute kidney injury in critically ill patients. Intensive Care Med. 2011;37(1):68–76.
- Ninet S, Schnell D, Dewitte A, et al. Doppler-based renal resistive index for prediction of renal dysfunction reversibility: a systematic review and meta-analysis. J Crit Care. 2015;30(3):629–635.
- Schnell D, Reynaud M, Venot M, et al. Resistive index or color-Doppler semi-quantitative evaluation of renal perfusion by inexperienced physicians: results of a pilot study. Minerva Anestesiol. 2014;80(12):1273–1281.
- Venot M, Weis L, Clec’H C, et al. Acute kidney injury in severe sepsis and septic shock in patients with and without diabetes mellitus: a multicenter study. PLoS One. 2015;10(5):e0127411.
- Montomoli J, Donati A, Ince C. Acute kidney injury and fluid resuscitation in septic patients: are We protecting the kidney? Nephron. 2019;143(3):170–173.
- Fotopoulou G, Poularas I, Kokkoris S, et al. Renal resistive index on intensive care unit admission correlates with tissue hypoperfusion indices and predicts clinical outcome. Shock. 2022;57(4):501–507. Apr 1
- Gaiao SM, Gomes AA, Paiva JA. Prognostics factors for mortality and renal recovery in critically ill patients with acute kidney injury and renal replacement therapy. Rev Bras Ter Intensiva. 2016 Jan-Mar;28(1):70–77.
- Fu Y, He C, Bai Y, et al. [Value of the combination of renal resistive index and Central venous pressure to predict septic shock induced acute kidney injury]. Zhonghua Wei Zhong Bing Ji Jiu Yi Xue. 2020;32(4):473–477.
- Singer M, Deutschman CS, Seymour CW, et al. The third international consensus definitions for sepsis and septic shock (sepsis-3). Jama. 2016;315(8):801–810.
- Khwaja A. KDIGO clinical practice guidelines for acute kidney injury. Nephron Clin Pract. 2012;120(4):c179–84.
- Ma YC, Zuo L, Chen JH, et al. Modified glomerular filtration rate estimating equation for chinese patients with chronic kidney disease. J Am Soc Nephrol. 2006;17(10):2937–2944.
- Rhodes A, Evans LE, Alhazzani W, et al. Surviving sepsis campaign: international guidelines for management of sepsis and septic shock: 2016. Intensive Care Med. 2017;43(3):304–377.
- Dewitte A, Coquin J, Meyssignac B, et al. Doppler resistive index to reflect regulation of renal vascular tone during sepsis and acute kidney injury. Crit Care. 2012 Sep 12;16(5):R165.
- Vittinghoff E, McCulloch CE. Relaxing the rule of ten events per variable in logistic and cox regression. Am J Epidemiol. 2007;165(6):710–718.
- DeLong ER, DeLong DM, Clarke-Pearson DL. Comparing the areas under two or more correlated receiver operating characteristic curves: a nonparametric approach. Biometrics. 1988;44(3):837–845.
- Garnier F, Daubin D, Larcher R, et al. Reversibility of acute kidney injury in medical ICU patients: predictability performance of urinary tissue inhibitor of metalloproteinase-2 x Insulin-Like growth Factor-Binding protein 7 and renal resistive index. Crit Care Med. 2020;48(4):e277–e284.
- Lerolle N. Please don’t call me RI anymore; I may not be the one you think I am!. Crit Care. 2012;16(6):174.
- Deruddre S, Cheisson G, Mazoit JX, et al. Renal arterial resistance in septic shock: effects of increasing mean arterial pressure with norepinephrine on the renal resistive index assessed with doppler ultrasonography. Intensive Care Med. 2007;33(9):1557–1562. Sep
- Tublin ME, Tessler FN, Murphy ME. Correlation between renal vascular resistance, pulse pressure, and the resistive index in isolated perfused rabbit kidneys. Radiology. 1999;213(1):258–264.
- Schnell D, Camous L, Guyomarc’H S, et al. Renal perfusion assessment by renal doppler during fluid challenge in sepsis. Crit Care Med. 2013;41(5):1214–1220.
- Darmon M, Schortgen F, Leon R, et al. Impact of mild hypoxemia on renal function and renal resistive index during mechanical ventilation. Intensive Care Med. 2009;35(6):1031–1038.
- Sharkey RA, Mulloy EM, O'Neill SJ. The acute effects of oxygen and carbon dioxide on renal vascular resistance in patients with an acute exacerbation of COPD. Chest. 1999;115(6):1588–1592.
- Bude RO, Rubin JM. Relationship between the resistive index and vascular compliance and resistance. Radiology. 1999;211(2):411–417.
- Murphy ME, Tublin ME. Understanding the doppler RI: impact of renal arterial distensibility on the RI in a hydronephrotic ex vivo rabbit kidney model. J Ultrasound Med. 2000;19(5):303–314.
- Bude RO, DiPietro MA, Platt JF, et al. Age dependency of the renal resistive index in healthy children. Radiology. 1992;184(2):469–473.
- Naesens M, Heylen L, Lerut E, et al. Intrarenal resistive index after renal transplantation. N Engl J Med. 2013;369(19):1797–1806.
- Ohta Y, Fujii K, Arima H, et al. Increased renal resistive index in atherosclerosis and diabetic nephropathy assessed by doppler sonography. J Hypertens. 2005;23(10):1905–1911.
- Kirkpatrick AW, Colistro R, Laupland KB, et al. Renal arterial resistive index response to intraabdominal hypertension in a porcine model. Crit Care Med. 2007;35(1):207–213.
- Kopitko C, Medve L, Gondos T. The value of combined hemodynamic, respiratory and intra-abdominal pressure monitoring in predicting acute kidney injury after major intraabdominal surgeries. Ren Fail. 2019;41(1):150–158.
- Ostermann M, Wu V, Sokolov D, et al. Definitions of acute renal dysfunction: an evolving clinical and biomarker paradigm. Curr Opin Crit Care. 2021;27(6):553–559.
- Langenberg C, Gobe G, Hood S, et al. Renal histopathology during experimental septic acute kidney injury and recovery. Crit Care Med. 2014;42(1):e58-67–e67.
- Hoste E, Bihorac A, Al-Khafaji A, the RUBY Investigators, et al. Identification and validation of biomarkers of persistent acute kidney injury: the RUBY study. Intensive Care Med. 2020;46(5):943–953.
- Darmon M, Bourmaud A, Reynaud M, et al. Performance of doppler-based resistive index and semi-quantitative renal perfusion in predicting persistent AKI: results of a prospective multicenter study. Intensive Care Med. 2018;44(11):1904–1913.
- Zhi HJ, Li Y, Wang B, et al. Renal echography for predicting acute kidney injury in critically ill patients: a prospective observational study. Ren Fail. 2020;42(1):263–269.
- Dewitte A, Joannes-Boyau O, Sidobre C, et al. Kinetic eGFR and novel AKI biomarkers to predict renal recovery. Clin J Am Soc Nephrol. 2015;10(11):1900–1910.
- Jia HM, Cheng L, Weng YB, et al. Cell cycle arrest biomarkers for predicting renal recovery from acute kidney injury: a prospective validation study. Ann Intensive Care. 2022;12(1):14.
- Chawla L, Goldstein S, Kellum J, et al. Renal angina: concept and development of pretest probability assessment in acute kidney injury. Crit Care. 2015;19(1):93.
- Basu RK, Kaddourah A, Goldstein SL, AWARE Study Investigators Assessment of a renal angina index for prediction of severe acute kidney injury in critically ill children: a multicentre, multinational, prospective observational study. Lancet Child Adolesc Health. 2018;2(2):112–120.
- Matsuura R, Iwagami M, Moriya H, et al. A simple scoring method for predicting the low risk of persistent acute kidney injury in critically ill adult patients. Sci Rep. 2020;10(1):5726.
- Matsuura R, Srisawat N, Claure-Del Granado R, et al. Use of the renal angina index in determining acute kidney injury. Kidney Int Rep. 2018 May;3(3):677–683.
- Darmon M, Truche AS, Abdel-Nabey M, et al. Early recognition of persistent acute kidney injury. Semin Nephrol. 2019;39(5):431–441.
- Ferreira FL, Bota DP, Bross A, et al. Serial evaluation of the SOFA score to predict outcome in critically ill patients. JAMA. 2001;286(14):1754–1758.
- Beloncle F, Rousseau N, Hamel JF, et al. Determinants of doppler-based renal resistive index in patients with septic shock: impact of hemodynamic parameters, acute kidney injury and predisposing factors. Ann Intensive Care. 2019;9(1):51.
- Sharkey RA, Mulloy EM, Long M, et al. The effect of continuous positive airway pressure (CPAP) on renal vascular resistance: the influence of renal denervation. Crit Care. 1999;3(1):33–37.