Abstract
Introduction
Nephrotoxic medication (NTM) is one of the common causes of acute kidney injury (AKI) in critically ill infants. Current knowledge about the long-term effects of NTM exposure and associated AKI during the neonatal period and early infancy is limited. Hence, we aimed to explore the risk of chronic kidney disease (CKD) after NTM-AKI in this age group.
Methods
We performed a cross-sectional study including children 2–7 years of age, who had a history of high NTM exposure during NICU hospitalization. Cases and controls were defined as children who developed AKI and who did not develop AKI after NTM exposure, respectively. The primary outcome of interest was to explore the prevalence of composite CKD. In addition, we explored differences in urinary biomarker kidney injury molecule-1 (KIM-1) between the groups.
Results
We enrolled 48 children, 18 cases and 30 controls in which 25/48 (52%) had at least one finding of CKD. The composite CKD outcome tended to be higher in cases vs controls (61.1% vs. 46.6%, odds ratio = 1.79 (95% confidence interval 0.54-5.8)); however, this was not statistically significant. Median urinary KIM-1 value trended higher in controls, 0.367(0.23-0.59) vs. 0.20 (IQR 0.11-0.47), which was not statistically significant.
Conclusion
In this study, 52% of children exposed to NTM had at least one marker of CKD at a median age of 5 years. Multicenter, large prospective studies are needed to improve our understanding of the natural course of NTM-AKI and to determine risk factors and strategies to reduce CKD in this high-risk population.
Introduction
Critically ill neonates and infants hospitalized in the neonatal intensive care unit (NICU) are at high risk for AKI [Citation1,Citation2]. One-fourth of critically ill neonates admitted to intensive care develop at least 1 episode of AKI. In addition to hypovolemia, perinatal asphyxia, sepsis, vascular thrombosis, and ischemia after cardiac surgery for congenital heart defects, NTM (nephrotoxic medication) exposure is one of the most common causes of neonatal AKI [Citation3,Citation4].
NTM use is extremely common in NICU [Citation5]. Barhight et al. showed that 84% of neonates with a birth weight of <1500 grams and or gestation age of <30 weeks had at least one NTM exposure [Citation6]. Mohamed et al. found that 74% of neonates regardless of gestation age and 90–95% of neonates born ≤28-week gestational age were treated with at least one NTM [Citation7]. In March 2015, Children’s of Alabama implemented a quality improvement project (Baby NINJA) designed to reduce the rates of AKI due to NTM exposure in infants hospitalized in NICU. Stoops et al. documented 476 individual high NTM exposure events during 18 months period with 19.7% of those exposed developing AKI. After the implementation of this protocol, the rate of high NTM exposure was reduced by 42%, the rate of AKI by 78%, the percentage of AKI in those with high NTM exposure by 64%, and the number of AKI days by 68% [Citation8]. The long-term implication of NTM exposure and the presence of NTM-associated AKI during the NICU course has yet to be explored.
Several adult and pediatric studies have demonstrated that AKI may not be a completely reversible event and those with AKI are at risk for CKD [Citation9–17]. Proposed mechanisms underlying the progression of CKD following AKI include nephron loss which leads to hyperfiltration, vascular insufficiency due to endothelial dysfunction, maladaptive repair due to unchecked infiltration of inflammatory cells and myofibroblasts causing tubulointerstitial fibrosis and glomerulosclerosis (11). Literature review shows a wide range (10–70%) of CKD rates after AKI in children. Menon et al. showed that 70% of non-critically sick children who developed NTM-AKI, had at least one sign of CKD at 6 months follow-up [Citation17].
Recent evidence indicates that prematurity and low birth weight are risk factors for the development of CKD [Citation18–23]. Whether neonatal AKI is a risk factor for CKD has yet to be determined. Harer et al. showed higher CKD rates at 5 years of age in neonates who had AKI compared with the group without AKI [Citation16]. On the contrary, Cooper et al. performed a follow-up study 5–7 years after cardiopulmonary bypass surgery for a congenital heart defect and did not find a significant difference between mean eGFR, proteinuria and hypertension in neonates with and without AKI [Citation24]. The long-term prognosis after neonatal NTM-associated AKI is unknown.
Hence, we conducted a cross-sectional study to improve our understanding of CKD in children who were exposed to NTM in the NICU. We aimed to determine the prevalence and risk factors for developing CKD in this fragile population. Our primary hypothesis was that at 2-5 years of follow-up, children who developed NTM-associated AKI in infancy are at higher risk of CKD compared to the infants who had NTM exposure but did not develop AKI.
Material and methods
Population
We performed a cross-sectional follow-up study between December 2020 to February 2022, on children between 2–7 years of age, who were hospitalized in a level IV NICU and were enrolled in the Baby NINJA QI project between March 2015 and August 2018. In this QI project, patients who had a high NTM exposure, defined as having 3 nephrotoxic medications within 24 h or at least four calendar days of an intravenous aminoglycoside exposure, were tracked. AKI was defined as a rise in SCr by 50% or more and SCr value of at least 0.5 mg/dl. Specifics of the project have been previously published (8).
Inclusion criteria were a history of high NTM exposure during NICU stay (we used the exact same list of nephrotoxic medications used in the baby NINJA project) and at least 2 years of age at the time of enrollment. Babies transferred from an outside hospital to UAB NICU with a history of nephrotoxic medication exposure were included. Exclusion criteria included (a) congenital malformation of the kidney and urinary tract, (b) history of AKI not associated with NTM exposure and (c) history of cardiopulmonary bypass surgery. This study was approved by the institutional review board, University of Alabama at Birmingham (UAB IRB). Informed written consent from the parent or legal guardian was obtained before enrollment in the study.
Demographics
Clinical and demographic information was collected from the electronic medical records. Data collected from maternal history included the presence of diabetes and hypertension during pregnancy. Data collected from the neonates included gestational age, birth weight, small for gestation age, Apgar score at 5-min, history of necrotizing enterocolitis needing surgical intervention, history of patent ductus arteriosus needing medical or surgical intervention, history of chronic lung disease due to bronchopulmonary dysplasia with the ongoing need of oxygen support at the time of follow up, post-conception age at the time of first NTM exposure (small subset of participants had multiple NTM exposure events but we included the age at the time of first NTM exposure only), NTM exposure days, type of NTM exposure.
We categorized NTM exposure in 4 different categories, Vancomycin with or without other NTM other than Aminoglycoside labeled as (V), Aminoglycoside with or without other NTM other than Vancomycin labeled as (A), Vancomycin and Aminoglycoside exposure labeled as (VA), Other NTM medication exposure in addition to Vancomycin and Aminoglycoside labeled as (VA + 1). Other clinical variables analyzed in the participants with NTM-AKI included, total AKI events, AKI days, peak SCr and need for kidney replacement therapy.
Outcomes
The primary outcome of interest was composite CKD outcome, defined by the presence of any one of the following: eGFR less than 90 mL/min/1.73 m2, random UPCR ≥ 0.2 mg/mg, and/or hypertension, systolic blood pressure (SBP) and/or diastolic blood pressure (DBP) ≥ 95th percentile for age, sex and height based on 2017 clinical practice guidelines at the time of follow up [Citation25].
We used CKiD under 25 (U 25) equations to estimate GFR using SCr and Cystatin C [Citation26]. Blood pressure was measured manually in the right upper extremity with an appropriately sized cuff in a seated position. Three readings were recorded at 2–5 min intervals and average SBP, DBP were calculated. Random urine sample was collected without using indwelling catheters. Urine was centrifuged at 1500 rpm for 10 min and stored at − 70 °C. Venous blood sample was collected at phlebotomy lab in our hospital. Serum was separated from whole blood followed by centrifugation at 2200 rpm for 15 min and stored at −70 °C. Both urine and blood samples were transported to Cincinnati Children’s Hospital lab under appropriate conditions for measurement of SCr, Cystatin C and urine protein, creatinine and KIM-1.
Secondary outcomes were the elements of the composite outcome evaluated as a continuous value. Because a substantial amount of kidney damage needs to occur before a decrease in glomerular filtration rate is evident, early markers of structural damage are being tested to better characterize kidney damage after AKI events. One such urinary biomarker for early CKD after AKI is KIM-1, a transmembrane protein which is low in normal kidneys but significantly expressed in damaged tubular epithelial cells [Citation27–34]. For these reasons, we evaluated KIM-1 as an exploratory outcome in addition to traditional CKD markers.
Laboratory analysis
Serum and urinary creatinine (mg/dl) were measured by enzymatic method. Serum Cystatin C (mg/L) was measured by particle-enhanced immunoturbidimetric assay (PETIA) [Citation35]. Urinary protein (mg/dl) was determined by the turbidimetric method [Citation36]. The mean minimum detectable dose (MDD) was 4 mg/dl, we assumed urinary protein to be 2 mg/dl for samples reported as <4 mg/dl because of the inability to further dilute the sample. Urinary KIM-1 (ng/ml) was determined by quantitative sandwich enzyme immunoassay technique [Citation37]. MDD ranged from 0.003–0.046 ng/ml. The mean MDD was 0.009 ng/mL, we assumed urinary KIM-1 to be 0.0045 ng/ml for samples reported as <0.009 mg/ml. We calculated UPCR (mg/mg) by dividing urine protein to urine creatinine, and urine KIM-1 Creatinine ratio (UKC, ng/mg) by dividing urine KIM1 to urine creatinine.
Statistical analysis
We used power and sample size calculation software (Vanderbilt, version 3.1.6) to calculate the sample size. We aimed for a power of 0.8 and one-sided alpha value of 0.05. Assuming an effect size of 33%, we needed at least 15 cases and 30 controls to be able to reject the null hypothesis. We used JMP (Cary, NC version 16.0) software for statistical analysis. Continuous variables were expressed as median with interquartile range (IQR). Non-continuous variables were expressed as proportions. Wilcoxon rank sum tests were used to compare continuous variables and Fisher’s exact or Chi-squared tests were used to compare categorical variables, as appropriate. p-value <0.05 was considered statistically significant.
Results
As noted in , between March 2015–August 2018, 466 infants were enrolled in the baby NINJA QI project. By the time of follow-up, 308 survived. Of the 308 survivors, 261 met the inclusion criteria, 36 in AKI group and 225 in non-AKI group. All 36 potential participants in AKI group were contacted, 10 denied to participate and 8 did not answer. Out of 225 potential participants in non-AKI group, 70 were contacted who had upcoming appointments at our hospital, 24 refused to participate, 16 did not answer. We recruited 18 participants in AKI and 30 in non- AKI group.
Figure 1. shows the flow chart of the study population recruitment. Of 466 eligible participants, 18 cases and 30 controls were enrolled for the study.
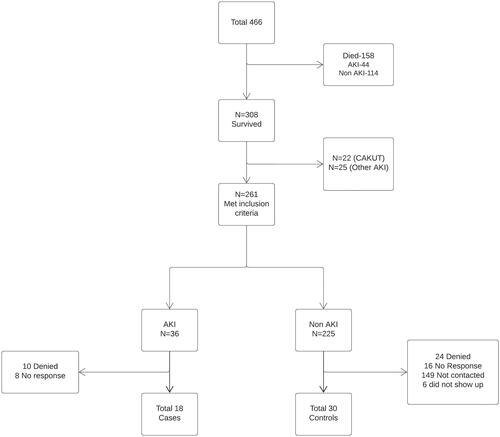
Baseline characteristics
No statistically significant difference was evident between groups as shown in except NTM exposure days (14 vs.7 days, p value = 0.001). The most common NTM exposure was Aminoglycoside (48.7%) followed by a combination of Vancomycin and Aminoglycoside (33.3%) followed by Vancomycin (12.5%). The median value of peak SCr was 0.6 mg/dl (IQR 0.5–0.9, max value 1.4), AKI events were 1 (IQR 1-1) and AKI days were 2 (IQR 1–5, max days 15). One participant received peritoneal dialysis.
Table 1. Demographic characteristics by AKI status.
Outcomes
CKD composite outcome trended higher in case vs. control, 11/18 (61.1%) vs. 14/30 (46.6%) but not statistically significant; p value of 0.33. Odds ratio of 1.79, 95% confidence interval 0.54–5.8. Children with a history of PDA needing medical or surgical intervention were found to be at statistically significantly higher risk of CKD; p value of 0.006. Clinical outcome by AKI status is shown in and baseline characteristics by composite CKD outcome are shown in .
Table 2. Clinical outcome by AKI status.
Table 3. Baseline characteristics by composite primary outcome (CKD).
Kidney function
An eGFR < 90 mL/min/1.73 m2 was present in 4/18 (22.2%) of cases and 5/30 (16.6%) of controls (p-value = 0.63). The median value of eGFR was 98 (IQR 90-104) vs 101 (IQR 93-113) in the case vs control group, respectively (p-value = 0.25).
Blood pressure
We obtained BP measurements for all 18 cases and 29 out 30 controls (one not obtained due to abnormal arm movements from an underlying neurologic issue). One participant from each case and control group was on Clonidine for neurologic reasons. Hypertension (SBP and or DBP ≥ 95th percentile) was found in 1 participant in the case and none in the control. SBP between 90–95th percentile was found in 1 participant in the case group. DBP between 90–95th percentile was found in 2 participants in the case group and 1 in the control group. The median value of average SBP was 91 mm Hg in both groups, and the median value of average DBP was 57 mm Hg and 54 mm Hg in the cases and control group respectively.
Proteinuria
We obtained a urine sample for all 18 cases and 29 out of 30 controls. Random UPCR was abnormal (≥ 0.2 mg/mg) in 8/18 (44.4%) of cases vs. 9/29 (31%) of controls (p = 0.31). The median value of UPCR was 0.17 mg/mg (IQR 0.12–0.30) in cases vs 0.15 (IQR 0.11- 0.23) in controls (p = 0.30).
Urine KIM-1
UKC (ng/mg) trended to be higher in the control group with a median value of 0.37 (0.23–0.59) vs. 0.20 (IQR 0.11–0.47) in cases, which was not statistically significant with p value of 0.12.
Baseline characteristics by composite outcome
Of the 48 children who returned for follow-up, 25/48 (52%) had at least one component of the composite CKD outcome as listed in . We did not find it to be strongly associated with any of the clinical variables except PDA requiring intervention. All participants with a history of PDA needing medical or surgical intervention had at least one finding of CKD risk at the time of follow up; p value of <0.05.
Discussion
We performed this study to look at long-term implications of NTM AKI encountered during neonatal and early infancy period. At a median age of 5 years, 52% of NICU graduates exposed to NTM had at least one marker of CKD risk which was higher in NTM- AKI group, 61% vs. 46%, but it was not statistically significant. An eGFR less than 90 mL/min/1.73 m2 was found in 22% vs. 16% in AKI and non-AKI group, respectively, but did not reach statistical significance.
Growing evidence indicates that AKI may not be completely reversible and it can progress to CKD. Observational studies in children have shown a wide range of incidence of CKD (10–70%) after AKI [Citation9–16]. However, existing evidence is limited due to the degree of heterogeneity in terms of study population, sample size, etiology and definition of AKI, measurement of kidney health-related outcomes, method of eGFR calculation and duration of follow-up.
Current evidence on long-term prognosis of NTM- AKI in children is limited. A retrospective study done by Menon et al. showed that 70% of non-critically ill children who had NTM-AKI had at least one sign of CKD 6 months post AKI event vs. 61% in our study [Citation17]. Their study population was composed of 100 children with a mean age of 9 years who were hospitalized under bone marrow transplant/oncology, gastroenterology, liver transplant, cardiology, or pulmonary service. High NTM exposure was defined as, an aminoglycoside for 3 or more days or 3 or more NTM medications within 24 h. CKD was defined as eGFR less than 90 or above 150 mL/min/1.73 m2, random UPC 0.3 or above and or hypertension. While we enrolled 48 children who were NICU graduates and had high nephrotoxic medication exposure, the median age at the time of follow-up was 5 years. We defined kidney dysfunction as eGFR less than 90, random UPCR 0.2 or above and or hypertension. The results of our study cannot be directly compared given the difference in the study population, risk of CKD in both case and control group because of a history of extreme prematurity and low birth weight, the definition of CKD and follow-up not limited to 6 months post NTM-AKI. Several differences are noteworthy; hence results cannot be directly compared.
Accurate data on the epidemiology of earlier stages of CKD after critical neonatal course in children is not well known since early CKD is mostly asymptomatic. Interestingly, our study showed that 52% of the study population had at least one finding of CKD at the time of follow up which is considerably higher than healthy 5-year-olds. As evidence shows that prematurity and low birth weight are strongly associated with the later development of CKD, due to low kidney reserve at birth, the development of AKI in this group could act as a potential effect modifier [Citation16,Citation18–23]. The majority of children enrolled in our study were extremely preterm and had low birth weight. The median gestation age in AKI and non-AKI group was 25 and 29 weeks, and the median birth weight was 695 and 1040 grams, respectively.
The FANCY study by Harer et al. assessed the risk of CKD in children born with very low birth weights with a history of AKI during NICU hospitalization [Citation16]. This study involved 34 (20 AKI, 14 non-AKI) children with a median age of 5 years at the time of follow-up. CKD was defined as UPCR above 0.2, BP > 95th percentile and or eGFR less than 90 mL/min/1.73 m2 but the equation of eGFR estimation was solely based on serum cystatin C, while we used pediatric U25-based equation using both serum creatinine ad cystatin C. They showed that 65% of VLBW infants with a history of AKI had at least one finding of CKD as compared to 14% of children with no history of AKI; p value 0.01, relative risk 4.5 (95% CI 1.2–17.1). Prevalence of overall composite CKD and low eGFR in AKI group were comparable to our study, 65% vs. 61% and 26% vs. 22%, respectively. Alternatively, we found a higher rate of CKD in the control group (46% vs. 14%) despite similar median gestation age (29 weeks) and birth weight (1040 grams) which could be due to selection bias.
Similarly, Abitbol et al. assessed the risk of CKD in children born with extreme prematurity and a history of AKI [Citation23]. This study involved 20 participants with mean gestation age of 25 weeks. At the median follow-up period of 6.6 years, 85% of children had reduced eGFR (less than 75 mL/min/1.73 m2) and 5 children had developed end-stage renal disease but the severity of AKI was high as compared to our study.
Like our study, the FRAIL-AKI study did not find a significant difference in the prevalence of CKD by conventional markers between those with vs. those without neonatal AKI after cardiopulmonary bypass surgery. They did however find higher urinary concentrations of KIM-1 in children who developed AKI compared with those who did not develop AKI [Citation24]. The median value of urinary KIM-1 creatinine ratio (UKC, ng/mg) was 0.619 and 0.352 in AKI and Non-AKI groups respectively; p value 0.03. Median UKC was 0.378 in healthy controls of 5–10 years of age. In our results, UKC was high in non-AKI group (0.366, IQR 0.226–0.59) as compared to AKI group (0.197, IQR 0.110-0.474) but it was not statistically significant. Existing knowledge is limited about age-adjusted normal values of urinary KIM-1. Hence, more work is needed to validate urinary biomarkers. Summary of studies looking at the risk of CKD after neonatal AKI is shown in .
Table 4. Comparison of studies looking at CKD risk in children with a history of AKI during infancy.
We looked at various neonatal/maternal clinical variables to determine if there was an association to CKD risk and found that PDA requiring intervention was significantly associated with CKD. All 7 participants who required medical or surgical intervention for PDA (one received Indomethacin, two received fluid restriction, three received Ibuprofen and one received surgical closure) had at least one finding of CKD risk. However, as we only had 7 participants that had PDA, this result should be interpreted with caution and warrants further study.
Median gestation age in CKD and non-CKD group was 29 and 27 weeks, median birth weight was 840 and 950 grams, respectively. We did not find a statistically significant association between these variables and CKD risk.
Our study has several strengths. It includes a relatively homogenous study population, and enrollment of children 2 years and above to mitigate the effect of functional immaturity of kidneys below this age. Additionally, we estimated GFR using the most up-to-date eGFR equation that incorporates both SCr and cystatin C. Despite these strengths, we acknowledge several limitations. We acknowledge that it was a single-center study with a small sample size. The study is at risk for selection bias, as many patients with severe NTM-AKI did not survive and may have had a higher risk of progression to CKD and one-third of the children in non-AKI group were contacted only. Orthostatic proteinuria cannot be ruled out as urine sample was collected at random times, which may have overestimated the prevalence of CKD. We diagnosed children with HTN at one time point, while the diagnosis of childhood hypertension is made when BP is elevated on three separate visits, to rule out white coat hypertension.
Conclusion
We aimed to evaluate the long-term consequences of NTM-associated AKI encountered during the neonatal period and early infancy. About half of our study population was found to be at risk of CKD which was higher in AKI group though not statistically significant. Large prospective studies are needed to improve our understanding of the long-term prognosis of NTM-AKI and to determine risk factors for the development of CKD in this high-risk population. Further research is required to find early urine biomarkers of kidney disease. Until strong evidence becomes available, children with a history of neonatal intensive care unit admission and NTM-AKI, need intermittent monitoring of kidney health.
Supplemental Material
Download PDF (114.6 KB)Disclosure statement
This statement is to certify that all Authors have seen and approved the manuscript being submitted. We warrant that the article is the Authors’ original work. We warrant that the article has not received prior publication and is not under consideration for publication elsewhere. On behalf of all Coauthors, the corresponding Author shall bear full responsibility for the submission. This research has not been submitted for publication nor has it been published in whole or in part elsewhere. We attest to the fact that all Authors listed on the title page have contributed significantly to the work, have read the manuscript, and attest to the validity and legitimacy of the data and its interpretation. The work for this study was performed at the University of Alabama at Birmingham.
Additional information
Funding
References
- Andreoli SP. Acute renal failure in the newborn. Semin Perinatol. 2004;28(2):1–8.
- Askenazi DJ, Ambalavanan N, Goldstein SL. Acute kidney injury in critically ill newborns: what do we know? What do we need to learn? Pediatr Nephrol. 2009;24(2):265–274.
- Jetton JG, Boohaker LJ, Sethi SK, et al. Incidence and outcomes of neonatal acute kidney injury (AWAKEN): a multicentre, multinational, observational cohort study. Lancet Child Adolesc Health. 2017;1(3):184–194.
- Askenazi DJ, Griffin R, McGwin G, et al. Acute kidney injury is independently associated with mortality in very low birthweight infants: a matched case-control analysis. Pediatr Nephrol. 2009;24(5):991–997.
- Rhone ET, Carmody JB, Swanson JR, et al. Nephrotoxic medication exposure in very low birth weight infants. J Matern Fetal Neonatal Med. 2014;27(14):1485–1490.
- Barhight M, Altaye M, Gist KM, et al. Nephrotoxic medications and associated acute kidney injury in very low birth weight infants. J Clin Nephrol Res. 2017;4(4):1070.
- Mohamed TH, Abdi HH, Magers J, et al. Nephrotoxic medications and associated acute kidney injury in hospitalized neonates. J Nephrol. 2022;35(6):1679–1687.
- Stoops C, Stone S, Evans E, et al. Baby NINJA (nephrotoxic injury negated by just-in-time action): reduction of nephrotoxic medication-associated acute kidney injury in the neonatal intensive care unit. J Pediatr. 2019;215:223–228.e226.
- Greenberg JH, Coca S, Parikh CR. Long-term risk of chronic kidney disease and mortality in children after acute kidney injury: a systematic review. BMC Nephrol. 2014; 15:184.
- Lebel A, Teoh CW, Zappitelli M. Long-term complications of acute kidney injury in children. Curr Opin Pediatr. 2020;32(3):367–375.
- Chawla LS, Eggers PW, Star RA, et al. Acute kidney injury and chronic kidney disease as interconnected syndromes. N Engl J Med. 2014;371(1):58–66.
- Goldstein SL, Jaber BL, Faubel S, et al. AKI transition of care: a potential opportunity to detect and prevent CKD. Clin J Am Soc Nephrol. 2013;8(3):476–483.
- Coca SG, Singanamala S, Parikh CR. Chronic kidney disease after acute kidney injury: a systematic review and meta-analysis. Kidney Int. 2012;81(5):442–448.
- Askenazi DJ, Feig DI, Graham NM, et al. 3–5 year longitudinal follow-up of pediatric patients after acute renal failure. Kidney Int. 2006;69(1):184–189.
- Mammen C, Al Abbas A, Skippen P, et al. Long-term risk of CKD in children surviving episodes of acute kidney injury in the intensive care unit: a prospective cohort study. Am J Kidney Dis. 2012;59(4):523–530.
- Harer MW, Pope CF, Conaway MR, et al. Follow-up of acute kidney injury in neonates during childhood years (FANCY): a prospective cohort study. Pediatr Nephrol. 2017;32(6):1067–1076.
- Menon S, Kirkendall ES, Nguyen H, et al. Acute kidney injury associated with high nephrotoxic medication exposure leads to chronic kidney disease after 6 months. J Pediatr. 2014;165(3):522–527.e522.
- White SL, Perkovic V, Cass A, et al. Is low birth weight an antecedent of CKD in later life? A systematic review of observational studies. Am J Kidney Dis. 2009;54(2):248–261.
- Khalsa DD, Beydoun HA, Carmody JB. Prevalence of chronic kidney disease risk factors among low birth weight adolescents. Pediatr Nephrol. 2016;31(9):1509–1516.
- Zaffanello M, Brugnara M, Bruno C, et al. Renal function and volume of infants born with a very low birth-weight: a preliminary cross-sectional study. Acta Paediatr. 2010;99(8):1192–1198.
- Iacobelli S, Loprieno S, Bonsante F, et al. Renal function in early childhood in very low birthweight infants. Am J Perinatol. 2007;24(10):587–592.
- Hirano D, Ishikura K, Uemura O, et al. Association between low birth weight and childhood-onset chronic kidney disease in Japan: a combined analysis of a nationwide survey for paediatric chronic kidney disease and the national vital statistics report. Nephrol Dial Transplant. 2016;31(11):1895–1900.
- Abitbol CL, Bauer CR, Montané B, et al. Long-term follow-up of extremely low birth weight infants with neonatal renal failure. Pediatr Nephrol. 2003;18(9):887–893.
- Cooper DS, Claes D, Goldstein SL, et al. Follow-up renal assessment of injury long-term after acute kidney injury (FRAIL-AKI). Clin J Am Soc Nephrol. 2016;11(1):21–29.
- Flynn JT, Kaelber DC, Baker-Smith CM, et al. Clinical practice guideline for screening and management of high blood pressure in children and adolescents. Pediatrics. 2017;140(3):140.)
- Pierce CB, Muñoz A, Ng DK, et al. Age- and sex-dependent clinical equations to estimate glomerular filtration rates in children and young adults with chronic kidney disease. Kidney Int. 2021;99(4):948–956.
- Zeisberg M, Neilson EG. Mechanisms of tubulointerstitial fibrosis. J Am Soc Nephrol. 2010;21(11):1819–1834.
- Ichimura T, Bonventre JV, Bailly V, et al. Kidney injury molecule-1 (KIM-1), a putative epithelial cell adhesion molecule containing a novel immunoglobulin domain, is up-regulated in renal cells after injury. J Biol Chem. 1998;273(7):4135–4142.
- Ichimura T, Asseldonk EJ, Humphreys BD, et al. Kidney injury molecule-1 is a phosphatidylserine receptor that confers a phagocytic phenotype on epithelial cells. J Clin Invest. 2008;118(5):1657–1668.
- Bonventre JV, Yang L. Kidney injury molecule-1. Curr Opin Crit Care. 2010;16(6):556–561.
- Fazel M, Sarveazad A, Mohamed Ali K, et al. Accuracy of urine kidney injury molecule-1 in predicting acute kidney injury in children; a systematic review and meta-analysis. Arch Acad Emerg Med. 2020;8(1):e44.
- Ko GJ, Grigoryev DN, Linfert D, et al. Transcriptional analysis of kidneys during repair from AKI reveals possible roles for NGAL and KIM-1 as biomarkers of AKI-to-CKD transition. Am J Physiol Renal Physiol. 2010;298(6):F1472–1483.
- Greenberg JH, Abraham AG, Xu Y, et al. Urine biomarkers of kidney tubule health, injury, and inflammation are associated with progression of CKD in children. J Am Soc Nephrol. 2021;32(10):2664–2677.
- van Timmeren MM, Vaidya VS, van Ree RM, et al. High urinary excretion of kidney injury molecule-1 is an independent predictor of graft loss in renal transplant recipients. Transplantation. 2007;84(12):1625–1630.
- Kyhse-Andersen J, Schmidt C, Nordin G, et al. Serum cystatin C, determined by a rapid, automated particle-enhanced turbidimetric method, is a better marker than serum creatinine for glomerular filtration rate. Clin Chem. 1994;40(10):1921–1926.
- Luxton RW, Patel P, Keir G, et al. A micro-method for measuring total protein in cerebrospinal fluid by using benzethonium chloride in microtiter plate wells. Clin Chem. 1989;35(8):1731–1734.
- Chaturvedi S, Farmer T, Kapke GF. Assay validation for KIM-1: human urinary renal dysfunction biomarker. Int J Biol Sci. 2009;5(2):128–134.