Abstract
Background
Left ventricular hypertrophy is associated with adverse outcomes among peritoneal dialysis patients. The aim of this study was to evaluate the prognostic impact of baseline left ventricular hypertrophy and its relationship with baseline peritoneal transfer characteristics in peritoneal dialysis patients.
Methods
We enrolled 151 incident peritoneal dialysis patients to perform a multicentric retrospective cohort study since January 1, 2017 to January 31, 2021. Patients were grouped based on baseline dialysate-to-plasma creatinine ratio at 4 h as follows: low (<0.50), low average (0.5–0.64), high average (0.65–0.80) and high (≥0.81). Echocardiography and clinic data were recorded yearly. The Cox proportional hazards models and competing risk model were used to evaluate patients’ survival. Generalized linear mixed models were performed to explore risk factors associated with left ventricular hypertrophy.
Results
During a median follow-up period of 33 months (range, 16–48 months), 21 (13.9%) patients died, including 16 (10.60%) cardiovascular deaths. Controlling the competing risks of switching to hemodialysis, kidney transplantation and loss to follow-up, baseline left ventricular hypertrophy was an independent risk factor for all-cause mortality (subdistribution hazard ratio, 2.645; 95% confidence interval, 1.156–6.056; p = 0.021). Baseline high and high average transport status were positively related to left ventricular mass index and left atrium diameter 2 years after PD initiation.
Conclusion
Baseline fast peritoneal solute transport rate may be an effect factor for aggravating left ventricular hypertrophy which predicted poor outcomes for peritoneal dialysis patients. The findings offered important ideas for further prospective intervention study.
Introduction
Cardiovascular disease (CVD) is a common complication and has been considered as an important reason of death in patients with maintenance dialysis. Compared with hemodialysis (HD), peritoneal dialysis (PD) may have an advantage of survival in a relatively early period [Citation1,Citation2]. Nonetheless, approximately 1–2 years after PD initial, the patient’s survival often be comparable to or even poorer than HD [Citation1,Citation3]. A prospective cohort study demonstrated that patients with heart failure who initially received PD therapy had a lower survival rate than those who received HD [Citation4]. These deleterious consequences could be explained partly by altered peritoneal transport patterns occurring over time.
Long-term success of PD is depended on the ability of the peritoneal membrane to provide adequate solute clearances and ultrafiltration over time. Peritoneal solute clearances is described as peritoneal solute transport rate (PSTR), which can be measured by the peritoneal equilibration test (PET) through dialysate-to-plasma (D/P) ratios of low-molecular-weight solutes [Citation5]. Ultrafiltration failure is one of the consequences of fast solute transportation, which is the main limitation of long-term PD treatment. Fast PSTR accompanied by a rapid disappearing glucose osmosis gradient and increasing in protein loss, ultimately step in fluid overload and malnutrition [Citation6]. Therefore, cardiovascular prognosis of patients with fast PSTR at baseline is more worthy of attention.
Left ventricular hypertrophy (LVH) is a major risk factor for cardiovascular complications and death in end stage kidney disease (ESKD) patients [Citation7]. The prevalence of LVH is about 75% in patients with dialysis [Citation8,Citation9]. LVH and left atrium (LA) dilation now are risk factors closely tied to worse survival and cardiovascular outcomes [Citation10,Citation11]. Due to its convenience, echocardiography was a widespread way to evaluate cardiac structure and function noninvasively in PD patients. Adjusted with body surface area, left ventricular mass index (LVMI) can be an individualized way to assess left ventricular geometry and myocardial systolic and diastolic functions as well as their alternations in the follow-up visit. In previous studies, baseline fast peritoneal transport status was considered closely related to the increase of CVD occurrence in PD patients [Citation12–14].
This present study focused on both baseline PSTR and echocardiography parameters, determining whether baseline fast PSTR and LVH could provide complementary predictive information on prognosis in PD patients in multi-center. Meanwhile, we detected the association between peritoneal transfer characters and LVH at the early stage of PD therapy.
Materials and methods
Study design and study population
The present study was a multicenter retrospective cohort study which recruited all the patients, aged between 18 and 75 years, who initiated continuous ambulatory peritoneal dialysis (CAPD) in four medical centers (Shanghai East Hospital Affiliated to Tongji University School of Medicine, Baoshan Branch of Shanghai First People’s Hospital, Shanghai Songjiang District Central Hospital and Qingpu Branch of Zhongshan Hospital Affiliated to Fudan University) since January 1st, 2017 to January 1st, 2019, and followed up until January 31st, 2021. All patients initiated CAPD with 1.5% or 2.5% dextrose PD fluid (Baxter Healthcare, Guangzhou, China), daily dialysis dose ranged from 6000 to 10000 mL. All the participants underwent a PET after the first month of PD treatment. Patients were excluded if they lacked complete echocardiography data, had previously received HD or kidney transplantation, had a history of malignant tumor, liver cirrhosis, active tuberculosis, acute myocardial infarction, severe heart valve lesions or peritonitis at the baseline. These enrolled patients were followed until cessation of PD, death, or on January 31st, 2021.
Demographic and clinical data
Baseline demographic, medication history and clinical data were collected after the first month of PD treatment. Uncontrolled hypertension was defined as BP ≥140/90 mmHg. CVD included previous and present history of congestive heart failure, ischemic heart disease, or cerebrovascular disease. Baseline laboratory information at the first months after the initiation of CAPD, including hemoglobin (HB), serum albumin (ALB), high-sensitivity C-reactive protein (hs-CRP), corrected serum calcium (cSCa), phosphate (P), parathyroid hormone (PTH), uric acid (UA), total cholesterol (TC), triglyceride (TG), low-density lipoprotein (LDL) and high-density lipoprotein (HDL). The PD adequacy and residual renal function (RRF) were assessed by peritoneal and kidney Kt/V urea (urea clearance index) using data from 24-h dialysate and urine collections.
Peritoneal equilibration test
According to Twardowski [Citation5], a standard 4-h dwell period was used to perform PET test. Using 2.5% dextrose PD solution for a 2 L volume exchange, after 2.5% dextrose PD solution dwelling overnight (8–12 h). PD effluent sample was collected at 0, 2, 4 h of dwell and venous blood sample at 2 h, respectively. Both dialysate and plasma glucose and creatinine were measured. According to dialysate-to-plasma (D/P) creatinine ratio at 4 h (4 h D/P Cr), the patients were classified into four groups of peritoneal transport characteristics: low (L, < 0.50); low-average (LA, 0.50–0.64); high-average (HA, 0.65–0.80); high (H, > 0.81). The first PET test was defined as baseline data.
Echocardiography
We collected echocardiography data of the first two consecutive years since PD initial. Comprehensive examination data of transthoracic two-dimensional echocardiography were collected by using Philips EpiQ-7 (Koninklijke Philips N.V., Netherlands) equipped with a multifrequency transducer (S5-1, 5 MHz). All images were obtained with standard techniques using M-mode, two-dimensional, and Doppler measurements in accordance with the American Society of Echocardiography guidelines (ASE). Echocardiographic measurements included left atrium diameter (LAD), left ventricular end-diastolic dimension (LVEDD), interventricular septum thickness (IVST) and posterior wall thickness (PWT). LV ejection fraction (LVEF) was calculated using the modified biplane Simpson’s method in apical two-chamber and four-chamber views. LVEF > 55% was defined as normal ventricle systolic function. Left ventricular mass (LVM) was calculated using the modification by Devereux et al. of the ASE cube formula: 0.8 × (1.04 × [LVEDd + IVST + PWT]Citation3 − LVEDdCitation3) + 0.6 [Citation15]. LVMI was calculated according to the formula from linear dimensions and indexed to body surface area calculated using the formula: 0.0001 × 71.84 × (weight [kg])0.25 × (height [cm])0.725[Citation16]. LVH was defined as LVMI > 131 g/m2 for men and > 100 g/m2 for women. LA enlargement(LAE) defined as LAD > 40 mm for men and LAD > 38 mm for women [Citation17].
Outcomes
The primary outcome was all-cause mortality, and the secondary one was cardiovascular mortality. For mortality analyses, patients were censored at the time of switching to HD treatment, kidney transplantation, lost to follow-up or the end of the study period (January 31st, 2021).
Statistical analysis
Results were expressed as absolute number (percentage) for categorical variables, mean ± SD for continuous data and median (interquartile range) for nonnormally distributed continuous variables. Data distribution normality was evaluated by the Kolmogorov–Smirnov test. A comparison among the different peritoneal transport types was performed by one-way ANOVA (parametric distribution) or the Kruskal-Wallis test (non-parametric distribution), chi-square test was applied to compare categorical data. Linear regression analysis was used to definite the change slope of LVMI and LAD, the slope was expressed as the regression coefficient. Depending on whether the slope value is greater than 0, the changing trends of echocardiography parameters were differentiated as ‘rise’ or ‘fall’.
The Kaplan–Meier survival curves were drawn for each event of interest (overall death and cardiovascular death) and the log-rank test was used to compare curves. Univariate and multivariate Cox proportional hazards models were used to search significant risk factors associated with study outcomes, and group of baseline low average transporters was defined as reference. We used Z-score method to approximate normal data distribution. Variables that were statistically significant with a p-value < 0.2 in univariate Cox models were further assessed with multivariate Cox models using a forward stepwise method. The results were presented as adjusted hazard ratios (AHRs) with 95% confidence intervals (CIs). The Fine-Gray subdistribution hazards model was used to evaluate competing risks of censor events. The results were presented as subdistribution hazard ratios (SHRs) with 95% CIs. Generalized linear mixed models were used to explore risk factors associated with the elevated echocardiography parameters 2 years after PD initiation. All statistical analyses were performed by using SPSS software, version 23.0 (IBM SPSS, Chicago, IL, USA) and Stata/MP version17.0. A two-tailed p-value < 0.05 was considered statistically significant.
Results
Baseline characteristics of the entire cohort
The flow chart of patients for the study was shown in . 151 incident PD patients were enrolled into this retrospective study. The median follow-up time was 33 months (range, 16–48 months). The median age was 61 years (range, 52–68 years) old, and there were 82 males (54.30%). All patients were categorized by their baseline peritoneal transport status, 31 patients (20.53%) were classified as high peritoneal transporters (H group), 65 patients (43.05%) as high-average transporters (HA group), 46 patients (30.46%) as low-average transporters (LA group), and 9 patients (5.90%) as low transporters (L group). Compared with L and LA groups, less patients in group HA and H had their BP controlled (only 15.4% and 12.9%, respectively). Albumin levels in H group seemed to be significantly lower than in other groups while UA and cSCa levels was the opposite. The baseline characteristics of the participants were listed in .
Figure 1. Flow chart of the participants in the study cohort. KT: kidney transplant; PD: peritoneal dialysis; HD: hemodialysis.
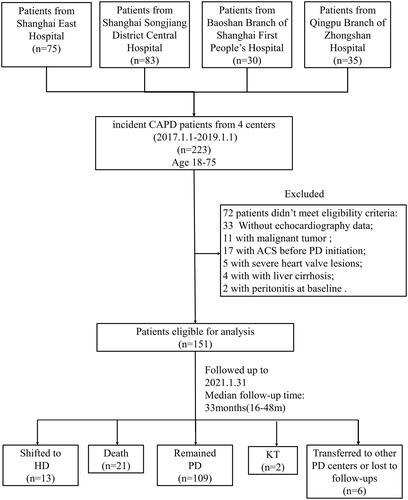
Table 1. Baseline clinical parameters of 151 patients on continuous ambulatory peritoneal dialysis.
Echocardiographic characteristics of the entire cohort
Echocardiographic parameters at baseline and two-year follow-up were presented in . Regarding LA group as the reference, only baseline LAD was significantly higher in H group. While during the 2-year follow-up period, multiple echocardiographic parameters were found to be significantly altered in H group which presented with higher value of heart chamber size such as LVESV, LVEDD and LAD as well as progressive cardiac hypertrophy accompanied by deterioration of the left ventricular ejection fraction. Similarly, the same changes were also shown in HA group in 2nd year. To show the changes more visual, variation of echocardiographic parameters about LVH was described as the slope of line regression based on the follow-up data. There were 94 (62.3%) and 80 (53.0%) patients had their LVMI and LAD elevated 2 years after PD initiation respectively. In comparison with LA group, the slopes of LVMI and LAD in H group indicated statistically differences as shown in .
Table 2. Baseline and follow-up visit clinical echocardiographic parameters of 151 patients on continuous ambulatory peritoneal dialysis.
Patient survival and cardiovascular survival
In this retrospective study, all-cause death in 21 (13.90%) and cardiovascular death in 16 (10.60%) subjects were observed. Details on all-cause and cardiovascular deaths were listed in Supplementary Table 1. Cumulative overall survival at 1, 2, 3 and 4 year was 100%, 98.0%, 84.5% and 74.6%, respectively, cumulative cardiovascular survival was 100%, 99.3%, 88.9% and 78.5%, respectively. Compared with the other groups, patient overall survival was significantly decreased in H group (p < 0.01) (). A higher cardiovascular mortality was also observed in H group (p < 0.01) (). Based on baseline LVMI, patients were divided into LVH and non-LVH group. It was noticed that patients in LVH group had higher all-cause mortality, but no significant statistical difference in CV survival. (p = 0.022 and p = 0.065, respectively) (). Similarly, patients were divided into LAE and non-LAE group based on baseline LAD. A significant decreased overall survival was observed in LAE group (p = 0.017) (), while CV survival did not differ between the two groups. (p = 0.434) ().
Figure 2. Kaplan-Meier analysis of overall survival and cardiovascular survival of the participants. (A) The overall survival of participants classified according to the type of peritoneal transportation. (B) The cardiovascular survival of the participants classified according to the type of peritoneal transportation. (C) The overall survival of the participants classified according to the diagnostic criteria for LVH by echocardiography. (D) The cardiovascular survival of the participants classified according to the diagnostic criteria for LVH by echocardiography. (E) The overall survival of the participants classified according to the diagnostic criteria for LAE by echocardiography. (F) The cardiovascular survival of the participants classified according to the diagnostic criteria for LAE by echocardiography. L: low; LA: low-average; HA: high-average; H: high; LVH: left ventricular hypertrophy; LAE: left atrial enlargement.
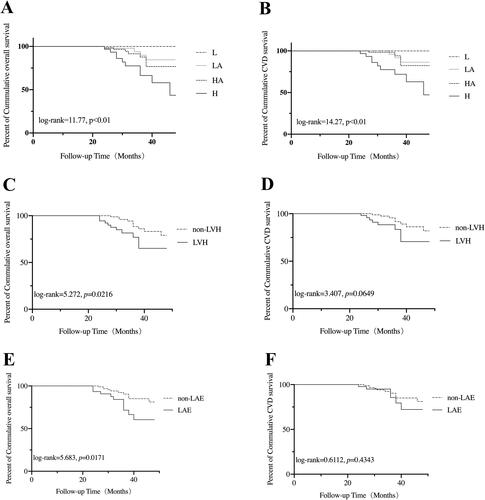
We performed univariate and multivariate Cox proportional hazard regression analysis to assess the risk factors for all-cause mortality. In the univariate model, baseline H transport status (p = 0.015) and hemoglobin (p = 0.036) was associated with all-cause mortality. When LVMI and LAD was converted to a binary classification variable according to the diagnostic criteria of LVH and LAE by echocardiography, we found LVH (p = 0.028) and LAE (p = 0.023) were risk factors for all-cause mortality. Similarly, the univariate Cox regression analysis also showed positive relationship between baseline H transport status and cardiovascular mortality (p = 0.014). Then, a multivariate Cox model was constructed. Baseline LVH predicted an increased risk of all-cause death (AHR, 3.716; 95% CI, 1.432–9.640; p = 0.007) and cardiovascular death (AHR, 3.923; 95% CI, 1.224–12.567; p = 0.021). Meanwhile, hemoglobin appeared predicting a decreased risk of all-cause death (AHR, 0.971; 95% CI, 0.946–0.997; p = 0.026) and cardiovascular death (AHR, 0.962; 95% CI, 0.934–0.992; p = 0.013). Baseline H transport status and LAE were no longer significant for outcome events in multivariate analysis.
Considered that switching to HD, kidney transplantation and lost to follow-up events had competing risks, Fine and Gray’s hazard model was also used for the competing risk analyses. After controlling competing risk events above, baseline LVH was still significantly risk factor for all-cause death (SHR, 2.645; 95% CI, 1.156–6.056; p = 0.021; SHR, 2.526; 95% CI, 1.097–5.817; p = 0.029 and SHR, 2.625; 95% CI, 1.144–6.027; p = 0.023, respectively). All the details were shown in .
Table 3. Predictors of overall and CVD mortality in 151 PD patients based on the results of Cox regression analysis and competing risk models.
Risk factor of LVH
To explore the underlying risk factor of LVH and LAE, correlation analysis was conducted between baseline clinic data and the rise slope of LVMI as well as LAD. As shown in , baseline 4 h D/P Cr levels showed a positive linear correlation with the rise slope of both LVMI () and LAD () (p < 0.0001 and p = 0.021, respectively).
Figure 3. Scatter plot for correlation between baseline data and the rise slope of LVMI and LAD. (A) Baseline 4 h D/P Cr and the rise slope of LVMI. (B) Baseline 4 h D/P Cr and the rise slope of LAD. 4 h D/P Cr: dialysate/plasma creatinine at 4 h; LVMI: left ventricular mass index; LAD: left atrial diameter.
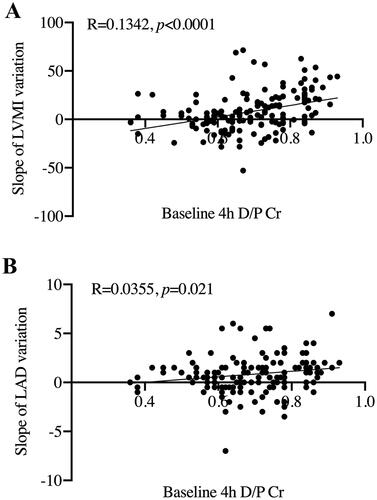
Since this was a multicenter study, multivariate analysis was performed by using a generalized linear mixed model to evaluate the association between baseline clinic data and LVMI and LAD value 2 years after PD initiation. The results were showed in . baseline H and HA transport status was closely related to elevated LVMI (59.798 ± 12.15, p < 0.001 and 29.038 ± 8.76, p = 0.001, respectively) and LAD (11.316 ± 4.01, p = 0.005 and 6.424 ± 2.21, p = 0.004, respectively) 2 years after PD initiation. Apart from this, HbA1c levels showed negative association with LVMI (-7.994 ± 3.87, p = 0.042), Serum hs-CRP concentration showed positive associations with the value of LAD (0.125 ± 0.06, p = 0.038). Inverse correlation was also observed between LAD and non-diabetes (-3.532 ± 1.11, p = 0.002).
Table 4. Generalized linear mixed model (LVMI and LAD value as dependent variables after 2 years of PD initiation).
Discussion
The current study highlighted the assessment of the prognoses of PD patients by the combination of baseline peritoneal transport characteristic with echocardiography parameters. We demonstrated that patients with LVH estimated by echocardiography at the initiation of PD may have worse prognosis.
As we known, LVH is common among patients with CKD or on dialysis. In a retrospective cohort study in Japan, concentric LVH at PD initiation was demonstrated as an independent risk factor for mortality (HR, 3.32; 95% CI, 1.13–9.70; p < 0.001) [Citation18]. That conclusion was consistent with our result and the prevalence of baseline LVH in that cohort was even lower than ours (22% vs 36%). Some researchers suggested that LVH was the predominant characteristic of uremic cardiomyopathy which was often associated with diffuse myocardial fibrosis [Citation19,Citation20]. The uremic form of chronic cardiac fibrosis presents with uniform intramyocardial fibrosis, which is not the same as the endocardial to epicardial perivascular fibrosis seen in patients with hypertension and ischemic cardiomyopathies [Citation21]. Histological features of endomyocardial biopsies in patients undergoing dialysis mainly included myocyte hypertrophy and diffuse fibrosis [Citation22,Citation23]. Diffuse myocardial fibrosis was associated with greater LV mass but not with risk factors for coronary atheroma, LV systolic dysfunction or angiographic coronary artery disease [Citation20]. However, LV remodeling occur early during CKD. In a cross-sectional echocardiographic study of 3,487 patients, the detection rate of LVH could reach 32%, 48%, 57% and 75% for estimated GFR (eGFR) categories ≥60, 45 to 59, 30 to 44, and <30 mL/min/1.73 m2, respectively [Citation9]. Therefore, we have reasons to believe that treatment should be focused on the earlier phases of CKD to prevent the progression of LVH.
LAE is closely related to LVH and diastolic dysfunction [Citation24]. Several studies have demonstrated that left atrial volume index (LAVI) is an independent predictor of mortality in dialysis populations [Citation25–28]. A prospective cohort study proved that changes in left atrial volume (LAV) had an independent association with cardiovascular outcomes (HR [1-mL/m2.7 per yr increase in LAV] 1.12; 95% CI, 1.05–1.20; p < 0.001), and the predictive ability was independent of left ventricular mass [Citation29]. LA size as a predictor of adverse cardiovascular events may be associated with atrial fibrillation, acute myocardial infarction, heart failure, and cerebrovascular events [Citation11]. In our study, LAE did not show a significant relationship with patients’ survival in the multivariate analysis, it still needed to be explored in larger samples.
The multivariate Cox regression model also showed the risk of all-cause and cardiovascular mortality decreasing with the rising hemoglobin. Considering the relatively high number of censored events competing for the primary outcome, no consistent conclusions have been drawn in competing risk models. However, numerous studies have identified anemia as an important risk factor for survival in dialysis patients [Citation30,Citation31]. Anemia is also proved to be a risk factor for LVH in both dialysis patients and non-dialysis CKD patients [Citation32].
As in our results, baseline H transporters were found to exhibit an increased risk of overall and cardiovascular mortality in univariate analysis. Baseline H and HA transport status were closely associated with LVH and LAE in early stage of PD. Recently, Theerasak et al. retrospectively reviewed data on prevalent PD patients with repeat echocardiograms and extracellular water (ECW) measured by multifrequency bioelectrical impedance. They reported that ECW/height was independently associated with the percent change in LVMI (OR, 1.25; 95% CI, 1.08–1.36; p = 0.007), volume expansion was a more significant factor in determining LVH than blood pressure [Citation33]. This result can also partly explain our findings. Fast transporters rapidly absorb dialysate glucose, leading to early eliminate of the osmotic gradient between dialysate and blood that is required to sustain ultrafiltration. Once the osmotic gradient is dissipated, the stimulus for ultrafiltration is gone, and ultrafiltration ceases. Therefore, patients with fast PSTR were more prone to develop into poor net ultrafiltration, low drain volume, and potential systemic volume expansion. Another disadvantage of fast PSTR was leading more and faster glucose absorption from higher concentrations of dialysate dextrose, which bring no benefits for patients to maintain good ventricular function. Hassan et al. reported that fluid overload, mean arterial pressure and mean LVMI were higher in patients treated with high peritoneal glucose dialysate group, whereas 24 h ultrafiltration, weekly Kt/V, serum albumin levels and RRF were better in low peritoneal glucose dialysate group [Citation34]. Based on these findings, our results showed the value of baseline fast PSTR in predicting adverse outcomes due to its effects in promoting LVH and LAE. Actually, fast PSTR was also associated with many other adverse complications. Hu et al. recently reported that a higher baseline peritoneal transport status were risk factors for the first episode of peritonitis [Citation35]. Therefore, peritoneal transport state may be a prognostic indicator as well as a therapeutic target for PD patients.
We also detected other clinical cardiovascular risk factors. High hs-CRP level was found to be a predictor of elevated LAD. Chronic inflammatory processes are common in ESKD. It is considered that CRP appears in every inflammatory state and is a sensitive biomarker of inflammation. In PD situation, many factors such as PD-associated peritonitis, long-term exposure to the bioincompatible solution, loss of RRF, fluid overload, and comorbid conditions like diabetes mellitus can increase chronic inflammation states. CRP has been widely proposed as a risk factor and an indicator of cardiovascular disease [Citation36]. There is a strong association between the degree of CRP reduction and the corresponding reduction in CVD risk [Citation37]. Chen et al. performed a retrospective cohort study included 574 dialysis patients (HD 347, PD 227) with median follow‐up of 3.5 years. They concluded that higher CRP quintiles were associated with increased risk of major adverse cardiovascular events in PD patients (p = 0.002) only, but not in hemodialysis patients [Citation38]. Furthermore, higher HbA1c and non-diabetes patients were less associated with LVMI and LAD enlargement in our study. DM appeared to predict the risk of fatal cardiovascular events and all-cause mortality in PD patients [Citation39]. But some dialysis patients tend to have false low HbA1c levels due to anemia and erythropoiesis-stimulating agents (ESAs) use. Actually, poor glycemic control and very low HbA1c levels attributed to poor nutritional status were associated with an increased mortality risk [Citation40]. Due to the routine use of glucose-based fluids, glucose load is markedly higher in PD compared to HD patients. Novel non-glucose PD solutions have been reported to reduce cardiovascular events [Citation41,Citation42].
To the best of our knowledge, the studies focusing on the combination value of peritoneal transport characteristic and echocardiography for mortality risk are limited. This study particularly links baseline peritoneal transport characteristic and the changes in echocardiography together to provide a noninvasive assessment of all-cause and cardiovascular mortality risk in PD patients.
Limitations
There are some limitations in this study. First, this was a retrospective study with a limited sample size. Some of the clinical data in the hospital recording system was incomplete. There were 33 (14.8%) patients be excluded due to lack of complete echocardiographic data. Therefore, the potential selection bias and confounding bias was inevitable. Secondly, although we further analyzed and compared slopes of echocardiography parameters changes in patients, only baseline PET results were enrolled in regression analysis, which did not cover the detail longitudinal D/P, Kt/V as well as RRF changes and their relations between echocardiography parameters variation during the observation period. In view of these confounding factors, additional larger multi-center studies are required to explore the correlation between peritoneal transfer characteristic and cardiac structure changes. Early intervention studies are urgently needed to improve the survival of PD patients.
Conclusions
Baseline fast peritoneal solute transport rate may be an aggravating factor for left ventricular hypertrophy and predict poor outcomes for peritoneal dialysis patients. The findings offered that echocardiographic may be a useful adjunct for further prospective intervention trials in dialysis patients.
Statement of ethics
This study was conducted according to the guidelines of the Helsinki Declaration and written informed consents were obtained from all patients. The study protocol was approved by human Research Ethics Committee of Shanghai East Hospital Affiliated to Tongji University School of Medicine, Baoshan Branch of Shanghai First People’s Hospital, Shanghai Songjiang District Central Hospital and Qingpu Branch of Zhongshan Hospital Affiliated to Fudan University (ChiCTR2000030053).
Author contributions
Na Liu designed the study. Yi Wang, Guansen Huang, Xiaoyan Ma, Xiujuan Zang, Shoujun Bai, Yakun Wang, Lin Du, Zexin Lv, Jinqing Li, Hui Chen, Yan Hu, Yingfeng Shi, Xun Zhou, Min Tao performed data collection. Yi Wang, Guansen Huang, Min Tao, Na Liu performed statistical analysis. Yi Wang, Guansen Huang, Na Liu, Shougang Zhuang wrote or contributed to the writing of the manuscript. All authors have read and approved the final version of the manuscript.
Supplemental Material
Download PDF (22 KB)Acknowledgements
We acknowledge and appreciate our colleagues for their valuable efforts and comments on this paper.
Disclosure statement
The authors have read and understood Renal Failure’s policy on disclosing conflicts of interest and declare that authors have none.
Additional information
Funding
References
- McDonald SP, Marshall MR, Johnson DW, et al. Relationship between dialysis modality and mortality. J Am Soc Nephrol. 2009;20(1):155–163.
- Mehrotra R, Devuyst O, Davies SJ, et al. The current state of peritoneal dialysis. J Am Soc Nephrol. 2016;27(11):3238–3252.
- Liem YS, Wong JB, Hunink MG, et al. Comparison of hemodialysis and peritoneal dialysis survival in The Netherlands. Kidney Int. 2007;71(2):153–158.
- Stack AG, Molony DA, Rahman NS, et al. Impact of dialysis modality on survival of new ESRD patients with congestive heart failure in the United States. Kidney Int. 2003;64(3):1071–1079.
- Twardowski ZJ. PET–a simpler approach for determining prescriptions for adequate dialysis therapy. Adv Perit Dial. 1990;6:186–191.
- Chung SH, Heimbürger O, Lindholm B. Poor outcomes for fast transporters on PD: the rise and fall of a clinical concern. Semin Dial. 2008;21(1):7–10.
- Paoletti E, Specchia C, Di Maio G, et al. The worsening of left ventricular hypertrophy is the strongest predictor of sudden cardiac death in haemodialysis patients: a 10 year survey. Nephrol Dial Transplant. 2004;19(7):1829–1834.
- Stewart GA, Gansevoort RT, Mark PB, et al. Electrocardiographic abnormalities and uremic cardiomyopathy. Kidney Int. 2005;67(1):217–226.
- Park M, Hsu CY, Li Y, Chronic Renal Insufficiency Cohort (CRIC) Study Group, et al. Associations between kidney function and subclinical cardiac abnormalities in CKD. J Am Soc Nephrol. 2012;23(10):1725–1734.
- Stewart MH, Lavie CJ, Shah S, et al. Prognostic implications of left ventricular hypertrophy. Prog Cardiovasc Dis. 2018;61(5-6):446–455.
- Bouzas-Mosquera A, Broullón FJ, Álvarez-García N, et al. Left atrial size and risk for all-cause mortality and ischemic stroke. CMAJ. 2011;183(10):E657–64.
- Krediet RT, Balafa O. Cardiovascular risk in the peritoneal dialysis patient. Nat Rev Nephrol. 2010;6(8):451–460.
- Rumpsfeld M, McDonald SP, Johnson DW. Higher peritoneal transport status is associated with higher mortality and technique failure in the Australian and New Zealand peritoneal dialysis patient populations. J Am Soc Nephrol. 2006;17(1):271–278.
- Churchill DN, Thorpe KE, Nolph KD, et al. Increased peritoneal membrane transport is associated with decreased patient and technique survival for continuous peritoneal dialysis patients. The Canada-USA (CANUSA) Peritoneal dialysis study group. J Am Soc Nephrol. 1998;9(7):1285–1292.
- Devereux RB, Alonso DR, Lutas EM, et al. Echocardiographic assessment of left ventricular hypertrophy: comparison to necropsy findings. Am J Cardiol. 1986;57(6):450–458.
- Burton BT, Foster WR. Health implications of obesity: an NIH consensus development conference. J Am Diet Assoc. 1985;85(9):1117–1121.
- Lang RM, Bierig M, Devereux RB, European Association of Echocardiography, et al. Recommendations for chamber quantification: a report from the American society of echocardiography’s guidelines and standards committee and the chamber quantification writing group, developed in conjunction with the european association of echocardiography, a branch of the european society of cardiology. J Am Soc Echocardiogr. 2005;18(12):1440–1463.
- Tomura M, Hamasaki Y, Komaru Y, et al. Prognostic significance of concentric left ventricular hypertrophy at peritoneal dialysis initiation. BMC Nephrol. 2021;22(1):135. M
- Edwards NC, Moody WE, Chue CD, et al. Defining the natural history of uremic cardiomyopathy in chronic kidney disease: the role of cardiovascular magnetic resonance. JACC Cardiovasc Imaging. 2014;7(7):703–714.
- Mark PB, Johnston N, Groenning BA, et al. Redefinition of uremic cardiomyopathy by contrast-enhanced cardiac magnetic resonance imaging. Kidney Int. 2006;69(10):1839–1845.
- Ham O, Jin W, Lei L, et al. Pathological cardiac remodeling occurs early in CKD mice from unilateral urinary obstruction, and is attenuated by enalapril. Sci Rep. 2018;8(1):16087.
- Yoshizawa S, Uto K, Nishikawa T, et al. Histological features of endomyocardial biopsies in patients undergoing hemodialysis: comparison with dilated cardiomyopathy and hypertensive heart disease. Cardiovasc Pathol. 2020;49:107256.
- Aoki J, Ikari Y, Nakajima H, et al. Clinical and pathologic characteristics of dilated cardiomyopathy in hemodialysis patients. Kidney Int. 2005;67(1):333–340.
- Cuspidi C, Negri F, Sala C, et al. Association of left atrial enlargement with left ventricular hypertrophy and diastolic dysfunction: a tissue doppler study in echocardiographic practice. Blood Press. 2012;21(1):24–30.
- Tripepi G, Benedetto FA, Mallamaci F, et al. Left atrial volume in end-stage renal disease: a prospective cohort study. J Hypertens. 2006;24(6):1173–1180.
- Ozdogan O, Kayikcioglu M, Asci G, et al. Left atrial volume predicts mortality in low-risk dialysis population on long-term low-salt diet. Am Heart J. 2010;159(6):1089–1094.
- Patel RK, Jardine AG, Mark PB, et al. Association of left atrial volume with mortality among ESRD patients with left ventricular hypertrophy referred for kidney transplantation. Am J Kidney Dis. 2010;55(6):1088–1096.
- Kim SJ, Han SH, Park JT, et al. Left atrial volume is an independent predictor of mortality in CAPD patients. Nephrol Dial Transplant. 2011;26(11):3732–3739.
- Tripepi G, Benedetto FA, Mallamaci F, et al. Left atrial volume monitoring and cardiovascular risk in patients with end-stage renal disease: a prospective cohort study. J Am Soc Nephrol. 2007;18(4):1316–1322.
- Molnar MZ, Mehrotra R, Duong U, et al. Association of hemoglobin and survival in peritoneal dialysis patients. Clin J Am Soc Nephrol. 2011;6(8):1973–1981.
- Palaka E, Grandy S, van Haalen H, et al. The impact of CKD anaemia on patients: incidence, risk factors, and clinical Outcomes-A systematic literature review. Int J Nephrol. 2020;2020:7692376.
- Eckardt KU, Scherhag A, Macdougall IC, et al. Left ventricular geometry predicts cardiovascular outcomes associated with anemia correction in CKD. J Am Soc Nephrol. 2009;20(12):2651–2660.
- Tangwonglert T, Davenport A. Changes in extracellular water and left ventricular mass in peritoneal dialysis patients. Kidney Res Clin Pract. 2021;40(1):135–142.
- Hassan K, Hassan F, Hassan D, et al. Does low peritoneal glucose load protect from the development of left ventricular hypertrophy in peritoneal dialysis patients? Clin Exp Nephrol. 2016;20(5):770–777.
- Hu J, Zhang H, Yi B. Peritoneal transport status and first episode of peritonitis: a large cohort study. Ren Fail. 2021;43(1):1094–1103.
- Sahebkar A. Effect of L-carnitine supplementation on circulating C-reactive protein levels: a systematic review and meta-analysis. J Med Biochem. 2015;34(2):151–159.
- Ridker PM, Danielson E, Fonseca FA, JUPITER Trial Study Group, et al. Reduction in C-reactive protein and LDL cholesterol and cardiovascular event rates after initiation of rosuvastatin: a prospective study of the JUPITER trial. Lancet. 2009;373(9670):1175–1182.
- Chen T, Hassan HC, Qian P, et al. High-Sensitivity troponin T and C-Reactive protein have different prognostic values in hemo- and peritoneal dialysis populations: a cohort study. J Am Heart Assoc. 2018;7(5):1–13.
- Zhang J, Lu X, Li H, et al. Risk factors for mortality in patients undergoing peritoneal dialysis: a systematic review and meta-analysis. Ren Fail. 2021;43(1):743–753.
- Ramirez SP, McCullough KP, Thumma JR, et al. Hemoglobin A(1c) levels and mortality in the diabetic hemodialysis population: findings from the dialysis outcomes and practice patterns study (DOPPS). Diabetes Care. 2012;35(12):2527–2532.
- Wang IK, Lin CL, Yen TH, et al. Icodextrin reduces the risk of congestive heart failure in peritoneal dialysis patients. Pharmacoepidemiol Drug Saf. 2018;27(4):447–452.
- Wang IK, Tsai TH, Chang SS, et al. Icodextrin is associated with a lower risk of atrial fibrillation in peritoneal dialysis patients. Nephrology (Carlton). 2019;24(12):1273–1278.