Abstract
Background
Some studies have found that ferroptosis plays an important role in the incidence of acute kidney injury (AKI) after cardiac surgery. However, whether iron metabolism-related indicators can be used as predictors of the incidence of AKI after cardiac surgery remains unclear.
Objectives
We aimed to systematically evaluate whether iron metabolism-related indicators can be used as predictors of the incidence of AKI after cardiac surgery via meta-analysis.
Search methods: The PubMed, Embase, Web of Science, and Cochrane Library databases were searched from January 1971 to February 2023 to identify prospective observational and retrospective observational studies examining iron metabolism-related indicators and the incidence of AKI after cardiac surgery among adults.
Data Extraction and Synthesis: The following data were extracted by two independent authors (ZLM and YXY): date of publication, first author, country, age, sex, number of included patients, iron metabolism-related indicators, outcomes of patients, patient types, study types, sample, and specimen sampling time. The level of agreement between authors was determined using Cohen’s κ value. The Newcastle–Ottawa Scale (NOS) was used to evaluate the quality of studies. Statistical heterogeneity across the studies was measured by the I2 statistic. The standardized mean difference (SMD) and 95% confidence interval (CI) were used as effect size measures. Meta-analysis was performed using Stata 15.
Results
After applying the inclusion and exclusion criteria, 9 articles on iron metabolism-related indicators and the incidence of AKI after cardiac surgery were included in this study. Meta-analysis revealed that after cardiac surgery, baseline serum ferritin (μg/L) (I2 = 43%, fixed effects model, SMD = −0.3, 95% CI:-0.54 to −0.07, p = 0.010), preoperative and 6-hour postoperative fractional excretion (FE) of hepcidin (%) (I2 = 0.0%, fixed effects model, SMD = −0.41, 95% CI: −0.79 to −0.02, p = 0.038; I2 = 27.0%, fixed effects model, SMD = −0.49, 95% CI: −0.88 to −0.11, p = 0.012), 24-hour postoperative urinary hepcidin (μg/L) (I2 = 0.0%, fixed effects model, SMD = −0.60, 95% CI: −0.82 to −0.37, p < 0.001) and urine hepcidin/urine creatinine ratio (μg/mmoL) (I2 = 0.0%, fixed effects model, SMD = −0.65, 95% CI: −0.86 to −0.43, p < 0.001) were significantly lower in patients who developed to AKI than in those who did not.
Conclusion
After cardiac surgery, patients with lower baseline serum ferritin levels (μg/L), lower preoperative and 6-hour postoperative FE of hepcidin (%), lower 24-hour postoperative hepcidin/urine creatinine ratios (μg/mmol) and lower 24-hour postoperative urinary hepcidin levels (μg/L) are more likely to develop AKI. Therefore, these parameters have the potential to be predictors for AKI after cardiac surgery in the future. In addition, there is a need for relevant clinical research of larger scale and with multiple centers to further test these parameters and prove our conclusion.
Trial Registration: PROSPERO identifier: CRD42022369380.
1. Introduction
Acute kidney injury (AKI) is a common complication in patients undergoing cardiac surgery with cardiopulmonary bypass (CPB), with an incidence rate of up to 23.5%–40% [Citation1]. CPB-associated AKI (CPB-AKI) increases the need for mechanical ventilation, the days of intensive care, and the risk of mortality [Citation2, Citation3]. CPB-AKI involves several injury pathways: endogenous and exogenous toxins, ischemia and reperfusion, metabolic factors, inflammation, and oxidative stress [Citation4]. The dominant mechanism of injury is thought to be intraoperative ischemia–reperfusion injury. Data from animal studies show that AKI due to ischemia–reperfusion injury is potentially reversible, provided that the therapeutic intervention is administered at or shortly after the time of injury during a window of time corresponding to the initiation or early extension phases of ischemia–reperfusion injury [Citation5–7]. Clinical studies have also confirmed that early identification paired with effective interventions can reduce the frequency and severity of postoperative AKI after cardiac surgery [Citation8]. However, at present, serum creatinine and urine have a lag in the diagnosis of AKI [Citation9]. Therefore, it is necessary to identify risk factors associated with AKI incidence after cardiac surgery to allow that preventive and early diagnosis measures to be taken, thereby reducing the incidence of AKI after cardiac surgery.
Ferroptosis is a recently discovered mechanism of regulated cell death with unique features, such as the iron-dependent accumulation of lipid peroxides [Citation10]. Circulating iron in the human body exists mainly in the form of ferric (Fe3+) iron bound to transferrin. Fe3+ enters the cell through the membrane protein transferrin receptor 1 (TFR1) and then localizes to the endonucleosome. In the endonucleosome, ferric reductase reduces Fe3+ to ferrous iron (Fe2+). Finally, divalent metal transporter protein 1 (DMT1) facilitates the release of Fe2+ from the endonucleosome into the unstable iron pool in the cytoplasm, where excess iron is stored in ferritin. Elevated serum iron levels in the body can induce hepcidin upregulation, which in turn reduces serum iron. When iron homeostasis is out of balance in the human body, iron overload can induce lipid peroxidation through the Fenton reaction, leading to ferroptosis [Citation11]. After cardiac surgery, a large amount of catalytic iron is released, which may trigger AKI by inducing ferroptosis [Citation12–14]. Some studies have found that iron metabolism-related indicators (serum ferritin, hepcidin, transferrin, urine catalytic iron) can be used as predictors of the incidence of AKI after cardiac surgery [Citation15, Citation16]; however, existing studies have the disadvantages of small sample sizes and inconsistent findings. Therefore, in this meta-analysis, we systematically evaluated whether these indicators can be used as predictors of the incidence of AKI after cardiac surgery. This study will provide evidence-based medical guidance for the early clinical prediction of AKI incidence after cardiac surgery.
2. Method
2.1. Search strategy
In accordance with the PRISMA guidelines, we systematically searched the PubMed, Web of Science, Embase and Cochrane Library databases from January 1971 to February 2023. The following keywords were used: (Acute Kidney Injury OR Acute Kidney Injuries OR Kidney Injuries, Acute OR Kidney Injury, Acute OR Acute Renal Injury OR Acute Renal Injuries OR Renal Injuries, Acute OR Renal Injury, Acute OR Renal Insufficiency, Acute OR Acute Renal Insufficiencies OR Renal Insufficiencies, Acute OR Acute Renal Insufficiency OR Kidney Insufficiency, Acute OR Acute Kidney Insufficiencies OR Kidney Insufficiencies, Acute OR Acute Kidney Insufficiency OR Kidney Failure, Acute OR Acute Kidney Failures OR Kidney Failures, Acute OR Acute Renal Failure OR Acute Renal Failures OR Renal Failures, Acute OR Renal Failure, Acute OR Acute Kidney Failure) AND (iron OR ferritin OR transferrin OR transferrin saturation OR TSAT OR hepcidin OR hepc) AND (cardiac surgery OR cardiac surgical procedures OR cardiac operation OR cardio surgery OR cardiopulmonary bypass). The detailed search strategy is shown in Supplementary material Table 1. The study protocol for this review was registered in the International Prospective Register of Systematic Reviews (PROSPERO, registration number CRD42022369380). This study was reported in accordance with the Preferred Reporting Items for Systematic Reviews and Meta-Analysis guidelines [Citation17].
2.2. Study selection
To minimize bias, two authors (ZLM and YXY) independently screened the titles and abstracts of the initially retrieved articles to determine their eligibility. Then, the full texts of selected articles were carefully reviewed based on the inclusion and exclusion criteria. The level of agreement between authors was determined using Cohen’s κ statistics. We contacted the corresponding authors for additional details if full-text articles contained insufficient information to determine their eligibility. Disagreements were resolved by discussion with the third researcher (ZXS).
The inclusion criteria were as follows: 1. articles on iron metabolism-related indicators and the incidence of AKI after cardiac surgery that were published between January 1971 and February 2023 and that included data on iron metabolism-related indicators (serum ferritin, hepcidin, transferrin, urine catalytic iron); 2. study population included patients ≥ 18 years old undergoing cardiac surgery, and the study outcome was AKI incidence (based on the Kidney Disease: Improving Global Outcomes (KDIGO) [Citation18] criteria, AKI was defined as an increase in serum creatinine (SCr) by ≥ 26.5 μmol/L within 48 h or an increase in SCr to ≥ 1.5 times from baseline values within 7 days); 3. prospective observational studies and retrospective observational studies; 4. Newcastle–Ottawa Scale (NOS) score ≥ 6; and 5. the full text of the study was available in English.
The exclusion criteria were as follows: 1. review articles, letters, comments, or opinions; 2. nonprospective observational studies and nonretrospective observational studies; and 3. incomplete data or unavailable data.
2.3. Quality assessment
All included studies were retrospective cohort studies or prospective cohort studies. Therefore, the Newcastle–Ottawa Scale (NOS) was used to evaluate the quality (). The major components of the NOS include adequate definition of cases, representativeness of the cases, selection of controls, definition of controls, comparability, ascertainment of exposure, the same method of ascertainment for cases and controls, and nonresponse rate. The quality rating ranges from 0 to 10 stars, and a score ≥ 6 stars indicates high-quality articles.
Table 1. The detail NOS score of studies included.
Two reviewers (ZLM and YXY) independently performed quality assessments, and disagreements were resolved by discussion with a third reviewer (ZXS).
2.4. Data extraction
Two independent authors (ZLM and YXY) independently extracted the following data by using standardized forms: date of publication, first author, country, age, sex, number of included patients, iron metabolism-related indicators, outcomes of patients, patient types, study types, sample, and specimen sampling time ().
Table 2. The basic information table of studies included.
2.5. Statistical analysis
Stata Statistical Software 15 was used for statistical analysis. Because all the indicators (serum ferritin, urinary hepcidin, fractional excretion (FE) of hepcidin, hepcidin/urine creatinine ratios) in this meta-analysis were continuous variables, the standardized mean difference (SMD) and 95% confidence interval (CI) were used as effect size measures. The original data are presented as the median (interquartile range) or median (min, max) and were converted to means ± standard deviations using the formula from Luo et al. (2018) [Citation19] and Wan et al. (2014) [Citation20]. Statistical heterogeneity was estimated by the Cochran Q test and the I2 statistic. Heterogeneity was considered significant when the Cochran Q test was p < 0.1 or I2 exceeded 50%. When p ≥ 0.1 and I2 ≤ 50%, the fixed effects model was used; when p < 0.1 and I2 > 50%, the random effects model was used. Additionally, the sources of heterogeneity were analyzed by subgroup analysis and by rereading the full text.
In addition, to examine the reliability and stability of our meta-analyses, sensitivity analysis was carried out by comparing the point and interval estimates of combined effect sizes when different effect models were used and by using the leave-one-out method. As fewer than 10 articles were included in this meta-analysis, publication bias was assessed by Egger’s test and the trim-and-fill method.
3. Results
3.1. Study inclusion
The process of study selection is shown in . The electronic search and other search methods yielded 179 studies. After removing duplicates, 127 publications remained, of which 41 were excluded after the title and abstract reading (κ = 0.68). The full texts of 27 citations were screened against the inclusion/exclusion criteria (κ = 0.85). There was a high level of interrater agreement in determining the final study cohort from the 27 screened citations (κ = 0.83). Ultimately, 9 studies met the inclusion criteria and were included in the meta-analysis. All 9 included studies had NOS scores ≥ 6. The interrater agreement data are shown in Supplementary material Table 4.
3.2. Results of meta-analysis
3.2.1. Relationship between serum ferritin and AKI incidence
Three cohort studies [Citation16,Citation21,Citation22] compared the levels of baseline serum ferritin (μg/L) in patients undergoing cardiac surgery who developed AKI with those who did not. The total number of participants in the study was 451 (103 patients in the AKI group and 348 patients without AKI). The fixed effects model was used because the meta-analysis revealed no significant heterogeneity among the studies (p = 0.173, I2 = 43%). The results showed that patients who developed AKI after cardiac surgery had lower baseline serum ferritin levels than those who did not develop AKI (SMD = −0.30, 95% CI:-0.54 to −0.07, p = 0.010, ), indicating that serum ferritin (μg/L) was a protective factor for AKI after cardiac surgery.
Figure 2. Forest plot of the incidence of acute kidney injury after cardiac surgery and serum ferritin. Forest plot revealing that patients who developed AKI after cardiac surgery had lower baseline serum ferritin levels than those who did not develop AKI (p = 0.010). 95% CI, 95% confidence interval.
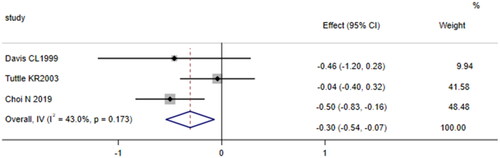
3.2.2. Relationship between plasma hepcidin and AKI incidence
Two papers [Citation23,Citation24] compared the levels of preoperative and 6-h postoperative plasma hepcidin (μg/L) in patients undergoing cardiac surgery who developed AKI with those who did not. Substantial between-study heterogeneity was identified (I2 = 93.8%, p < 0.001; I2 = 94.9%, p < 0.001); therefore, the random effects model was used. The results showed no significant difference in plasma hepcidin levels between patients undergoing cardiac surgery who developed AKI and those who did not (SMD = −0.71, 95% CI: −2.42 to 1.00, p = 0.416; SMD = −0.60, 95% CI: −2.50 to 1.30, p = 0.539; ).
Figure 3. Forest plot of the incidence of acute kidney injury after cardiac surgery and plasma hepcidin. Forest plot indicating no significant difference in plasma hepcidin levels between patients undergoing cardiac surgery who developed AKI and those who did not (p = 0.416; p = 0.539). 95% CI, 95% confidence interval.
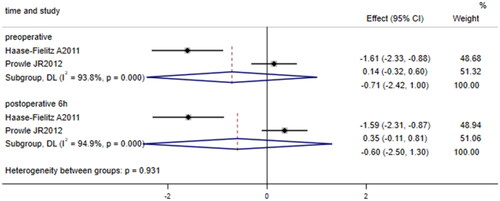
3.2.3. Relationship between urinary hepcidin and AKI incidence
Five papers [Citation15, Citation23, Citation25–27] compared the levels of urinary hepcidin (μg/L) between patients undergoing cardiac surgery who developed AKI and those who did not. Two of these studies [Citation23, Citation25] compared the levels of urinary hepcidin before surgery, two studies [Citation15, Citation23] compared the levels of 6-h postoperative urinary hepcidin, and three studies [Citation23, Citation26, Citation27] compared the levels of 24-h postoperative urinary hepcidin. The fixed effects model was used to pool data on preoperative and 24-h postoperative urine hepcidin because no significant heterogeneity was identified (I2 = 33.3%, p = 0.221; I2 = 0.0%, p = 0.086). The random effects model was used to pool data on 6-h postoperative urinary hepcidin because heterogeneity was identified between studies (I2 = 71.2%, p = 0.063). The results showed that after cardiac surgery, the level of 24-h postoperative urinary hepcidin in patients who developed AKI was lower than that in patients who did not develop AKI (SMD = −0.60, 95% CI: −0.82 to −0.37, p < 0.001); however, preoperative and 6-h postoperative urinary hepcidin levels were not significantly different between patients who developed AKI and those who did not develop AKI (SMD = −0.22, 95% CI: −0.60 to 0.16, p = 0.254; SMD = −0.24, 95% CI: −1.01 to 0.53, p = 0.542, ).
Figure 4. Forest plot of the incidence of acute kidney injury after cardiac surgery and urinary hepcidin. Forest plot showing that after cardiac surgery, the level of 24-h postoperative urinary hepcidin in patients who developed AKI was lower than that in patients who did not develop AKI (p = 0.000); however, preoperative and 6-h postoperative urinary hepcidin levels were not significantly different between patients who developed AKI and those who did not develop AKI (p = 0.254; p = 0.542). 95% CI, 95% confidence interval.
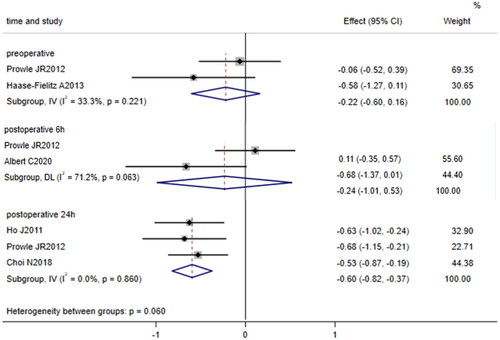
3.2.4. Relationship between urine hepcidin/urine creatinine ratio and AKI incidence
Four studies [Citation23, Citation24, Citation26, Citation27] compared the 24-h postoperative urinary hepcidin/urine creatinine ratio (μg/mmol) between patients undergoing cardiac surgery who developed AKI and those who did not, and two of these studies [Citation23, Citation24] compared the 6-h postoperative urine hepcidin/urine creatinine ratio between patients undergoing cardiac surgery who developed AKI and those who did not. The fixed effects model was used to pool data on the 24-h postoperative urine hepcidin/urine creatinine ratio because no significant heterogeneity was identified (I2 = 0.0%, p = 0.711), while the random effects model was used to pool data on the 6-h postoperative urine hepcidin/urine creatinine ratio because heterogeneity was substantial (I2 = 71.4%, p = 0.061). The results showed that after cardiac surgery, the 24-h postoperative urinary hepcidin/urine creatinine ratio in patients who developed AKI was lower than that in patients who did not develop AKI (SMD = −0.65, 95% CI: −0.86 to −0.43, p < 0.001, ); however, the 6-h postoperative urinary hepcidin/urine creatinine ratio did not significantly differ between patients who developed AKI and those who did not develop AKI (SMD = −0.35, 95% CI: −1.12 to 0.42, p = 0.377, ).
Figure 5. Forest plot of the incidence of acute kidney injury after cardiac surgery and urinary hepcidin/urine creatinine ratio. Forest plot indicating that patients who developed AKI after cardiac surgery had a lower 24-h postoperative urinary hepcidin/urine creatinine ratio than those who did not develop AKI after cardiac surgery (p = 0.000); however, there was no significant difference in the 6-h postoperative urinary hepcidin/urine creatinine ratio between patients who developed AKI and those who did not develop AKI (p = 0.377). 95% CI, 95% confidence interval.
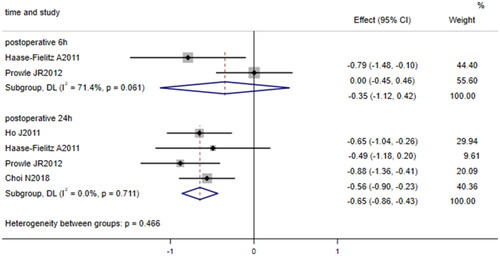
3.2.5. Relationship between urine FE of hepcidin and AKI incidence
Two studies [Citation23, Citation24] compared the levels of preoperative, 6-h postoperative, and 24-h postoperative urine FE of hepcidin (%) between patients undergoing cardiac surgery who developed AKI and those who did not. The fixed effects model was used to pool data on the preoperative and 6-h postoperative urine FE of hepcidin because no significant heterogeneity was identified (I2 = 0.0%, p = 0.630; I2 = 27.0%, p = 0.242), while the random effects model was used to pool data on the 24-h postoperative urine FE of hepcidin because heterogeneity was substantial (I2 = 68.4%, p = 0.075). The results showed that after cardiac surgery, the levels of preoperative and 6-h postoperative urine FE of hepcidin (SMD = −0.41, 95% CI: −0.79 to −0.02, p = 0.038; SMD = −0.49, 95% CI: −0.88 to −0.11, p = 0.012, ) were lower in patients who developed AKI than in patients who did not develop AKI; however, the levels of 24-h postoperative urine FE of hepcidin did not significantly differ between patients who developed AKI and those who did not develop AKI (SMD = −0.13, 95% CI: −0.86 to 0.60, p = 0.726, ).
Figure 6. Forest plot of the incidence of acute kidney injury after cardiac surgery and urinary FE of hepcidin. Forest plot displaying that the levels of preoperative and 6-h postoperative urine FE of hepcidin (p = 0.038; p = 0.012) in patients who developed AKI after cardiac surgery were lower than those in patients who did not develop AKI after cardiac surgery; however, there was no significant difference in the levels of 24-h postoperative FE of hepcidin between patients who developed AKI after cardiac surgery and those who did not develop AKI after cardiac surgery (p = 0.726). 95% CI, 95% confidence interval.
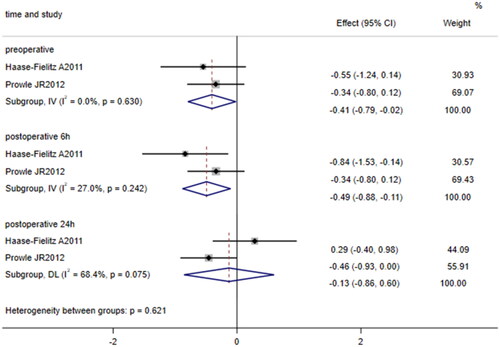
3.3. Analysis of publication bias
In this study, 9 studies were included; since fewer than 10 studies were included, Egger’s test was used to evaluate publication bias. When the p value for Egger’s test was < 0.05 or was not shown, the trim-and-fill method was used to analyze publication bias. The results showed no significant changes in the statistical effect sizes of most indicators before and after the trim-and-fill method, suggesting that publication bias had little effect on the results (see Supplementary material Table 2).
3.4. Sensitivity analysis
Sensitivity analysis was performed by comparing the point and interval estimates of the combined effect sizes between different models (random effects models vs. fixed effects models). The results showed that the combined effect size of serum ferritin significantly differed based on the model, while the other effect sizes of the other indicators did not significantly differ based on the model used to pool data (see Table 3 in the Supplementary material). Therefore, the leave-one-out method was further used for sensitivity analysis. The combined effect size of serum ferritin did not significantly change after excluding a single study (see in the Supplementary material). These analyses indicate that the results of the meta-analysis were stable.
3.5. Heterogeneity analysis
After reviewing the full text, we found that the source of the heterogeneity may be the definition of the sampling time point. The time point of preoperative sampling was not consistent across studies: the sampling time point of 6 h after surgery in the study by Prowle JR [Citation23] et al. was 3.58 to 9.13 h after CPB.
4. Discussion
After applying the inclusion and exclusion criteria, 9 articles on iron metabolism-related indicators and the incidence of AKI after cardiac surgery were included in this study. Meta-analysis revealed that after cardiac surgery, the levels of baseline serum ferritin (μg/L), preoperative and 6-h postoperative FE of hepcidin (%), 24-h postoperative urinary hepcidin (μg/L) and the urine hepcidin/urine creatinine ratio (μg/mmol) were lower in patients with AKI than in patients without AKI.
Ferritin is a ubiquitously expressed spherical protein that consists of 24 subunits of heavy (FtH) and light (FtL) chains. FtH is a ferroxidase that converts ferrous iron into ferric iron, which is subsequently stored within the ferritin shell. FtL does not have ferroxidase activity, and its presumed function is limited to iron nucleation [Citation28]. Iron overload in the body can lead to simultaneous elevations of FtH and FtL [Citation29, Citation30]. Intracellular tissue ferritin mostly consists of FtH, whereas serum ferritin, a subgroup of intracellular ferritin, mostly consists of FtL [Citation31], which is low in iron and mainly originates from macrophages and renal tubular epithelial cells [Citation32]. The level of serum ferritin can indirectly reflect the level of FTH in the body. Zarjou A et al. [Citation30] found that FtL attenuated mitogen-activated protein kinase (MAPK) and nuclear factor kappa-B (NF-κB) activation, which play an important role in ischemia–reperfusion-induced AKI [Citation33]. The results of our study also showed that patients with higher baseline serum ferritin levels have a low probability of developing AKI after cardiac surgery. It is further suggested that high serum ferritin levels may not only reflect iron levels in the body but also reflect the body’s immunoregulatory capacity and ability to rapidly process catalytic iron release in the extracorporeal circulation [Citation16]. The kidney is a highly metabolic organ, and approximately 20% to 25% of the cardiac output goes to the kidney. After cardiac surgery, a large amount of free iron is released into the circulatory system and then flows to the kidney, where it is reabsorbed [Citation31, Citation34]. The ability to handle iron release is limited in patients with low levels of baseline serum ferritin, leading to renal iron overload and the development of AKI. Therefore, patients with low baseline serum ferritin levels should be made aware of the risk of AKI after cardiac surgery. In addition, animal experiments showed that exogenous FLT could alleviate lipopolysaccharide-induced hyperinflammation, reduce organ dysfunction and improve the survival rate in mice [Citation30, Citation35]. Therefore, ferritin may be a therapeutic target for AKI after cardiac surgery.
Hepcidin, the only protein known to regulate the iron concentration in humans, is synthesized not only in the liver but also in the distal nephron [Citation36]. Hepcidin can mediate ferroportin degradation, leading to an increase in intracellular iron, followed by an increase in FtH to compound free iron, which in turn reduces free iron-mediated lipid peroxidation [Citation31]. Under physiological conditions, circulating hepcidin is freely filtered by glomeruli and reabsorbed by macroprotein-dependent endocytosis in the proximal tubule, with reabsorption up to 97% [Citation37, Citation38]. However, hepcidin that is synthesized by epithelial renal tubule and duct cells can be released luminally into the urine, where it apparently escapes tubular proteolysis and recycling [Citation39]. The results of this study showed that after cardiac surgery, patients with lower levels of preoperative and 6-h postoperative FE of hepcidin, lower levels of 24-h postoperative urine hepcidin, and lower urine hepcidin/urine creatinine ratios are more likely to develop AKI. However, no correlation between plasma hepcidin and the incidence of AKI was found, suggesting that the production of hepcidin locally in the kidney can contribute greatly to the protection of kidney injury. The urine hepcidin/urine creatinine ratio has been found to be a more effective predictor of the incidence of AKI than serum creatinine [Citation40]. Combined with the results of this meta-analysis, these findings suggest that preoperative and 6-h postoperative urine FE of hepcidin, 24-h postoperative urine hepcidin/urine creatinine ratio and urinary hepcidin may replace urine creatinine as early diagnostic indicators and predictors of AKI. Moreover, the administration of exogenous hepcidin has been found to significantly improve ischemia–reperfusion-induced renal injury [Citation41], suggesting that hepcidin may be used in the treatment of AKI after cardiac surgery in the future.
However, 24-h postoperative FE of hepcidin, preoperative and 6-h postoperative urine hepcidin, and 6-h postoperative urine hepcidin/urine creatinine ratios were not found to be associated with AKI after cardiac surgery in the study, which may be related to the time point of the increase in plasma hepcidin and the time point at which the kidney locally begins to produce hepcidin. Xie GL et al. [Citation42] found that the expression of ferroportin decreased at 4 h after renal ischemia–reperfusion and decreased to the lowest level at 24 h after renal ischemia–reperfusion. The degradation of ferroportin was mediated by hepcidin. Therefore, hepcidin may be produced locally in the kidney 4 h after ischemia–reperfusion and reach its peak at 24 h after ischemia–reperfusion. In addition, the study found that plasma hepcidin began to increase at 8 h after renal ischemia–reperfusion, peaked after 16 h, and remained significantly higher than the baseline level after 24 h. Therefore, the preoperative FE of hepcidin may reflect the renal blood flow and glomerular filtration rate [Citation23], and the 6-h postoperative FE of hepcidin may reflect not only these two but also the ability of the kidneys to produce hepcidin locally. However, due to the increase in plasma hepcidin and the local production of hepcidin in the kidney, the 24-h postoperative FE of hepcidin may not necessarily be related to renal function. Urinary hepcidin is mainly derived from glomerular filtration and local production in the kidney. Before and 6 h after cardiac surgery, the local production of hepcidin in the kidney is lower, and urinary hepcidin is mainly derived from glomerular filtration, which is greatly affected by the individual’s baseline plasma hepcidin. Therefore, preoperative and 6-h postoperative urine hepcidin, as well as 6-h postoperative urine hepcidin/urine creatinine ratios, may not be strongly related to the occurrence of AKI after cardiac surgery. Twenty-four hours after cardiac surgery, the local production of hepcidin in the kidney reaches a peak, and urinary hepcidin is mainly locally produced by the kidney, which is less affected by the individual’s baseline plasma hepcidin. Therefore, the 24-h postoperative urinary hepcidin and urine hepcidin/urine creatinine ratio may primarily reflect the ability of the kidney to locally produce hepcidin.
Regarding the respective relationship between transferrin saturation, serum iron, urine catalytic iron and AKI after cardiac surgery, no meta-analysis was performed because only one article for each relationship was found during the literature search. Akrawinthawong K et al. [Citation43] studied the relationship between urine catalytic iron and AKI after cardiac surgery and found that patients with AKI after cardiac surgery showed a significant increase in urine catalytic urinary iron 8 h after surgery, while creatinine levels did not change significantly until 12 h later. Thus, urine catalytic iron may predict AKI earlier than creatinine after cardiac surgery. Choi N et al. [Citation16] found that higher transferrin saturation at 1 h after cardiopulmonary bypass was an independent predictor of acute kidney injury, and animal experiments have also revealed that intraperitoneal injection of unconjugated ferrotransferrin can reduce free iron in the circulation of ischemia–reperfusion mice, eliminate superoxide formation in the kidney, and reduce postischemic inflammation characterized by neutrophil infiltration [Citation44], suggesting that transferrin saturation can be used as an early predictor of AKI after cardiac surgery and that unconjugated ferrotransferrin can be used to enhance endogenous iron binding capacity. These findings reveal a potential treatment strategy for acute renal failure.
The level of heterogeneity was high for some indicators in this meta-analysis. However, the level of heterogeneity was low for the meaningful indicators, suggesting that the results of this meta-analysis are reliable.
4.1. Study strengths and limitations
Our study has several important strengths. This study is the first meta-analysis to examine the relationship between iron metabolism-related metabolic indicators and AKI after cardiac surgery. The quality of the evidence was high because all included studies were cohort studies rather than case–control studies. This study has potential clinical value for the early identification and prediction of AKI after clinical cardiac surgery. Our study also has some limitations. Because few studies have examined the relationship between serum iron, serum transferrin, urine catalytic iron and AKI after cardiac surgery, no meta-analysis was performed. At present, few relevant studies are available, and few studies were consequently included in this paper. Therefore, more large-scale, multicenter clinical and basic prospective studies are needed to confirm this hypothesis in the future.
5. Conclusion
In summary, the results of this study show that ferritin and hepcidin are protective factors against the incidence of AKI after cardiac surgery. Patients with low levels of baseline serum ferritin (μg/L), low levels of preoperative and 6-h postoperative FE of hepcidin (%), low 24-h postoperative hepcidin/urine creatinine ratios (μg/mmol) and low levels of 24-h postoperative urinary hepcidin (μg/L) are more likely to develop AKI after cardiac surgery. Therefore, in the future, these parameters have the potential to be predictors for AKI after cardiac surgery; ferritin, transferrin and hepcidin may be targets for the clinical treatment of AKI after cardiac surgery.
Amendments to the information provided at registration or in the protocol
The study population was changed from critically ill patients to patients undergoing cardiac surgery.
Ethics approval and consent to participate
An ethics statement is not applicable because this study is based exclusively on published literature.
Author contributions
Xiaoshuang Zhou designed and outlined the work; Shengchao Zhang revised the whole paper; Limei Zhao, Xiaoyu Yang and Xiaoshuang Zhou selected studies, evaluated the quality of the included studies, and drafted and revised the manuscript. All authors approved the article’s final version and agreed to be accountable for all aspects of the work.
Supplemental Material
Download PDF (224.1 KB)Disclosure statement
No potential conflict of interest was reported by the author(s).
Data availability statement
All data analyzed during this study are available in the public domain.
Additional information
Funding
References
- Mavromatidis K, Fytil C, Kynigopoulou P, et al. Serum ferritin levels are increased in patients with acute renal failure. Clin Nephrol. 1998;49(5):1–12.
- Chertow GM, Burdick E, Honour M, et al. Acute kidney injury, mortality, length of stay, and costs in hospitalized patients. J Am Soc Nephrol. 2005;16(11):3365–3370.
- Hobson CE, Yavas S, Segal MS, et al. Acute kidney injury is associated with increased long-term mortality after cardiothoracic surgery. Circulation. 2009;119(18):2444–2453.
- O’Neal JB, Shaw AD, Billings F. Acute kidney injury following cardiac surgery: current understanding and future directions. Crit Care. 2016;20(1):187.
- Bonventre JV, Weinberg JM. Recent advances in the pathophysiology of ischemic acute renal failure. J Am Soc Nephrol. 2003;14(8):2199–2210.
- Denton MD, Chertow GM, Brady HR. Renal-dose dopamine for the treatment of acute renal failure: scientific rationale, experimental studies and clinical trials. Kidney Int. 1996;50(1):4–14.
- Mishra J, Mori K, Ma Q, et al. Amelioration of ischemic acute renal injury by neutrophil gelatinase-associated lipocalin. J Am Soc Nephrol. 2004;15(12):3073–3082.
- Meersch M, Schmidt C, Hoffmeier A, et al. Prevention of cardiac surgery-associated AKI by implementing the KDIGO guidelines in high risk patients identified by biomarkers: the PrevAKI randomized controlled trial. Intensive Care Med. 2017;43(11):1551–1561.
- Ostermann M, Joannidis M. Acute kidney injury 2016: diagnosis and diagnostic workup. Crit Care. 2016;20(1):299.
- Ramakrishnan M, Arivalagan J, Satish L, et al. Selenium: a potent regulator of ferroptosis and biomass production. Chemosphere. 2022;306:135531.
- Borawski B, Malyszko J. Iron, ferroptosis, and new insights for prevention in acute kidney injury. Adv Med Sci. 2020;65(2):361–370.
- Linkermann A, Skouta R, Himmerkus N, et al. Synchronized renal tubular cell death involves ferroptosis. Proc Natl Acad Sci U S A. 2014;111(47):16836–16841.
- Stoppe C, Averdunk L, Goetzenich A, et al. The protective role of macrophage migration inhibitory factor in acute kidney injury after cardiac surgery. Sci Transl Med. 2018;10(441):eaan4886.
- Leaf DE, Rajapurkar M, Lele SS, et al. Increased plasma catalytic iron in patients may mediate acute kidney injury and death following cardiac surgery. Kidney Int. 2015;87(5):1046–1054.
- Albert C, Haase M, Albert A, et al. Urinary biomarkers may complement the cleveland score for prediction of adverse kidney events after cardiac surgery: a pilot study. Ann Lab Med. 2020;40(2):131–141.
- Choi N, Whitlock R, Klassen J, et al. Early intraoperative iron-binding proteins are associated with acute kidney injury after cardiac surgery. J Thorac Cardiovasc Surg. 2019;157(1):287–297.e282.
- Page MJ, McKenzie JE, Bossuyt PM, et al. The PRISMA 2020 statement: an updated guideline for reporting systematic reviews. BMJ. 2021;372:n71.
- Kellum JA, Lameire N, Diagnosis, evaluation, and management of acute kidney injury: a KDIGO summary (part 1). Crit Care. 2013;17(1):204.
- Luo D, Wan X, Liu J, et al. Optimally estimating the sample mean from the sample size, median, mid-range, and/or mid-quartile range. Stat Methods Med Res. 2018;27(6):1785–1805.
- Wan X, Wang W, Liu J, et al. Estimating the sample mean and standard deviation from the sample size, median, range and/or interquartile range. BMC Med Res Methodol. 2014;14:135.
- Tuttle KR, Worrall NK, Dahlstrom LR, et al. Predictors of ARF after cardiac surgical procedures. Am J Kidney Dis. 2003;41(1):76–83.
- Davis CL, Kausz AT, Zager RA, et al. Acute renal failure after cardiopulmonary bypass in related to decreased serum ferritin levels. J Am Soc Nephrol. 1999;10(11):2396–2402.
- Prowle JR, Ostland V, Calzavacca P, et al. Greater increase in urinary hepcidin predicts protection from acute kidney injury after cardiopulmonary bypass. Nephrol Dial Transplant. 2012;27(2):595–602.
- Haase-Fielitz A, Mertens PR, Plass M, et al. Urine hepcidin has additive value in ruling out cardiopulmonary bypass-associated acute kidney injury: an observational cohort study. Crit Care. 2011;15(4):R186.
- Haase-Fielitz A, Plaß M, Kuppe H, et al. Low preoperative hepcidin concentration as a risk factor for mortality after cardiac surgery: a pilot study. J Thorac Cardiovasc Surg. 2013;145(5):1380–1386.
- Choi N, Rigatto C, Zappitelli M, et al. Urinary hepcidin-25 is elevated in patients that avoid acute kidney injury following cardiac surgery. Can J Kidney Health Dis. 2018;5:2054358117744224.
- Ho J, Reslerova M, Gali B, et al. Urinary hepcidin-25 and risk of acute kidney injury following cardiopulmonary bypass. Clin J Am Soc Nephrol. 2011;6(10):2340–2346.
- Bradley JM, Le Brun NE, Moore GR. Ferritins: furnishing proteins with iron. J Biol Inorg Chem. 2016;21(1):13–28.
- Kawabata H. Transferrin and transferrin receptors update. Free Radic Biol Med. 2019;133:46–54.
- Zarjou A, Black LM, McCullough KR, et al. Ferritin light chain confers protection against sepsis-induced inflammation and organ injury. Front Immunol. 2019;10:131.
- Scindia PD, Leeds MJ, Swaminathan MS. Iron homeostasis in healthy kidney and its role in acute kidney injury. Semin Nephrol. 2019;39(1):76–84.
- Cohen LA, Gutierrez L, Weiss A, et al. Serum ferritin is derived primarily from macrophages through a nonclassical secretory pathway. Blood. 2010;116(9):1574–1584.
- Wu CK, Wu CL, Lee TS, et al. Renal tubular epithelial TRPA1 acts as an oxidative stress sensor to mediate ischemia-reperfusion-induced kidney injury through MAPKs/NF-κB signaling. Int J Mol Sci. 2021;22(5):2309.
- Haase M, Bellomo R, Haase-Fielitz A. Novel biomarkers, oxidative stress, and the role of labile iron toxicity in cardiopulmonary bypass-associated acute kidney injury. J Am Coll Cardiol. 2010;55(19):2024–2033.
- McCullough K, Bolisetty S. Iron homeostasis and ferritin in sepsis-associated kidney injury. Nephron. 2020;144(12):616–620.
- Houamel D, Ducrot N, Lefebvre T, et al. Hepcidin as a major component of renal antibacterial defenses against uropathogenic Escherichia coli. J Am Soc Nephrol. 2016;27(3):835–846.
- Peters HP, Laarakkers CM, Pickkers P, et al. Tubular reabsorption and local production of urine hepcidin-25. BMC Nephrol. 2013;14:70.
- van Swelm RP, Wetzels JF, Verweij VG, et al. Renal handling of circulating and renal-synthesized hepcidin and its protective effects against hemoglobin-mediated kidney injury. J Am Soc Nephrol. 2016;27(9):2720–2732.
- Kulaksiz H, Theilig F, Bachmann S, et al. The iron-regulatory peptide hormone hepcidin: expression and cellular localization in the mammalian kidney. J Endocrinol. 2005;184(2):361–370.
- Afsar RE, Kanbay M, Ibis A, et al. In-depth review: is hepcidin a marker for the heart and the kidney? Mol Cell Biochem. 2021;476(9):3365–3381.
- Scindia Y, Dey P, Thirunagari A, et al. Hepcidin mitigates renal ischemia-reperfusion injury by modulating systemic iron homeostasis. J Am Soc Nephrol. 2015;26(11):2800–2814.
- Xie GL, Zhu L, Zhang YM, et al. Change in iron metabolism in rats after renal ischemia/reperfusion injury. PLOS One. 2017;12(4):e0175945.
- Akrawinthawong K, Shaw MK, Kachner J, et al. Urine catalytic iron and neutrophil gelatinase-associated lipocalin as companion early markers of acute kidney injury after cardiac surgery: a prospective pilot study. Cardiorenal Med. 2013;3(1):7–16.
- de Vries B, Walter SJ, von Bonsdorff L, et al. Reduction of circulating redox-active iron by apotransferrin protects against renal ischemia-reperfusion injury. Transplantation. 2004;77(5):669–675.