Abstract
The type of hemodialysis access and its preservation impact the quality of life and survival of patients undergoing hemodialysis. Vascular access complications are among the top causes of morbidity, hospitalization, and catheter use, with significant economic burden. Poor maturation and stenosis continue to be key impediments to upper arm arteriovenous fistula feasibility. Cephalic arch is a common location for vascular access dysfunction due to its distinctive anatomy, complex valves, and biochemical alterations attributable to renal failure. Understanding cephalic arch stenosis is critical due to its high prevalence and treatment failure. The appropriate management option is highly debatable and mostly dependent on patient characteristics and interventionist’s preference. Current options include, percutaneous transluminal balloon angioplasty, stent grafts, bare metal stents, cutting balloon angioplasty, endovascular banding, and surgical procedures. This article discusses the etiologies of cephalic arch stenosis as well as currents trends in management including endovascular and surgical options.
Introduction
Chronic kidney disease CKD is associated with a poor prognosis, including cardiovascular complications and early mortality [Citation1]. Renal replacement therapy either in the form of hemodialysis or peritoneal dialysis is considered a lifesaving treatment, available to patients with end stage renal disease (ESRD) and can serve as bridge to renal transplantation. Patients with end stage renal disease undergoing hemodialysis require adequate vascular access to maintain their quality of life and improve survival. The type of hemodialysis access and its preservation greatly influence the survival and quality of life of hemodialysis patients.
Complications related to vascular access are among the leading causes of morbidity, hospitalization, catheter use in dialysis patients with nearly $2 billion cost burden to the US health care system [Citation2]. Vascular access sites can be an arteriovenous fistula (AVF), arteriovenous graft (AVG), or a central venous catheter (CVC). AVF is primarily the preferred type of vascular access in patients undergoing HD due to their durability and lower risk of infection when compared to other vascular accesses [Citation3]. The National Kidney Foundation adjures the use of arteriovenous fistulas rather than grafts in HD patients [Citation4].
The radiocephalic (RCF) and brachiocephalic fistulas (BCF) are the commonly used upper arm hemodialysis fistulas access. RCF () is regarded as the first choice for hemodialysis access as it has reliable patency, low complication rate and the ability to preserve other potential access points in the future [Citation5]. An important limitation of RCF is the high primary failure rate of about 15% at one year following fistula placement [Citation6]. This is due to early thrombosis and non-maturation, especially in elderly and in patients with underlying vascular disease such as diabetes [Citation7,Citation8]. BCF () access has relatively higher maturation and patency rates than the RCF and are the most common access used in HD in the United States [Citation9]. In fact, the kidney disease outcomes quality initiative (KDOQI) recommendations favor BCF however, its placement precludes future distal access placement and has high rates of steal syndrome [Citation5]. Therefore, placement is only considered as initial access only in patients with unsuitable vessels or in those with failed RCF.
Figure 2. Anatomy of the cephalic arch [Citation10].
![Figure 2. Anatomy of the cephalic arch [Citation10].](/cms/asset/67617a30-e09c-4a31-9331-c2b4201a8bbd/irnf_a_2176166_f0002_c.jpg)
Cephalic arch is a common site of vascular access dysfunction because of its unique morphology, anatomical characteristics, multiple valves and various biochemical changes associated with renal failure [Citation10]. Poor maturation and stenosis remain major limitations to upper arm AVF viability, especially in the RCF [Citation8]. The mean number of years to development of CAS is estimated at 2.93 ± 1.93 years [Citation7]. Preoperative planning with doppler ultrasound has been suggested [Citation8]. However, there is evidence that clinical assessment in the absence of imaging is sufficient, with no significant differences in surgical outcomes and fistula survival [Citation8,Citation11]. The management of CAS has been particularly difficult due to higher rates of resistance to angioplasty.
The purpose of the present review is to discuss the anatomy, etiologies, as well as the various endovascular and surgical treatment options available for the management of cephalic arch stenosis (CAS).
Epidemiology of cephalic arch stenosis
The reported incidence and prevalence of CAS is highly variable in literature, ranging from 4.25–64% [Citation12–14]. The prevalence also depends on the upper extremity fistula location with the brachiocephalic fistula (BCF) more commonly involved relative to the radiocephalic fistula (RCF) [Citation13–16]. The true incidence may be poorly represented due to patient selection, as clinically significant CAS is likely to be diagnosed while subclinical cases go undetected. Furthermore, there may be institutional differences in the recommendation and choices of the location of the upper arm fistular recommended. A retrospective study by Rajan et al. in a cohort of 177 dysfunctional autogenous fistulas treated over a 48-month period reported the overall prevalence of CAS to be 15% (26 of 177). The prevalence of CAS in brachiocephalic fistulas was 39% as compared to 2% in radiocephalic fistulas (p <.001) [Citation13]. CAS was 37 more likely in the BCF compared to the RCF. This pattern of prevalence is similar to the investigation by Turmel-Rodrigues et al. reporting CAS to be more common with BCF compared to RCF at 55% versus 7% respectively [Citation16]. Hammes et al. investigated the incidence of CAS among 127 patients (97 BCF and 30 RCF) with upper arm fistula access and venogram confirming evidence of CAS at 64%. The incidence of CAS among brachiocephalic fistula patients was 77% and 20% among radiocephalic fistula patients [Citation15]. Nam et al. reported a 4.25% prevalence of CAS in one of the largest case series. Of 1623 patients (1325 RCF and 298 BCF) with failed upper arm fistulas, CAS occurred in about 19.5% BCF and 0.83% in RCF groups [Citation17]. The lower prevalence of CAS in the RCF could be explained by presence of alternative outflow veins (basilic and median cubital veins) for the RCF other than the cephalic vein as is the case in BCF. Furthermore, the RCF is located further away from the cephalic arch relative to the BCF and may have a more favorable hemodynamic effect.
Anatomy
The Cephalic vein is part of the upper extremity’s superficial venous system. It originates in the anatomical snuffbox from the radial aspect of the superficial venous network of the dorsum of the hand. Coursing along the anterolateral forearm to the elbow, it communicates with the basilic veins via median ante-cubital veins. It then courses along the lateral aspect of the biceps toward the pectoralis major muscle as it enters the deltopectoral groove (a triangular space formed by the adjacent borders of the deltoid and pectoralis major muscles ). It then passes under the clavicle, turning sharply to pierce the clavipectoral fascia terminating as the axillary vein. The cephalic arch refers to the final arch of the cephalic vein before it drains into the first part of the axillary vein.
Depending upon the individual’s cephalic vein, its arterialization may be undertaken at different levels. Snuff box fistula is the first access if suitable cephalic vein and strong pulse of radial artery in anatomical snuff box can be palpated. Distal radiocephalic fistula of forearm at wrist is the most preferred native access if there is expressed sclerosis of radial artery in the snuff box or a strong pulse remains imperceivable at wrist. This access has been proved to have the best long-term function. Proximal brachiocephalic fistula in upper arm is frequently used as a secondary procedure after the distal radiocephalic fistula has failed.
The position of the cephalic vein has been disputed to be mostly superficial versus deep by different authors [Citation18,Citation19]. This is noteworthy as procedural failure may result from not properly locating this vein. Yeri et al. described the anatomy of the cephalic vein in the deltopectoral triangle among 50 cadaveric shoulders dissections focusing on its path and termination. The authors noted that all 50 cephalic veins were located in the deltopectoral fascia, an aponeurotic conduit connecting the pectoralis major and deltoid muscles. The deltopectoral fascia was noted to be variable in appearance, occasionally thin or disrupted by fat segments resembling subcutaneous tissue [Citation18].
More recently, Bennett et al. conducted a study on 69 patients with BCF and characterized the cephalic arch segment into four domains () in the direction of blood flow through cephalic vein. Domain 1 is the peripheral portion of the arch and domain IV is the distal portion near its termination with axillary vein. Significant stenosis was defined as 50% stenosis when compared with the standard diameter of nearest normal cephalic vein peripheral to arch. To define the 4 parts, the authors determined the domains by drawing a line through the arch’s apex that was perpendicular to the vein wall. The distance from the cephalic-axillary junction to the apex was measured, then divided into equal distance, defining the mid-point. Another line was then inserted perpendicular to the arch at midpoint. A 3rd line divided the distance between the midpoint and the apex [Citation7]. The authors concluded the order of stenosis in various domains as IV > III > II > I [Citation7]. The factors contributing to increased prevalence of stenosis in domain IV include bending of the vein as it enters the axillary vein to form subclavian vein, maximum number of valves and highest pressure and flow of blood in this domain. Anatomic variants and complex collateral circulations, including bifid and trifid cephalic arches have been described [Citation7,Citation20].
Etiology of cephalic arch stenosis
The pathophysiology of cephalic arch stenosis remains poorly understood. However, several mechanisms have been proposed which may include altered blood flow, extrinsic compression of the vessel by fascia and pectoralis major muscle, the anatomy of the cephalic arch and the entry angle of the cephalic vein as it enters the axillary vein and turbulent and presence of multiple valves. The functional profile of CAS is reported to differ from other HD access stenosis which could be explained by the involvement of the proximal segment of the cephalic vein in the arch [Citation21]. The interplay between several of these factors may predispose to the development of CAS in susceptible fistulas.
Altered blood flow
Altered blood flow has been associated with an increased risk of cephalic arch dysfunction. Laminar blood flow facilitates high shear stress thereby promoting endothelial cell survival, unidirectional flow, and release of endothelial vasodilators, and prevent neointimal hyperplasia, all of which can aid in the preservation of the AV fistula [Citation22]. On the other hand, low shear stress, or turbulent flow, promotes endothelial cell alteration, proliferation, and apoptosis, as well as the secretion of substances promoting vasoconstriction, coagulation, and platelet aggregation. High flow may also result from low resistance venous outflow. Miller et al. supported this mechanism by demonstrating that by decreasing AVF flow rates in patients with CAS, the rate of cephalic arch intervention per access year decreased from 3.34 before banding to 0.9 after banding [Citation23].
Extrinsic compression
The cephalic arch traverses within and sometimes deep to the deltopectoral and clavipectoral fascia in its course. In some individuals, this compresses the cephalic arch preventing its dilation. The proposed mechanism is that vascular compression prevents appropriate shear stress, encourages turbulent flow, and leads to an increase in venous pressure. These pathophysiologic mechanisms culminate in endothelial damage, hyperplasia, medial hypertrophy, and clinically significant cephalic arch stenosis [Citation24,Citation25].
Anatomy of the cephalic arch and the entry angle of the cephalic vein
Angulation of the cephalic vein as it enters the axillary vein has been proposed as a mechanism in the development of CAS. This angulation is theorized to precipitate turbulence, increased flow rate across the vein that injures the endothelial cells resulting in hyperplasia. However, Jaberi et al. [Citation24]. Did not find any evidence to support this hypothesis when they compared entry angles between patients with clinically significant CAS and no CAS. Hammes et al. [Citation26] investigated the cephalic arch anatomy between diabetic and non-diabetic patients. The authors reported larger alpha angles (the angle formed by lines tangent to the straight part of the cephalic vein before and after the cephalic arch) and low risk of CAS among diabetics [Citation15,Citation26]. It is unclear if there is a conclusion to be drawn in respect to cause-and-effect relationship in diabetic patients and their low risk for CAS.
Turbulence from increased flow rate and presence of venous valves
There are more valves in the central region of the cephalic vein and the number increases as it joins the axillary vein [Citation27]. In veins with suboptimal dilation, there valves may induce turbulent flow that alters the shear stress mechanism, causing endothelial injury, valvular hypertrophy, hyperplasia, all culminating in CAS [Citation27].
Intimal hyperplasia
Intimal hyperplasia has been implicated in the evolution of CAS. Studies have demonstrated high plasma levels of proinflammatory proteins such as interleukin 6, platelet derived growth factor and monocyte chemoattractant protein 1, which are known to propagate neointima formation and vascular smooth muscle hyperplasia among patients undergoing hemodialysis. This may explain the recurrent AVF stenosis [Citation28–32].
Management of cephalic arch stenosis
Early fistula maturation, high primary or secondary patency rate is desirable when choosing an HD access site stenosis management procedure. Endovascular and surgical techniques have been investigated in the management of CAS, however, there is still no consensus regarding the most effective of them yet. The failure of AVF is explained by outcome variables including maturation, primary and secondary patency rates. Periodic vascular access blood flow (QA) testing is the recommended technique for surveilling vascular access performance [Citation33]. Many authors, regard fistula failure as decreased volumetric blood flow rate less than 500 mL/min; or reduction by 20% from the baseline; or greater than 5% recirculation, stenosis or thrombosis in the fistulized segment, or significantly increased venous pressures above threshold [Citation10]. Yarar et al. [Citation34] developed and validated a novel ultrafiltration method to assesses flow (QA) and function of HD vascular access. This method detects changes in the hematocrit resulting from sudden variations in the ultrafiltration rates with the dialysis blood lines in the normal (δHn) and reverse (δHr) configurations without consideration for dialyzer blood flow rate and use of intravenous saline [Citation34].
According to the Kidney Disease Outcomes Quality Initiative statement, primary patency refers to the absence of vascular access dysfunction, patent lesion or residual stenosis <30% with no indication for further reintervention of the lesion [Citation35]. Assisted primary patency is the interval from the time of access placement to the time of access thrombosis requiring surgical or endovascular interventions to maintain access patency. Secondary patency, therefore, refers to patency occurring between the time of primary intervention and the time when the AV access is revised or abandoned [Citation35,Citation36]. The long-term patency of arteriovenous fistulas is influenced by several factors such as timely referral for placement of fistula, collaborative approach of team to secure the access, continuous quality assessment and practicing routine pre-operative mapping of arteries and veins.
Percutaneous transluminal balloon angioplasty
Percutaneous balloon angioplasty with or without stent is currently considered the first line management option for venous stenosis in HD. The primary indication for angioplasty for management of AVF or AVG stenosis in a HD patient includes anatomic; stenosis greater than 50% of the vessel luminal diameter, or clinicopathologic findings; decreased access blood flow (<600 mL/min) as evaluated on color doppler ultrasound, elevated venous pressure, decreased dialysis dose (kt/V) recirculation or urea, and abnormal physical exam. Lawrence et al. reported the first use of angioplasty for the management of AV fistula stenosis among 6 patients using the Gruntzig balloon catheter technique [Citation37]. Since then, there have been ongoing investigations into percutaneous management of vascular HD sites dysfunction. Angioplasty has the advantages of low infection risk and can be used for hemodialysis on the same procedure with no requirement for catheter placement. Despite relatively good outcomes reported with PTA in managing other HD access, CAS appears highly resistant to angioplasty with tendency for recurrent stenosis, often requiring multiple angioplasty procedures with high pressure balloons sometimes employed to overcome resistant lesions [Citation13,Citation16]. Notably, the use of high-pressure balloons carries the risk of rupture [Citation13].
Few studies have investigated outcomes of angioplasty in the management of cephalic arch stenosis. Turmel-Rodrigues et al. [Citation16] investigated this in a series of 1,118 procedures performed in native AV fistulas and AV grafts. Of the 74 upper arm fistulas reported in this cohort, more than half (55%) of lesions were noted in the outflow vein. Upper arm fistulas, mostly in the cephalic arch, had more angioplasty resistant lesions (4.8%) when compared to resistant lesions in the forearm fistulas (1.3%) [Citation16]. The reported rupture rate in this study was 14.9% in the upper arm fistulas, again, mostly in the cephalic arch, compared with 8.3% in the forearm angioplasties. The authors reported superior primary patency rates in forearm AVF at 50%, followed by 34% for upper arm AVF and the lowest at 25% for AV graft angioplasties. Secondary patency rates were comparable in the three groups at 1 year (80–86%) and at 2 years (68–80%) [Citation16].
Angioplasty outcomes specific to CAS were investigated by Rajan et al. among 117 functional AV fistulas. In their retrospective study, 66% (116) fistulas were in the upper arm and 34% (61) fistulas in the forearm. The authors reported a primary patency rate of 42% at 6 months and 23% at 1 year. The 6 months primary assisted patency was 83%, and 75% at 1 year. High inflation pressure balloons at 15 atm were required in more than half of the angioplasties (58%) with 6% rupture rate [Citation13].
A recent case control study by Neves et al. investigated strong predictors of recurrence of CAS after successful angioplasty [Citation38]. The authors reported that diabetes and low-grade stenosis (<30%) were strong predictors of CAS recurrence.
Intravascular stent deployment
Due to poor angioplasty outcomes, in part due to the need for recurrent interventions and poor primary patency, alternative management options for CAS are being investigated. Stent graft placement improves angioplasty outcomes and is becoming more employed in the primary management of CAS (). Bare metal stents (BMS) are falling out of favor due to high in-stent restenosis and low patency rates making stent grafts a better alternative. Bare metal stents appear relatively superior to angioplasty alone [Citation39]. Dukkipati et al. compared outcomes following angioplasty alone to BMS in their study of 45 patients. The authors reported a median patency of 152 days among BMSs patients versus 91.5 days in those who underwent only angioplasty [Citation40]. Shemesh et al. conducted a randomized control trial comparing the patency rates of bare metal stents and stent graft among 25 patients undergoing management for failed angioplasty for the management of recurrent CAS. They reported primary patency at 6 months for BMS at 39% compared to stent grafts at 82%. Primary patency at 1 year was 32% in the stent graft group versus 0% in the bare stent group. Rate of restenosis was 70% (10) in the BMS group and 18% (11) in the stent graft group [Citation41]. Jones et al. described a group of 39 patients who underwent stent graft placement for management of CAS. They reported primary patency of 85%, 67%, and 4%, at 3, 6, and 12 months respectively. Primary assisted patency was 95% at 12 months [Citation42].
Figure 4. Left: Cephalic arch stenosis. Right: Stent placement for management of cephalic arch stenosis.
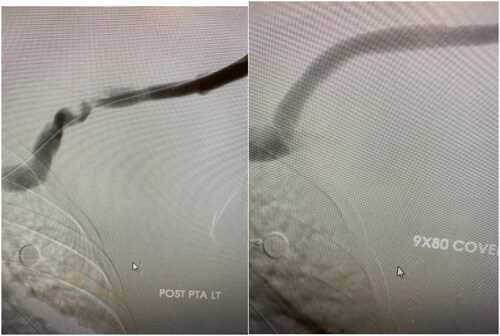
Placing appropriately sized stent at the inflow mitigates stent migration and guttering which can lead to turbulent flow and precipitate restenosis. Stent protrusion into the veins can lead to axillary or subclavian stenosis or even jailing of the basilic vein and may cause arm swelling and preclude future fistula formation [Citation41]. Cahalane et al. [Citation43] conducted a retrospective study investigating the association between anatomic characteristics and the primary patency of BC fistulas following stent graft placement in CAS management. The SG placed were 0–1 mm larger than the adjacent segment. The investigators reported primary patency rates of 64%, 49.9%, and 23.5%, at 6, 12 months and 3 years respectively among 63 patients that underwent stent graft placement. They also reported an association between stent graft size and CAS but not with cephalic arch anatomy. They posit that under sizing the stent graft (determined by the diameter of the adjacent healthy cephalic vein segment) would not accommodate for potential expansion of the cephalic venous outflow that may occur over time resulting in access aneurysm and subsequent dysfunction. One of the limitations of this study is the small patient population that precluded meaningful small group analysis to make substantial conclusions [Citation43]
Overall, among studies reporting on covered stent grafts, the 6 months primary and secondary patency rates were 67–82% and 88–90%, respectively [Citation10]. After one-year, primary patency dropped to 22–72.72% [Citation10]. Stent-grafts seem to be the most durable endovascular intervention; however, they frequently require re-intervention at one year to maintain patency. There is also increased risk of thrombosis or stenosis in the central venous outflow if the stent graft crosses into the axillary vein from the cephalic arch during intervention. Reintervention rates for bare metal stents have been shown to be 0.75–1.9 versus 0.9 in stent grafts per patient-year [Citation40,Citation41].
Stent placement during angioplasty improves overall patency rate compared to angioplasty alone. Self-expanding stents are preferred due to their low migration risk. Dukkipati et al. compared the mean number of angioplasties required among 20 patients who underwent angioplasty with stent and 25 patients without stents and reported 0.75 and 2.76 reintervention rates respectively [Citation40].
Drug coated balloon
Drug coated balloon (DCB) has been investigated as a management option in treating dysfunctional hemodialysis AV access, both AV fistula and grafts [Citation44–47]. The use of DCB for these lesions evolved as an avenue to address high rates of restenosis and failures associated with standard plain balloon angioplasty. Majority of studies report on the outcomes for DCB in the management of a wide variety of dysfunctional HD access and are often not specific to cephalic arch stenosis [Citation47–49]. The reported primary patency and restenosis rates are variable, with most reporting improve primary patency within the first 6 months, after which no difference existed between DCB and standard PTA [Citation45,Citation48–51].
Recently, Tng et al. [Citation51] conducted a retrospective cohort study comparing patency rates among 91 patients who underwent plain balloon angioplasty (65 patients) and DCB (26 patients, paclitaxel-coated balloons) over a 3-year period for the management of CAS. BCF was the predominant access type in these patient populations at 94.5% vs 5.5% for radiocephalic fistula. Comparative outcomes included primary patency rates (from index procedure to thrombosis), primary assisted-patency rates and CAS target lesion (stenosis >50%) at 3, 6 and 12 months. Primary patency rates were not statistically significant for standard PTA versus DCB reported to as 76.2% vs. 60% (p = 0.21), 43.5% vs. 36% (p = 0.69) and 22% vs. 9.1% (p = 0.22) at 3, 6 and 12-months, respectively. The assisted primary patency rates following index intervention were 93.7% vs. 92% (p = 1.00), 87.1% vs. 80% (p = 0.51) and 76.3% vs. 81.8% (p = 0.77) while CAS target lesion intervention free patency rates were 79.4% vs. 68% (p = 0.40), 51.6% vs. 52% (p = 1.00) and 33.9% vs. 22.7% (p = 0.49) at 3, 6, and 12-months, respectively.
A global prospective non-blinded control trial by Lookstein et al. [Citation48], randomized 330 patients with new or re-stenotic lesions involving the upper extremity AVF, to either drug coated balloons or standard balloon angioplasty [Citation48]. Majority of the target lesions were in the venous outflow, including the cephalic arch. There was an equal number of arteriovenous access lesions in the forearm (radiocephalic) and upper arm (brachiocephalic and brachiobasilic) included in the study [Citation48]. The primary endpoint was target lesion primary patency as determined by clinically driven target-lesion revascularization or safety information for any major adverse event at 30 days. The drug coated balloon arm had a higher 6-month TLPP at 82.2% vs 59.5% for the standard PTA arm (p < 0.001), and sensitivity analysis confirmed these findings. DCB was found to be non-inferior to standard PTA in the primary safety endpoint (4.2% vs. 4.4%, respectively; p = 0.002) [Citation48]. A similar RCT conducted by Irani et al. [Citation47] showed comparable results. The target lesion primary patency and primary access circuit patency at 6 months was significantly improved at 6 months in the DCB arm (TLPP 81% vs 61% p 0.03: ACPP 76% vs 56% p 0.048) [Citation47]. DCB may require high pressure dilatation to achieve device success, augmenting its procedural success rate at a level comparable to standard PTA [Citation45,Citation48].
Peripheral cutting balloons angioplasty
Cutting balloon angioplasty was initially employed for lesions due to atherosclerotic plaque seen in coronary artery disease but has now been adapted to the management of intimal hyperplasia more commonly seen in dysfunctional hemodialysis access site. Very few studies investigating its efficacy and outcomes in managing cephalic arch stenosis are few. Majority of available studies included a wide range of dysfunctional AVF lesions. In HD access management, CBA use has been restricted primarily to management of resistant, hard to dilate venous stenoses especially following failure of conventional PTA [Citation52,Citation53]. The high recurrence rate after percutaneous transluminal balloon angioplasty has been attributed in part to intimal injury from the initial endovascular intervention. This is thought to be mitigated by microsurgical incisions made into neointimal hyperplasia during cutting balloon angioplasty with the benefit of less intimal trauma [Citation53]. CBA also has a low rupture risk due to less wall tension and protects against secondary restenosis thereby prolonging patency [Citation53]. Another limitation to the use of CBA is the risk of elastic recoil necessitating stent placement. Notably, cutting balloons require an average of 0.9 interventions per patient per year [Citation14]. The application of the outcomes of some existing studies in the management of CAS is limited by the wide variety of access dysfunctions included in these investigations beyond CAS lesions.
Preliminary results from several investigations suggest some benefit in combining conventional PTA and cutting balloons in the management of hemodialysis access stenosis. Here, cutting balloon is performed before conventional or PTA to improve residual stenosis [Citation54–56]. However, the use of high-pressure balloon following CBA could in theory, counter the benefit of vascular trauma reduction attainable with CBA. The published reports on cutting balloon angioplasty for treatment of hemodialysis vascular access stenosis do not accurately inform on the inherent patency rates attributable to CBA. Several of these studies report on the use of cutting balloons alongside conventional PTA or high-pressure balloon or with stent placements, as these results are not reflective of the outcomes of CBA as a primary treatment [Citation54,Citation57,Citation58].
Several studies have compared the patency rates for cutting balloons to conventional percutaneous transluminal angioplasty in the management of autogenous venous graft, ingraft stenosis, intra-graft stenosis with mixed results [Citation59]. Heerwagen et al. in their retrospective review of endovascular outcomes among 17 patients who underwent cutting balloon angioplasty for the management of CAS, with and without conventional PTA reported primary patency rates at 3, 6, 12, and 15 months were 94%, 81%, 38 percent (14%), and 22 percent (15%), respectively. At similar durations, the assisted primary patency rates were 100%, 94%, 77%, and 63 13%, respectively. The mean duration between endovascular interventions was 13 months (SD = 8), with 0.9 interventions required per patient-year of dialysis [Citation14]. Authors found no improvement in the patency rates after CBA compared to what is known in literature about conventional PTA. This study is limited due to the small sample size and no control group.
Saleh et al. conducted one of the largest prospective studies comparing the rates of patency achievable with PTA using cutting balloon and conventional angioplasty in the management of HD access dysfunction. The authors randomized 623 patients into the 2 groups, either to receive cutting balloon angioplasty or conventional balloon angioplasty. In the cutting balloon angioplasty arm, there were 316 stenoses with 95 venous (including CAS), 80 graft-to-vein anastomosis, 61 intra-graft, and 80 arterial anastomotic lesions. The conventional PTA arm enrolled patients with a total of 307 stenosis, consisting of 81 venous (including CAS), 82 graft-to-vein anastomosis, 72 intra-graft and 72 arterial anastomotic stenosis. They reported higher 6 and 12 months assisted primary patency among patients who underwent cutting balloon angioplasty (86% and 63%) compared with patients who underwent conventional balloon angioplasty (56% and 37%) in the management of graft-to-vein anastomotic stenosis [Citation60]. There was no significant difference in the assisted primary patency rate among the intra-graft stenosis and venous stenosis subgroups [Citation60]. The authors did not report data on subgroup analysis for patients with CAS alone [Citation60]. Furthermore, the venous stenosis group in this study is relatively more powered than existing studies on CBA use in CAS suggesting the need for future adequately powered studies.
Surgical interventions
Flow reduction endovascular banding
High flow rate has been proposed as a potential mechanism in the development of CAS. Therapeutic flow reduction intervention has been explored. Miller et al. conducted a retrospective review of 33 patients with recurrent CAS who underwent fistula banding with balloon assisted technique to reduce flow. Primary patency rate after banding was 91%, 76%, and 57% at 3, 6, and 12 months, respectively. The rate of cephalic arch intervention reduced from 3.34 to 0.9 per access year (P.001). This further supports the idea that high flow rates in the BCF may contribute to cephalic arch stenosis [Citation23].
Cephalic vein transposition
Surgical intervention for the management of CAS is reserved for recurrent CAS failing angioplasty [Citation61]. It reduces rates of reintervention compared to angioplasty however they have a similar patency rate after 2 years. There is evidence of improved patency rates with surgery after failed angioplasty without increasing complication rates. Cephalic vein transposition involves surgically ligating the cephalic vein at the deltopectoral groove and transposing the remaining segment onto the distal axillary vein. Alternatively, interposition graft may be surgically transposed from the cephalic to the jugular vein other than the axillary vein [Citation62]. Studies investigating cephalic vein turn down as initial intervention in the management of CAS prior to angioplasty have reported poor outcomes [Citation63,Citation64]. Furthermore, CVT precludes future creation of basilic vein fistula in certain populations [Citation65].
Chen et al. [Citation61] in their report of 9 patients with brachiocephalic fistulas, (median age of 14 months) who underwent surgical revision, cephalic vein transposition was done for 7 fistulas and two fistulas had basilic vein transpositions. Primary patency rates were 70% at 6 months and 60% at 1 year. The authors reported higher patency rates with cephalic transposition than those reported for CAS angioplasty [Citation13,Citation61].
Henry et al. retrospectively reviewed 23 patients with CAS who underwent cephalic vein transposition, most of which were after recurrent angioplasties. Transposition was primary in 3 patients. The average number of angioplasty interventions to the cephalic arch prior to transposition was 2.3 ± 0.9. Ninety seven percent (23/24) were able to keep their regular access schedule. The two-year primary patency rate was 70.9% and secondary patency 94.7%. The primary patency rate is comparable to those reported by similar studies [Citation64,Citation66,Citation67].
Best outcomes after CV transposition are achieved when the axillary and central veins are patent, mature, and active. It is not suitable for intervening on axillary, subclavian, or innominate vein occlusion and should rarely be performed on non-matured, non-utilized fistulas [Citation68]. CVT has a low re-intervention rate of about 0.16 interventions per patient per year which is comparable across similar studies [Citation66,Citation68].
Prior arch stenting should not discourage future cephalic arch transposition if the stent does not extend significantly into the axillary vein. Although angioplasty is regarded as the first line for CAS, with no restriction on the number of angioplasties that could be attempted prior to surgery in recurrent CAS, CVT should be considered early in the course in appropriate clinical scenario due to its low rate of reintervention.
Cephalic vein turn-down
Cephalic turn down is another surgical intervention which has shown promising outcomes in the management of CAS. Kian et al. [Citation65] investigated the role of cephalic vein turndown in the management of CAS in 13 patients. The authors compared the patency rates for angioplasty procedure prior to the surgical revision which were 23%, 8%, and 0%, at 3, 6, and 12 months, respectively. The secondary patency rates for balloon angioplasty before surgical revision were 100%, 39%, and 8%, at 3, 6, and 12 months respectively. Primary patency rates for angioplasty after surgical revision were 92%, 69%, and 39%, at 3, 6, and 12 months respectively (P .0001). Following the surgical revision, all patients required percutaneous balloon angioplasty for access dysfunction. Secondary patency following surgical revision was 92% at 3, 6, and 12 months [Citation65]. Studies indicate that surgical intervention can prolong subsequent patency rates for percutaneous balloon angioplasty without increasing the complication rates.
Conclusion
The best management approach for CAS should maintain the integrity of the fistula for long term patency and for effective flow. Though there are no guidelines for the number of angioplasties that may be performed on the cephalic arch, secondary procedures, such as endovascular interventions and surgical transpositions are considerable alternatives in refractory cephalic arch stenosis. Appropriate patient selection increases the likelihood of prolonged patency and viability of AV access.
Author contributions
GE, RD and DK conceptualized and designed the topic. GE, SS, DK drafted the manuscript. GE, JS, RD, DK critically revised the manuscript. All authors approved the final version of the manuscript.
Disclosure statement
No potential conflict of interest was reported by the author(s).
Additional information
Funding
References
- Eknoyan G, Lameire N, Barsoum R, et al. The burden of kidney disease: improving global outcomes. Kidney Int. 2004;66(4):1310–1314.
- USRDS: USRDS 2010. Annual data report: Atlas of chronic kidney disease and end-stage renal disease in the United States. National Institutes of Health, National Institute of Diabetes and Digestive and Kidney Diseases. 2010.
- Allon M, Robbin ML. Increasing arteriovenous fistulas in hemodialysis patients: problems and solutions. Kidney Int. 2002;62(4):1109–1124.
- NKF-DOQI clinical practice guidelines for vascular access. National Kidney Foundation. 75(4):S1–S164.
- Gilmore J. KDOQI clinical practice guidelines and clinical practice recommendations–2006 updates. Nephrol Nurs J. 2006;33(5):487–488.
- Rooijens PP, Tordoir JH, Stijnen T, et al. Radiocephalic wrist arteriovenous fistula for hemodialysis: meta-analysis indicates a high primary failure rate. Eur J Vasc Endovasc Surg. 2004;28(6):583–589.
- Bennett S, Hammes MS, Blicharski T, et al. Characterization of the cephalic arch and location of stenosis. J Vasc Access. 2015;16(1):13–18.
- Tordoir JH, Rooyens P, Dammers R, et al. Prospective evaluation of failure modes in autogenous radiocephalic wrist access for haemodialysis. Nephrol Dial Transplant. 2003;18(2):378–383.
- Pisoni RL, Zepel L, Fluck R, et al. International differences in the location and use of arteriovenous accesses created for hemodialysis: results from the dialysis outcomes and practice patterns study (DOPPS). Am J Kidney Dis. 2018;71(4):469–478.
- Sivananthan G, Menashe L, Halin NJ. Cephalic arch stenosis in dialysis patients: review of clinical relevance, anatomy, current theories on etiology and management. J Vasc Access. 2014;15(3):157–162.
- Nursal TO, Tercan F, Torer N, et al. Is routine preoperative ultrasonographic mapping for arteriovenous fistula creation necessary in patients with favorable physical examination findings? Results of a randomized controlled trial. World J Surg. 2006;30(6):1100–1107.
- Rajan DK, Bunston S, Misra S, et al. Dysfunctional autogenous hemodialysis fistulas: outcomes after angioplasty–are there clinical predictors of patency? Radiology. 2004;232(2):508–515.
- Rajan DK, Clark TW, Patel NK, et al. Prevalence and treatment of cephalic arch stenosis in dysfunctional autogenous hemodialysis fistulas. J Vasc Interv Radiol. 2003;14(5):567–573.
- Heerwagen ST, Lönn L, Schroeder TV, et al. Cephalic arch stenosis in autogenous brachiocephalic hemodialysis fistulas: results of cutting balloon angioplasty. J Vasc Access. 2010;11(1):41–45.
- Hammes M, Funaki B, Coe FL. Cephalic arch stenosis in patients with fistula access for hemodialysis: relationship to diabetes and thrombosis. Hemodial Int. 2008;12(1):85–89.
- Turmel-Rodrigues L, Pengloan J, Baudin S, et al. Treatment of stenosis and thrombosis in haemodialysis fistulas and grafts by interventional radiology. Nephrol Dial Transplant. 2000;15(12):2029–2036.
- Nam DH, Kim YJ, Goo DE. Abstract no. 91: Percutaneous angioplasty in a cephalic arch stenosis of native arteriovenous fistula. J Vasc Inter Radiol. 2008;19.
- Yeri LA, Houghton EJ, Bruno P, et al. Cephalic vein. Detail of its anatomy in the deltopectoral triangle. Int. J. Morphol. 27(4):1037–1042.
- Loukas M, Myers CS, Wartmann CT, et al. The clinical anatomy of the cephalic vein in the deltopectoral triangle. Folia Morphol. 2008;67(1):72–77.
- Kian K, Asif A. Cephalic arch stenosis. Semin Dial. 2008;21(1):78–82.
- Roca-Tey R, Samón R, Ibrik O, et al. Functional profile of cephalic arch stenosis. Nefrologia. 2009;29(4):350–353.
- Paszkowiak JJ, Dardik A. Arterial wall shear stress: observations from the bench to the bedside. Vasc Endovascular Surg. 2003;37(1):47–57.
- Miller GA, Friedman A, Khariton A, et al. Access flow reduction and recurrent symptomatic cephalic arch stenosis in brachiocephalic hemodialysis arteriovenous fistulas. J Vasc Access. 2010;11(4):281–287.
- Jaberi A, Schwartz D, Marticorena R, et al. Risk factors for the development of cephalic arch stenosis. J Vasc Access. 2007;8(4):287–295.
- Gusic RJ, Myung R, Petko M, et al. Shear stress and pressure modulate saphenous vein remodeling ex vivo. J Biomech. 2005;38(9):1760–1769.
- Hammes MS, Boghosian ME, Cassel KW, et al. Characteristic differences in cephalic arch geometry for diabetic and non-diabetic ESRD patients. Nephrol Dial Transplant. 2009;24(7):2190–2194.
- Iimura A, Nakamura Y, Itoh M. Anatomical study of distribution of valves of the cutaneous veins of adult’s limbs. Ann Anat. 2003;185(1):91–95.
- Liani M, Salvati F, Tresca E, et al. Arteriovenous fistula obstruction and expression of platelet receptors for von willebrand factor and for fibrinogen (glycoproteins GPib and GPiib/iiia) in hemodialysis patients. Int J Artif Organs. 1996;19(8):451–454.
- De Marchi S, Cecchin E, Falleti E, et al. Long-term effects of erythropoietin therapy on fistula stenosis and plasma concentrations of PDGF and MCP-1 in hemodialysis patients. J Am Soc Nephrol. 1997;8(7):1147–1156.
- De Marchi S, Falleti E, Giacomello R, et al. Risk factors for vascular disease and arteriovenous fistula dysfunction in hemodialysis patients. J Am Soc Nephrol. 1996;7(8):1169–1177.
- Wali MA, Eid RA, Dewan M, et al. Intimal changes in the cephalic vein of renal failure patients before arterio-venous fistula (AVF) construction. J Smooth Muscle Res. 2003;39(4):95–105.
- Wali MA, Eid RA, Dewan M, et al. Pre-existing histopathological changes in the cephalic vein of renal failure patients before arterio-venous fistula (AVF) construction. Ann Thorac Cardiovasc Surg. 2006;12(5):341–348.
- Lok CE, Huber TS, Lee T, et al.; National Kidney Foundation. KDOQI clinical practice guideline for vascular access: 2019 update. Am J Kidney Dis. 2020;75(4 Suppl 2):S1–S164.
- Yarar D, Cheung AK, Sakiewicz P, et al. Ultrafiltration method for measuring vascular access flow rates during hemodialysis. Kidney Int. 1999;56(3):1129–1135.
- Group VAW. Clinical practice guidelines for vascular access. Am J Kidney Dis. 2006;48(Suppl 1):S176–S247.
- Sidawy AN, Gray R, Besarab A, et al. Recommended standards for reports dealing with arteriovenous hemodialysis accesses. J Vasc Surg. 2002;35(3):603–610.
- Lawrence PF, Miller FJ, Mineaud E. Balloon catheter dilatation in patients with failing arteriovenous fistulas. Surgery. 1981;89(4):439–442.
- Neves M, Outerelo C, Pereira M, et al. Predictive factors of recurrent endovascular intervention for cephalic arch stenosis after percutaneous transluminal angioplasty. J Vasc Surg. 2018;68(3):836–842.
- Mickley V. Central vein obstruction in vascular access. Eur J Vasc Endovasc Surg. 2006;32(4):439–444.
- Dukkipati R, Lee L, Atray N, et al. Outcomes of cephalic arch stenosis with and without stent placement after percutaneous balloon angioplasty in hemodialysis patients. Semin Dial. 2015;28(1):E7–E10.
- Shemesh D, Goldin I, Zaghal I, et al. Angioplasty with stent graft versus bare stent for recurrent cephalic arch stenosis in autogenous arteriovenous access for hemodialysis: a prospective randomized clinical trial. J Vasc Surg. 2008;48(6):1524–1531.
- Jones RG, Willis AP, Tullett K, et al. Results of stent graft placement to treat cephalic arch stenosis in hemodialysis patients with dysfunctional brachiocephalic arteriovenous fistulas. J Vasc Interv Radiol. Oct 2017;28(10):1417–1421.
- Cahalane AM, Abboud SE, Kawai T, et al. Stent diameter, not cephalic arch anatomy, predicts stent graft patency in cephalic arch stenosis. J Vasc Interv Radiol. 2022;33(11):1321–1328.e1.
- Patanè D, Giuffrida S, Morale W, et al. Drug-eluting balloon for the treatment of failing hemodialytic radiocephalic arteriovenous fistulas: our experience in the treatment of juxta-anastomotic stenoses. J Vasc Access. 2014;15(5):338–343.
- Katsanos K, Karnabatidis D, Kitrou P, et al. Paclitaxel-coated balloon angioplasty vs. plain balloon dilation for the treatment of failing dialysis access: 6-month interim results from a prospective randomized controlled trial. J Endovasc Ther. 2012;19(2):263–272.
- Kitrou PM, Spiliopoulos S, Papadimatos P, et al. Paclitaxel-Coated balloons for the treatment of dysfunctional dialysis access. Results from a Single-Center, retrospective analysis. Cardiovasc Intervent Radiol. 2017;40(1):50–54.
- Irani FG, Teo TKB, Tay KH, et al. Hemodialysis arteriovenous fistula and graft stenoses: randomized trial comparing drug-eluting balloon angioplasty with conventional angioplasty. Radiology. 2018;289(1):238–247.
- Lookstein RA, Haruguchi H, Ouriel K, et al. Drug-Coated balloons for dysfunctional dialysis arteriovenous fistulas. N Engl J Med. 2020;383(8):733–742.
- Trerotola SO, Lawson J, Roy-Chaudhury P, Lutonix AV Clinical Trial Investigators, et al. Drug coated balloon angioplasty in failing AV fistulas: a randomized controlled trial. Clin J Am Soc Nephrol. 2018;13(8):1215–1224.
- Lai CC, Fang HC, Tseng CJ, et al. Percutaneous angioplasty using a paclitaxel-coated balloon improves target lesion restenosis on inflow lesions of autogenous radiocephalic fistulas: a pilot study. J Vasc Interv Radiol. Apr 2014;25(4):535–541.
- Tng RKA, Tan RY, Soon SXY, et al. Treatment of cephalic arch stenosis in dysfunctional arteriovenous fistulas with paclitaxel-coated versus conventional balloon angioplasty. CVIR Endovasc. 2021;4(1):80.
- Funaki B. Cutting balloon angioplasty in arteriovenous fistulas. J Vasc Interv Radiol. 2005;16(1):5–7.
- Tsetis D, Morgan R, Belli AM. Cutting balloons for the treatment of vascular stenoses. Eur Radiol. 2006;16(8):1675–1683.
- Vorwerk D, Adam G, Müller-Leisse C, et al. Hemodialysis fistulas and grafts: use of cutting balloons to dilate venous stenoses. Radiology. 1996;201(3):864–867.
- Vorwerk D, Günther RW, Schürmann K, et al. Use of a cutting balloon for dilatation of a resistant venous stenosis of a hemodialysis fistula. Cardiovasc Intervent Radiol. 1995;18(1):62–64.
- Ryan JM, Dumbleton SA, Smith TP. Technical innovation. Using a cutting balloon to treat resistant high-grade dialysis graft stenosis. AJR Am J Roentgenol. 2003;180(4):1072–1074.
- Song HH, Kim KT, Chung SK, et al. Cutting balloon angioplasty for resistant venous stenoses of Brescia-Cimino fistulas. J Vasc Interv Radiol. 2004;15(12):1463–1467.
- Sreenarasimhaiah VP, Margassery SK, Martin KJ, et al. Cutting balloon angioplasty for resistant venous anastomotic stenoses. Semin Dial. 2004;17(6):523–527.
- Vesely TM, Siegel JB. Use of the peripheral cutting balloon to treat hemodialysis-related stenoses. J Vasc Interv Radiol. 2005;16(12):1593–1603.
- Saleh HM, Gabr AK, Tawfik MM, et al. Prospective, randomized study of cutting balloon angioplasty versus conventional balloon angioplasty for the treatment of hemodialysis access stenoses. J Vasc Surg. 2014;60(3):735–740.
- Chen JC, Kamal DM, Jastrzebski J, et al. Venovenostomy for outflow venous obstruction in patients with upper extremity autogenous hemodialysis arteriovenous access. Ann Vasc Surg. 2005;19(5):629–635.
- Hoballah JJ, Eid GM, Nazzal MM, et al. Contralateral internal jugular vein interposition for salvage of a functioning arteriovenous fistula. Ann Vasc Surg. 2000;14(6):679–682.
- Kim SM, Yoon KW, Woo SY, et al. Treatment strategies for cephalic arch stenosis in patients with brachiocephalic arteriovenous fistula. Ann Vasc Surg. 2019;54:248–253.
- Wang S, Almehmi A, Asif A. Surgical management of cephalic arch occlusive lesions: are there predictors for outcomes? Semin Dial. 2013;26(4):E33–41.
- Kian K, Unger SW, Mishler R, et al. Role of surgical intervention for cephalic arch stenosis in the "fistula first" era. Semin Dial. 2008;21(1):93–96.
- Sigala F, Saßen R, Kontis E, et al. Surgical treatment of cephalic arch stenosis by Central transposition of the cephalic vein. J Vasc Access. 2014;15(4):272–277.
- Jang J, Jung H, Cho J, et al. Central transposition of the cephalic vein in patients with brachiocephalic arteriovenous fistula and cephalic arch stenosis. Vasc Specialist Int. 2014;30(2):62–67.
- Henry JC, Sachdev U, Hager E, et al. Cephalic vein transposition is a durable approach to managing cephalic arch stenosis. J Vasc Access. 2018;2017(0):jva.5000802.